- 1Department of Diabetes, Endocrinology and Nutrition, Girona Biomedical Research Institute (IdIBGi), CIBEROBN (CB06/03/010) and Carlos III Health Institute (ISCIII), Girona, Spain
- 2Department of Medicine, University of Girona, Girona, Spain
Background and objective: Aging is a physiological process known to produce changes in body composition, affecting the musculature and leading to decreased muscle strength. Muscle in response to exercise acts as an endocrine organ, producing and releasing myokines such as irisin and myostatin that modulate muscular growth. Here, we aimed to evaluate the effects of low intensity resistance exercise, with or without protein supplementation, on body composition, anthropometric parameters and circulating irisin and myostatin in elderly subjects.
Methods: This is a prospective and controlled clinical trial in which subjects were randomized into 3 groups: (1) control group (n = 20), (2) low intensity resistance exercise group (RE) (n = 14), and (3) low intensity resistance exercise and nutritional support group (RENS) (n = 9). Participants, aged 60–75 years, were studied at baseline and 16 weeks thereafter. Body composition was evaluated through bioelectric impedance. Serum irisin and myostatin was measured using ELISA.
Results: At follow-up, RENS resulted in a significant increase in fat free mass (47.4 ± 7.4 vs. 46.5 ± 7.4, p = 0.046), the calf muscle circumference (36.4 ± 1.3 vs. 32.3 ± 4.3, p = 0.025), and circulating irisin (3 ± 1.1 vs. 2.6 ± 1.3, p = 0.030) compared to baseline. RE resulted in a significant increase in grip strength (17.2 ± 4.6 vs. 15.3 ± 4.6, p = 0.011) and irisin (3.1 ± 0.8 vs. 2.4 ± 0.3, p = 0.011) and decreased walking speed at different distance (p < 0.02). Opposite findings in these parameters were observed in control intervention. In line with these findings, the percent change of calf muscle circumference (p = 0.003) and fat free mass (p < 0.0001) were significantly increased in RENS compared to control, whereas fat mass (p = 0.033) was decreased. Interestingly, in this group, strength was positively correlated with fat free mass (r = 0.782, p = 0.008), and circulating irisin was significantly decreased in those participants with strength loss at the end of the study (p = 0.002). No significant correlation between circulating irisin and myostatin in any group was observed.
Conclusion: Circulating irisin, but not myostatin, constitutes a marker for improved muscular performance in elderly subjects.
Introduction
Aging of population is rapidly accelerating, with dramatic increase of people older than 65 years and a change in the age structure of worldwide population (Christensen et al., 2009). Aging is a physiologic process characterized by a gradual impairment in many body functions and growing risk of disease (Holloszy, 2000), with a decrease in strength, flexibility, aerobic capacity, and force output due to progressive loss of muscle tissue and free fat mass whereas fat mass tend to increase (Vinciguerra et al., 2010).
Evidence from human studies supports the notion that regular, vigorous aerobic exercise is a useful tool, with a dose-response effect, to improve the overall health status and longevity (Teramoto and Bungum, 2010; Ruiz et al., 2011). Exercise stimulates the release of cytokines with autocrine, paracrine and endocrine functions produced in skeletal muscle, termed myokines. Thus, skeletal muscle can be classified as endocrine organ. Irisin and myostatin are myokines involved in adaptations to regular training such as increased muscle mass or muscle hypertrophy. Irisin seems a positive regulator whereas myostatin inhibits muscle growth (Kalinkovichs and Livshi, 2015).
Irisin is the result of proteolytic cleavage product of the fibronectin type III “domain-containing” protein 5 (FDNC5) and acts on skeletal muscle, resulting in increased energy expenditure and oxidative metabolism through the induction of metabolic genes (Li et al., 2017). Previous reports demonstrated anti-diabetic effect of irisin through increased energy expenditure in mice (Boström et al., 2012). Moreover, irisin plays a key role on the skeleton by increasing cortical bone mineral density, modifying its structure and improving bone strength (Colaianni et al., 2015). In humans, contradictory effects of exercise in irisin levels have been reported (Moreno-Navarrete et al., 2013; Lopez-Legarrea et al., 2014; Shi et al., 2016). While higher irisin plasma levels after exercise in humans have been described, Timmons et al. (2012) using gene expression arrays, failed to detect a robust and consistent increase in FNDC5 mRNA in human muscle biopsies after exercise. The threshold of exercise required to produce these effects might be crucial, because acute exercise has been demonstrated to result in increased serum irisin concentrations in humans (Huh et al., 2012; Anastasilakis et al., 2014). Lower circulating irisin is a marker of muscle weakness and atrophy and is associated with total muscle mass (Huh et al., 2012; Kim et al., 2015; Chang et al., 2017). Taken together, these data suggest that resistance exercise may result in increased circulating irisin.
Myostatin, also known as growth differentiation factor 8, is a secreted TGF-β superfamily protein that is expressed in skeletal muscle, controlling myoblast proliferation, but being a potent negative regulator of skeletal muscle growth and development (Lee, 2004). Myostatin is synthesized as a 376 amino acid (aa) preprotein that consists of 24 aa signal peptide, a 243 aa propeptide, and a 109 aa mature protein (McPherron et al., 1997). The secreted proprotein is cleaved by BMP-1 family proteases to separate the propeptide from the bioactive mature protein (Lee and McPherron, 2001; Zimmers et al., 2002; Wolfaman et al., 2003). This cleavage results in a latent complex containing a disulfide-linked dimer of the mature protein and two associated propeptides (Lee and McPherron, 2001; Zimmers et al., 2002), in which the myostatin propeptide inhibits the active form.
In the current study, we aimed to investigate the association between resistance exercise and circulating levels of irisin and myostatin in elderly subjects (more than 60 years).
