- 1School of Molecular and Life Sciences, Curtin University, Perth, WA, Australia
- 2Department of Biological Sciences, Macquarie University, Sydney, NSW, Australia
- 3School of Biological Sciences, University of Western Australia, Perth, WA, Australia
- 4School of Biological, Earth, and Environmental Sciences, University of New South Wales Sydney, Sydney, NSW, Australia
Global environmental change is leading to an increase in the frequency, intensity, and duration of extreme weather events, so effective environmental management requires an understanding not only of the physiological response of organisms to increased mean temperatures, but also to extreme environmental conditions. To determine the physiological consequences of heatwaves on energy and water balance of arid-adapted zebra finches (Taeniopygia guttata), we measured field metabolic rate and water turnover rate of wild, free-living finches during a heatwave (consecutive days of maximum ambient temperature of 40–45°C) and during a cooler period (maximum ambient temperature of 28°C) during a summer drought. To understand how birds accommodated their energy and water requirements, we also monitored feeding and drinking behavior of zebra finches at the study site on hot and cold days over 2.5 months during the same summer. Zebra finches can accommodate heatwaves without major impacts on field energy or water turnover, even when the heatwave is superimposed on high summer temperatures and long-term drought, so long as drinking water is available. In fact, cooler periods may pose a greater energetic challenge than heatwaves during drought, when food availability is limited, due to the increased thermoregulatory cost of maintaining a high body temperature against a thermal gradient. Zebra finches avoided or limited activity during the most thermally challenging periods of the day. Their pre-emptive feeding and drinking in preparation for hours of relative inactivity at high ambient temperature, together with a high body water content and reduced midday activity and metabolic heat production, enabled zebra finches to maintain body mass during a heatwave. Predicting upcoming periods of unfavorably high ambient temperature, together with a high body water content, may be essential for survival by desert birds of extreme ambient temperature during heatwaves.
Introduction
Global environmental change is not only impacting average climatic conditions such as mean ambient temperature and annual rainfall but is also increasing the intensity, frequency, and duration of extreme weather events such as heatwaves (Meehl and Tebaldi, 2004; Tebaldi et al., 2006; Diffenbaugh and Field, 2013; IPCC, 2014). These extreme weather events may have a significant, immediate, and long-lasting impact on biodiversity, especially when combined with an underlying gradual mean change in climate (Saunders et al., 2011; Harris et al., 2018; Ruthrof et al., 2018). How animals respond to extreme environmental conditions provides exemplary insights into animal function as it is under these conditions that physiological processes must be most highly refined for survival. Therefore studying species during these extreme events will provide essential basic information about the physiological ecology of species. In addition, attempts to limit biodiversity loss by managing species in these changing conditions require a detailed mechanistic understanding of the physiological response of organisms to environmental variability (Harris et al., 2018; Conradie et al., 2019). However, our current understanding of the physiological function of most organisms is inadequate to determine effective mitigation strategies for even background climate change, and is even less able to predict and manage their response to unpredictable, extreme weather events (Chown et al., 2010; Ratnayake et al., 2019).
Birds are particularly susceptible to extreme weather events (Albright et al., 2017). They have a small body mass, are generally diurnally active and restricted to surface microhabitats, have high mass-specific energy and water requirements and a high body temperature (Tb) that is close to the lethal maximum for endotherms (Kendeigh, 1969; Withers et al., 2004; Williams and Tieleman, 2005). Mass mortality as a consequence of extreme heatwaves has been reported for various avian species, even iconic desert species such as budgerigars (Melopsittacus undulatus) and zebra finches (Taeniopygia guttata; Finlayson, 1932; McKechnie and Wolf, 2010; Saunders et al., 2011; McKechnie et al., 2012; Birdlife Australia, 2014), which inhabit naturally harsh, unpredictable arid environments. If these mortality events impact on uncommon or range-restricted species, or occur at a sufficiently large scale or frequency, they can have substantial impacts on the long-term viability of populations and even species (Saunders et al., 2011; Franklin et al., 2014; Ruthrof et al., 2018). For example, a sub-continental heatwave in Western Australia during 2011 was implicated in wide-spread abrupt and synchronized biotic effects in marine and terrestrial environments (Ruthrof et al., 2018), including reduced chick production and increased mortality of breeding little penguins (Eudyptula minor; Cannell et al., 2012). On a single day of extreme high temperature in southern Western Australia during 2010, at least 208 endangered Carnaby’s cockatoos (Calyptorhynchus latirostris) were killed, with likely further deaths of orphaned chicks (Saunders et al., 2011).
During extreme heatwaves, terrestrial birds are impacted by the immediate trade-off between maintaining Tb at sub-lethal levels, typically <46°C (Kendeigh, 1969; Withers et al., 2004; Conradie et al., 2019; McKechnie and Wolf, 2019), using evaporative cooling, and fatal dehydration, generally assumed to be 11–15% body mass loss (Wolf, 2000; Albright et al., 2017). Birds can manipulate this trade-off by integration of physiological and behavioral responses, including reduction in activity and retreat to shaded, cooler microclimates, facultative hyperthermia, enhanced evaporative heat loss, and heat dissipation behaviors such as wing drooping and panting/gular fluttering (Wolf, 2000). However, these responses may affect a bird’s ability to replenish body water by drinking, and may also hamper maintaining energy balance, due to reduced foraging opportunities and efficiency (Smit et al., 2016; Conradie et al., 2019; Funghi et al., 2019). For example, southern pied babblers (Turdoides bicolor) were unable to maintain body mass on days with maximum ambient temperature (Ta) >35.5°C due to the impact of heat dissipation behavior on foraging efficiency (du Plessis et al., 2012). Balancing energy and especially water budgets with fatal hyperthermia is especially challenging for small birds during periods of extreme heat compared to larger species, as they have a smaller body water pool and energy store and consequently a shorter survival window, coupled with higher rates of environmental heat gain and high mass-specific evaporative water loss (EWL) and energy use (Smit et al., 2016).
Here we examine how short periods of extreme temperature, superimposed over longer term conditions of drought and high summer temperature, impact on the energy and water turnover of wild, free-living zebra finches in an arid habitat in central Australia. Zebra finches are small (10–13 g), granivorous, estrildid finches that inhabit much of the Australian arid zone. They are a widely used model for investigating the physiological and behavioral mechanisms by which desert birds survive and reproduce in desert environments (Zann, 1996). We measure field metabolic rate (FMR) and water turnover rate (WTR) of free-living birds during periods of extremely high Ta (>40°C) when maximum Ta exceeds the average diurnal passerine Tb (McKechnie et al., 2012; Albright et al., 2017), and comparatively milder Ta (maximum Ta ≤ 28°C). We compare these rates of energy and water turnover with diurnal patterns of foraging and drinking behavior, to provide information regarding the physiological and behavioral mechanisms that determine how extreme weather events may impact the survival and of small desert birds (McKechnie et al., 2012). We hypothesize that extreme heatwave conditions will reduce foraging opportunities, with a negative impact on energy turnover and significant body mass loss, and that water turnover and drinking rates will increase, during hot compared to cooler conditions.
Methods
Zebra finches were studied at Gap Hills (31°05′ S, 142°42′ E), Fowlers Gap Arid Zone Research Station, approximately 112 km north of Broken Hill, New South Wales, Australia. The study occurred during December, January, and February 2018, within a 2-year period of extended drought and above-average temperatures (Australian Bureau of Meteorology, 2018). Four feeders, consisting of a 50 cm × 30 cm seed tray of commercial finch seed placed inside a 70 cm × 40 cm × 50 cm wire cage with a drop door, were opened at the site approximately 2 months before the study. The only free water known to be available within at least 5 km of the empty dam at Gap Hills was provided at two small drinking troughs (about 800 m and 100 m from the dry dam), filled from enclosed plastic water tanks with a float valve, and protected from large mammals and emus by a mesh fence. In the 50 months leading up to the fieldwork, a total of 771 finches had been captured at the site and were tagged subcutaneously with a passive integrated transponder (PIT; Minichip; Micro Products Australia, Perth, Australia), as part of other studies (e.g., Brandl et al., 2018; Funghi et al., 2019). All individuals were also banded with an individually numbered metal leg band (size 02) supplied by the Australian Bird and Bat Banding Scheme (ABBBS). Mark-recapture estimates suggest that approximately 350 individual birds were resident at the site during the study (Cooper, personal observation), but these were not all PIT tagged.
