- 1Department of Occupational Health Science, School of Mechanical Engineering and Safety Engineering, University of Wuppertal, Wuppertal, Germany
- 2Department of Immunology, Leibniz Research Centre for Working Environment and Human Factors (IfADo), Dortmund, Germany
Heat acclimation (HA) is an essential modifier of physiological strain when working or exercising in the heat. It is unknown whether HA influences the increase of energy expenditure (Q10 effect) or heart rate (thermal cardiac reactivity TCR) due to increased body temperature. Therefore, we studied these effects using a heat strain database of climatic chamber experiments performed by five semi-nude young males in either non-acclimated or acclimated state. Measured oxygen consumption rate (VO2), heart rate (HR), and rectal temperature (Tre) averaged over the third hour of exposure were obtained from 273 trials in total. While workload (walking 4 km/h on level) was constant, heat stress conditions varied widely with air temperature 25–55°C, vapor pressure 0.5–5.3 kPa, and air velocity 0.3–2 m/s. HA was induced by repeated heat exposures over a minimum of 3 weeks. Non-acclimated experiments took place in wintertime with a maximum of two exposures per week. The influence of Tre and HA on VO2 and HR was analyzed separately with mixed model ANCOVA. Rising Tre significantly (p < 0.01) increased both VO2 (by about 7% per degree increase of Tre) and HR (by 39–41 bpm per degree Tre); neither slope nor intercept depended significantly on HA (p > 0.4). The effects of Tre in this study agree with former outcomes for VO2 (7%/°C increase corresponding to Q10 = 2) and for HR (TCR of 33 bpm/°C in ISO 9886). Our results indicate that both relations are independent of HA with implications for heat stress assessment at workplaces and for modeling heat balance.
Introduction
Heat acclimation (HA) refers to adaptations of physiological functions to repeated exposures to heat stress enhancing the tolerance to that stressor and, thus, reducing physiological strain (Taylor, 2014). This manifests, among others, in increased sweat rates accompanied by reduced rates of energy expenditure, heart rates, and body temperatures when exercising in the heat with relevance in military (Sawka et al., 2011), occupational (Strydom et al., 1966; Kampmann, 2000), or sports context (Garrett et al., 2011; Périard et al., 2015).
The above-mentioned heat strain indicators are interlinked, e.g., by the well-known temperature dependency of the rates of chemical and physiological processes (van’t Hoff, 1884), which is conveniently described as Q10 coefficient, defined as “the ratio of the rate of a physiological process at a particular temperature to the rate at a temperature 10°C lower” (IUPS Thermal Commission, 2003). Using oxygen uptake rate (VO2) as indicator of metabolic rate and rectal temperature (Tre) characterizing body temperature, this is mathematically expressed as (Chaui-Berlinck et al., 2002):
VO2,ref refers to the oxygen uptake rate at a reference rectal temperature, e.g., Tre,ref = 36.8°C. Re-arranging Eq. 1, it expresses percentage change in oxygen uptake rate (%VO2) due to a change in Tre (ΔTre) as follows (Bröde and Kampmann, 2019):
Q10 coefficients for biological systems typically vary between 2 and 3 (Chaui-Berlinck et al., 2002; IUPS Thermal Commission, 2003; Seebacher et al., 2015), with relevance not only during hyperthermia (Nadel et al., 1971; Howells et al., 2013), but also during body cooling (Erecinska et al., 2003). Furthermore, the setting Q10 = 2 is applied in human thermoregulation models (Werner and Buse, 1988; Fiala et al., 2012). A recent study on the influence of core temperature on oxygen uptake with 11 young acclimated males (Kampmann and Bröde, 2015) confirmed this with Q10 = 2.1 on average, corresponding to a 7% increase in VO2 per degree rise in Tre according to Eq. 2. However, there was large inter-individual variation from Q10 = 1, i.e., no increase in VO2 due to Tre, to Q10 = 8, corresponding to 23% VO2 increase per degree rise in Tre.
There are reports on decreased Q10 after acclimation to heat indicating a reduced sensitivity of metabolic rates to increasing environmental temperature in ectotherms (Sandblom et al., 2014; Seebacher et al., 2015). Aiming at a comparative human study related to body temperature, we would like to extend the preceding works and analyze “whole organism” Q10 effects with acclimated compared to non-acclimated participants.