Materials and Methods
Subjects’ Recruitment
This is a prospective and controlled clinical trial in which subjects were randomized into 3 groups: (1) control group (n = 15), (2) resistance exercise group (RE) (n = 14), and (3) resistance exercise nutritional support group (RENS) (n = 9) during 16 weeks. To increase the sample size of control group, five additional patients were recruited. Randomization was done with the EPIDAT 4.0 program. The subjects (men and women aged 60–75 years) residing in province of Girona, Spain; users of civic centers and primary health care center were invited to participate. Those who accepted were enrolled after giving written informed consent, being studied at baseline and after 16 weeks. This study excluded subjects who (i) were known to have associated chronic diseases (i.e., type 2 diabetes poorly controlled); (ii) were under treatment that could cause myopathy; and (iii) suffer from dementia, cognitive limitation, respiratory impairment or malnutrition (IMC <17 kg/m2, involuntary weight loss >10% and albumin levels <2.5 g/dl).
The night before the visit, all participants followed their usual diet. The post-intervention visit was performed a week after the last session of exercise. For each patient the following measurements at baseline and at post-intervention visit were carried out: (i) Analysis of the biochemical parameters at fasting state; (ii) Anthropometric measurements: brachial circumference, arm circumference, calf circumference, abdominal circumference, tricipital fold; (iii) Fragility test (Fried et al., 2001); (iv) World Health Organization Quality of Life – BREF (WHOQOL-BREF); (v) Mini nutrition assessment (MNA); (vi) Evaluation of muscle strength with a dynamometer; (vii) Assessment of the physical condition with the Short Physical Performance Battery (SPPB).
Exercise Program
The exercise program lasted 16 weeks and followed the basic principles of the training: (1) loading principle, (2) progression, (3) specificity and individuality, and (4) recovery [American college of sport medicine position stand (1998)]. The subjects performed two weekly sessions of approximately 45 min, with a minimum day of rest between sessions. The session consisted of 3 parts: (i) Warm up with stretching and joint mobilization exercise; (ii) Main part where the resistance exercises will be worked out: 4 upper body exercises and 3 lower body exercises; (iii) Return to the calm with stretching and relaxation. The first weeks of the program were for the anatomical adaptation and the knowledge for the exercises execution; later and progressively increased the load and the volume. All subjects were directly supervised by the physical educator who was in charge.
Nutritional Intervention
The individuals in the control group (C) and the resistance exercise group (RE) followed their usual diet. Individuals in the resistance exercise and nutritional support group (RENS) followed a balanced and varied diet. A protein intake of 0.8–1 g of protein per kg of weight was guaranteed, distributed in 3 meals per day (breakfast/lunch/dinner). The protein intake was calculated from three 24-h reminders as previously reported (Wu et al., 2017). Dietary advice was given to follow a diet enriched with proteins and also was provided with powdered protein (calcium caseinate, T. Aliment – Espècies Teixidor, Barcelona, Spain).
Nutritional Support
The 10 g of protein (calcium caseinate) powder diluted in water or juice was taken daily at breakfast and twice week additional 10 g of protein administration just after exercise performance.
Analytical Methods
Serum glucose, glycated hemoglobin (HbA1c), serum insulin, total cholesterol, LDL and HDL cholesterol, triglycerides, were determined as described elsewhere (Moreno-Navarrete et al., 2013). C-reactive protein (ultrasensitive assay; 110 Beckman, Fullerton, CA, United States) was determined by a routine laboratory test. Leucocytes, neutrophils and lymphocytes cell count and albumin and creatinine measurement was performed by routine laboratory analysis. Irisin were determined using Irisin ELISA Kit (Catalog Number RAG018R, BIOVENDOR, Brno, Czechia). According to manufacturer information, this ELISA is specific for the measurement of natural and recombinant irisin in human samples, and it does not cross-react with FNDC4, human adiponectin, human Nampt, human RBP4, human clusterin, human leptin, human vaspin, human GPX3, human resistin, human ACE2, human lipocalin-2, human ANGPTL3, human ANGPTL6, human DNER, human DLK1, human calreticulin, and human IL-33. Myostatin concentrations were measured by Quantikine®ELISA GDF-8 / Myostatin Immunoassay (Catalog Number DGDF80, R&D Systems, Inc., Minneapolis, MN, United States). In both irisin and myostatin ELISA, intra- and interassay coefficients of variation were lower than 10%. Irisin was analyzed in all participants from RE and RENS group, but only in first recruited control (n = 15) subjects. Otherwise myostatin, which was measured several months after the end of the study, was analyzed in all participants.
Statistical Analyses
Statistical analyses were performed using SPSS 12.0 software. Unless otherwise stated, descriptive results of continuous variables are expressed as mean and SD for Gaussian variables or median and interquartile range. The relation between variables was analyzed by simple correlation (Spearman’s test). One factor ANOVA with post hoc Bonferroni test and paired t-test were used to compare categorical parameters. Levels of statistical significance were set at p < 0.05.
Results
The current study included 20 participants (18 women and 2 men) in C, 14 participants (12 women and 2 men) in RE and 9 participants (only women) in RENS, with an age range of 60–75 years-old (Table 1). Mean age was significantly higher in RENS compared to RE group (71.2 ± 3.3 vs. 64.9 ± 5.5, p = 0.02) (Table 1).
Changes in body composition, anthropometric and physical condition parameters at baseline and at the end of study in each group were shown in Table 1. At baseline, no significant differences were found between control and intervention groups (Table 1).
Compared to C, RENS resulted in increased percent change of fat free mass kg and calf circumference and in decreased percent change of fat mass kg (Figure 1A). RE also resulted in increased percent change of fat free mass kg compared to C (p = 0.027) (Figure 1A).