Field metabolic rate and WTR were estimated using the doubly labeled water method (Nagy, 1983; Speakman, 1997) to measure rates of CO2 production and water flux for 10 wild, free-living zebra finches during a period of four consecutive days (18–21 January 2018) where maximum ambient temperature for the station exceeded 40°C (range 40–44.5°C; hot period), and for 10 finches during a period of 2 days (1–2 February 2018) when maximum ambient temperature was 27–27.6°C (cool period). The maximum temperature anomaly (deviation from the 30-year monthly average during 1961–1990; Australian Bureau of Meteorology) for these periods ranged from 4.4 to 8.3°C and −6.9 to −7.7°C respectively.
During the FMR and WTR measurements, only one feeder (and the two water tanks) remained open. A vehicle was used as a hide near the feeder, and finches were observed with binoculars and captured by the observer dropping the feeder door using a string trigger. Twenty finches were initially captured shortly after sunrise of the first day of the hot or cool period respectively. They were weighed to 0.1 g on an electronic balance, banded with both a uniquely numbered ABBBS metal band and a plastic color band, and given an IP injection of ~0.057 ml of water consisting of a 2:1 ratio of 98% enriched 18O and 2H; injection syringes were weighed before and after, to 0.0001 g. Finches were held in a cloth bag for approximately an hour (mean 1 h 9 min), after which an equilibration blood sample (~75 μl) was taken from the brachial vein before they were released. Ten finches in each experimental period were recaptured 18.7–71 h later by observing color-banded birds entering the feeder and triggering the drop door, and a recapture blood sample (~75 μl) was immediately taken. Blood samples (~75 μl) from six additional finches not involved in the turnover study were taken for analysis of background level of isotopes.
Blood samples were immediately flame-sealed on-site in the heparinized glass capillary tubes in which they were collected, using a butane burner. They were returned to the laboratory at the University of Western Australia, where water was extracted by vacuum distillation in flint glass pipettes. The enrichment of the injectate was calculated by diluting 0.061 g of the isotope solution into 7.56 g of water taken from the water troughs at the site. The solution was thoroughly mixed and samples before (background) and after (equilibration) addition of the injectate were also vacuum distilled and analyzed.
The stable isotope composition of distilled samples was analyzed by the West Australian Geochemistry Centre at the University of Western Australia (Skrzypek and Ford, 2014), using a Picarro L1115-I isotopic liquid water analyzer (Picarro, Santa Clara, California, USA). Prior to analyses, all samples were isotopically diluted with deionized water with a known isotope composition. The δ2H and δ18O raw values were normalized to the Vienna Standard Mean Ocean Water (VSMOW) scale after Skrzypek (2013), with three-point normalization replicated twice and calibrated against international standards provided by the International Atomic Energy Agency (Coplen, 1996). Body water content (BWC), water flux, and CO2 production were calculated from these values (converted to ppm), after Speakman (1997). WTR was calculated as rH2O = NHkD, where rH2O is the H2O turnover rate (mol h−1), NH is the average 2H dilution space (mol), and kD is the 2H turnover (h−1). FMR was calculated using Speakman’s (1997) revision (i) of the Lifson and McClintock (1966) method as rCO2 = NO(0.48123kO − 0.48743kD), where rCO2 is the CO2 turnover rate (mol h−1), NO is the average 18O dilution space, and kO is the 18O turnover (h−1).
The mean of the six background values was used in calculations for all birds. For five birds, for which equilibration samples could not be analyzed, the mean value for the equilibration samples of the other 15 birds was used. Total BWC was calculated from the 18O dilution. FMR was converted from ml CO2 day−1 to kJ day−1, assuming 25 kJ L−1 CO2 (Nagy et al., 1999). Metabolic water production (MWP) was calculated (based on a millet seed diet) as 0.62 mg ml−1 O2 consumed (MacMillen and Hinds, 1983; MacMillen and Baudinette, 1993) and an oxyequivalence of 20.3 ml O2 kJ−1 (Withers et al., 2016).
Feeding and drinking behavior was assessed from 1 December to 19 February (excluding the days on which FMR and WTR were measured) by monitoring birds accessing the feeders and water troughs using an 11-cm diameter antenna connected to a PIT tag reader (RFIDRW-E-232; Priority 1 Design, Melbourne, Australia) that recorded a tagged bird’s unique ID code every time it passed through the antenna, with the date and time. Feeders were topped up with seed and water troughs checked daily, and the PIT recorder and timing checked (by passing a PIT through the antenna at a known time) and data downloaded every 1–5 days. Feeding behavior was analyzed for the four feeders on seven cool days when the maximum Ta was <30°C (mean maximum 25.6 ± 0.95°C, maximum range 22.2–29.8°C) and 17 hot days when the maximum Ta was >40°C (mean maximum 42.5 ± 0.42°C, maximum range 40.1–45.2°C), and for the water troughs for 26 hot and nine cool days, within 7 weeks of the FMR/WTR studies. The total number of times a bird passed through the antennae was summed for the four feeders and summarized into 30-min bin ranges, as were the data for the two water troughs.
Values are presented as mean ± S.E. with N = number of individuals, and times are Australian Central Summer Time (ACST; GMT + 10.5 h). Statistical analyses were accomplished with statistiXL (www.statistiXL.com). Body water content, FMR, and WTR were compared during hot and cool periods using two sample t-tests with a test, and if necessary correction, for equality of variance. Frequency of visits to the feeders and water troughs (analyzed separately) throughout the day was compared for hot and cool periods using a two-way contingency table (hot/cool vs. time of day). The frequency distribution of visits to feeders and water troughs during hot periods was directly compared to that during cool periods by using the distribution during cool periods as the expected values (adjusted to the same total number of visits during hot periods).
Results
Birds captured during hot periods had a higher body mass (12.2 ± 0.20 g, N = 20) than during cool periods (11.5 ± 0.17 g, N = 20; t38 = 2.43; p = 0.020), but body mass did not differ significantly for those 10 individuals that were recaptured during each period and for which FMR and WTR were measured (12.1 ± 0.23 hot and 11.4 ± 0.21 cool, mean = 11.7 ± 0.17 g, N = 20; t18 = 2.09; p = 0.051). There was no difference in the body mass change between initial capture and recapture for hot and cool periods (mean = 0.06 ± 0.116 g; t18 = 0.966, p = 0.347).
Enrichment of 18O and 2H for recaptured birds was at least 268 and 216 ppm higher than the mean background value respectively, and exceeded the maximum measured for background samples by more than three (18O) and seven (2H) times. Body water content was 75.4 ± 2.14% (N = 20) and WTR was 4.5 ± 0.30 ml day−1 (N = 20), and neither differed between hot and cool periods (t18 ≤ 1.73; p ≥ 0.187). Field metabolic rate was significantly lower (t18 = 3.54, p = 0.002) during hot (29.4 ± 2.41 kJ day−1) compared with cool periods (44.0 ± 3.35 kJ day−1). As a consequence, MWP calculated from FMR was correspondingly lower during hot (0.953 ± 0.092 ml day−1) compared to cool periods (1.317 ± 1.00 ml day−1), and was 20 ± 2.3 and 34 ± 2.6% of the birds’ WTR respectively.