Body temperature also influences heart rate (HR) with a typical increase of 30–40 bpm per degree rise in Tre (Vogt et al., 1973; Kuhlemeier and Miller, 1978; Kampmann, 2000; ISO 9886, 2004; Bröde and Kampmann, 2019). This increase is termed “thermal cardiac reactivity” (TCR), and also “thermal pulses” (Kampmann et al., 2001) or “thermal heart rate component” (ISO 8996, 2004; Dubé et al., 2019), and shows considerable inter-individual variation between 16 and 60 bpm/°C (Kampmann, 2000; Bröde and Kampmann, 2019). An earlier study (Kuhlemeier and Miller, 1978) estimated TCR from pooled intra- and inter-individual data under different workloads of workers classified in “hot” and “cold-neutral” professions during summer and winter months, thus considering “natural” acclimation effects. The authors reported 6–7 bpm lower HR in summer compared to winter, and a 5–6 bpm reduction in HR in “hot” professions compared to the reference group, but did not allow for changes in the slope, i.e., TCR, depending on acclimation in their analyses, which were performed using the estimated overall value of 29 bpm/°C. Thus, it is unclear, whether acclimation changes TCR.
Q10 and TCR are relevant for the assessment of thermal stress and strain in different fields of application, e.g., as a potential source of error when estimating metabolic rate from heart rate measurements (ISO 8996, 2004; Malchaire et al., 2017). Here, TCR may induce an overestimation bias (Bröde and Kampmann, 2019) requiring dedicated correction procedures (Vogt et al., 1973; Kampmann et al., 2001; Dubé et al., 2019). Q10 also helps to explain the reduced cycling gross efficiency observed with increasing body temperature (Daanen et al., 2006).
Recently, Q10 and TCR were explicitly and implicitly applied for the non-invasive determination of core temperature from peripheral signals including heart rate, sometimes also involving the estimation of metabolic rate. Algorithms have been developed typically for work in protective clothing in industry (Richmond et al., 2015), firefighting (Kim, 2018), and military scenarios (Buller et al., 2013; Welles et al., 2018; Hunt et al., 2019).
For those applications, it is important to know whether the underlying algorithms will require adjustments considering the heat acclimation state of the individual. A recent pooled analysis (Bröde et al., 2009) of the changes in Tre (ΔTre) and HR (ΔHR) after 5 days of short-term HA observed in 23 females and 34 males showed a significant positive correlation with ΔHR increasing with ΔTre by 32.6 bpm/°C, close to the 33 bpm/°C reported for TCR in international standards (ISO 9886, 2004). Thus, TCR may have a role in explaining the effects of HA. However, it is unknown whether Q10 or TCR will depend on HA.
Therefore, the aim of this research was to study the influence of HA on Q10 and TCR using an extensive heat strain database compiled from controlled climatic chamber experiments.
Materials and Methods
Heat Strain Database