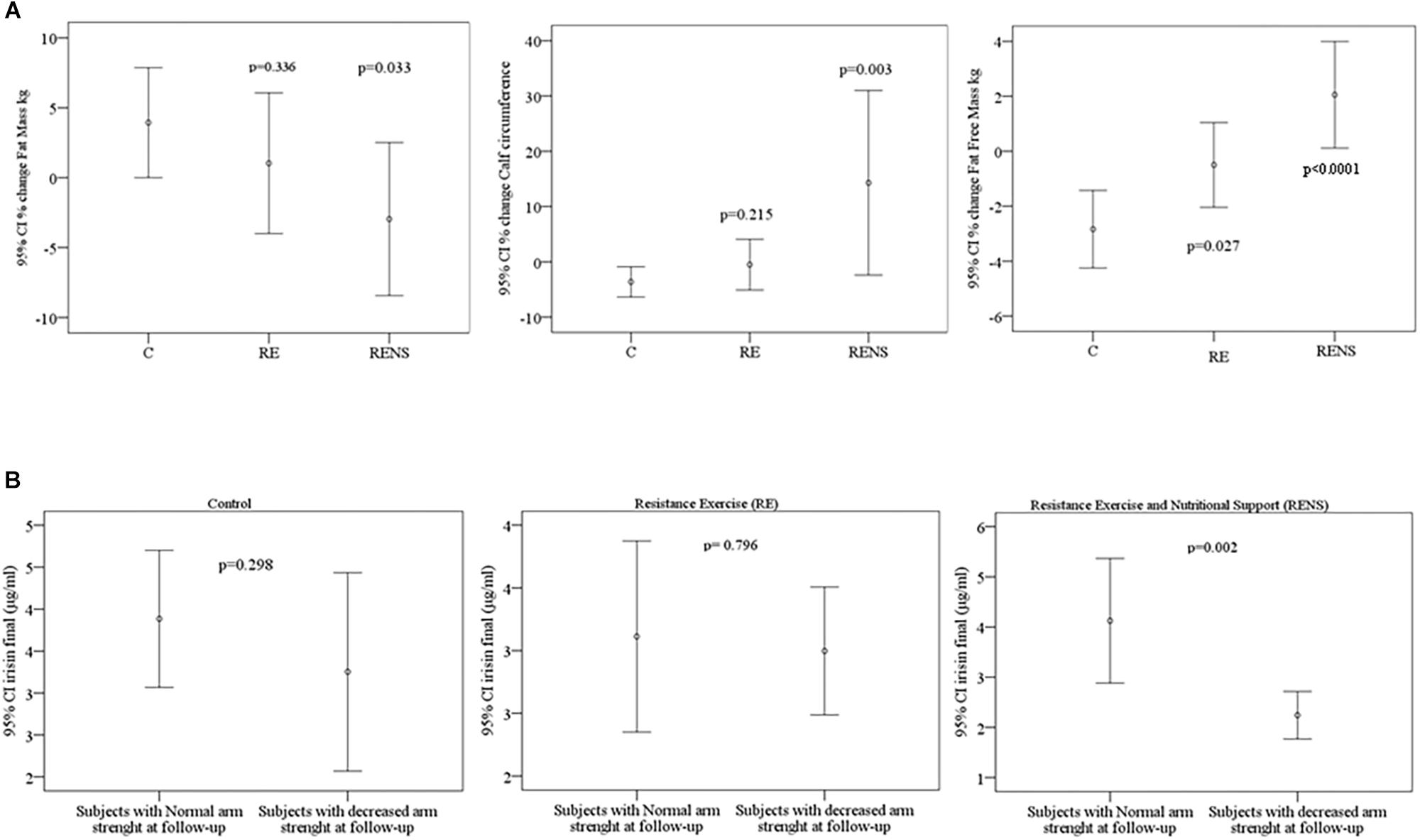
Figure 1. (A) Comparison of percent change of fat free mass, calf circumference and fat mass according to control (C), resistance exercise (RE), and resistance exercise plus nutritional support (RENS). (B) Comparison of circulating irisin according to arm strength in C, RE, and RENS groups.
In C group, a significant decrease in waist and calf circumference, fat free mass, HbA1c, serum myostatin and speed at 3 m, but in the context of increased fat mass was found (Tables 1, 2). In RE group, a significant increase in strength and circulating irisin, and a decrease in speed at 2.44, 4.5, and 3 m was found (Tables 1, 2). In RENS group, a significant increase in muscle arm circumference, calf circumference, fat free mass and circulating irisin was observed (Tables 1, 2). Interestingly, in this group, strength was positively correlated with fat free mass [(r = 0.782, p = 0.008), Table 3].
Since mean age in RENS was higher than in RE, multivariate regression analysis was performed after adjusting for age. This analysis indicated that fat-free mass (β = 0.850, p = 0.004) contributed independently to strength variance at follow up after controlling for age in RENS group.
The study showed a percentage of change in force of 2.9% in C group, of 16% in RE and 4% in RENS (p = 0.181). In RE group, the gain of strength expressed in kg significantly increased from an at baseline strength (from 15.3 to 17.2 kg, p = 0.011), and in RENS group also tended to increase (from 16.8 to 17.8 kg, p = 0.185). Moreover, in RENS group, the force was positively correlated with kg of fat-free mass (p = 0.008), kg of muscle mass (p = 0.008), kg of bone (p = 0.008) and kg protein mass (p = 0.009) (Tables 1, 3).
Of note, in RENS group, circulating irisin was significantly decreased in those participants with decreased arm strength at the end of the study (Figure 1B).
No significant correlation between circulating irisin and myostatin in any group was observed (Table 4).
At baseline, no significant correlation between circulating irisin and anthropometrical and clinical parameters were observed. Circulating myostatin was positively correlated with weight, muscle arm circumference, basal metabolism, fat-free mass, total water and negatively correlated with C-reactive protein (Table 5). In control group, circulating myostatin was positively correlated with speed at 2.44 m (Figure 2).