There were 236 individual birds recorded visiting feeders, and 252 individuals recorded visiting water troughs during the study period. Birds visited the feeders between 05:30 and 20:00, and the water tanks between 03:30 and 20:00, with between 707 and 7,838 total visits to the feeders, and 116–13,182 total visits to the water troughs recorded per day. Two-way contingency tables indicated a highly significant interaction between hourly visitation frequency and hot and cool periods for both feeding and drinking ( = 13,310, p < 0.001; = 2,817, p < 0.001 respectively; Figure 1), and the frequency of feeding and drinking activity throughout the day was significantly different during hot (predominantly morning and evening activity) and cool (more uniform over the daytime) periods ( = 38,433, p < 0.001; = 172,814, p < 0.001, respectively; Figure 2).
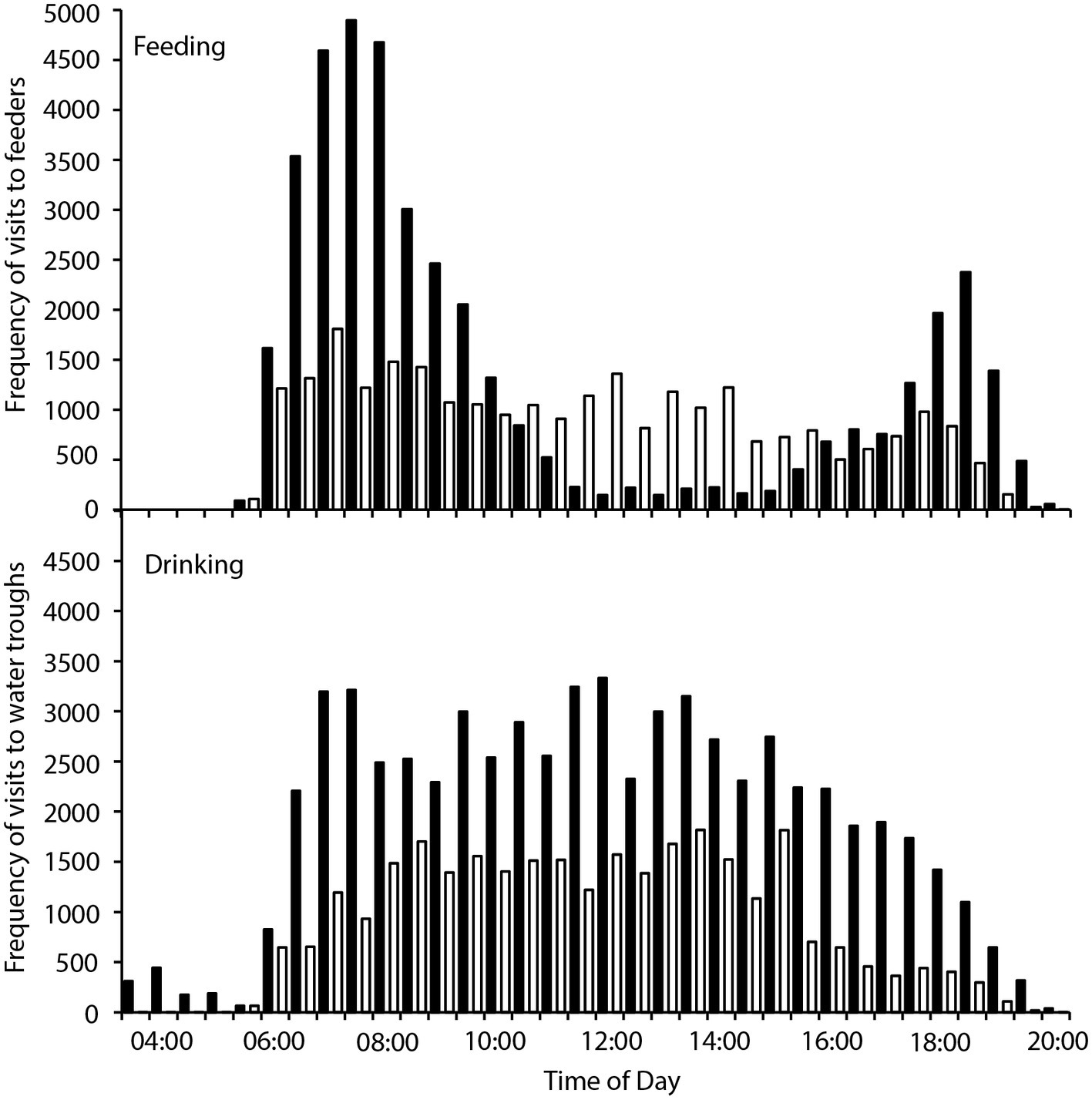
Figure 1. Frequency of visits to four feeders (top panel) and two water tanks (bottom panel) by zebra finches (Taeniopygia guttata) in an arid habitat during hot periods (maximum daily Ta > 40°C; black bars) and cool periods (maximum daily Ta < 30°C; white bars).
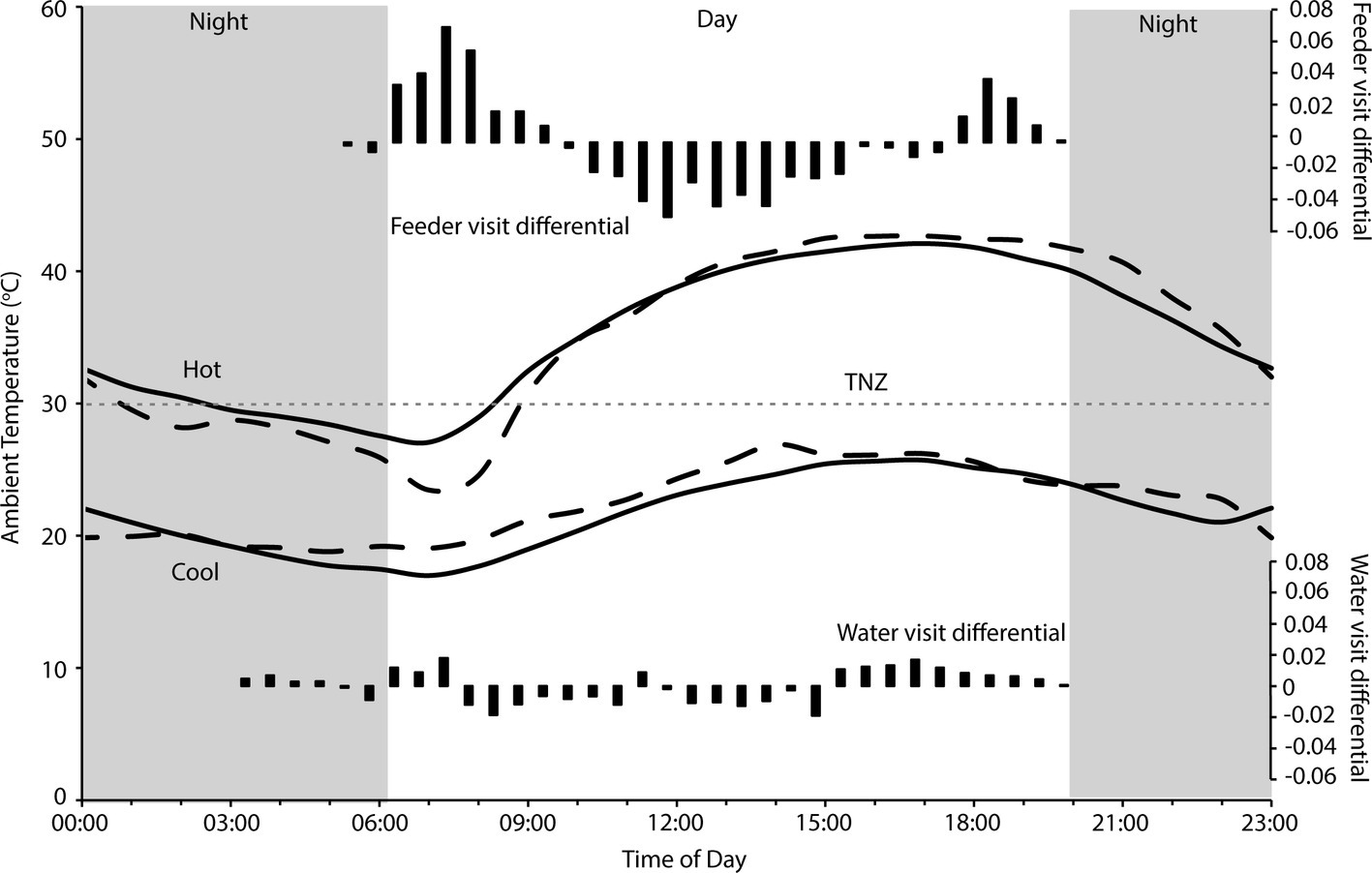
Figure 2. Hourly temperature during hot and cool periods at Fowlers Gap research station, when visits to feeders and water troughs by zebra finches (Taeniopygia guttata) were monitored (solid lines), and when FMR and WTR were measured (dashed lines). The gray dashed line indicates thermoneutrality (TNZ; 30°C) for zebra finches (Calder, 1964; Cade et al., 1965) and the gray panels indicate the period from sunset to sunrise. Black bars indicate the differential of frequencies (from Figure 1) of visits on hot to cool days, standardized to total number of hot day visits, to four feeders (top panel) and two water tanks (bottom panel). Temperature data sourced from the Australian Bureau of Meteorology for Fowlers Gap (station number 046128).