We used a heat strain database of climate chamber experiments conducted previously at IfADo (Wenzel et al., 1989; Kampmann, 2000) according to the ethical principles of the Declaration of Helsinki with approval by IfADo’s local Ethics Committee. Figure 1 illustrates the recordings of rectal temperature (Tre) and heart rate (HR) for a typical heat stress exposure.
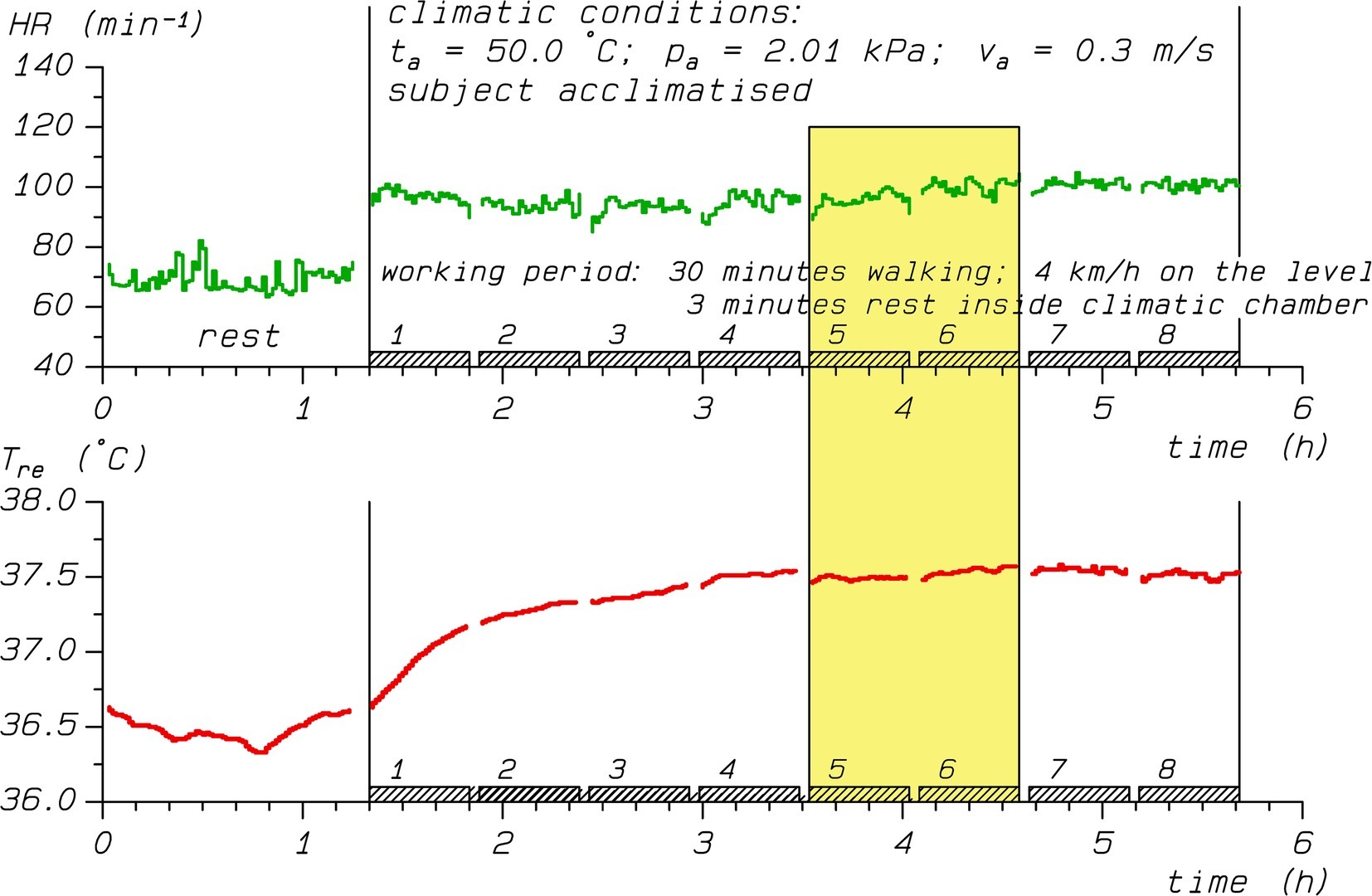
Figure 1. Time course of heart rate (HR, upper panel) and rectal temperature (Tre, lower panel) during a heat stress experiment. The yellow shaded area marks the time interval with averaged values of HR = 98 bpm and Tre = 37.5°C used for analyses, while VO2 (not shown) was 714 mL/min.
We searched our database for individuals having performed series of experiments in both non-acclimated (HA0) and acclimated (HA1) states. Inclusion criteria were a minimum number of 15 experiments per series with comparable workload and clothing in order to determine Q10 and TCR on an individual level. We retrieved 273 trials organized in 10 series, which originated from five semi-nude young fit males in either HA0 or HA1 state. The number of experiments in each series varied depending on acclimation state and individual between 15 and 47 experiments, with total figures of 118 trials for HA0 and 155 for HA1. The personal characteristics (mean ± SD) of the participants were 20.2 ± 0.8 years of age, 1.84 ± 0.02 m of body height, 71.4 ± 7.5 kg of body weight, 1.9 ± 0.1 m2 of body surface area, and 47.1 ± 9.8 mL/min/kg of peak rate of oxygen uptake.
As the procedures have been described in detail elsewhere (Kampmann, 2000), they are only briefly summarized here. Each trial consisted of treadmill work with constant workload of walking 4 km/h on the level for at least 3 h organized in 30 min work periods interrupted by 3 min breaks for determining body weight loss (Figure 1). The participants were exposed to varying levels of heat stress with conditions characterized by different combinations of air temperature (range 25–55°C), water vapor pressure (0.5–5.3 kPa), and air velocity (0.3–2.0 m/s). Mean radiant temperature was equal to air temperature.
Rectal temperatures were recorded continuously using a thermistor probe (YSI 401, Yellow Springs) inserted 10 cm past the anal sphincter, as well as heart rates, which were obtained using ECG electrodes. Tre and HR were stored as 1-min averages, and means calculated over the third hour of exposure were used for further analyses (Figure 1). They were matched to oxygen uptake rates (VO2) obtained toward the end of the third hour of exposure by collecting the expired air with Douglas bags (Douglas, 1911). We determined the oxygen and carbon dioxide concentrations with a paramagnetic gas analyzer (Servomex) and infrared analyzer (UNOR Mark 2), respectively. The VO2 calculations based on the Haldane transformation (Poole and Whipp, 1988) are detailed in Rutenfranz and Wenzel (1980), while the methods were historically reviewed recently (Shephard, 2017).
