- Department of Molecular and Cellular Therapeutics, Royal College of Surgeons in Ireland, Dublin, Ireland
The first ascent of Mount Everest by Tenzing Norgay and Sir Edmund Hillary in 1953 brought global attention to the Sherpa people and human performance at altitude. The Sherpa inhabit the Khumbu Valley of Nepal, and are descendants of a population that has resided continuously on the Tibetan plateau for the past ∼25,000 to 40,000 years. The long exposure of the Sherpa to an inhospitable environment has driven genetic selection and produced distinct adaptive phenotypes. This review summarizes the population history of the Sherpa and their physiological and genetic adaptation to hypoxia. Genomic studies have identified robust signals of positive selection across EPAS1, EGLN1, and PPARA, that are associated with hemoglobin levels, which likely protect the Sherpa from altitude sickness. However, the biological underpinnings of other adaptive phenotypes such as birth weight and the increased reproductive success of Sherpa women are unknown. Further studies are required to identify additional signatures of selection and refine existing Sherpa-specific adaptive phenotypes to understand how genetic factors have underpinned adaptation in this population. By correlating known and emerging signals of genetic selection with adaptive phenotypes, we can further reveal hypoxia-related biological mechanisms of adaptation. Ultimately this work could provide valuable information regarding treatments of hypoxia-related illnesses including stroke, heart failure, lung disease and cancer.
Introduction
The term “sher-pa” is the Tibetan for “eastern-people”. The Sherpa reside primarily in the Solukhumbu district of Nepal but there are also smaller settlements in the Tibet Autonomous Region of China. The Sherpa speak a Tibetan dialect, and they share similar cultural and religious practices with Tibetans. They are traditionally engaged in farming; cultivating barley, potatoes and rearing yak and sheep. Starting with the first Everest expeditions in the 1920’s, the Sherpa have become renowned for their ability as mountaineers and today they often aid and lead climbing expeditions in the Himalayas. Examples of their exceptional climbing feats include the first ascent of Mount Everest by Tenzing Norgay Sherpa, who accompanied Sir Edmund Hillary in the final stage of the 1953 expedition and Ang Rita Sherpa (known as “The Snow Leopard”) who, between 1983 and 1996, summited Everest ten times without the use of supplemental oxygen. The remarkable tolerance of the Sherpa to hypoxia has, over the last 60 years, been a focus of attention for the scientific community, in particular physiologists (Gilbert-Kawai et al., 2014).
The Sherpa are direct descendants of an ancestral population that has resided continuously on the Tibetan plateau for the past 25,000 to 40,000 years (Aldenderfer, 2011; Zhang et al., 2018). This long exposure to the evolutionary pressure presented by high altitude has driven physiological adaptation, which in turn has allowed the Sherpa to thrive. The adaptive physiological makeup of the Sherpa can inform on treatments for hypoxia-related illness including pulmonary, cardiac, neurological and renal disorders (Martin et al., 2013; Luks and Hackett, 2014; Gilbert-Kawai et al., 2015). Thus, studying the Sherpa at altitude offers a unique, “natural laboratory” that can provide insight to the molecular mechanisms of hypoxia.
An early paper on Sherpa physiology, published in 1965, suggested that the Sherpa have an efficient mechanism of oxygen utilization at the cellular level, allowing them to perform well under hypoxia (Lahiri and Milledge, 1965). Since then, our knowledge of Sherpa adaptation has grown, largely by comparing different physiological parameters between the Sherpa and people of lowland origin. With the development of high throughput DNA genotyping and sequencing platforms, genomic studies of indigenous high-altitude populations, including the Sherpa, have begun to emerge. These have provided insight into population history and genetic signatures of altitude-driven natural selection. In this review, we (1) summarize the population history of, (2) describe distinct adaptive phenotypes and (3) discuss signatures of selection, in the Sherpa. We highlight the need for further research connecting genetic factors to physiological adaptation in the Sherpa at extreme altitude.
The Sherpa, a Recently Derived Tibetan Population
Stone tools used by early humans have been found at Nwya Devu in central Tibet at an altitude of 4,600 m. Dating to 30,000 to 40,000 years before present (YBP), these findings represent the earliest archeological record of human colonization of the Tibetan plateau (Zhang et al., 2018). Genetic studies have suggested that the ancestors of both the Sherpa and Tibetans diverged from a Han Chinese population and arrived on the Tibetan plateau from lowland East Asia around 40,000 years ago (Qi et al., 2013; Jeong et al., 2014).
The prevailing hypothesis is that, during the 16th century, the ancestors of the Sherpa migrated from Tibet to the Khumbu Valley of Nepal, driven by political and religious turmoil resulting from a Mongol invasion (Oppitz, 1974). The presence of Sherpa-specific mitochondrial DNA (mtDNA) lineages (Kang et al., 2013) in a Nepalese context, with an estimated age of less than 1,500 years and derived from Tibetans, further supports this hypothesis of a recent migration of the Sherpa to the Khumbu valley (Bhandari et al., 2015).
There is a long history of migration from the Tibetan plateau to Nepal. To illustrate, genomic analysis of human dental samples (dating to between 1,700 and 3,000 YBP) from a northern region of Nepal show strong affinity for contemporary Tibetans (Jeong et al., 2016). Analysis of both autosomal data (Lu et al., 2016; Gnecchi-Ruscone et al., 2017) and uniparental mtDNA and Y-chromosome markers (Bhandari et al., 2015) have shown the Sherpa and Tibetans to share relatively recent common ancestry. Tibetans also share recent common ancestry with other Nepalese populations including the Rai, Magar, Tamang, and Gurung (Cole et al., 2017). The Sherpa share more genetic affinity with these Tibeto-Burman speaking populations than with other Indo-Aryan populations of Nepal. However, the Sherpa are distinct from other Nepalese populations in that the Sherpa have elevated levels of runs of homozygosity (Cole et al., 2017), and illustrate very little or no admixture with Nepalese or South Asian populations (Cole et al., 2017). Thus, the Khumbu Valley Sherpa can be considered from the perspective of population genetics as a “bottlenecked” population recently derived from Tibetans.
Comparative Physiological Studies Between Sherpa and Lowlanders
In 1952, Griffith Pugh conducted a series of pioneering physiological experiments on Mount Cho Oyo (at 8,188 m, 20 km west of Mount Everest) that suggested a superior work capacity of the Sherpa at high altitude (Pugh, 1962; Pugh et al., 1964). They also provided the scientific rationale for the hydration, nutrition and oxygen requirements for the first Everest summiting in 1953 (Milledge, 2002). Although the physiology of the Sherpa has been studied over the intervening 60 years, the scientific literature is limited in number, and most of the studies are based on small sample sizes. There are obvious challenges to studying the Sherpa; they reside in a remote region, at an altitude over 2,800 m, where altitude sickness is common for sojourners. Despite this, several remarkable findings have emerged and below we discuss specific phenotypes that may be linked to hypoxia-related genetic signals of selection reported to date. For a discussion of other hypoxia-related physiological parameters studied in Sherpa, such as ventilation, lung volume, exercise capacity and cerebral function (see Gilbert-Kawai et al., 2014; Table 1).
Hemoglobin Concentration
The hypoxic challenge presented by high altitude drives changes in hemoglobin concentration. Elevated hemoglobin levels (≥19 g/dl in females; and ≥21 g/dl in males) resulting from hypoxia can lead to chronic mountain sickness (Leon-Velarde et al., 2005). Relative to lowland controls, the literature suggests the Sherpa display lower hemoglobin concentrations at high altitude (Beall and Reichsman, 1984; Wu et al., 2013; Bhandari et al., 2016). Sherpa women with lower hemoglobin concentrations (13.8 g/dl ± 1.3 g/dl) are reported to have better reproductive outcomes (Beall et al., 1997, 2004; Cho et al., 2017). Increased exercise capacity has been reported in Tibetan males with a low erythropoietic response (Simonson et al., 2015). It is yet to be determined whether the lower hemoglobin concentration observed in Sherpa is due to a blunted erythropoietic response or to some other physiological parameters that impact hemoglobin concentration.
Nitric Oxide Concentration
Nitric oxide acts as a vasodilator and is believed to protect against pulmonary hypertension at high altitude (Busch et al., 2001). It also plays a role in haematocrit regulation by controlling blood viscosity (Ashmore et al., 2014). Serum nitric oxide levels have been reported as reduced in the Sherpa relative to lowlanders (Droma et al., 2006), and a recent study reported no differences in circulating nitric oxide metabolites [N-nitrosamine (RNNO), S-nitrosothiol, nitrate, or nitrite concentrations] between Sherpa and lowlanders at both low and high altitude (Horscroft et al., 2017). However, a non-synonymous variant (rs549340789) in NOS1 (nitric oxide synthases 1) has been identified as under positive selection in the Sherpa (Zhang et al., 2017). Thus, it seems that nitric oxide may play an important role in hypoxic adaptation (Erzurum et al., 2007; Beall et al., 2012), but the exact mechanisms remain poorly understood.