Discussion
The wild, free-living zebra finches that we studied at Fowlers Gap are iconic desert birds, withstanding short periods of extreme temperature in their harsh, arid-zone environment. A period of 3–4 days with maximum Ta > 40°C had minimal impact on the energy and water balance of the zebra finches. A combination of high BWC, a granivorous diet (with high digestibility that reduces foraging requirements), and the ability to behaviorally adjust foraging times in anticipation of high daytime environmental temperatures allows zebra finches to maintain body mass and meet their energy and water requirements when Ta exceeds thermoneutraltity for the majority of the day. Our data suggest that high Ta is actually less of an energetic stress than cooler days, and zebra finches are able to thermoregulate within tolerable limits without excessive water use, at least when maximum Ta does not exceed 45°C. Our results provide insight into the mechanisms by which birds can withstand extreme temperatures, and highlight situations when extreme environments may become lethal to birds.
Body Water Content
The BWC of birds is typically about 63–66% (Hughes et al., 1987; Ellis and Jehl, 1991; Speakman, 1997), although values of 70–80% have been reported, particularly for arid-habitat birds (e.g. Ambrose et al., 1996; Anava et al., 2000). Our values for zebra finches were relatively high (75%), and higher than for captive zebra finches (63%; Skadhauge and Bradshaw, 1974), and may indicate poor body condition with a low fat content (Johnson et al., 1985; Hughes et al., 1987; Ellis and Jehl, 1991). This presumably reflects the long-term drought and limited availability of food in an already harsh arid environment. This inference is supported by the observation that there had been little to no breeding activity of these birds in artificial next boxes (which generally support breeding, e.g., Griffith et al., 2008; Brandl et al., 2018) at the site during the previous year (Hurley and Griffith, personal observation). Although BWC can be overestimated if the period between injection of the isotope and sampling of blood for the equilibration sample is too long, our equilibration time of 1 h is typical for small birds (Speakman, 1997). A MWP of 1.317 ml day−1 (during cool periods when MWP was highest) would have produced only 0.055 ml of water during the 1-h equilibration period, which cannot account for the “extra” 1.028 ml of body water of a zebra finch with a calculated BWC of 75% compared to 66%. Body water content can also be overestimated if the injection volume was overestimated, for example by leakage from the injection site, or loss of injection solution from the syringe after pre-injection weighing. We cannot unequivocally eliminate this as a source of error, but did not observe leakage or spillage of injection solution. We therefore consider that our high value for BWC of zebra finches is real, and likely adaptive (see below).
Field Metabolic Rate and Water Turnover Rate
Our measures of FMR and WTR are consistent with allometric predictions for desert birds, and generally consistent with observations from captive individuals. Field WTRs of our zebra finches (4.5 ml day−1) were 99–113% of that predicted for desert birds, and 49–57% of that for all birds, after Tieleman and Williams (2000), and 80–92% and 74–85% respectively after Nagy and Peterson (1988), reflecting the more frugal water turnover of birds in arid habitats. The WTR we measured for wild, free-living zebra finches was also within the range of water intake rates of 3.8–5.4 ml water day−1 measured for captive zebra finches (Skadhauge and Bradshaw, 1974). The small body size and therefore relatively high metabolic rate of small birds means that they can meet a considerable proportion of their daily water requirements via MWP (Bartholomew, 1972; Bradshaw, 2003). For wild, free-living finches in an arid habitat in summer, MWP accounted for 20–34% of WTR, which was consistent with the 31.3% of daily water flux of zebra finches in the laboratory (Skadhauge and Bradshaw, 1974).
The extremely hot conditions during our FMR measurements did not negatively impact the zebra finches’ energy use, with finches maintaining body mass over the hot period. We calculated 1.9–2.9 g fresh matter intake day−1 for our finches (from FMR for a granivore after Nagy, 2001), which is 63–90% of that predicted for a passerine bird and 32–46% of that predicted for a desert bird, reflecting the high metabolizable energy content, and therefore low fresh matter intake, of a granivorous diet (Nagy, 2001). The FMR that we measured for our birds during this study was 2.0–2.8 times BMR for this species (Rønning et al., 2005; Cooper et al., in review), consistent with the generalization that FMR is 2–3 times BMR (Degen and Kam, 1995; but see Koteja, 1991 and Ricklefs et al., 1996).
Feeding and Drinking
On days of extremely high Ta, zebra finches dramatically reduced foraging, and to a lesser extent drinking, during the hottest part of the day, as is typical for birds experiencing extreme heat and solar radiation (Wolf, 2000). Activity for foraging and drinking will increase metabolic heat production, and doing so in unshaded locations typical of arid environments also exacerbates an already high Ta with high incident solar radiation (Abdu et al., 2018). This may increase the effective Ta experienced by a small bird by about 12°C (Wolf, 2000), meaning birds in our study would be exposed to temperatures in excess of 57°C, which is well above the tolerance limit of most passerine birds (Abdu et al., 2018; McKechnie and Wolf, 2019). Therefore, zebra finches, like other desert birds, presumably face a trade-off between maintaining energy and water balance during extremely hot conditions and accessing food and water with exacerbated heat gain (Smit et al., 2016).
We found that zebra finches reduced their foraging activity for approximately 8 h in the middle of the day on hot compared to cool days. Finches balanced the reduced midday foraging on days with a high maximum Ta with increased foraging in the early cooler part of the day, and to a lesser extent in the evening (Figure 1). In contrast, birds foraged more consistently throughout the day during cooler weather. Previous studies of zebra finches at this site found that increased evening, rather than morning, foraging compensated for reduced midday foraging (Funghi et al., 2019), but for diurnal birds this strategy relies on sufficient environmental cooling in the evenings before dark for birds to forage. Environmental conditions are cooler in the early mornings than evenings (Figure 2), so anticipatory early morning foraging is likely a more favorable strategy during extremely hot conditions. However, birds must be able to anticipate reduced midday foraging to increase feeding in preparation for prohibitive daytime and evening conditions. Our data suggest that zebra finches were able to anticipate hot midday temperatures, presumably from overnight and early morning conditions, and increase their morning foraging accordingly. Similar pre-emptive responses to high maximum daytime Ta have been described for red kangaroos (Osphranter rufus) and western gray kangaroos (Macropus fuliginosus), whose nychthemeral reductions in Tb were greater in the early mornings of days with high maximum temperature than on days with lower maximum temperature, presumably facilitating heat storage later in the day (Brown and Dawson, 1977; Maloney et al., 2004). However, unlike kangaroos, which appear to pre-empt a favorable thermal response via an autonomous physiological feedback mechanism to environmental conditions (warming of the skin surface, resulting in distribution of core body heat to the cooler periphery and therefore a decrease in core Tb; Maloney et al., 2004), our data suggest that finches behaviorally “plan” to withstand upcoming harsh environmental conditions.