Heat Acclimation Protocol
HA was induced by repeated experiments in warm-humid climates (air temperature 38–40°C with 65–70% relative humidity) over 3–4 weeks in a way that the subjects could sustain 3 h of heat exposure reaching a Tre of 38.5°C. To counteract a decay in acclimation over the weekend (Daanen et al., 2018), HA was re-established on Mondays and measurements for the series started the day after.
Non-acclimated exposures took place in wintertime in order to avoid seasonal adaptation, and with a maximum of two exposures per week on non-consecutive days to prevent short-term HA effects.
Data Analysis and Statistics
Statistical analysis was performed using R version 3.6.1 (R Core Team, 2019). The influence of Tre, which was centered to a reference value of 36.8°C, and HA on VO2 and HR was analyzed separately with linear mixed model ANCOVA (Bates et al., 2015). The models included random intercepts and Tre slopes for individuals nested within acclimation status with tests for statistical significance carried out applying Kenward-Roger approximations for denominator degrees of freedom (Kuznetsova et al., 2017).
Results
Figure 2 illustrates the influence of Tre on HR (Figure 2A) and VO2 (Figure 2B), respectively. While the information for Tre and HR was almost complete (only 5 missing HR values), 34 VO2 observations were missing, the majority (24) for ID5 in HA1 due to a defect in the O2 analyzer requiring repairing while the series of exposures had to be continued. Nevertheless, linear regression lines showed positive correlations with Tre for both dependent variables in each series.
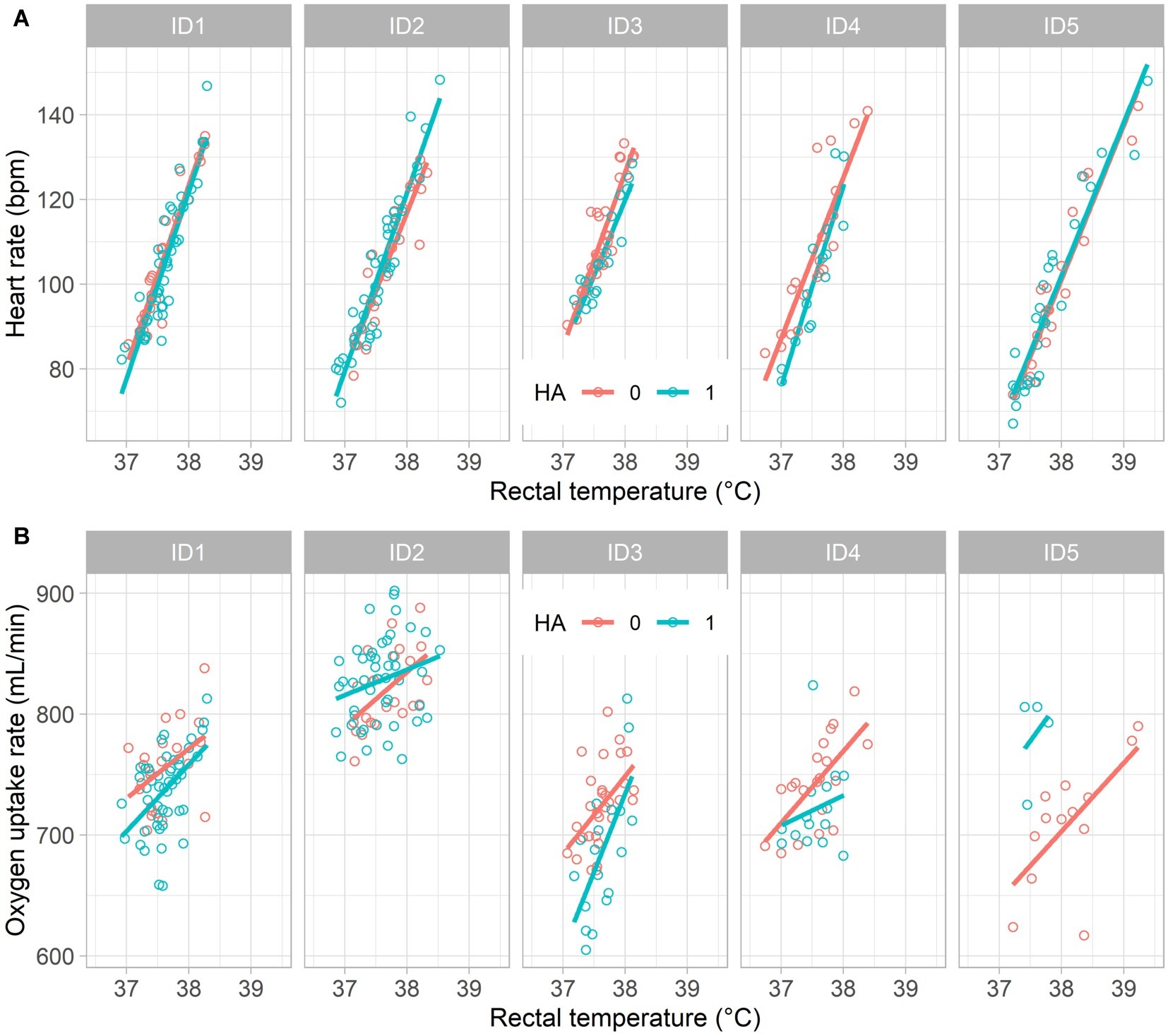
Figure 2. Measured values and linear regression lines illustrating the influence of rectal temperature on (A) heart rate (thermal cardiac reactivity) and on (B) oxygen uptake rate (Q10 effect) for five participants (ID1–ID5) in non-acclimated (HA0) and acclimated (HA1) states, respectively.