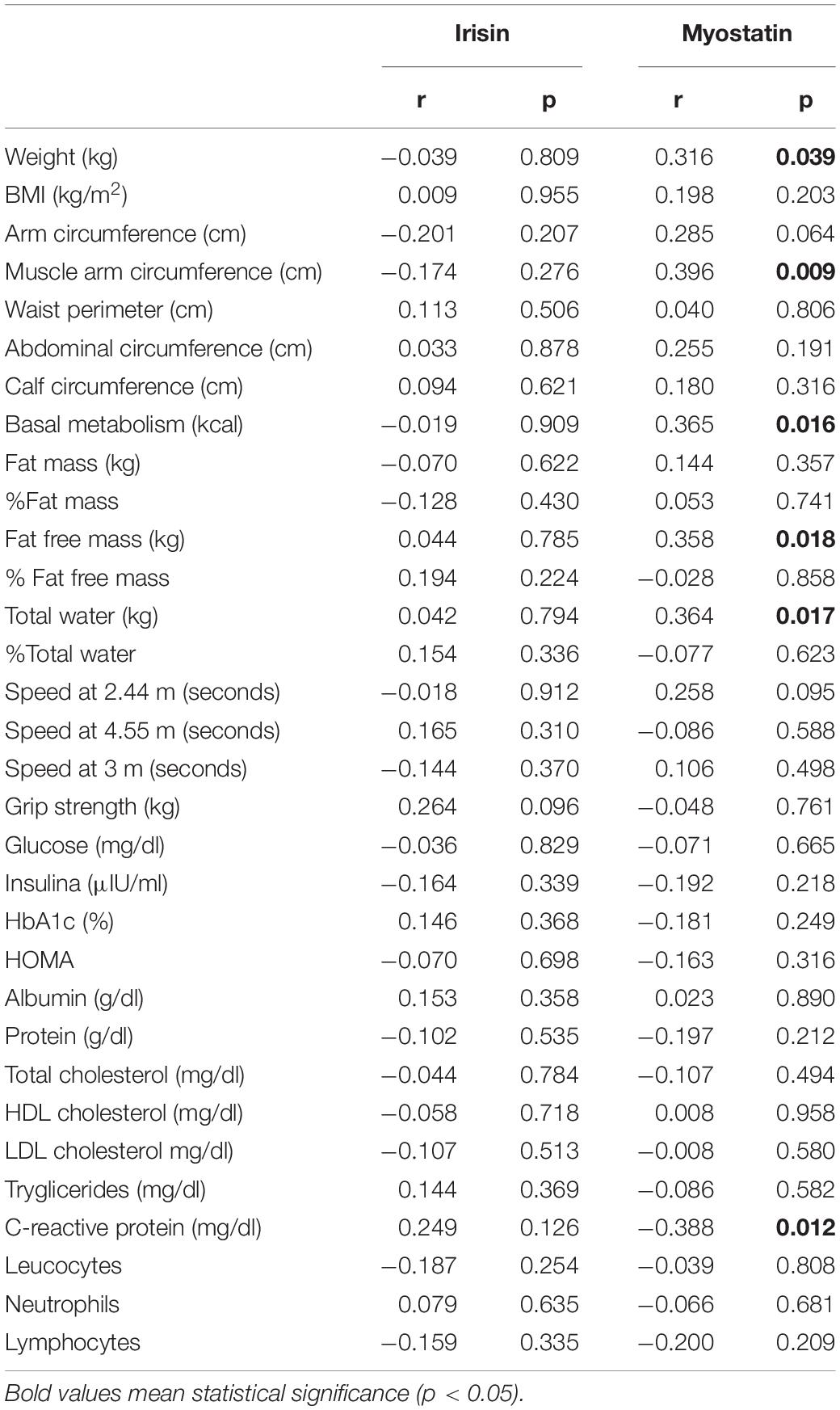
Table 5. Bivariate correlations between anthropometrical and clinical parameters and circulating irisin and myostatin in all participants at baseline.
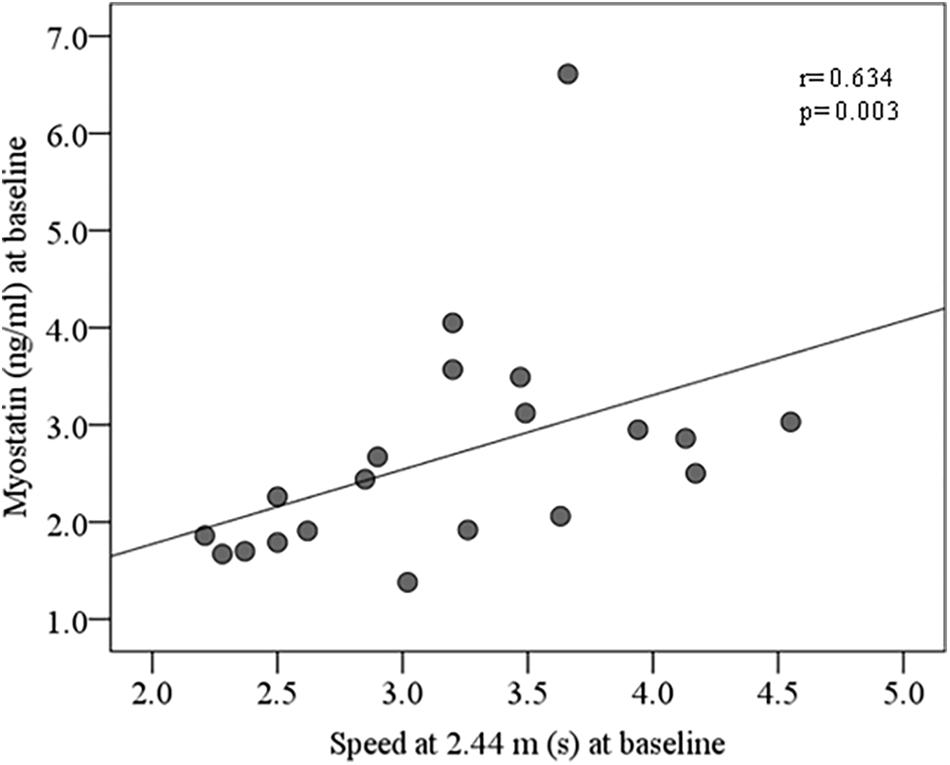
Figure 2. Bivariate correlation between circulating myostatin and speed at 2.44 m at baseline in control group.