Mass, Energy, and Heat Balance
We found no difference in the zebra finches’ ability to maintain body mass over consecutive hot or cool days, despite evidence that reductions in foraging time and/or efficiency due to trade-offs with thermoregulatory behavior may significantly impact on energy balance of other birds, and that birds at high Ta may not be able to maintain body mass after consecutive warm days (du Plessis et al., 2012; Cunningham et al., 2015; Van de Ven, 2017). In fact, zebra finches maintained a higher body mass when it was hotter, and Ta was within or above the thermoneutral zone for the majority of each day (Figure 2). Although total foraging time of zebra finches during hot weather is less than during cool weather (Funghi et al., 2019), the reduced requirement for MHP means that total energetic requirements are lower, and a high-energy, highly digestible granivorous diet (MacMillen, 1990; Nagy, 2001) minimizes the fresh matter intake required for these birds compared to other species. Our data suggest that extremely hot weather did not place energetic constraints on the zebra finches by limiting feeding. Rather, cooler periods posed greater energetic challenges by requiring increased MHP to maintain a homeothermic Tb at Ta below thermoneutrality (Figure 2), and this may have been more challenging during a drought when natural food availability is low. Body size, diet, and foraging strategy presumably influence which bird species are most likely to experience energetic constraints from high temperatures (Edwards et al., 2015; Smit et al., 2016). Granivores, even those with food involving considerable handling time, may be able to meet their daily energetic requirements with “time to spare” and therefore do not need to forage for the entire daytime period. For example, cockatoos can meet their energetic returns by foraging for only 4–5 h a day (Cooper et al., 2002) and rest in shady locations for many hours, especially during summer (Noske, 1982; Cooper, personal observation). The finches in this study were provided with supplementary food to facilitate capture and data collection, which presumably assisted them in meeting their energy requirements more quickly than if they had to forage widely for all of their food. However, foraging behavior of individual birds suggests that they maintained natural foraging patterns when visiting the feeders (Funghi et al., 2019). A high BWC (75 vs. ~66%) and lack of breeding at the site also suggest poor body condition, and are evidence that the supplemental food provided was not excessive and did not compensate entirely for a scarcity of food as a consequence of the long-term drought.
Due to lower FMRs during hot periods, MWP was a smaller proportion of the total WTR of zebra finches, and therefore this difference (14% of WTR) had to be gained, presumably by increased drinking. Indeed, finches drank more frequently on hot compared to cool days (Figure 1), which maintained the same WTR. Measurements of physiological variables for zebra finches in the laboratory (Cooper et al,. in review) suggest that, despite their small body size, they use facultative hyperthermia to conserve water over the short term; a water savings of 9.17 mg g−1 h−1 measured for hyperthermic finches at 40°C (Cooper et al., in review) equates to a water savings of 57% of the mean water use by wild birds over 8 h. Finches can therefore delay dissipation of body heat until cooler environmental conditions facilitate non-evaporative heat loss, and this presumably is why birds during hot periods did not have a higher overall WTR than those during cool periods.
Despite overall higher drinking rates, distribution of visits by finches to water troughs decreased more in the middle of the day when it was hottest, and they increased their visits to water more in the early mornings and especially late afternoons (Figure 2), presumably preparing for, and then replenishing, water lost throughout the day. As they did for foraging, birds appear to be anticipating upcoming limitations on daytime drinking by drinking earlier, and more frequently early in the day, on extremely hot days. A high BWC may reduce the impact of short-term dehydration (e.g., as for amphibians; Hillman et al., 2009), and therefore limit reliance on visits to water during the heat of the day. If we assume that an 11.7-g bird with 66% BWC (Speakman, 1997) can lose ~11% of body mass as water before facing lethal dehydration (Wolf, 2000), then it can lose 1.287 ml of water (29% of their WTR) to retain 6.4 ml of body water. However, a bird of the same body mass starting with 75% body water can lose 2.34 ml of water to retain 6.4 ml of body water; they can lose 54% of their daily water turnover before risking lethal dehydration, extending the period birds can avoid drinking during periods of extreme environmental temperature. Indeed, finches did increase their drinking in the late afternoon on hot compared to cool days, presumably replenishing their body water store once the contribution of solar radiation to environmental temperature had decreased (Figure 2). Spinifexbirds (Eremiornis carteri; Ambrose et al., 1996) and Arabian babblers (Turdoides squamiceps; Anava et al., 2000) are other arid-habitat birds that have similarly high BWCs (up to 80%) that may also serve as a water store.
Implications for Understanding High-Temperature Avian Mass Mortality
Overall, our results indicate that heatwaves of 40–45°C maximum Ta can be accommodated by arid-adapted zebra finches without major impacts on energy and water turnover. Finches modified their behavior to avoid or limit activity during the most thermally challenging periods of the day and, together with a high BWC that presumably increased their body water store, were able to maintain body mass during a heatwave by pre-emptively feeding and drinking in preparation for hours of relative inactivity at high Ta. We can apply this information to identify potential situations where avian mass mortality events can occur. Predicting upcoming periods of unfavorably high Ta may be essential for survival of extreme Ta. One recently recorded avian mortality event, at Hopetoun, Western Australia, occurred when an extremely hot day (maximum Ta = 48°C) occurred between two much cooler days (maximum Ta = 24–26°C; Saunders et al., 2011; Figure 3), and following at least a fortnight of relatively cool summer maximum temperatures. Early morning temperatures on this day were indistinguishable from those of the preceding days; so, it is likely that birds experiencing this hot day did not “prepare” sufficiently in the cooler morning when feeding and more importantly drinking were possible, if the extremely high Ta was unexpected. The short-term predictability of heatwaves may be a determinate of avian survival. Another recent avian mass mortality event occurred at Overlander Roadhouse in the mid-west region of Western Australia (Birdlife Australia, 2014). In this case, maximum temperatures were very extreme, >40° for more than a fortnight, and ≥45°C for several consecutive days (Figure 4). Unfortunately, hourly temperature data are not available for a weather station close to this site, but it is likely that temperatures did not drop sufficiently during daylight hours over this prolonged heatwave to allow sufficient feeding or drinking to maintain energy and water stores. In addition, a previously good season with high winter and spring rainfall that facilitated breeding and subsequent large numbers of birds in the region (Birdlife Australia, 2014) presumably meant that birds were in good body condition, and may not have had the high lean BWC that we hypothesize may be critical for facilitating dehydration tolerance during the heat of the day.