The parameter estimates from the statistical analysis (Table 1) indicate that on average HR rose from 72 bpm at reference Tre = 36.8°C by 39 bpm per degree increase in Tre, i.e. TCR was 39 bpm/°C for non-acclimated individuals. When acclimated, the intercept was reduced by 4 bpm, while TCR slightly increased to 41 bpm/°C. However, while the TCR effect was highly statistically significant (p < 0.0001), adjustments due to HA to both intercept and slope were non-significant (p > 0.4).
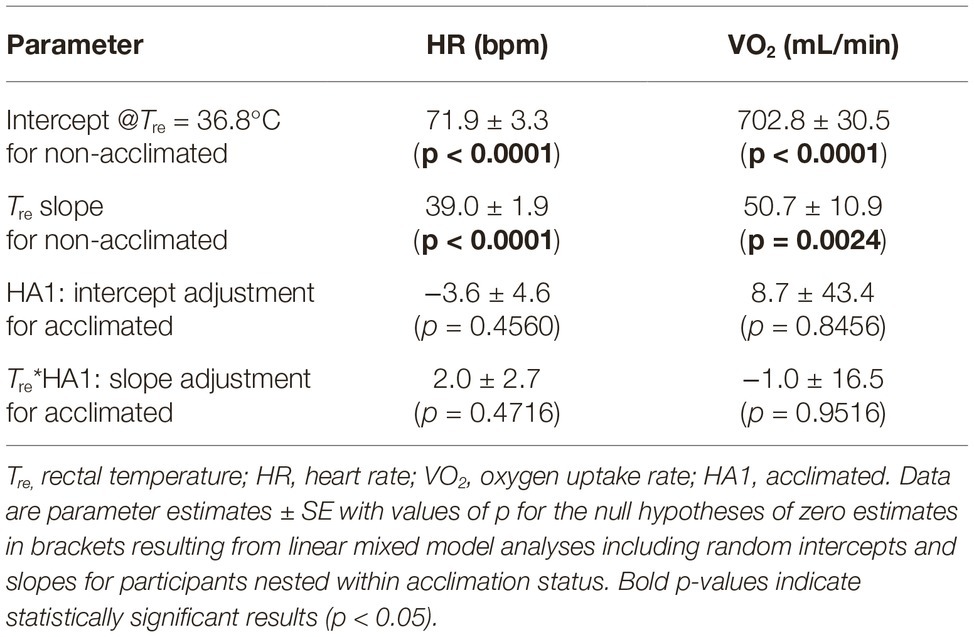
Table 1. Mixed effects ANCOVA results for the influence of Tre, centered to a reference value of 36.8°C, and heat acclimation (HA) on HR (thermal cardiac reactivity) and on VO2 (Q10 effect).
Similar to TCR, rising Tre also significantly (p < 0.01) increased VO2 by about 7% per degree increase of Tre compared to the reference VO2 at Tre = 36.8°C for both HA0 and HA1; neither slope (i.e., Q10) nor intercept depended significantly on HA (p > 0.8).
Discussion
Our results regarding the impact of Tre on HR conform with reports of TCR between 30 and 40 bpm/°C in previous studies (Vogt et al., 1973; Kuhlemeier and Miller, 1978; Bröde and Kampmann, 2019) and in ISO 9886 (2004). They also agree with former effect sizes for VO2, as the observed increase of 7%/°C corresponds to a Q10 coefficient around 2, which were reported as mean value in human trials (Kampmann and Bröde, 2015) and used in advanced models of human thermoregulation (Werner and Buse, 1988; Fiala et al., 2012).
A novel finding of our study was that heat acclimation did neither modify thermal cardiac reactivity nor influence Q10.