Microcirculation
Lowlanders exhibit, in a hypoxic environment, reduced sublingual microcirculatory blood flow (Martin et al., 2009). However, the Sherpa maintain sublingual capillary densities and microcirculatory blood flow (Gilbert-Kawai et al., 2017) at altitude. Compared to mountaineers of European-ancestry, during an expedition to Mount Everest, Sherpa exhibit elevated basal levels of angiogenic elements including vascular endothelial growth factor A (VEGF-A), interleukins (IL-8) and lymphangiogenic factors (VEGF-C and D), which likely facilitate increased microcirculatory flow (Patitucci and Lugrin, 2009). The Sherpa display an elevated oxygen unloading rate, and increased myogenic activity relative to lowlanders, further supporting higher peripheral microcirculatory perfusion (Davies et al., 2018). Following a defined period of induced leg occlusion and muscle ischemia, the Sherpa are reported to display increased blood flow velocity, relative to lowlanders (Schneider et al., 2001). This is likely due to differences in conduit vessel function. Thus, the Sherpa appear to exhibit distinct microcirculation patterns, which might facilitate increased tissue oxygen transfer to overcome hypoxia.
Pulmonary and Cardiac Physiology
The Sherpa have greater spirometry values, forced expiratory volumes and forced vital capacity relative to lowlanders at high altitude (Pugh, 1962; Havryk et al., 2002). Lowlanders often experience apnea-induced brady-arrhythmias at high altitude, while Sherpa typically do not (Busch et al., 2017). The Sherpa display lower pulmonary vascular resistance and smaller left ventricular end-diastolic volume (Stembridge et al., 2014). However, the mechanism by which this reduced myocardial relaxation impacts on the exercise capacity of the Sherpa is unclear (Stembridge et al., 2015).
Evidence suggests a shift in cardiac substrate preference, from fat to glucose, in Sherpa relative to lowland controls (Holden et al., 1995). Some patients with heart failure display a reduction of the myocardial PCr to ATP ratio (Neubauer et al., 1997). Lowlanders returning from high altitude also display a significant decrease in myocardial PCr/ATP ratio (Holloway et al., 2011), but this ratio remains steady in the Sherpa (Hochachka et al., 1996a).
Skeletal Muscle
Sherpa muscle contains a significantly greater number of capillaries per cross-sectional area, in comparison to lowlanders (Kayser et al., 1991). Sherpa also display a reduced mitochondrial content, but their muscle is somehow maximizing the oxygen consumption to mitochondrial volume ratio (Kayser et al., 1991; Horscroft et al., 2017). Under hypoxia, Sherpa skeletal muscle prefers carbohydrate over fatty acids as a metabolic substrate (Murray, 2009). Sherpa muscle maintains fatty acid oxidation relative to lowlanders at high altitude. Incomplete fatty acid oxidation results in production of byproducts such as acylcarnitines and reactive oxygen species. Acylcarnitines and markers of oxidative stress (e.g., reduced/oxidized glutathione and methionine sulfoxide) are increased in lowlander muscle relative to the Sherpa (Gelfi et al., 2004; Horscroft et al., 2017). However, oxidative damage in lowlanders was reduced to levels comparable with the Sherpa, where acclimatization has taken place (Janocha et al., 2017). Lactate dehydrogenase activity is elevated in Sherpa muscle (Allen et al., 1997; Horscroft et al., 2017), indicating greater capacity for anaerobic lactate production. With increasing altitude, lowlanders experience a gradual reduction in phosphocreatine (PCr) and ATP levels (Levett et al., 2015). But the Sherpa maintain PCr and ATP levels at altitude (Horscroft et al., 2017). Thus, the superior muscle energetics displayed by the Sherpa is probably the result of adaptation at the metabolic level.
Birth Weight
Women of European and Han Chinese ancestry exhibit reduced birth weights following gestation at high altitude, quantified at 100 g reduction for every 1,000 m elevation (Moore, 2003; Julian et al., 2009; Moore et al., 2011). The Sherpa (and Tibetans), however, maintain normal birth weight at both low (1,330 m) and high (3,930 m) altitude (Smith, 1997; Moore et al., 2001). Genes including PPARA are expressed in the placenta (Barak et al., 2008) and have been shown to influence female reproductive function (Bogacka et al., 2015). HIFs play a critical role in mammalian embryo and placental development (Dunwoodie, 2009; Pringle et al., 2009). EPAS1 expression appears reduced in umbilical endothelial cells and placentas of Tibetan women (Peng et al., 2017). Intronic variants in CCDC141 have been shown in Tibetan and Sherpa women to associate with the number of live births, and the same locus also shows evidence of positive selection (Jeong et al., 2018). The increased reproductive success of the Sherpa is therefore likely to be, at least in part, due to cardiac-related traits (Jeong et al., 2018) and placental adaptation (Burton et al., 2016). Further studies are required to understand the molecular mechanisms by which the Sherpa maintain normal intrauterine growth at altitude.
In summary, the Sherpa display distinct physiological responses to hypoxia that contrast to lowlanders at high altitude (Table 1). These are presumably the result of exposure over many generations to the hypoxia-related selective pressure presented by the Tibetan plateau. Indeed, some examples have already emerged of specific genetic signatures of selection associating with distinct adapative traits (Simonson, 2015; Moore, 2017).
Signatures of Altitude-Related Genetic Selection in the Sherpa
With developments in sequencing and genotyping technology over the past decade, it has become possible to identify population-specific signatures of selection for adaptation across the human genome. There are now several complementary genomic tests available for detecting genetic selection (Scheinfeldt and Tishkoff, 2013) and the application of these tests to data from indigenous high-altitude people including the Sherpa have identified numerous and remarkable genetic signals of selection. Here, we focus on the three most robust signals of selection detected to date in the Sherpa: EPAS1, EGLN1, and PPARA (Table 2).
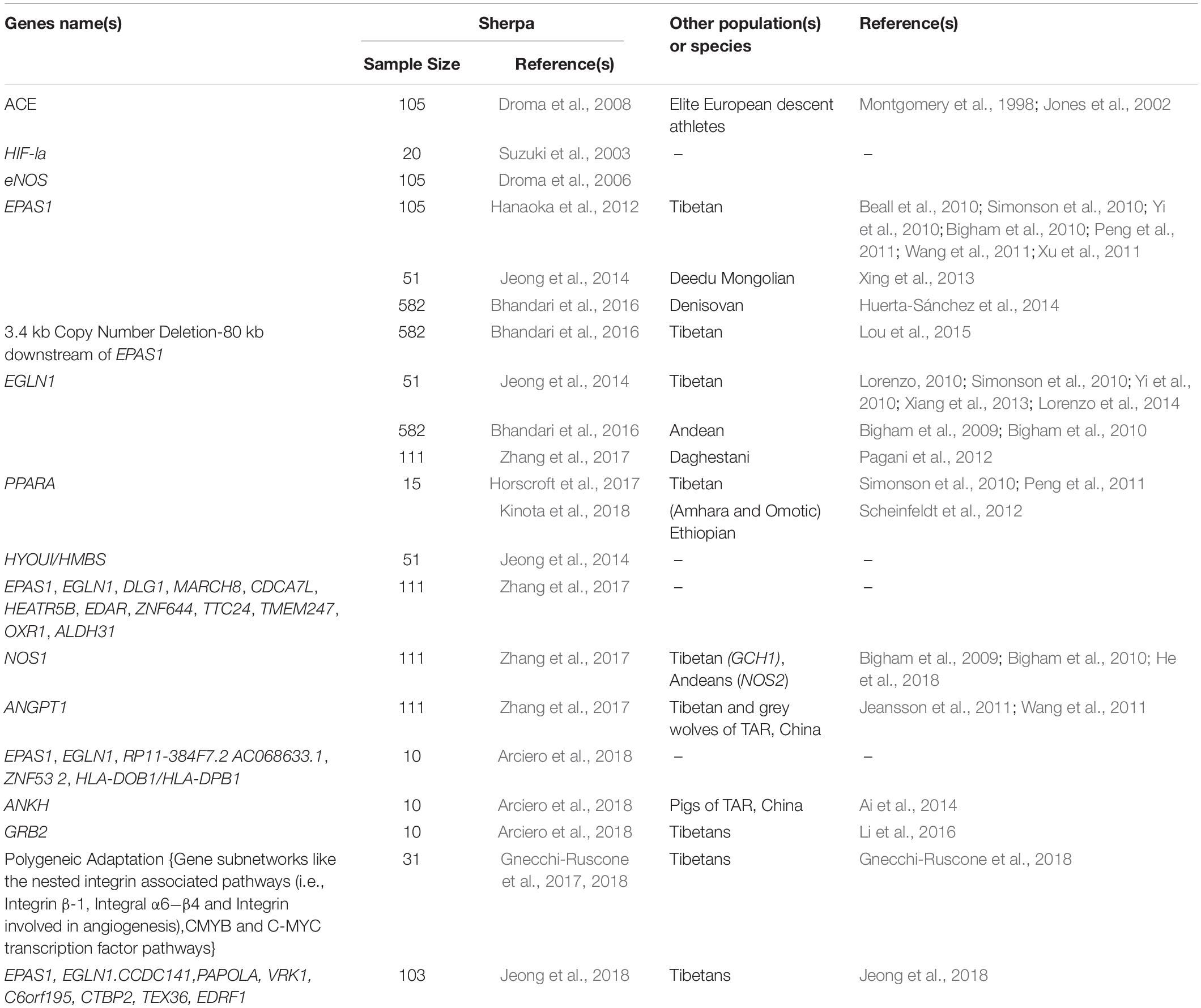
Table 2. A summary of genetic adaptations reported in the Sherpa, and replication in other population(s) or species.