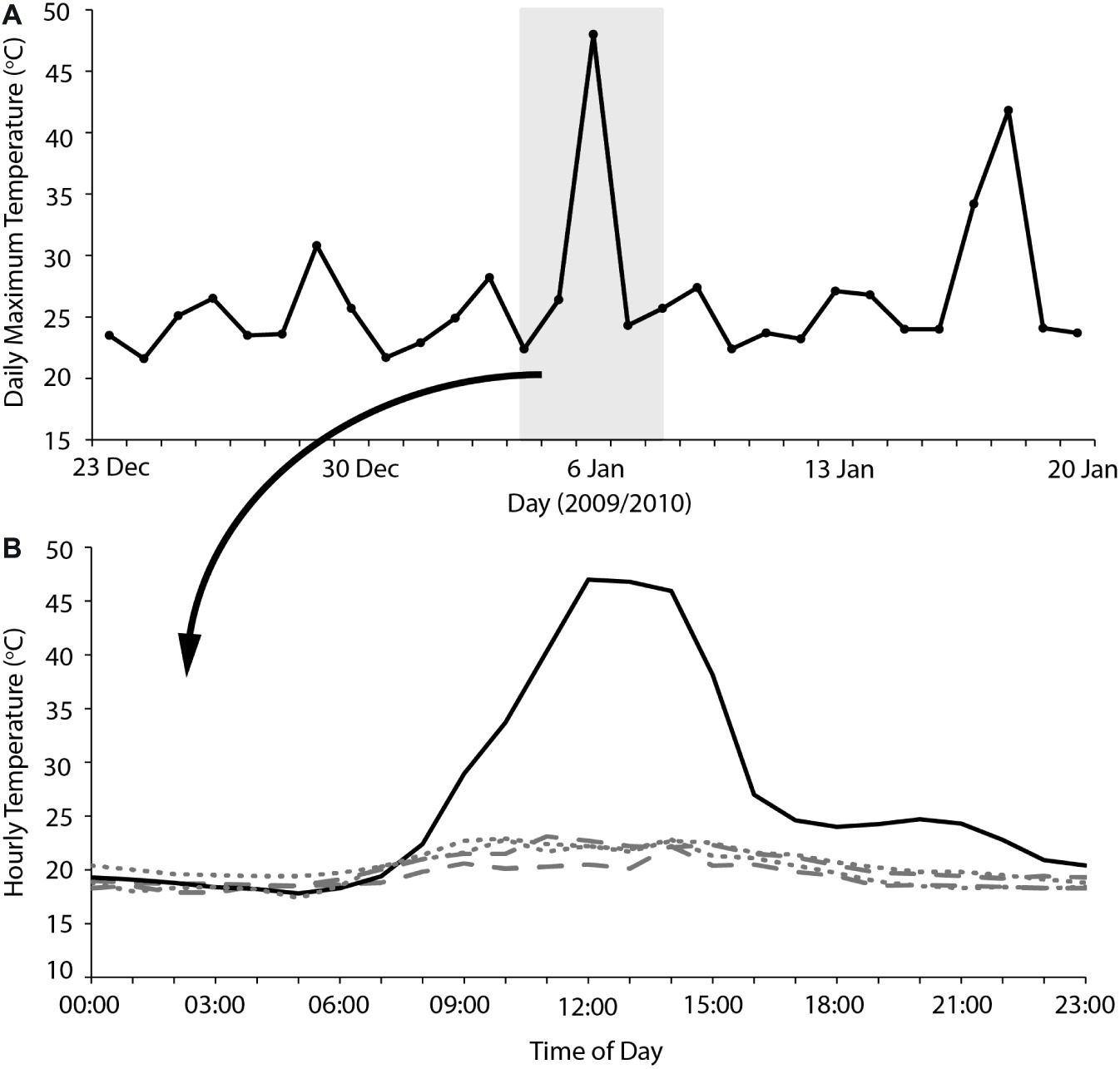
Figure 3. (A) Maximum daily temperatures for 2 weeks either side of an avian mass mortality event that occurred on the 6/2/2010 at Hopetoun, Western Australia. The gray shaded section indicates the 5 days where hourly temperature is plotted (B) for the 2 days before (dashes), 2 days after (dots), and the day of the avian mass mortality event (solid). Temperature data sourced from the Australian Bureau of Meteorology for Hopetoun North (station number 009961).
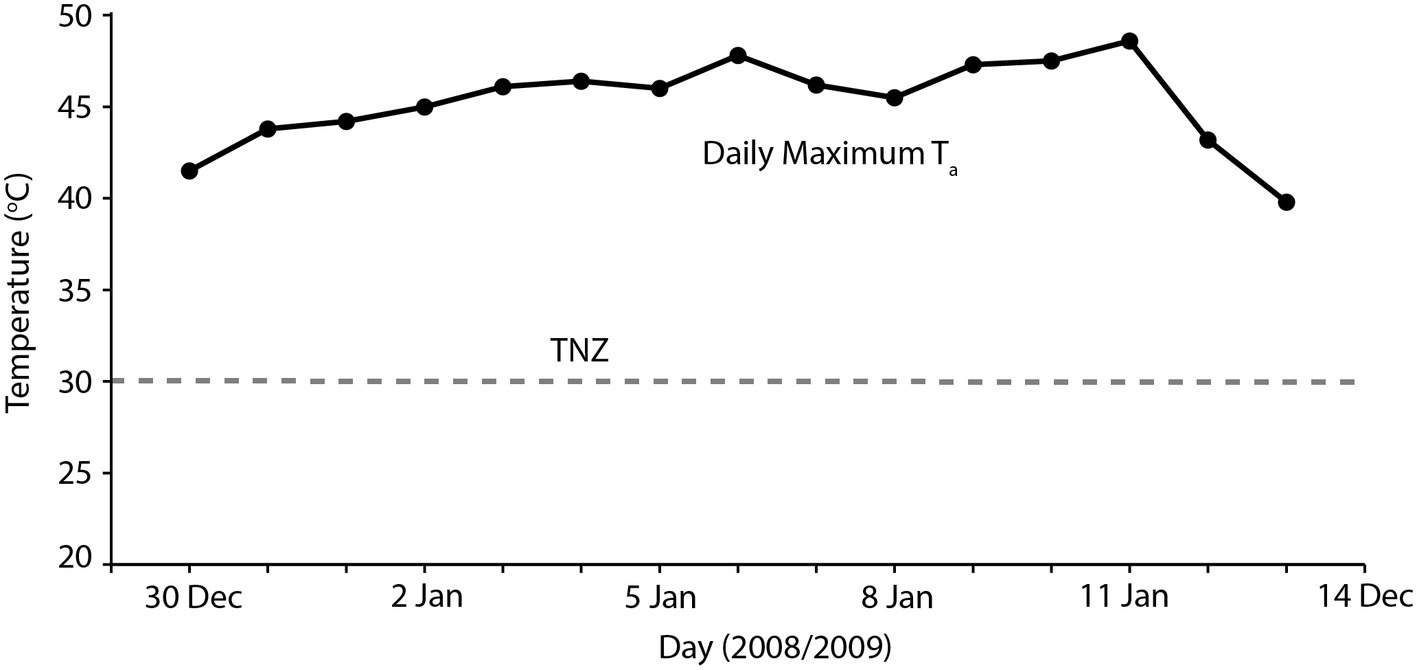
Figure 4. Maximum daily temperatures (black symbols) for 2 weeks during which time an avian mass mortality event occurred during January 2019, in the Pilbara region of Western Australia. The gray dashed line indicates thermoneutrality (TNZ; 30°C) for zebra finches (Calder, 1964; Cade et al., 1965). Temperature data sourced from the Australian Bureau of Meteorology for Gascoyne Junction (station number 006022).
To manage species and minimize biodiversity loss under the inevitable climatic changes that are occurring as a consequence of anthropogenic activity, we need a greater mechanistic understanding of how species physiologically accommodate environmental variability (Harris et al., 2018; Conradie et al., 2019). Our results reveal the consequences of short periods of high temperatures for an iconic desert bird in its natural habitat, and indicate the physiological and behavioral mechanisms by which zebra finches withstand extreme environmental conditions. This information has revealed that periods of low Ta during summer and particularly during droughts may pose greater energetic challenges than heatwaves for granivores. We also identify high BWC and predictable foraging limitations as potentially important elements for avian survival during periods of extreme heat. This information may assist in predicting and managing avian responses to periodic extreme weather events.
Data Availability Statement
The datasets generated for this study are available on request to the corresponding author.
Ethics Statement
The animal study was reviewed and approved by the Macquarie University and Curtin University Animal Ethics Committees (ARA 2017/024 and ARE2017-16).
Author Contributions
CC and SG designed the study, provided equipment and logistical support, and obtained funding. CC and LH conducted the fieldwork. CC and PW carried out laboratory procedures and analyzed the data. CC drafted the manuscript. All authors edited the manuscript.
Funding
This research was supported by the Australian Research Council’s Discovery Projects funding scheme (DP170103619).
Conflict of Interest
The authors declare that the research was conducted in the absence of any commercial or financial relationships that could be construed as a potential conflict of interest.
Acknowledgments
We thank Keith Leggett and staff at Fowlers Gap Research Station for logistical assistance with this study, Hanja Brandl and Wiebke Schuett for implanting PITs in many of the birds at the site, and Caterina Funghi and Katrin Quiring for recording activity at the feeders and water troughs during early December. We are extremely grateful to Greg and Ela Skrzypek and Douglas Ford for their expert advice concerning sample preparation and for isotope analyses.