At follow up, no significant associations among irisin, anthropometrical and clinical parameters in control (Table 6), RE (Table 7) or RENS (Table 8) were found. In control group, circulating myostatin was positively correlated BMI, abdominal circumference, total water, HOMA, triglycerides, C-reactive protein (Table 6), but these associations were not observed in RE (Table 7) or RENS groups (Table 8).
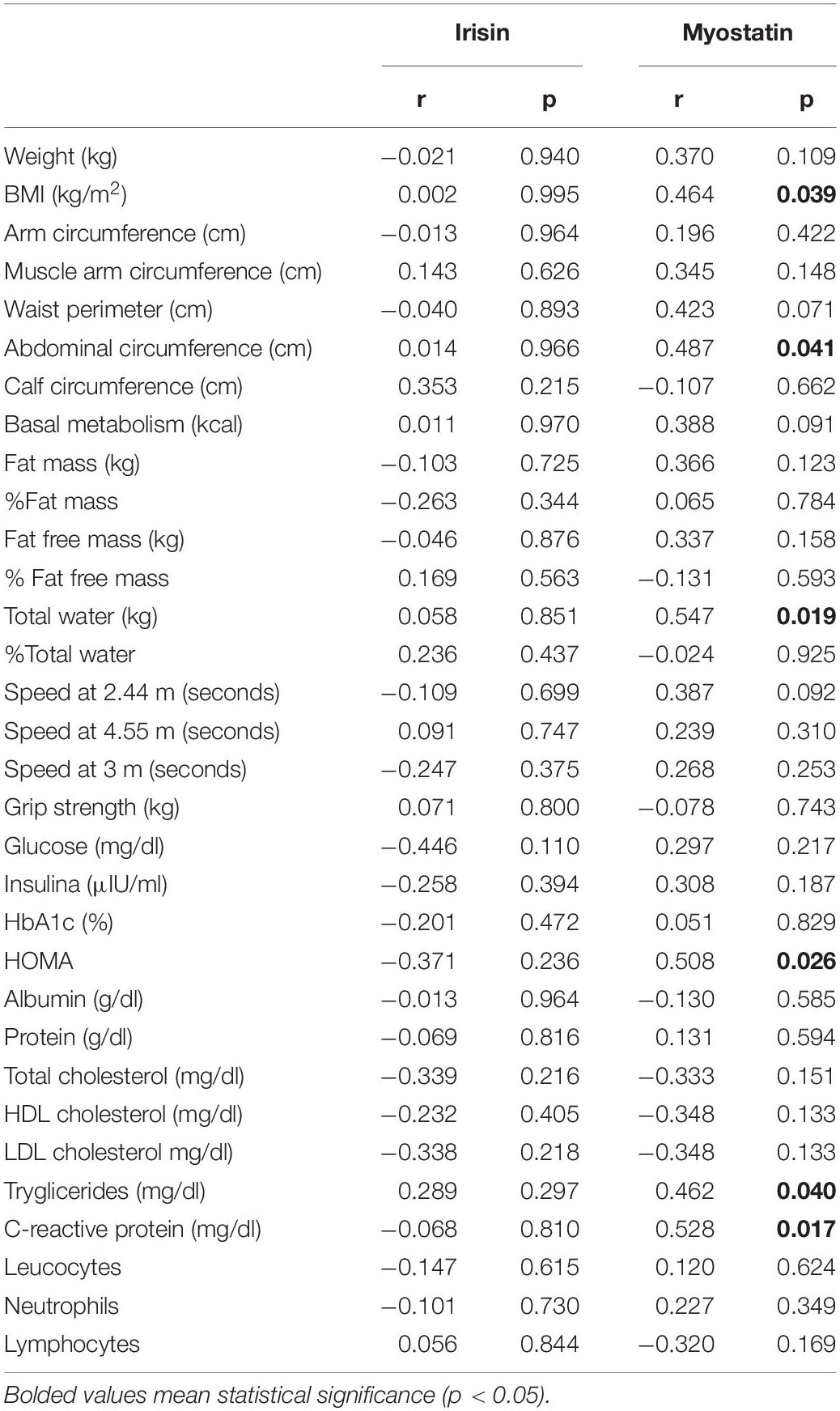
Table 6. Bivariate correlations between anthropometrical and clinical parameters and circulating irisin and myostatin in control group at follow-up.