Endothelial PAS Domain-Containing Protein 1 (EPAS1)
One of the earliest signals for altitude-related adaptation to emerge from genomic selection studies was EPAS1. Initially discovered in Tibetans (Beall et al., 2010), the EPAS1 signal has been replicated in multiple other Tibetan populations (Bigham et al., 2010; Simonson et al., 2010; Yi et al., 2010; Peng et al., 2011; Wang et al., 2011; Xu et al., 2011) as well as the Sherpa (Hanaoka et al., 2012; Jeong et al., 2014; Bhandari et al., 2016). The selected EPAS1 haplotype is associated with lowered hemoglobin concentrations (Beall et al., 2010). Remarkably, it seems the adaptive EPAS1 haplotype likely descends from an introgression event with the Denisovan people, an extinct species of archaic humans (Huerta-Sánchez et al., 2014; Hu et al., 2017). A 3.4 kb copy number deletion, downstream of EPAS1, is elevated in frequency, in Tibetans and Sherpas relative to lowland controls (Lou et al., 2015). This deletion is in strong linkage disequilibrium with the previously reported (Beall et al., 2010) EPAS1 haplotype and has also been associated with lower hemoglobin levels. The actual functional EPAS1 variant(s) that are conferring advantage in relation to hypoxic adaptation remain unknown. However, the intronic and intergenic location of the selected variants would be consistent with a role in HIF-related transcriptional regulation.
EPAS1 encodes the HIF2 alpha subunit of HIF2. The postnatal deletion of EPAS1 in adult mice causes anaemia (Gruber et al., 2007). Some cases of erythrocytosis are caused by missense mutations (e.g., G536W) in EPAS1 (Percy et al., 2008). Mice carrying the EPAS1 G536W mutation display excessive erythrocytosis and pulmonary hypertension (Tan et al., 2013). Another study in heterozygous EPAS1 knockout mice reported a blunted physiological response to chronic hypoxia (Peng et al., 2017). Further in-vivo and in-vitro studies are necessary to understand how the adaptive version of the EPAS1 gene is shaping human adaptation to altitude.
Egl-9 Family Hypoxia Inducible Factor 1 (EGLN1)
Another high altitude genetic selection signal to emerge from early studies on Tibetans was EGLN1 (Simonson et al., 2010; Yi et al., 2010). Similar to EPAS1, this signal was later demonstrated in the Sherpa (Jeong et al., 2014). Two functional EGLN1 mutations (rs12097901, D4E, and rs186996510, S127C) appear to be driving the selection signal and are present in both Sherpa (Bhandari et al., 2016) and Tibetans (Lorenzo, 2010; Xiang et al., 2013; Lorenzo et al., 2014). Whether the mode of action of these two mutations is via gain of function (Lorenzo et al., 2014) or loss of function (Song et al., 2014) remains unclear.
EGLN1 encodes proline hydroxylase 2 (PHD2), an isoform of HIF prolyl-hydroxylase. Homozygous knockout PHD2 mice are unviable and die at the embryonic stage due to severe placental defects (Takeda et al., 2006). Knockout mice with PHD2 disruption targeted to specific organs including the liver, heart, kidney and lung develop excessive vascular growth (Takeda et al., 2007). Adult mice deficient for PHD2 display excessive erythrocytosis (Takeda et al., 2008) and heterozygous PHD2 mice have an increased ventilatory sensitivity to hypoxia and carotid body hyperplasia (Bishop et al., 2013).
Peroxisome Proliferator-Activated Nuclear Receptor A (PPARA)
PPARA encodes PPARα, a transcriptional regulator of fatty acid oxidation in liver, heart and muscle (Gilde and Van Bilsen, 2003). PPARA has tissue-specific expression and, under hypoxic conditions, is downregulated by HIFs (Narravula and Colgan, 2001). Positive selection across the PPARA gene has been reported in Tibetans (Simonson et al., 2010) and Sherpa (Horscroft et al., 2017), and the selected PPARA SNPs correlate with reduced hemoglobin levels (Simonson et al., 2010). Sherpa carriers of the positively selected PPARA alleles switch to more efficient fuels such as glucose and display decreased muscular fatty acid oxidation (Horscroft et al., 2017). Most of the PPARA SNPs reported to be under selection appear to be non-coding variants (Kinota et al., 2018). It is unclear if these variants directly affect transcriptional regulation or are linked with functional variants in other genes or nearby inter-genic regions.
Conclusion
The Sherpa show remarkable performance in the hypoxic environment presented by high altitude. Comparative physiological studies have suggested numerous distinct, adaptive phenotypes in the Sherpa including advantageous levels of hemoglobin, oxygen saturation and birth weight, and the elevated reproductive success of Sherpa women. Genomic studies have identified robust signals of positive selection across genes including EPAS1, EGLN1 and PPARA. All three of these signals of genetic selection have been shown to correlate with advantageous levels of hemoglobin. However, Sherpa-specific signals of genetic selection have also been reported, suggesting that whilst some of the genetic basis for adaptation in the Sherpa is shared with Tibetans, there may be features unique to the Sherpa, which could in turn explain distinct Sherpa phenotypes. Collectively, this illustrates how the outstanding physiological performance of the Sherpa at altitude is, at least in part, a result of hypoxia driven genetic selection spanning the ∼35,000 years of seasonal migration on the Himalayan plateau. Further comparative physiological studies are required to refine existing, and identify additional adaptive phenotypes, in particular those that are specific to the Sherpa. By correlating these phenotypes with known and emerging signals of genetic selection, we can shed light on biological mechanisms of Sherpa hypoxic adaptation. Ultimately this work can inform on treatments of hypoxia-related illness including pulmonary, cardiac, neurological and renal disorders.
Author Contributions
Both authors drafted, edited, and approved the final version of the manuscript.
Funding
SB was supported by a Government of Ireland Postdoctoral Fellowship from the Irish Research Council (GOIPD/2018/408). This work was also supported by an Investigators Programme grant from Science Foundation Ireland (12/IP/1727).
Conflict of Interest Statement
The authors declare that the research was conducted in the absence of any commercial or financial relationships that could be construed as a potential conflict of interest.