References
Abdu, S., McKechnie, A. E., Lee, A. T., and Cunningham, S. J. (2018). Can providing shade at water points help Kalahari birds beat the heat? J. Arid Environ. 152, 21–27. doi: 10.1016/j.jaridenv.2018.01.018
Albright, T. P., Mutiibwa, D., Gerson, A. R., Smith, E. K., Talbot, W. A., O’Neill, J. J., et al. (2017). Mapping evaporative water loss in desert passerines reveals an expanding threat of lethal dehydration. Proc. Natl. Acad. Sci. USA 114, 2283–2288. doi: 10.1073/pnas.1613625114
Ambrose, S. J., Bradshaw, S. D., Withers, P. C., and Murphy, D. P. (1996). Water and energy balance of captive and free-ranging Spinifexbirds (Eremiornis carteri) north (Aves: Sylviidae) on Barrow Island, Western Australia. Aust. J. Zool. 44, 107–117. doi: 10.1071/ZO9960107
Anava, A., Kam, M., Shkolnik, A., and Degen, A. A. (2000). Seasonal field metabolic rate and dietary intake in Arabian babblers (Turdoides squamiceps) inhabiting extreme deserts. Funct. Ecol. 1, 607–613. doi: 10.1046/j.1365-2435.2000.t01-1-00461.x
Australian Bureau of Meteorology (2018). An abnormally dry period in eastern Australia. Special Climate Statement 66, Australian Government.
Bartholomew, G. A. (1972). “The water economy of seed-eating birds that survive without drinking” in Proceedings of the 15th international ornithological congress. ed. K. H. Voous (Leiden: E. J. Brill).
Birdlife Australia (2014). Thousands of birds die in sweltering heat. Available at: http://www.birdsinbackyards.net/content/article/Thousands-birds-die-sweltering-heat (Accessed November 17, 2019).
Bradshaw, D. (2003). Vertebrate ecophysiology: An introduction to its principles and applications. Cambridge: Cambridge University Press.
Brandl, H. B., Griffith, S. C., and Schuett, W. (2018). Wild zebra finches do not use social information from conspecific reproductive success for nest site choice and clutch size decisions. Behav. Ecol. Sociobiol. 72:114. doi: 10.1007/s00265-018-2533-3
Brown, G. D., and Dawson, T. J. (1977). Seasonal variations in the body temperatures of unrestrained kangaroos (Macropodidae: Marsupialia). Comp. Biochem. Physiol. A 56, 59–67.
Cade, T. J., Tobin, C. A., and Gold, A. (1965). Water economy and metabolism of two estrildine finches. Physiol. Zool. 38, 9–33. doi: 10.1086/physzool.38.1.30152342
Calder, W. A. (1964). Gaseous metabolism and water relations of the zebra finch, Taeniopygia castanotis. Physiol. Zool. 37, 400–413. doi: 10.1086/physzool.37.4.30152758
Cannell, B. L., Chambers, L. E., Wooller, R. D., and Bradley, J. S. (2012). Poorer breeding by little penguins near Perth, Western Australia is correlated with above average sea surface temperatures and a stronger Leeuwin current. Mar. Freshw. Res. 63, 914–925. doi: 10.1071/MF12139
Chown, S. L., Hoffmann, A. A., Kristensen, T. N., Angilletta, M. J., Stenseth, N. C., and Pertoldi, C. (2010). Adapting to climate change: a perspective from evolutionary physiology. Clim. Res. 43, 3–15. doi: 10.3354/cr00879
Conradie, S. R., Woodborne, S. M., Cunningham, S. J., and McKechnie, A. E. (2019). Chronic, sublethal effects of high temperatures will cause severe declines in southern African arid-zone birds during the 21st century. Proc. Natl. Acad. Sci. USA 116, 14065–14070. doi: 10.1073/pnas.1821312116
Cooper, C. E., Withers, P. C., Mawson, P. R., Bradshaw, S. D., Prince, J., and Roberston, H. (2002). Metabolic ecology of cockatoos in the south-west of Western Australia. Aust. J. Zool. 50, 67–76. doi: 10.1071/ZO00067
Coplen, T. B. (1996). New guidelines for reporting stable hydrogen, carbon, and oxygen isotope-ratio data. Geochim. Cosmochim. Acta 60, 3359–3360. doi: 10.1016/0016-7037(96)00263-3
Cunningham, S. J., Martin, R. O., and Hockey, P. A. (2015). Can behaviour buffer the impacts of climate change on an arid-zone bird? Ostrich 86, 119–126. doi: 10.2989/00306525.2015.1016469
Degen, A. A., and Kam, M. (1995). Scaling of field metabolic rate to basal metabolic rate ratio in homeotherms. Ecoscience 2, 48–54.
Diffenbaugh, N. S., and Field, C. B. (2013). Changes in ecologically critical terrestrial climate conditions. Science 341, 486–492. doi: 10.1126/science.1237123
Edwards, E. K., Mitchell, N. J., and Ridley, A. R. (2015). The impact of high temperatures on foraging behaviour and body condition in the Western Australian magpie Cracticus tibicen dorsalis. Ostrich 86, 137–144. doi: 10.2989/00306525.2015.1034219
Ellis, H. I., and Jehl, J. R. (1991). Total body water and body composition in phalaropes and other birds. Physiol. Zool. 64, 973–984. doi: 10.1086/physzool.64.4.30157952
Finlayson, H. H. (1932). Heat in the interior of South Australia – holocaust of bird-life. South Aust. Ornith. 11, 158–160.
Franklin, D. C., Ehmke, G., Van Der Wal, J., and Garnett, S. T. (2014). “The exposure of Australian birds to climate change” in Climate change adaptation plan for Australian birds. eds. S. T. Garnett and D. C. Franklin (Collingwood, Victoria: CSIRO Publishing), 7–25.
Funghi, C., McCowan, L. S., Schuett, W., and Griffith, S. C. (2019). High air temperatures induce temporal, spatial and social changes in the foraging behaviour of wild zebra finches. Animal Behav. 149, 33–43. doi: 10.1016/j.anbehav.2019.01.004
Griffith, S. C., Pryke, S. R., and Mariette, M. (2008). Use of nest-boxes by the Zebra finch (Taeniopygia guttata): implications for reproductive success and research. Emu 108, 311–319. doi: 10.1071/MU08033
Harris, R. M., Beaumont, L. J., Vance, T. R., Tozer, C. R., Remenyi, T. A., Perkins-Kirkpatrick, S., et al. (2018). Biological responses to the press and pulse of climate trends and extreme events. Nat. Clim. Chang. 8, 579–587. doi: 10.1038/s41558-018-0187-9
Hillman, S. S., Withers, P. C., Drewes, R. C., and Hillyard, S. D. (2009). Ecological and environmental physiology of amphibians. Oxford: Oxford University Press.
Hughes, M. R., Roberts, J. R., and Thomas, B. R. (1987). Total body water and its turnover in free-living nestling glaucous-winged gulls with a comparison of body water and water flux in avian species with and without salt glands. Physiol. Zool. 60, 481–491. doi: 10.1086/physzool.60.4.30157910
IPCC (2014). “Contribution of working groups I, II and III to the fifth assessment report of the intergovernmental panel on climate change” in Climate change 2014: Synthesis report. eds. Core Writing Team R. K. Pachauri and L. A. Meyer (Geneva, Switzerland: IPCC), 151.
Johnson, D. H., Krapu, G. L., Reinecke, K. J., and Jorde, D. G. (1985). An evaluation of condition indices for birds. J. Wildl. Manag. 49, 569–575. doi: 10.2307/3801673
Kendeigh, S. C. (1969). Energy responses of birds to their thermal environments. Wilson Bull. 81, 441–449.