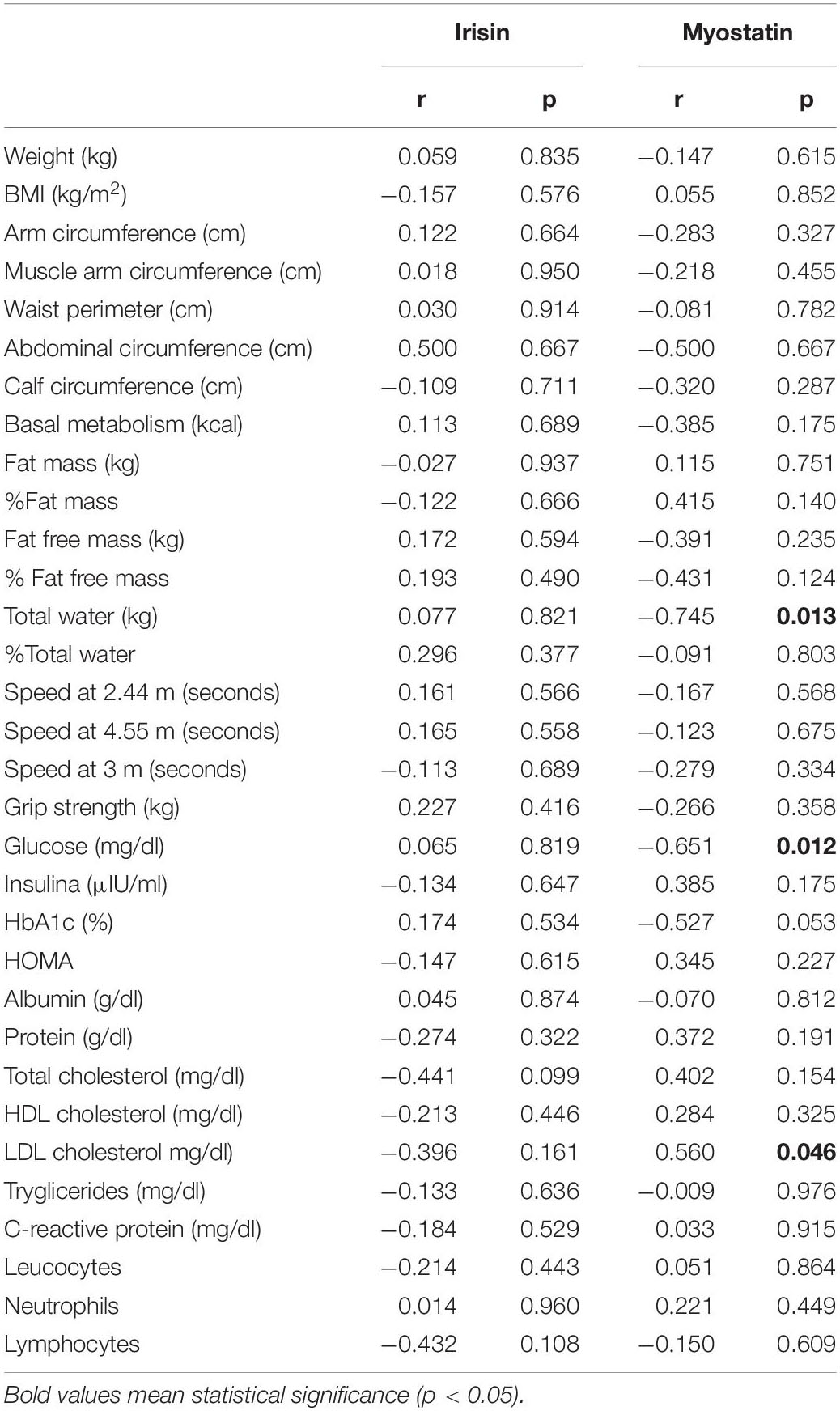
Table 7. Bivariate correlations between anthropometrical and clinical parameters and circulating irisin and myostatin in resistance exercise group at follow-up.
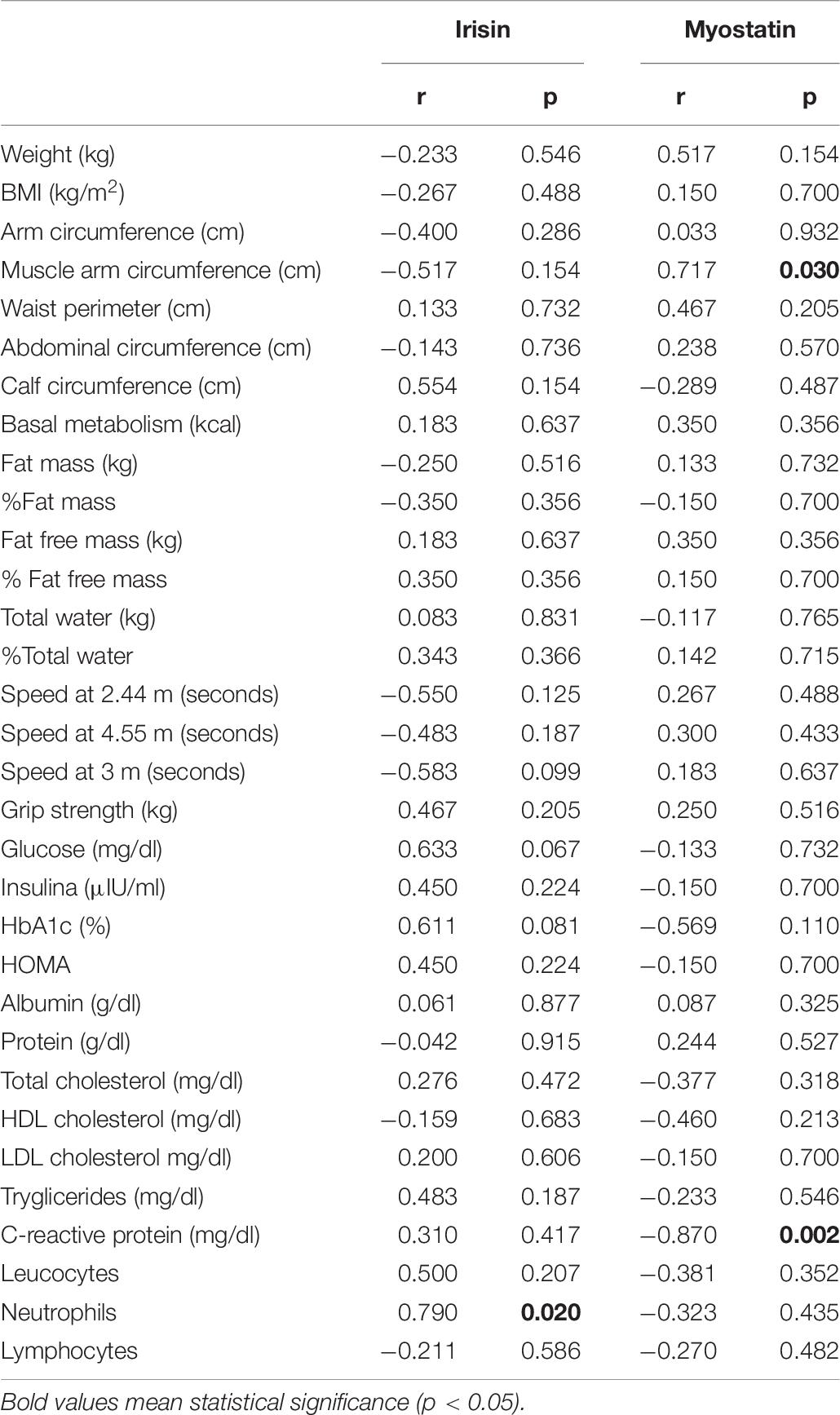
Table 8. Bivariate correlations between anthropometrical and clinical parameters and circulating irisin and myostatin in resistance exercise and nutritional support group at follow-up.