References
Adams, W. H., and Shresta, S. M. (1974). Hemoglobin levels, vitamin B12, and folate status in a Himalayan village. Am. J. Clin. Nutr. 27, 217–219. doi: 10.1093/ajcn/27.2.217
Adams, W. H., and Strang, L. J. (1975). Hemoglobin levels in persons of Tibetan ancestry living at high altitude. Exp. Biol. Med. 149, 1036–1039. doi: 10.3181/00379727-149-38952
Ai, H., Yang, B., Li, J., Xie, X., Chen, H., and Ren, J. (2014). Population history and genomic signatures for high-altitude adaptation in Tibetan pigs. BMC Genomics 15:834. doi: 10.1186/1471-2164-15-834
Aldenderfer, M. (2011). Peopling the Tibetan plateau: insights from archaeology. High Alt. Med. Biol. 12, 141–147. doi: 10.1089/ham.2010.1094
Allen, P., Matheson, G., Zhu, G., Gheorgiu, D., Dunlop, R., and Falconer, T., et al. (1997). Simultaneous 31P MRS of the soleus and gastrocnemius in Sherpas during graded calf muscle exercise. Am. J. Physiol. Regul. Integr. Comp. Physiol. 273, R999–R1007
Arciero, E., Kraaijenbrink, T., Haber, M., Mezzavilla, M., Ayub, Q., Wang, W., et al. (2018). Demographic history and genetic adaptation in the Himalayan region inferred from genome-wide SNP genotypes of 49 populations. Mol. Biol. Evol. 35, 1916–1933. doi: 10.1093/molbev/msy094
Ashmore, T., Fernandez, B. O., Evans, C. E., Huang, Y., Branco-Price, C., Griffin, J. L., et al. (2014). Suppression of erythropoiesis by dietary nitrate. FASEB J. 29, 1102–1112. doi: 10.1096/fj.14-263004
Bailey, D. M., Dehnert, C., Luks, A. M., Menold, E., Castell, C., Schendler, G., et al. (2010). High-altitude pulmonary hypertension is associated with a free radical-mediated reduction in pulmonary nitric oxide bioavailability. J. Physiol. 588, 4837–4847. doi: 10.1113/jphysiol.2010.194704
Barak, Y., Sadovsky, Y., and Shalom-Barak, T. (2008). PPAR signaling in placental development and function. PPAR Res. 2008:142082. doi: 10.1155/2008/142082
Bastien, G., Willems, P., Schepens, B., and Heglund, N. (2016). The mechanics of head-supported load carriage by Nepalese porters. J. Exp. Biol. 219, 3626–3634. doi: 10.1242/jeb.143875
Bastien, G. J., Schepens, B., Willems, P. A., and Heglund, N. C. (2005a). Energetics of load carrying in Nepalese porters. Science 308, 1755–1755. doi: 10.1126/science.1111513
Bastien, G. J., Willems, P. A., Schepens, B., and Heglund, N. C. (2005b). Effect of load and speed on the energetic cost of human walking. Eur. J. Appl. Physiol. 94, 76–83. doi: 10.1007/s00421-004-1286-z
Beall, C., and Reichsman, A. (1984). Hemoglobin levels in a Himalayan high altitude population. Am. J. Phys. Anthropol. 63, 301–306. doi: 10.1002/ajpa.1330630306
Beall, C. M., Cavalleri, G. L., Deng, L., Elston, R. C., Gao, Y., Knight, J., et al. (2010). Natural selection on EPAS1 (HIF2α) associated with low hemoglobin concentration in Tibetan highlanders. Proc. Natl. Acad. Sci. U.S.A. 107, 11459–11464. doi: 10.1073/pnas.1002443107
Beall, C. M., Laskowski, D., and Erzurum, S. C. (2012). Nitric oxide in adaptation to altitude. Free Radic. Biol. Med. 52, 1123–1134. doi: 10.1016/j.freeradbiomed.2011.12.028
Beall, C. M., Song, K., Elston, R. C., and Goldstein, M. C. (2004). Higher offspring survival among Tibetan women with high oxygen saturation genotypes residing at 4,000 m. Proc. Natl. Acad. Sci. U.S.A. 101, 14300–14304. doi: 10.1073/pnas.0405949101
Beall, C. M., Strohl, K. P., Blangero, J., Williams-Blangero, S., Decker, M. J., Brittenham, G. M., et al. (1997). Quantitative genetic analysis of arterial oxygen saturation in Tibetan highlanders. Hum. Biol. 69, 597–604.
Bhandari, S., Zhang, X., Cui, C., Liao, S., Peng, Y., Zhang, H., et al. (2015). Genetic evidence of a recent Tibetan ancestry to Sherpas in the Himalayan region. Sci. Rep. 5:16249. doi: 10.1038/srep16249
Bhandari, S., Zhang, X., Cui, C., Yangla Liu, L., and Ouzhuluobu, Baimakangzhuo, et al. (2016). Sherpas share genetic variations with Tibetans for high-altitude adaptation. Mol. Genet. Genom. Med. 5, 76–84. doi: 10.1002/mgg3.264
Bigham, A., Bauchet, M., Pinto, D., Mao, X., Akey, J. M., Mei, R., et al. (2010). Identifying signatures of natural selection in Tibetan and Andean populations using dense genome scan data. PLoS Genet 6:e1001116. doi: 10.1371/journal.pgen.1001116
Bigham, A. W., Mao, X., Mei, R., Brutsaert, T., Wilson, M. J., Julian, C. G., et al. (2009). Identifying positive selection candidate loci for high-altitude adaptation in Andean populations. Hum. Genomics 4, 79–90.
Bishop, T., Talbot, N. P., Turner, P. J., Nicholls, L. G., Pascual, A., Hodson, E. J., et al. (2013). Carotid body hyperplasia and enhanced ventilatory responses to hypoxia in mice with heterozygous deficiency of PHD2. J. Physiol. 591, 3565–3577. doi: 10.1113/jphysiol.2012.247254
Bogacka, I., Kurzynska, A., Bogacki, M., and Chojnowska, K. (2015). Peroxisome proliferator-activated receptors in the regulation of female reproductive functions. Folia Histochem. Cytobiol. 53, 189–200. doi: 10.5603/fhc.a2015.0023
Boyer, S. J., and Blume, F. D. (1984). Weight loss and changes in body composition at high altitude. J. Appl. Physiol. 57, 1580–1585. doi: 10.1152/jappl.1984.57.5.1580
Brooks, G., Butterfield, G., Wolfe, R., Groves, B., Mazzeo, R., Sutton, J., et al. (1991). Increased dependence on blood glucose after acclimatization to 4,300 m. J. Appl. Physiol. 70, 919–927. doi: 10.1152/jappl.1991.70.2.919
Bruno, R. M., Cogo, A., Ghiadoni, L., Duo, E., Pomidori, L., Sharma, R., et al. (2014). Cardiovascular function in healthy Himalayan high-altitude dwellers. Atherosclerosis 236, 47–53. doi: 10.1016/j.atherosclerosis.2014.06.017
Burton, G. J., Fowden, A. L., and Thornburg, K. L. (2016). Placental origins of chronic disease. Physiol. Rev. 96, 1509–1565. doi: 10.1152/physrev.00029.2015
Burtscher, M., Gatterer, H., Philadelphy, M., Burtscher, J., and Likar, R. (2018). Submaximal exercise testing at low altitude for prediction of exercise tolerance at high altitude. J. Travel Med. 25:tay011. doi: 10.1093/jtm/tay011
Burtscher, M., Philadelphy, M., Gatterer, H., Burtscher, J., Faulhaber, M., Nachbauer, W., et al. (2019). Physiological responses in humans acutely exposed to high altitude (3480?m): minute ventilation and oxygenation are predictive for the development of acute mountain sickness. High Alt. Med. Biol. 20, 192–197. doi: 10.1089/ham.2018.0143
Busch, S. A., Davies, H., Van Diepen, S., Simpson, L. L., Sobierajski, F., Riske, L., et al. (2017). Chemoreflex mediated arrhythmia during apnea at 5,050 m in low-but not high-altitude natives. J. Appl. Physiol. 124, 930–937. doi: 10.1152/japplphysiol.00774.2017
Busch, T., Bartsch, P., Pappert, D., Grunig, E., Hildebrandt, W., Elser, H., et al. (2001). Hypoxia decreases exhaled nitric oxide in mountaineers susceptible to high-altitude pulmonary edema. Am. J. Respir. Crit. Care Med. 163, 368–373. doi: 10.1164/ajrccm.163.2.2001134
Cho, J. I., Basnyat, B., Jeong, C., Di Rienzo, A., Childs, G., Craig, S. R., et al. (2017). Ethnically Tibetan women in Nepal with low hemoglobin concentration have better reproductive outcomes. Evol. Med. Public Health 2017, 82–96. doi: 10.1093/emph/eox008
Cole, A. M., Cox, S., Jeong, C., Petousi, N., Aryal, D. R., Droma, Y., et al. (2017). Genetic structure in the Sherpa and neighboring Nepalese populations. BMC Genomics 18:102. doi: 10.1186/s12864-016-3469-5
Cumpstey, A. F., Hennis, P. J., Gilbert-Kawai, E. T., Fernandez, B. O., Poudevigne, M., Cobb, A., et al. (2017). Effects of dietary nitrate on respiratory physiology at high altitude - Results from the Xtreme Alps study. Nitric Oxide 71, 57–68. doi: 10.1016/j.niox.2017.10.005