In contrast, a Q10 decrease after acclimation to warm conditions was reported for ectotherms and interpreted as lowered sensitivity to increasing environmental temperatures under climate change scenarios (Sandblom et al., 2014). However, those lowered Q10 were calculated across states of acclimation presuming that acclimation will shift the otherwise unchanged temperature-response function to the right (Seebacher et al., 2015). The latter would conform to the invariance regarding heat acclimation of the intra-individually determined Q10 in our study. On the other hand, the shift of the intercept observed in Table 1 was minimal and non-significant.
There are limitations within this study that only used observations retrieved from an existing database of semi-nude fit young males performing light to moderate work. It would be worthwhile to verify our results involving other populations, e.g., females or elderly, under higher activity levels or working in protective clothing. Future studies might further include heart rate variability (HRV) measurements quantifying the sympathetic and vagal impacts on HR. Earlier studies had indicated vagal dominance following HA (Flouris et al., 2014), and negative correlations of vagal tone with Tre and HR (Brenner et al., 1997). However, as HRV calculations require beat-to-beat (RR) intervals, we could not perform these analyses with our aggregated HR data.
Nevertheless, our analyses of 273 experiments indicate that intra-individually determined Q10 and TCR remain unaltered following heat acclimation. This stability could have implications for the development and application of methods using the Q10 and TCR relationships for the heat stress assessment at workplaces (Malchaire et al., 2017; Bröde and Kampmann, 2019), and for the modeling of heat balance, e.g., for predicting core temperature from non-invasive signals when working with protective clothing in industrial, military, or firefighting operations (Richmond et al., 2015; Kim, 2018; Welles et al., 2018).
Data Availability Statement
The raw data including the R scripts used for the analyses supporting the conclusions of this article are provided as Supplementary Material to this article.
Ethics Statement
The studies involving human participants were reviewed and approved by IfADo’s Local Ethics Commission. The patients/participants provided their written informed consent to participate in this study.
Author Contributions
BK and PB designed and conceived the analyses. BK collected the data. PB organized the database and performed the statistical analysis. Both authors interpreted the data, and wrote the manuscript, and, after critically reviewing and providing significant editing of its content, approved the final article.
Funding
The publication of this article was funded by the Open Access Fund of the Leibniz Association.
Conflict of Interest
The authors declare that the research was conducted in the absence of any commercial or financial relationships that could be construed as a potential conflict of interest.
Acknowledgments
The authors gratefully acknowledge the members of IfADo’s former department “Environmental Physiology” for skillfully running the experiments.
Supplementary Material
The Supplementary Material for this article can be found online at: https://www.frontiersin.org/articles/10.3389/fphys.2019.01524/full#supplementary-material
References
Bates, D., Mächler, M., Bolker, B., and Walker, S. (2015). Fitting linear mixed-effects models using lme4. J. Stat. Softw. 67, 1–48. doi: 10.18637/jss.v067.i01
Brenner, I. K. M., Thomas, S., and Shephard, R. J. (1997). Spectral analysis of heart rate variability during heat exposure and repeated exercise. Eur. J. Appl. Physiol. 76, 145–156. doi: 10.1007/s004210050227
Bröde, P., and Kampmann, B. (2019). Accuracy of metabolic rate estimates from heart rate under heat stress—an empirical validation study concerning ISO 8996. Ind. Health 57, 615–620. doi: 10.2486/indhealth.2018-0204
Bröde, P., Schütte, M., Kampmann, B., and Griefahn, B. (2009). Heat acclimation and its relation to resting core temperature and heart rate. Occup. Ergon. 8, 185–193. doi: 10.3233/OER-2009-0168
Buller, M. J., Tharion, W. J., Cheuvront, S. N., Montain, S. J., Kenefick, R. W., Castellani, J., et al. (2013). Estimation of human core temperature from sequential heart rate observations. Physiol. Meas. 34, 781–798. doi: 10.1088/0967-3334/34/7/781