The walking speed decreased significantly in C and RE group, moving from 3.75 to 3.4 m/s at the end (p = 0.016) in C and from 3.9 m/s to a at follow-up speed of 3.2 m/s (p = 0.003) in RE, but not in RENS, in which walking speed change from 3.9 m/s to a at follow-up speed of 3.8 m/s (p = 0.791) (Table 2).
Discussion
In this clinical trial, the impact of low-intensity resistance exercise and nutritional assistance program on body composition, anthropometric parameters, strength, speed of motion and myokines were examined in elderly people.
Body Composition and Anthropometric Parameters
Current data confirmed that low intensity exercising program and nutritional assistance prevented muscle deterioration in people aged 60–75 years old. Exercise improves muscle mass and contributes to the maintenance of daily life activities (Löllgen et al., 2009). Both aerobic and resistance exercise delay the deterioration of the muscles, but resistance exercise seems the most effective (Visvanathan and Chapman, 2010). Here, we showed that 16-week low intensity resistance program produced significant changes on body composition (fat mass, percent fat mass, fat-free mass, muscle mass kg and the calf circumference). These results were expected and are in agreement with previous studies (Liao et al., 2017), and indicated that low intensity resistance training promotes musculature improvement, is a useful and simple therapeutic approach to prevent sarcopenia, and when was combined with protein supplementation these beneficial effects were enhanced (Liao et al., 2017). However, resistance training presents some limitations (Morgan, 2012; Malafarina et al., 2013; Yu, 2015). For instance, one important limitation was the rapid loss of beneficial effects, such as the gain of muscle mass (in the study the improvement is 0.7% p < 0.001) and adherence to exercise. The study’s approach of performing low-intensity resistance exercises aims to inform to the subject about exercise strategies that can be performed at home, since equipment is not required to be able to perform them, and the importance of training as long as possible without causing muscle overload injuries (Johnston et al., 2008).
In control group, waist and calf circumference were reduced in parallel to fat free mass and decreased speed at 3 m, indicating aging-associated body weight reduction (Dzien et al., 2013), muscle mass loss and worsening of physical condition. Elderly associated physical inactivity is known to accelerate muscle mass loss (Evans, 2010). In fact, physical activity reduced the progression of muscle aging and decreased the strength of muscle contraction (Hughes et al., 2004; Zampieri et al., 2015), attenuating sarcopenia in pre- and post-menopausal women (Walsh et al., 2006) and in older men (Szulc et al., 2005). On the other hand, 10 days of bed rest resulted in a significant decreased lean mass and skeletal muscle loss in healthy older adults, even greater than young individuals after 28 days (Kortebein et al., 2007).
Otherwise, the reduction of HbA1c from 5.8 to 5.7 has not clinical relevance.
Here, we found the following effects of resistance training on strength, speed and myokines:
(i) Strength. Resistance training is the most effective strategy to increase muscle mass and strength (Leenders et al., 2013; Yoshimura et al., 2017). Being the grip strength is a good indicator of low muscle mass (Lauretani et al., 2003), this parameter detects individuals with low muscle mass that will make them more fragile and with greater risk of disability (Ling et al., 2010; Taekema et al., 2010). We observed that there was no significant association between the concentration of myostatin and muscular force, as concluded by Carvalho et al. (2018).
(ii) Speed. It is known that exercise interventions that increase muscle strength are very effective in improving walking speed (Gottlob, 2008). In the present study, the walking speed decreased in all groups producing in improvement of 15% in control group, 14% in Resistance Exercise group, and 7% in Resistance Exercise and Nutritional Support group. Different studies have shown improvements between 3 and 10% (Mendieta et al., 2015), being the results obtained in the current study similar to those reported. The walking speed reflects the health and functionality of the person (Abellan van Kan et al., 2009). This is recommended as a clinical indicator to assess survival. In a meta-analysis published in 2011 in the journal JAMA by Studenski et al. (2011) walking speed within their range of normality behaves as a protective factor against mortality, being the lowest in those individuals with faster walking. A lower driving speed is usually associated with disability, cognitive deterioration, accidental falls, various neurological diseases, cardiopulmonary and orthopedic diseases that contribute to increase mortality rate (Abellan van Kan et al., 2009; Studenski et al., 2011).
(iii) Myokines. Myostatin is a hormone that is mainly expressed in the muscle and inhibits the formation of muscle mass. Myostatin is generated as a precursor protein that requires the proteolytic division to release the N-terminal and the C-terminal propeptide. The active form of myostatin is a disulfide dimer attached to the C-terminal fragment (White and LeBrasseur, 2014).
Myostatin increases with age, although differences in total vs. active form have not been well investigated (Yarasheski et al., 2002). The current study, that analyzed serum myostatin concentrations before and after the resistance exercise program, found results that are contradictory with those reported in the literature. In the control group, circulating myostatin concentration decreased significantly after 16 weeks, in parallel to decreased fat free mass, whereas, in RE and RENS group, myostatin remained unchanged after the exercise, and fat free mass increased. These findings were in hard contrast to previous studies that demonstrated negative effects of myostatin in muscle mass development (McPherron et al., 1997; Lee and McPherron, 2001; Zimmers et al., 2002; Wolfaman et al., 2003; Lee, 2004). Possibly, in control group, a reduction of myostatin causes significant decrease in primary fibers of skeletal muscle, which is associated with increased fatigue susceptibility (Hennebry et al., 2009), indicating physical condition decline. In line with current data, in a recent study low serum myostatin levels have been associated with low skeletal muscle (Peng et al., 2018). Muscular force exercise is known to inhibit local myostatin (Brotto and Abreu, 2012). However, Laksmi et al. (2017) observed increased secretion of myostatin in blood in response to exercise. As, the antibodies used to detect myostatin (current ELISA) did not distinguish between the active or latent form, further research is needed.