Cumpstey, A. F., Minnion, M., Fernandez, B. O., Mikus-Lelinska, M., Mitchell, K., Martin, D. S., et al. (2019). Pushing arterial-venous plasma biomarkers to new heights: a model for personalised redox metabolomics? Redox Biol. 21:101113. doi: 10.1016/j.redox.2019.101113
Davenport, M. H., Steinback, C. D., Borle, K. J., Matenchuk, B. A., Vanden Berg, E. R., De Freitas, E. M., et al. (2018). Extreme pregnancy: maternal physical activity at Everest Base Camp. J. Appl. Physiol. 125, 580–585. doi: 10.1152/japplphysiol.00146.2018
Davies, T., Gilbert-Kawai, E., Wythe, S., Meale, P., Mythen, M., Levett, D., et al. (2018). Sustained vasomotor control of skin microcirculation in Sherpas versus altitude-naive lowlanders: experimental evidence from Xtreme Everest 2. Exp. Physiol. 103, 1494–1504. doi: 10.1113/EP087236
Droma, Y., Hanaoka, M., Basnyat, B., Arjyal, A., Neupane, P., Pandit, A., et al. (2006). Genetic contribution of the endothelial nitric oxide synthase gene to high altitude adaptation in sherpas. High Alt. Med. Biol. 7, 209–220. doi: 10.1089/ham.2006.7.209
Droma, Y., Hanaoka, M., Basnyat, B., Arjyal, A., Neupane, P., Pandit, A., et al. (2008). Adaptation to high altitude in Sherpas: association with the insertion/deletion polymorphism in the Angiotensin-converting enzyme gene. Wilderness Environ. Med. 19, 22–29. doi: 10.1580/06-WEME-OR-073.1
Dunwoodie, S. L. (2009). The role of hypoxia in development of the Mammalian embryo. Dev. Cell 17, 755–773. doi: 10.1016/j.devcel.2009.11.008
Edwards, L. M., Murray, A. J., Tyler, D. J., Kemp, G. J., Holloway, C. J., Robbins, P. A., et al. (2010). The effect of high-altitude on human skeletal muscle energetics: 31 P-MRS results from the Caudwell Xtreme Everest expedition. PLoS One 5:e10681. doi: 10.1371/journal.pone.0010681
Erzurum, S., Ghosh, S., Janocha, A., Xu, W., Bauer, S., Bryan, N., et al. (2007). Higher blood flow and circulating NO products offset high-altitude hypoxia among Tibetans. Proc. Natl. Acad. Sci. U.S.A. 104, 17593–17598. doi: 10.1073/pnas.0707462104
Faoro, V., Huez, S., Vanderpool, R., Groepenhoff, H., De Bisschop, C., Martinot, J. B., et al. (2014). Pulmonary circulation and gas exchange at exercise in Sherpas at high altitude. J. Appl. Physiol. 116, 919–926. doi: 10.1152/japplphysiol.00236.2013
Garrido, E., Segura, R., Capdevila, A., Pujol, J., Javierre, C., and Ventura, J. L. (1996). Are Himalayan Sherpas better protected against brain damage associated with extreme altitude climbs? Clin. Sci. 90, 81–85. doi: 10.1042/cs0900081
Gelfi, C., De Palma, S., Ripamonti, M., Eberini, I., Wait, R., Bajracharya, A., et al. (2004). New aspects of altitude adaptation in Tibetans: a proteomic approach. FASEB J. 18, 612–614. doi: 10.1096/fj.03-1077fje
Gilbert-Kawai, E., Coppel, J., Court, J., Van Der Kaaij, J., Vercueil, A., Feelisch, M., et al. (2017). Sublingual microcirculatory blood flow and vessel density in Sherpas at high altitude. J. Appl. Physiol. 122, 1011–1018. doi: 10.1152/japplphysiol.00970.2016
Gilbert-Kawai, E., Sheperdigian, A., Adams, T., Mitchell, K., Feelisch, M., Murray, A., et al. (2015). Design and conduct of Xtreme Everest 2: an observational cohort study of Sherpa and lowlander responses to graduated hypobaric hypoxia. F1000Res. 4:90. doi: 10.12688/f1000research.6297.1
Gilbert-Kawai, E. T., Milledge, J. S., Grocott, M. P., and Martin, D. S. (2014). King of the mountains: Tibetan and Sherpa physiological adaptations for life at high altitude. Physiology 29, 388–402. doi: 10.1152/physiol.00018.2014
Gilde, A., and Van Bilsen, M. (2003). Peroxisome proliferator-activated receptors (PPARS): regulators of gene expression in heart and skeletal muscle. Acta Physiol. Scand. 178, 425–434. doi: 10.1046/j.1365-201x.2003.01161.x
Gill, M., and Pugh, L. (1964). Basal metabolism and respiration in men living at 5,800 m (19,000 ft). J. Appl. Physiol. 19, 949–954. doi: 10.1152/jappl.1964.19.5.949
Gnecchi-Ruscone, G. A., Abondio, P., De Fanti, S., Sarno, S., Sherpa, M. G., Sherpa, P. T., et al. (2018). Evidence of polygenic adaptation to high altitude from Tibetan and Sherpa genomes. Genome Biol. Evol. 10, 2919–2930. doi: 10.1093/gbe/evy233
Gnecchi-Ruscone, G. A., Jeong, C., De Fanti, S., Sarno, S., Trancucci, M., Gentilini, D., et al. (2017). The genomic landscape of Nepalese Tibeto-Burmans reveals new insights into the recent peopling of Southern Himalayas. Sci. Rep. 7:15512. doi: 10.1038/s41598-017-15862-z
Grocott, M. P., Martin, D. S., Levett, D. Z., Mcmorrow, R., Windsor, J., and Montgomery, H. E. (2009). Arterial blood gases and oxygen content in climbers on Mount Everest. New Engl. J. Med. 360, 140–149. doi: 10.1056/NEJMoa0801581
Groves, B. M., Droma, T., Sutton, J. R., Mccullough, R. G., Mccullough, R. E., Zhuang, J., et al. (1993). Minimal hypoxic pulmonary hypertension in normal Tibetans at 3,658 m. J. Appl. Physiol. 74, 312–318. doi: 10.1152/jappl.1993.74.1.312
Gruber, M., Hu, C.-J., Johnson, R. S., Brown, E. J., Keith, B., and Simon, M. C. (2007). Acute postnatal ablation of Hif-2α results in anemia. Proc. Natl. Acad. Sci. U.S.A. 104, 2301–2306. doi: 10.1073/pnas.0608382104
Hackett, P. H., Reeves, J. T., Reeves, C. D., Grover, R. F., and Rennie, D. (1980). Control of breathing in Sherpas at low and high altitude. J. Appl. Physiol. 49, 374–379. doi: 10.1152/jappl.1980.49.3.374
Hanaoka, M., Droma, Y., Basnyat, B., Ito, M., Kobayashi, N., Katsuyama, Y., et al. (2012). Genetic variants in EPAS1 contribute to adaptation to high-altitude hypoxia in Sherpas. PLoS One 7:e50566. doi: 10.1371/journal.pone.0050566
Havryk, A. P., Gilbert, M., and Burgess, K. R. (2002). Spirometry values in Himalayan high altitude residents (Sherpas). Respir. Physiol. Neurobiol. 132, 223–232. doi: 10.1016/s1569-9048(02)00072-1
He, Y., Qi, X., Na, O., Liu, S., Li, J., Zhang, H., et al. (2018). Blunted nitric oxide regulation in tibetans under high altitude hypoxia. Natl. Sci. Rev. 5, 516–529. doi: 10.1093/nsr/nwy037
Hilty, M. P., Merz, T. M., Hefti, U., Ince, C., Maggiorini, M., and Pichler Hefti, J. (2019). Recruitment of non-perfused sublingual capillaries increases microcirculatory oxygen extraction capacity throughout ascent to 7126 m. J. Physiol. 597, 2623–2638. doi: 10.1113/JP277590
Hochachka, P. W., Clark, C. M., Holden, J. E., Stanley, C., Ugurbil, K., and Menon, R. S. (1996a). 31P magnetic resonance spectroscopy of the Sherpa heart: a phosphocreatine/adenosine triphosphate signature of metabolic defense against hypobaric hypoxia. Proc. Natl. Acad. Sci. U.S.A. 93, 1215–1220. doi: 10.1073/pnas.93.3.1215
Hochachka, P. W., Clark, C. M., Monge, C., Stanley, C., Brown, W., Stone, C., et al. (1996b). Sherpa brain glucose metabolism and defense adaptations against chronic hypoxia. J. Appl. Physiol. 81, 1355–1361. doi: 10.1152/jappl.1996.81.3.1355
Holden, J., Stone, C., Clark, C., Brown, W., Nickles, R., Stanley, C., et al. (1995). Enhanced cardiac metabolism of plasma glucose in high-altitude natives: adaptation against chronic hypoxia. J. Appl. Physiol. 79, 222–228. doi: 10.1152/jappl.1995.79.1.222
Holloway, C. J., Montgomery, H. E., Murray, A. J., Cochlin, L. E., Codreanu, I., Hopwood, N., et al. (2011). Cardiac response to hypobaric hypoxia: persistent changes in cardiac mass, function, and energy metabolism after a trek to Mt. Everest Base Camp. FASEB J. 25, 792–796. doi: 10.1096/fj.10-172999
Horscroft, J. A., Kotwica, A. O., Laner, V., West, J. A., Hennis, P. J., Levett, D. Z., et al. (2017). Metabolic basis to Sherpa altitude adaptation. Proc. Natl. Acad. Sci. U.S.A. 114, 6382–6387. doi: 10.1073/pnas.1700527114