Chaui-Berlinck, J. G., Monteiro, L. H. A., Navas, C. A., and Bicudo, J. E. P. W. (2002). Temperature effects on energy metabolism: a dynamic system analysis. Proc. R. Soc. Lond. Ser. B Biol. Sci. 269, 15–19. doi: 10.1098/rspb.2001.1845
Daanen, H. A. M., Racinais, S., and Périard, J. D. (2018). Heat acclimation decay and re-induction: a systematic review and meta-analysis. Sports Med. 48, 409–430. doi: 10.1007/s40279-017-0808-x
Daanen, H. A., van Es, E. M., and de Graaf, J. L. (2006). Heat strain and gross efficiency during endurance exercise after lower, upper, or whole body precooling in the heat. Int. J. Sports Med. 27, 379–388. doi: 10.1055/s-2005-865746
Douglas, C. G. (1911). A method for determining the total respiratory exchange in man. J. Physiol. 42(Suppl), xvii–xxii. doi: 10.1113/jphysiol.1911.sp001452
Dubé, P.-A., Imbeau, D., Dubeau, D., and Auger, I. (2019). Worker heat stress prevention and work metabolism estimation: comparing two assessment methods of the heart rate thermal component. Ergonomics 62, 1066–1085.doi: 10.1080/00140139.2019.1588386
Erecinska, M., Thoresen, M., and Silver, I. A. (2003). Effects of hypothermia on energy metabolism in mammalian central nervous system. J. Cereb. Blood Flow Metab. 23, 513–530. doi: 10.1097/01.Wcb.0000066287.21705.21
Fiala, D., Havenith, G., Bröde, P., Kampmann, B., and Jendritzky, G. (2012). UTCI-Fiala multi-node model of human heat transfer and temperature regulation. Int. J. Biometeorol. 56, 429–441. doi: 10.1007/s00484-011-0424-7
Flouris, A. D., Poirier, M. P., Bravi, A., Wright-Beatty, H. E., Herry, C., Seely, A. J., et al. (2014). Changes in heart rate variability during the induction and decay of heat acclimation. Eur. J. Appl. Physiol. 114, 2119–2128. doi: 10.1007/s00421-014-2935-5
Garrett, A., Creasy, R., Rehrer, N., Patterson, M., and Cotter, J. (2011). Effectiveness of short-term heat acclimation for highly trained athletes. Eur. J. Appl. Physiol. 112, 1827–1837. doi: 10.1007/s00421-011-2153-3
Howells, J., Czesnik, D., Trevillion, L., and Burke, D. (2013). Excitability and the safety margin in human axons during hyperthermia. J. Physiol. 591, 3063–3080. doi: 10.1113/jphysiol.2012.249060
Hunt, A. P., Buller, M. J., Maley, M. J., Costello, J. T., and Stewart, I. B. (2019). Validity of a noninvasive estimation of deep body temperature when wearing personal protective equipment during exercise and recovery. Mil. Med. Res. 6:20. doi: 10.1186/s40779-019-0208-7
ISO 8996 (2004). Ergonomics of the thermal environment - determination of metabolic rate. Geneva: International Organisation for Standardisation.
ISO 9886 (2004). Ergonomics - evaluation of thermal strain by physiological measurements. Geneva: International Organisation for Standardisation.
IUPS Thermal Commission (2003). Glossary of terms for thermal physiology. J. Therm. Biol. 28, 75–106. doi: 10.1016/S0306-4565(02)00055-4
Kampmann, B. (2000). Zur Physiologie der Arbeit in warmem Klima. Ergebnisse aus Laboruntersuchungen und aus Feldstudien im Steinkohlenbergbau. Habilitation thesis. Bergische Universität Wuppertal. Available at: http://elpub.bib.uni-wuppertal.de/servlets/DerivateServlet/Derivate-735/h140001.pdf (Accessed December 10, 2019).
Kampmann, B., and Bröde, P. (2015). Metabolic costs of physiological heat stress responses - Q10 coefficients relating oxygen consumption to body temperature. Extrem. Physiol. Med. 4(Suppl. 1):A103. doi: 10.1186/2046-7648-4-S1-A103
Kampmann, B., Kalkowsky, B., and Piekarski, C. (2001). Estimation of metabolic rate from cardiac frequency for field studies: correcting for “thermal pulses”. J. Therm. Biol. 26, 437–440. doi: 10.1016/S0306-4565(01)00057-2
Kim, S. (2018). Prediction of heat strain by heart rate for firefighters in protective clothing. PhD Thesis, Seoul National University. Available at: http://hdl.handle.net/10371/143235 (Accessed December 10, 2019).
Kuhlemeier, K. V., and Miller, J. M. (1978). Pulse rate-rectal temperature relationships during prolonged work. J. Appl. Physiol. 44, 450–454. doi: 10.1152/jappl.1978.44.3.450
Kuznetsova, A., Brockhoff, P. B., and Christensen, R. H. B. (2017). lmerTest package: tests in linear mixed effects models. J. Stat. Softw. 82, 1–26. doi: 10.18637/jss.v082.i13
Malchaire, J., d’Ambrosio Alfano, F. R., and Palella, B. I. (2017). Evaluation of the metabolic rate based on the recording of the heart rate. Ind. Health 55, 219–232. doi: 10.2486/indhealth.2016-0177
Nadel, E. R., Bullard, R. W., and Stolwijk, J. A. (1971). Importance of skin temperature in the regulation of sweating. J. Appl. Physiol. 31, 80–87.