Koteja, P. (1991). On the relation between basal and field metabolic rates in birds and mammals. Funct. Ecol. 5, 56–64. doi: 10.2307/2389555
Lifson, N., and McClintock, R. (1966). Theory of use of the turnover rates of body water for measuring energy and material balance. J. Theor. Biol. 12, 46–74. doi: 10.1016/0022-5193(66)90185-8
MacMillen, R. E. (1990). Water economy of granivorous birds: a predictive model. Condor 92, 379–392. doi: 10.2307/1368235
MacMillen, R. E., and Baudinette, R. V. (1993). Water economy of granivorous birds: Australian parrots. Funct. Ecol. 7, 704–712. doi: 10.2307/2390192
MacMillen, R. E., and Hinds, D. S. (1983). Water regulatory efficiency in heteromyid rodents: a model and its application. Ecology 64, 152–164. doi: 10.2307/1937337
Maloney, S. K., Fuller, A., Kamerman, P. R., Mitchell, G., and Mitchell, D. (2004). Variation in body temperature in free-ranging western grey kangaroos Macropus fuliginosus. Aust. Mammal. 26, 135–144. doi: 10.1071/AM04135
McKechnie, A. E., Hockey, P. A., and Wolf, B. O. (2012). Feeling the heat: Australian landbirds and climate change. Emu 112, 1–7. doi: 10.1071/MUv112n2_ED
McKechnie, A. E., and Wolf, B. O. (2010). Climate change increases the likelihood of catastrophic avian mortality events during extreme heat waves. Biol. Lett. 6, 253–256. doi: 10.1098/rsbl.2009.0702
McKechnie, A. E., and Wolf, B. O. (2019). The physiology of heat tolerance in small endotherms. Physiology 34, 302–313. doi: 10.1152/physiol.00011.2019
Meehl, G. A., and Tebaldi, C. (2004). More intense, more frequent, and longer lasting heat waves in the 21st century. Science 305, 994–997. doi: 10.1126/science.1098704
Nagy, K. A. (1983). The doubly labeled water (3HH180) method: A guide to its use. UCLA Publ. 12-1417. Los Angeles: UCLA.
Nagy, K. A. (2001). Food requirements of wild animals: predictive equations for free-living mammals, reptiles, and birds. Nutr. Abst. Rev. B 71, 21R–31R.
Nagy, K. A., Girard, I. A., and Brown, T. K. (1999). Energetics of free-ranging mammals, reptiles, and birds. Annu. Rev. Nutr. 19, 247–277. doi: 10.1146/annurev.nutr.19.1.247
Nagy, K. A., and Peterson, C. C. (1988). Scaling of water flux rate in animals. UC Pub. Zool. 120, 1–81.
Noske, S. (1982). Aspects of the behaviour and ecology of the white cockatoo (Cacatua galerita) and galah (C. roseicapilla) in croplands in North-East New South Wales. MSc thesis. Armidale, New South Wales: University of New England.
du Plessis, K. L., Martin, R. O., Hockey, P. A., Cunningham, S. J., and Ridley, A. R. (2012). The costs of keeping cool in a warming world: implications of high temperatures for foraging, thermoregulation and body condition of an arid-zone bird. Glob. Chang. Biol. 18, 3063–3070. doi: 10.1111/j.1365-2486.2012.02778.x
Ratnayake, H. U., Kearney, M. R., Govekar, P., Karoly, D., and Welbergen, J. A. (2019). Forecasting wildlife die-offs from extreme heat events. Anim. Conserv. 22, 386–396. doi: 10.1111/acv.12476
Ricklefs, R. E., Konarzewski, M., and Daan, S. (1996). The relationship between basal metabolic rate and daily energy expenditure in birds and mammals. Am. Nat. 147, 1047–1071. doi: 10.1086/285892
Rønning, B., Moe, B., and Bech, C. (2005). Long-term repeatability makes basal metabolic rate a likely heritable trait in the zebra finch Taneniopygia guttata. J. Exp. Biol. 208, 4663–4669. doi: 10.1242/jeb.01941
Ruthrof, K. X., Breshears, D. D., Fontaine, J. B., Froend, R. H., Matusick, G., Kala, J., et al. (2018). Subcontinental heat wave triggers terrestrial and marine, multi-taxa responses. Sci. Rep. 8:13094. doi: 10.1038/s41598-018-31236-5
Saunders, D. A., Mawson, P., and Dawson, R. (2011). The impact of two extreme weather events and other causes of death on Carnaby’s black cockatoo: a promise of things to come for a threatened species? Pacific Cons. Biol. 17, 141–148. doi: 10.1071/PC110141
Skadhauge, E., and Bradshaw, S. D. (1974). Saline drinking and cloacal excretion of salt and water in the zebra finch. Am. J. Phys. 227, 1263–1267.
Skrzypek, G. (2013). Normalization procedures and reference material selection in stable HCNOS isotope analyses: an overview. Anal. Bioanal. Chem. 405, 2815–2823. doi: 10.1007/s00216-012-6517-2
Skrzypek, G., and Ford, D. (2014). Stable isotope analysis of saline water samples on a cavity ring-down spectroscopy instrument. Environ. Sci. Technol. 48, 2827–2834. doi: 10.1021/es4049412
Smit, B., Zietsman, G., Martin, R. O., Cunningham, S. J., McKechnie, A. E., and Hockey, P. A. R. (2016). Behavioural responses to heat in desert birds: implications for predicting vulnerability to climate warming. Climate Change Resp. 3:9. doi: 10.1186/s40665-016-0023-2
Tebaldi, C., Hayhoe, K., Arblaster, J. M., and Meehl, G. A. (2006). Going to the extremes. Clim. Chang. 79, 185–211. doi: 10.1007/s10584-006-9051-4
Tieleman, B. I., and Williams, J. B. (2000). The adjustment of avian metabolic rates and water fluxes to desert environments. Physiol. Biochem. Zool. 73, 461–479. doi: 10.1086/317740
Van de Ven, T. M. E. N. (2017). Implication of climate change on the reproductive success of the southern yellow-billed hornbill (Tockus leucomelas). PhD thesis. Cape Town, South Africa: University of Cape Town.
Williams, J. B., and Tieleman, B. I. (2005). Physiological adaptation in desert birds. Bioscience 55, 416–425. doi: 10.1641/0006-3568(2005)055[0416:PAIDB]2.0.CO;2
Withers, P. C., Cooper, C. E., and Buttemer, W. A. (2004). Are day-active small mammals rare and small birds abundant in Australian desert environments because small mammals are inferior thermoregulators? Aust. Mammal. 26, 117–124. doi: 10.1071/AM04117
Withers, P. C., Cooper, C. E., Maloney, S. K., Bozinovic, F., and Cruz-Neto, A. P. (2016). Ecological and environmental physiology of mammals. Oxford U.K.: Oxford University Press.
Wolf, B. (2000). Global warming and avian occupancy of hot deserts; a physiological and behavioral perspective. Rev. Chil. Hist. Nat. 73, 395–400. doi: 10.4067/S0716-078X2000000300003
Keywords: bird, climate change, field metabolic rate, field water turnover rate, heatwave, foraging, temperature, zebra finch
Citation: Cooper CE, Withers PC, Hurley LL and Griffith SC (2019) The Field Metabolic Rate, Water Turnover, and Feeding and Drinking Behavior of a Small Avian Desert Granivore During a Summer Heatwave. Front. Physiol. 10:1405. doi: 10.3389/fphys.2019.01405
Edited by:
Julia Nowack, Liverpool John Moores University, United KingdomReviewed by:
Ivan Maggini, University of Veterinary Medicine Vienna, AustriaAlex Gerson, University of Massachusetts Amherst, United States
Copyright © 2019 Cooper, Withers, Hurley and Griffith. This is an open-access article distributed under the terms of the Creative Commons Attribution License (CC BY). The use, distribution or reproduction in other forums is permitted, provided the original author(s) and the copyright owner(s) are credited and that the original publication in this journal is cited, in accordance with accepted academic practice. No use, distribution or reproduction is permitted which does not comply with these terms.
*Correspondence: Christine Elizabeth Cooper, Yy5jb29wZXJAY3VydGluLmVkdS5hdQ==
†ORCID: Christine Elizabeth Cooper, orcid.org/0000-0001-6225-2324