Hu, H., Petousi, N., Glusman, G., Yu, Y., Bohlender, R., Tashi, T., et al. (2017). Evolutionary history of Tibetans inferred from whole-genome sequencing. PLoS Genetics 13:e1006675. doi: 10.1371/journal.pgen.1006675
Huerta-Sánchez, E., Jin, X., Bianba, Z., Peter, B. M., Vinckenbosch, N., Liang, Y., et al. (2014). Altitude adaptation in Tibetans caused by introgression of Denisovan-like DNA. Nature 512, 194–197. doi: 10.1038/nature13408
Janocha, A. J., Comhair, S. A. A., Basnyat, B., Neupane, M., Gebremedhin, A., Khan, A., et al. (2017). Antioxidant defense and oxidative damage vary widely among high-altitude residents. Am. J. Hum. Biol. 29:e23039. doi: 10.1002/ajhb.23039
Jansen, G. F., Krins, A., Basnyat, B., Bosch, A., and Odoom, J. A. (2000). Cerebral autoregulation in subjects adapted and not adapted to high altitude. Stroke 31, 2314–2318. doi: 10.1161/01.str.31.10.2314
Jansen, G. F., Krins, A., Basnyat, B., Odoom, J. A., and Ince, C. (2007). Role of the altitude level on cerebral autoregulation in residents at high altitude. J. Appl. Physiol. 103, 518–523. doi: 10.1152/japplphysiol.01429.2006
Jeansson, M., Gawlik, A., Anderson, G., Li, C., Kerjaschki, D., Henkelman, M., et al. (2011). Angiopoietin-1 is essential in mouse vasculature during development and in response to injury. J. Clin. Invest. 121, 2278–2289. doi: 10.1172/JCI46322
Jeong, C., Alkorta-Aranburu, G., Basnyat, B., Neupane, M., Witonsky, D. B., Pritchard, J. K., et al. (2014). Admixture facilitates genetic adaptations to high altitude in Tibet. Nat. Commun. 5:3281. doi: 10.1038/ncomms4281
Jeong, C., Ozga, A. T., Witonsky, D. B., Malmström, H., Edlund, H., Hofman, C. A., et al. (2016). Long-term genetic stability and a high-altitude East Asian origin for the peoples of the high valleys of the Himalayan arc. Proc. Natl. Acad. Sci. 113, 7485–7490. doi: 10.1073/pnas.1520844113
Jeong, C., Witonsky, D. B., Basnyat, B., Neupane, M., Beall, C. M., Childs, G., et al. (2018). Detecting past and ongoing natural selection among ethnically Tibetan women at high altitude in Nepal. PLoS Genetics 14:e1007650. doi: 10.1371/journal.pgen.1007650
Jones, A., Montgomery, H. E., and Woods, D. R. (2002). Human performance: a role for the ACE genotype? Exerc. Sport Sci. Rev. 30, 184–190. doi: 10.1097/00003677-200210000-00008
Julian, C. G., Wilson, M. J., and Moore, L. G. (2009). Evolutionary adaptation to high altitude: a view from in utero. Am. J. Hum. Biol. 21, 614–622. doi: 10.1002/ajhb.20900
Kang, L., Zheng, H.-X., Chen, F., Yan, S., Liu, K., Qin, Z., et al. (2013). mtDNA lineage expansions in Sherpa population suggest adaptive evolution in Tibetan highlands. Mol. Biol. Evol. 30, 2579–2587. doi: 10.1093/molbev/mst147
Kayser, B., Hoppeler, H., Claassen, H., and Cerretelli, P. (1991). Muscle structure and performance capacity of Himalayan Sherpas. J. Appl. Physiol. 70, 1938–1942. doi: 10.1152/jappl.1991.70.5.1938
Kinota, F., Droma, Y., Kobayashi, N., Horiuchi, T., Kitaguchi, Y., Yasuo, M., et al. (2018). The contribution of genetic variants of the peroxisome proliferator-activated receptor-alpha gene to high-altitude hypoxia adaptation in Sherpa highlanders. High Alt. Med. Biol. [Epub ahead of print].
Lahiri, S., and Milledge, J. (1967). Acid-base in Sherpa altitude residents and lowlanders at 4880 m. Respir. Physiol. 2, 323–334. doi: 10.1016/0034-5687(67)90037-0
Lahiri, S., Milledge, J. S., Chattopadhyay, H. P., Bhattacharyya, A. K., and Sinha, A. K. (1967). Respiration and heart rate of Sherpa highlanders during exercise. J. Appl. Physiol. 23, 545–554. doi: 10.1152/jappl.1967.23.4.545
Leacy, J. K., Zouboules, S. M., Mann, C. R., Peltonen, J. D. B., Saran, G., Nysten, C. E., et al. (2018). Neurovascular coupling remains intact during incremental ascent to high altitude (4240 m) in acclimatized healthy volunteers. Front. Physiol. 9:1691. doi: 10.3389/fphys.2018.01691
Leon-Velarde, F., Maggiorini, M., Reeves, J. T., Aldashev, A., Asmus, I., Bernardi, L., et al. (2005). Consensus statement on chronic and subacute high altitude diseases. High Alt. Med. Biol. 6, 147–157. doi: 10.1089/ham.2005.6.147
Levett, D. Z., Vigano, A., Capitanio, D., Vasso, M., De Palma, S., Moriggi, M., et al. (2015). Changes in muscle proteomics in the course of the caudwell research expedition to Mt. Everest. Proteomics 15, 160–171. doi: 10.1002/pmic.201400306
Lewis, N., Bailey, D. M., Dumanoir, G. R., Messinger, L., Lucas, S. J., Cotter, J. D., et al. (2014). Conduit artery structure and function in lowlanders and native highlanders: relationships with oxidative stress and role of sympathoexcitation. J. Physiol. 592, 1009–1024. doi: 10.1113/jphysiol.2013.268615
Li, K., Gesang, L., Dan, Z., and Gusang, L. (2016). Genome-wide transcriptional analysis reveals the protection against hypoxia-induced oxidative injury in the intestine of Tibetans via the inhibition of GRB2/EGFR/PTPN11 pathways. Oxid. Med. Cell. Longev. 2016:6967396. doi: 10.1155/2016/6967396
Lorenzo, F. R. (2010). “Novel PHD2 mutation associated with Tibetan genetic adaptation to high altitude hypoxia,” in Proceedings of the ASH 52nd Annual Meeting, (Orlando, FL: ASH).
Lorenzo, F. R., Huff, C., Myllymäki, M., Olenchock, B., Swierczek, S., Tashi, T., et al. (2014). A genetic mechanism for Tibetan high-altitude adaptation. Nat. Genet. 46, 951–956. doi: 10.1038/ng.3067
Lou, H., Lu, Y., Lu, D., Fu, R., Wang, X., Feng, Q., et al. (2015). A 3.4-kb Copy-Number Deletion near EPAS1 is significantly enriched in high-altitude Tibetans but absent from the denisovan sequence. Am. J. Hum. Genet. 97, 54–66. doi: 10.1016/j.ajhg.2015.05.005
Lu, D., Lou, H., Yuan, K., Wang, X., Wang, Y., Zhang, C., et al. (2016). Ancestral origins and genetic history of Tibetan highlanders. Am. J. Hum. Genet. 99, 580–594. doi: 10.1016/j.ajhg.2016.07.002
Luks, A. M., and Hackett, P. H. (2014). “High altitude and common medical conditions,” in Human Adaptation to Hypoxia, eds E. R. Swenson and P. Bartsch (New York, NY: Springer), 449–477. doi: 10.1007/978-1-4614-8772-2_23
Luks, A. M., Levett, D., Martin, D. S., Goss, C. H., Mitchell, K., Fernandez, B. O., et al. (2017). Changes in acute pulmonary vascular responsiveness to hypoxia during a progressive ascent to high altitude (5300 m). Exp. Physiol. 102, 711–724. doi: 10.1113/EP086083
Lundby, C., Calbet, J., Van Hall, G., Saltin, B., and Sander, M. (2018). Sustained sympathetic activity in altitude acclimatizing lowlanders and high-altitude natives. Scand. J. Med. Sci. Sports 28, 854–861. doi: 10.1111/sms.12976
Marconi, C., Marzorati, M., Grassi, B., Basnyat, B., Colombini, A., Kayser, B., et al. (2004). Second generation Tibetan lowlanders acclimatize to high altitude more quickly than Caucasians. J. Physiol. Lond. 556, 661–671. doi: 10.1113/jphysiol.2003.059188
Martin, D. S., Gilbert-Kawai, E., Levett, D. Z., Mitchell, K., Kumar, Bc. R., Mythen, M. G., et al. (2013). Xtreme Everest 2: unlocking the secrets of the Sherpa phenotype? Extreme Physiol. Med. 2, 30–30. doi: 10.1186/2046-7648-2-30
Martin, D. S., Ince, C., Goedhart, P., Levett, D. Z., Grocott, M. P., and Caudwell Xtreme Everest Research, G. (2009). Abnormal blood flow in the sublingual microcirculation at high altitude. Eur. J. Appl. Physiol. 106, 473–478. doi: 10.1007/s00421-009-1023-8
Milledge, J. S. (2002). The “Silver Hut” expedition—A commentary 40 years later. Wilderness Environ. Med. 13, 55–56. doi: 10.1580/1080-6032(2002)013
Montgomery, H. E., Marshall, R., Hemingway, H., Myerson, S., Clarkson, P., Dollery, C., et al. (1998). Human gene for physical performance. Nature 393, 221–222.