Périard, J. D., Racinais, S., and Sawka, M. N. (2015). Adaptations and mechanisms of human heat acclimation: applications for competitive athletes and sports. Scand. J. Med. Sci. Sports 25, 20–38. doi: 10.1111/sms.12408
R Core Team (2019). R: A language and environment for statistical computing. Vienna, Austria: R Foundation for Statistical Computing. Available at: https://www.R-project.org/ (Accessed December 10, 2019).
Richmond, V. L., Davey, S., Griggs, K., and Havenith, G. (2015). Prediction of core body temperature from multiple variables. Ann. Occup. Hyg. 59, 1168–1178. doi: 10.1093/annhyg/mev054
Rutenfranz, J., and Wenzel, H. G. (1980). “Energiehaushalt” in Biochemie und Physiologie der Ernährung (Ernährungslehre und Diätetik, Bd. 1, Teil 1). eds. H.-D. Cremer, D. Hötzel, and J. Kühnau (Stuttgart: Thieme), 240–256.
Sandblom, E., Gräns, A., Axelsson, M., and Seth, H. (2014). Temperature acclimation rate of aerobic scope and feeding metabolism in fishes: implications in a thermally extreme future. Proc. R. Soc. B Biol. Sci. 281:20141490. doi: 10.1098/rspb.2014.1490
Sawka, M. N., Leon, L. R., Montain, S. J., and Sonna, L. A. (2011). Integrated physiological mechanisms of exercise performance, adaptation, and maladaptation to heat stress. Compr. Physiol. 1, 1883–1928. doi: 10.1002/cphy.c100082
Seebacher, F., White, C. R., and Franklin, C. E. (2015). Physiological plasticity increases resilience of ectothermic animals to climate change. Nat. Clim. Chang. 5, 61–66. doi: 10.1038/nclimate2457
Shephard, R. J. (2017). Open-circuit respirometry: a brief historical review of the use of Douglas bags and chemical analyzers. Eur. J. Appl. Physiol. 117, 381–387. doi: 10.1007/s00421-017-3556-6
Strydom, N. B., Wyndham, C. H., Williams, C. G., Morrison, J. F., Bredell, G. A., Benade, A. J., et al. (1966). Acclimatization to humid heat and the role of physical conditioning. J. Appl. Physiol. 21, 636–642. doi: 10.1152/jappl.1966.21.2.636
Taylor, N. A. S. (2014). Human heat adaptation. Compr. Physiol. 4, 325–365. doi: 10.1002/cphy.c130022
van’t Hoff, M. J. H. (1884). Etudes de dynamique chimique. Rec. Trav. Chim. Pays Bas 3, 333–336. doi: 10.1002/recl.18840031003
Vogt, J. J., Meyer-Schwertz, M. T., Metz, B., and Foehr, R. (1973). Motor, thermal and sensory factors in heart rate variation: a methodology for indirect estimation of intermittent muscular work and environmental heat loads. Ergonomics 16, 45–60. doi: 10.1080/00140137308924481
Welles, A. P., Buller, M. J., Looney, D. P., Rumpler, W. V., Gribok, A. V., and Hoyt, R. W. (2018). Estimation of metabolic energy expenditure from core temperature using a human thermoregulatory model. J. Therm. Biol. 72, 44–52. doi: 10.1016/j.jtherbio.2017.12.007
Wenzel, H. G., Mehnert, C., and Schwarzenau, P. (1989). Evaluation of tolerance limits for humans under heat stress and the problems involved. Scand. J. Work Environ. Health 15(Suppl. 1), 7–14.
Keywords: heat acclimation, metabolic rate, heart rate, body temperature, rectal temperature, Q10 coefficient, heat strain, heat stress
Citation: Kampmann B and Bröde P (2019) Heat Acclimation Does Not Modify Q10 and Thermal Cardiac Reactivity. Front. Physiol. 10:1524. doi: 10.3389/fphys.2019.01524
Edited by:
Andrew T. Garrett, University of Hull, United KingdomReviewed by:
Michal Horowitz, Hebrew University of Jerusalem, IsraelFabien Andre Basset, Memorial University of Newfoundland, Canada
Copyright © 2019 Kampmann and Bröde. This is an open-access article distributed under the terms of the Creative Commons Attribution License (CC BY). The use, distribution or reproduction in other forums is permitted, provided the original author(s) and the copyright owner(s) are credited and that the original publication in this journal is cited, in accordance with accepted academic practice. No use, distribution or reproduction is permitted which does not comply with these terms.
*Correspondence: Peter Bröde, YnJvZWRlQGlmYWRvLmRl