Moore, L. G. (2003). Fetal growth restriction and maternal oxygen transport during high altitude pregnancy. High Alt. Med. Biol. 4, 141–156. doi: 10.1089/152702903322022767
Moore, L. G. (2017). Measuring high-altitude adaptation. J. Appl. Physiol. 123, 1371–1385. doi: 10.1152/japplphysiol.00321.2017
Moore, L. G., Charles, S. M., and Julian, C. G. (2011). Humans at high altitude: hypoxia and fetal growth. Respir. Physiol. Neurobiol. 178, 181–190. doi: 10.1016/j.resp.2011.04.017
Moore, L. G., Young, D., Mccullough, R. E., Droma, T., and Zamudio, S. (2001). Tibetan protection from intrauterine growth restriction (IUGR) and reproductive loss at high altitude. Am. J. Hum. Biol. 13, 635–644. doi: 10.1002/ajhb.1102.abs
Morpurgo, G., Arese, P., Bosia, A., Pescarmona, G., Luzzana, M., and Modiano, G. (1976). Sherpas living permanently at high altitutde: a new pattern of adaptation. Proc. Natl. Acad. Sci. U.S.A. 73, 747–751. doi: 10.1073/pnas.73.3.747
Murray, A. J. (2009). Metabolic adaptation of skeletal muscle to high altitude hypoxia: how new technologies could resolve the controversies. Genome Med. 1, 117–117. doi: 10.1186/gm117
Narravula, S., and Colgan, S. P. (2001). Hypoxia-inducible factor 1-mediated inhibition of peroxisome proliferator-activated receptor α expression during hypoxia. J. Immunol. 166, 7543–7548. doi: 10.4049/jimmunol.166.12.7543
Neubauer, S., Horn, M., Cramer, M., Harre, K., Newell, J. B., Peters, W., et al. (1997). Myocardial phosphocreatine-to-ATP ratio is a predictor of mortality in patients with dilated cardiomyopathy. Circulation 96, 2190–2196. doi: 10.1161/01.cir.96.7.2190
Oppitz, M. (1974). Myths and facts: reconsidering some data concerning the clan history of the Sherpas. Kailash 2, 121–131.
Pagani, L., Ayub, Q., Macarthur, D. G., Xue, Y., Baillie, J. K., Chen, Y., et al. (2012). High altitude adaptation in Daghestani populations from the Caucasus. Hum. Genet. 131, 423–433. doi: 10.1007/s00439-011-1084-8
Patitucci, M., and Lugrin, D. (2009). Angiogenic/lymphangiogenic factors and adaptation to extreme altitudes during an expedition to Mount Everest. Acta Physiol. 196, 259–265. doi: 10.1111/j.1748-1716.2008.01915.x
Peng, Y., Cui, C., He, Y., Zhang, H., Yang, D., Zhang, Q., et al. (2017). Down-regulation of EPAS1 transcription and genetic adaptation of Tibetans to high-altitude hypoxia. Mol. Biol. Evol. 34, 818–830. doi: 10.1093/molbev/msw280
Peng, Y., Yang, Z., Zhang, H., Cui, C., Qi, X., Luo, X., et al. (2011). Genetic variations in Tibetan populations and high-altitude adaptation at the Himalayas. Mol. Biol. Evol. 28, 1075–1081. doi: 10.1093/molbev/msq290
Percy, M. J., Furlow, P. W., Lucas, G. S., Li, X., Lappin, T. R., Mcmullin, M. F., et al. (2008). A gain-of-function mutation in the HIF2A gene in familial erythrocytosis. New Engl. J. Med. 358, 162–168.
Pringle, K., Kind, K., Sferruzzi-Perri, A., Thompson, J., and Roberts, C. (2009). Beyond oxygen: complex regulation and activity of hypoxia inducible factors in pregnancy. Hum. Reprod. update 16, 415–431. doi: 10.1093/humupd/dmp046
Pugh, L., Gill, M., Lahiri, S., Milledge, J., Ward, M., and West, J. (1964). Muscular exercise at great altitudes. J. Appl. Physiol. 19, 431–440. doi: 10.1152/jappl.1964.19.3.431
Pugh, L. G. C. E. (1962). Physiological and medical aspects of the Himalayan scientific and mountaineering expedition. BMJ 2, 621–627. doi: 10.1136/bmj.2.5305.621
Qi, X., Cui, C., Peng, Y., Zhang, X., Yang, Z., Zhong, H., et al. (2013). Genetic evidence of Paleolithic colonization and Neolithic expansion of modern humans on the Tibetan Plateau. Mol. Biol. Evol. 30, 1761–1778. doi: 10.1093/molbev/mst093
Ruggiero, L., Hoiland, R. L., Hansen, A. B., Ainslie, P. N., and Mcneil, C. J. (2018). UBC-Nepal expedition: peripheral fatigue recovers faster in Sherpa than lowlanders at high altitude. J. Physiol. 596, 5365–5377. doi: 10.1113/JP276599
Ruggiero, L., and Mcneil, C. J. (2018). Supraspinal fatigue and neural-evoked responses in Lowlanders and Sherpa at 5050 m. Med. Sci. Sports Exerc. 51, 183–192. doi: 10.1249/MSS.0000000000001748
Samaja, M., Mariani, C., Prestini, A., and Cerretelli, P. (1997). Acid-base balance and O2 transport at high altitude. Acta Physiol. Scand. 159, 249–256. doi: 10.1046/j.1365-201x.1997.574342000.x
Samaja, M., Veicsteinas, A., and Cerretelli, P. (1979). Oxygen affinity of blood in altitude Sherpas. J. Appl. Physiol. 47, 337–341. doi: 10.1152/jappl.1979.47.2.337
Santolaya, R. B., Lahiri, S., Alfaro, R. T., and Schoene, R. B. (1989). Respiratory adaptation in the highest inhabitants and highest Sherpa mountaineers. Respir. Physiol. 77, 253–262. doi: 10.1016/0034-5687(89)90011-x
Scheinfeldt, L. B., Soi, S., Thompson, S., Ranciaro, A., Woldemeskel, D., Beggs, W., et al. (2012). Genetic adaptation to high altitude in the Ethiopian highlands. Genome Biol. 13:R1. doi: 10.1186/gb-2012-13-1-r1
Scheinfeldt, L. B., and Tishkoff, S. A. (2013). Recent human adaptation: genomic approaches, interpretation and insights. Nat. Rev. Genet. 14, 692–702. doi: 10.1038/nrg3604
Schneider, A., Greene, R. E., Keyl, C., Bandinelli, G., Passino, C., Spadacini, G., et al. (2001). Peripheral arterial vascular function at altitude: sea-level natives versus Himalayan high-altitude natives. J. Hypertens. 19, 213–222. doi: 10.1097/00004872-200102000-00007
Schoene, R., Lahiri, S., Hackett, P., Peters, R., Milledge, J., Pizzo, C., et al. (1984). Relationship of hypoxic ventilatory response to exercise performance on Mount Everest. J. Appl. Physiol. 56, 1478–1483. doi: 10.1152/jappl.1984.56.6.1478
Simonson, T. S. (2015). Altitude adaptation: a glimpse through various lenses. High Alt. Med. Biol. 16, 125–137. doi: 10.1089/ham.2015.0033
Simonson, T. S., Wei, G., Wagner, H. E., Wuren, T., Qin, G., Yan, M., et al. (2015). Low haemoglobin concentration in Tibetan males is associated with greater high-altitude exercise capacity. J. Physiol. 593, 3207–3218. doi: 10.1113/JP270518
Simonson, T. S., Yang, Y., Huff, C. D., Yun, H., Qin, G., Witherspoon, D. J., et al. (2010). Genetic evidence for high-altitude adaptation in Tibet. Science 329, 72–75. doi: 10.1126/science.1189406
Simpson, L. L., Busch, S. A., Oliver, S. J., Ainslie, P. N., Stembridge, M., Steinback, C. D., et al. (2019). Baroreflex control of sympathetic vasomotor activity and resting arterial pressure at high altitude: insight from Lowlanders and Sherpa. J. Physiol. 597, 2379–2390. doi: 10.1113/JP277663
Smith, C. (1997). The effect of maternal nutritional variables on birthweight outcomes of infants born to Sherpa women at low and high altitudes in Nepal. Am. J. Hum. Biol. 9, 751–763. doi: 10.1002/(SICI)1520-630019979:6
Song, D., Li, L.-S., Arsenault, P. R., Tan, Q., Bigham, A. W., Heaton-Johnson, K. J., et al. (2014). Defective Tibetan PHD2 binding to p23 links high altitude adaptation to altered oxygen sensing. J. Biol. Chem. 289, 14656–14665. doi: 10.1074/jbc.M113.541227
Stembridge, M., Ainslie, P. N., Boulet, L. M., Anholm, J., Subedi, P., Tymko, M. M., et al. (2019). The independent effects of hypovolaemia and pulmonary vasoconstriction on ventricular function and exercise capacity during acclimatisation to 3800 m. J. Physiol. 597, 1059–1072. doi: 10.1113/JP275278
Stembridge, M., Ainslie, P. N., Hughes, M. G., Stöhr, E. J., Cotter, J. D., Nio, A. Q., et al. (2014). Ventricular structure, function, and mechanics at high altitude: chronic remodeling in Sherpa vs. short-term lowlander adaptation. J. Appl. Physiol. 117, 334–343. doi: 10.1152/japplphysiol.00233.2014
Stembridge, M., Ainslie, P. N., and Shave, R. (2015). Short-term adaptation and chronic cardiac remodelling to high altitude in lowlander natives and Himalayan Sherpa. Exp. Physiol. 100, 1242–1246. doi: 10.1113/expphysiol.2014.082503
Sutton, J. R., Reeves, J. T., Wagner, P. D., Groves, B. M., Cymerman, A., Malconian, M. K., et al. (1988). Operation Everest II: oxygen transport during exercise at extreme simulated altitude. J. Appl. Physiol. 64, 1309–1321. doi: 10.1152/jappl.1988.64.4.1309
Suzuki, K., Kizaki, T., Hitomi, Y., Nukita, M., Kimoto, K., Miyazawa, N., et al. (2003). Genetic variation in hypoxia-inducible factor 1α and its possible association with high altitude adaptation in Sherpas. Med. Hypotheses 61, 385–389. doi: 10.1016/s0306-9877(03)00178-6
Takeda, K., Aguila, H. L., Parikh, N. S., Li, X., Lamothe, K., Duan, L.-J., et al. (2008). Regulation of adult erythropoiesis by prolyl hydroxylase domain proteins. Blood 111, 3229–3235. doi: 10.1182/blood-2007-09-114561
Takeda, K., Cowan, A., and Fong, G.-H. (2007). Essential role for prolyl hydroxylase domain protein 2 in oxygen homeostasis of the adult vascular system. Circulation 116, 774–781. doi: 10.1161/circulationaha.107.701516
Takeda, K., Ho, V. C., Takeda, H., Duan, L.-J., Nagy, A., and Fong, G.-H. (2006). Placental but not heart defects are associated with elevated hypoxia-inducible factor α levels in mice lacking prolyl hydroxylase domain protein 2. Mol. Cell. Biol. 26, 8336–8346. doi: 10.1128/mcb.00425-06
Tan, Q., Kerestes, H., Percy, M. J., Pietrofesa, R., Chen, L., Khurana, T. S., et al. (2013). Erythrocytosis and pulmonary hypertension in a mouse model of human HIF2A gain-of-function mutation. J. Biol. Chem. 289, 14656–14665. doi: 10.1074/jbc.M112.444059
Tannheimer, M., Van Der Spek, R., Brenner, F., Lechner, R., Steinacker, J. M., and Treff, G. (2017). Oxygen saturation increases over the course of the night in mountaineers at high altitude (3050-6354 m). J. Travel Med. 24:tax041. doi: 10.1093/jtm/tax041
Tremblay, J. C., Hoiland, R. L., Carter, H. H., Howe, C. A., Stembridge, M., Willie, C. K., et al. (2018). UBC-nepal expedition: upper and lower limb conduit artery shear stress and flow-mediated dilation on ascent to 5050 m in Lowlanders and Sherpa. Am. J. Physiol. Heart Circ. Physiol. 315, H1532–H1543. doi: 10.1152/ajpheart.00345.2018
Tremblay, J. C., Thom, S. R., Yang, M., and Ainslie, P. N. (2017). Oscillatory shear stress, flow-mediated dilatation, and circulating microparticles at sea level and high altitude. Atherosclerosis 256, 115–122. doi: 10.1016/j.atherosclerosis.2016.12.004
Tymko, M. M., Tremblay, J. C., Hansen, A. B., Howe, C. A., Willie, C. K., Stembridge, M., et al. (2017a). The effect of alpha1 -adrenergic blockade on post-exercise brachial artery flow-mediated dilatation at sea level and high altitude. J. Physiol. 595, 1671–1686. doi: 10.1113/JP273183
Tymko, M. M., Tremblay, J. C., Steinback, C. D., Moore, J. P., Hansen, A. B., Patrician, A., et al. (2017b). UBC-Nepal Expedition: acute alterations in sympathetic nervous activity do not influence brachial artery endothelial function at sea level and high altitude. J. Appl. Physiol. 123, 1386–1396. doi: 10.1152/japplphysiol.00583.2017
Wang, B., Zhang, Y.-B., Zhang, F., Lin, H., Wang, X., Wan, N., et al. (2011). On the origin of Tibetans and their genetic basis in adapting high-altitude environments. PLoS One 6:e17002. doi: 10.1371/journal.pone.0017002
Weitz, C. A., and Garruto, R. M. (2007). A comparative analysis of arterial oxygen saturation among Tibetans and Han born and raised at high altitude. High Alt. Med. Biol. 8, 13–26. doi: 10.1089/ham.2006.1043
Willie, C. K., Stembridge, M., Hoiland, R. L., Tymko, M. M., Tremblay, J. C., Patrician, A., et al. (2018). UBC-nepal expedition: an experimental overview of the 2016 University of British Columbia scientific expedition to Nepal Himalaya. PLoS One 13:e0204660. doi: 10.1371/journal.pone.0204660
Wilson, M. H., Edsell, M. E., Davagnanam, I., Hirani, S. P., Martin, D. S., Levett, D. Z., et al. (2011). Cerebral artery dilatation maintains cerebral oxygenation at extreme altitude and in acute hypoxia—an ultrasound and MRI study. J. Cereb. Blood Flow Metab. 31, 2019–2029. doi: 10.1038/jcbfm.2011.81
Winslow, R. M., Chapman, K. W., Gibson, C. C., Samaja, M., Monge, C. C., Goldwasser, E., et al. (1989). Different hematologic responses to hypoxia in Sherpas and Quechua Indians. J. Appl. Physiol. 66, 1561–1569. doi: 10.1152/jappl.1989.66.4.1561
Wu, T. (1990). Changes in cardiac function at rest and during exercise in mountaineers at an extreme altitude. Zhonghua Yi Xue Za Zhi 70, 72–76.
Wu, T., and Kayser, B. (2006). High altitude adaptation in Tibetans. High Alt. Med. Biol. 7, 193–208. doi: 10.1089/ham.2006.7.193
Wu, T., Liu, F., Cui, C., Qi, X., and Su, B. (2013). A genetic adaptive pattern-low hemoglobin concentration in the Himalayan highlanders. Chin. J. Appl. Physiol. 29, 481–493.
Xiang, K., Ouzhuluobu, Peng, Y., Yang, Z., Zhang, X., Cui, C., et al. (2013). Identification of a Tibetan-specific mutation in the hypoxic gene EGLN1 and its contribution to high-altitude adaptation. Mol. Biol. Evol. 30, 1889–1898. doi: 10.1093/molbev/mst090
Xing, J., Wuren, T., Simonson, T. S., Watkins, W. S., Witherspoon, D. J., Wu, W., et al. (2013). Genomic analysis of natural selection and phenotypic variation in high-altitude Mongolians. PLoS Genetics 9:e1003634. doi: 10.1371/journal.pgen.1003634
Xu, S., Li, S., Yang, Y., Tan, J., Lou, H., Jin, W., et al. (2011). A genome-wide search for signals of high-altitude adaptation in Tibetans. Mol. Biol. Evol. 28, 1003–1011. doi: 10.1093/molbev/msq277
Yi, X., Liang, Y., Huerta-Sanchez, E., Jin, X., Cuo, Z. X. P., Pool, J. E., et al. (2010). Sequencing of 50 human exomes reveals adaptation to high altitude. Science 329, 75–78. doi: 10.1126/science.1190371
Zhang, C., Lu, Y., Feng, Q., Wang, X., Lou, H., Liu, J., et al. (2017). Differentiated demographic histories and local adaptations between Sherpas and Tibetans. Genome Biol. 18, 115. doi: 10.1186/s13059-017-1242-y
Zhang, X., Ha, B., Wang, S., Chen, Z., Ge, J., Long, H., et al. (2018). The earliest human occupation of the high-altitude Tibetan Plateau 40 thousand to 30 thousand years ago. Science 362, 1049–1051. doi: 10.1126/science.aat8824
Keywords: Sherpa, Tibetan, Sherpa physiology, hypoxia adaptation, genetic selection, high altitude adaptation, natural selection
Citation: Bhandari S and Cavalleri GL (2019) Population History and Altitude-Related Adaptation in the Sherpa. Front. Physiol. 10:1116. doi: 10.3389/fphys.2019.01116
Received: 30 January 2019; Accepted: 12 August 2019;
Published: 28 August 2019.
Edited by:
Tatum S. Simonson, University of California, San Diego, United StatesReviewed by:
Cynthia M. Beall, Case Western Reserve University, United StatesEdward Gilbert-Kawai, University College London, United Kingdom
Lorna Grindlay Moore, University of Colorado Denver, United States
Copyright © 2019 Bhandari and Cavalleri. This is an open-access article distributed under the terms of the Creative Commons Attribution License (CC BY). The use, distribution or reproduction in other forums is permitted, provided the original author(s) and the copyright owner(s) are credited and that the original publication in this journal is cited, in accordance with accepted academic practice. No use, distribution or reproduction is permitted which does not comply with these terms.
*Correspondence: Sushil Bhandari, sushilbhandari@rcsi.ie; Gianpiero L. Cavalleri, gcavalleri@rcsi.ie