Irisin is a myokine produced by the proteolytic cleavage of the membrane-protein, fibronectin type III “domain-containing” protein-5 (FNDC5) (Boström et al., 2012). It is regulated by peroxisome proliferator activated receptor (PPAR) and peroxisome proliferator activated receptor γ co-activator-1-α (PGC1α). It is suggested that irisin may act in some of the beneficial effects of exercise by inducing the uncoupling protein one (UCP-1), that afterward increases the energy expenditure of white adipocytes, process called “browning.” This process is characterized by a switch of white to beige adipose tissue in response to β-adrenergic stimuli (exposure to cold and physical activity) (Novelle et al., 2013). In humans, the effect of exercise on irisin production has provided contradictory findings (Huh et al., 2012; Lecker et al., 2012; Timmons et al., 2012). Huh et al. (2012) correlated the decrease in the circulating irisin concentration with the loss of muscle mass associated to aging. A recent study by Chang et al. (2017) concluded that a low blood concentration of irisin was a sensitive molecular marker for muscle weakness and atrophy. In post-menopausal women, decreased serum irisin concentration is an independent predictor of sarcopenia (Park et al., 2018). In fact, irisin has been proposed as a molecule that combines beneficial effects for treating osteoporosis and muscular atrophy through its effects restoring bone and preventing muscle wasting (Kim et al., 2015; Colaianni et al., 2017).
In agreement with these studies, current findings indicated that RE and RENS resulted in increased circulating irisin in parallel to strength and walking speed in elderly subjects. In fact, decreased circulating irisin was significantly associated with strength loss at the end of the study (Figure 1B). Thus, irisin could be a potential biomarker of muscle dysfunction (Chang et al., 2017).
In relation to protein supplementation, several studies indicated that protein supplementation improved the adaptations of resistance exercise (Burke et al., 2001; Candow et al., 2006; Cribb et al., 2006; Hulmi et al., 2009; Volek et al., 2013; Morton et al., 2017). Cribb et al. (2006) reported a 5 kg increase of fat-free mass after 10 weeks of resistance training combined with 45 g/day ingest of milk protein. Burke et al. (2001), Candow et al. (2006) demonstrated that milk protein supplementation during 6 weeks of resistance training promoted an increase of 2.3–2.5 kg of fat-free mass. Hulmi et al. (2009) also reported that supplementation with milk protein and 10 weeks of resistance exercise resulted in an increase of 2.5 kg fat free mass. Volek et al. (2013) reported that supplementation with milk protein from cow or soy in addition with resistance training for 9 months increased by 3.6 and 2.6 kg of fat-free mass, respectively. Morton et al. (2017) suggest that one of the mechanisms responsible for the enhancement of skeletal mass could be explained for the improvement of the anabolism of skeletal muscle. These positive effects of protein supplementation do not have the same effects as isolated supplementation with amino acids (Bird et al., 2006; Vieillevoye et al., 2010; Aguiar et al., 2017). In line with all these studies, current findings confirmed an improvement in fat-free mass in the exercise group with cow milk protein supplementation. Even though, this improvement was not so marked as that described in the literature, probably due to relative low protein supplementation (10 g of protein every day and 20 g during exercise days) did not reach the threshold to promote anabolism of muscle protein in older people (Traylor et al., 2018). An important consideration is that the attachment to nutritional support was not homogeneous in all participants.
Resistance exercise and nutritional support resulted in improved anthropometric parameters (muscle arm circumference, calf circumference, basal metabolism and fat free mass) compared with RE intervention. The improvement in walking speed and grip strength was not so marked in the RENS group compared to RE intervention. These differences could be due to significant increased mean age in RENS compared to RE subjects.
Conclusion
A program of physical exercise of low intensity resistance, together with a nutritional support, improved the deterioration of the musculature in people aged 60–75. Circulating irisin, but not myostatin, constituted a marker for improved muscle strength after resistance exercise in elderly subjects.
Ethics Statement
The development of the study was carried out in accordance with the Helsinki Declaration of the World Medical Association on the ethical principles for research in human subjects. The current study followed the ethical principles according to the Organic Law 15/99 (LOPD) and the guideline document of good clinical practices. The protocol was approved by the Ethics Committee of the Dr. Josep Trueta University Hospital of Girona on July 30, 2012 under the code 2012099.
Author Contributions
CP-F, WR, and JF-R participated in the study design and analysis of data. FC, MS-M, MM, JMM-N, and OR participated in acquisition of data. CP-F, WR, JMM-N, and JF-R participated in interpretation of data. CP-F, FC, and JF-R wrote and edited the manuscript. JMM-N and WR revised the manuscript critically for important intellectual content. All authors participated in final approval of the version to be published.
Funding
This work was partially supported by research grants PI18/01022 from the Instituto de Salud Carlos III from Spain and the FEDER funds. CIBEROBN Fisiopatología de la Obesidad y Nutrición is an initiative from the Instituto de Salud Carlos III and the Fondo Europeo de Desarrollo Regional (FEDER) from Spain.
Conflict of Interest Statement
The authors declare that the research was conducted in the absence of any commercial or financial relationships that could be construed as a potential conflict of interest.
Acknowledgments
We acknowledge the technical assistance of Emili Loshuertos (IdIBGi) and Oscar Rovira (IdIBGi).
References
Abellan van Kan, G., Rolland, Y., Andrieu, S., Bauer, J., Beauchet, O., Bonnefoy, M., et al. (2009). Gait speed at usual pace as a predictor of adverse outcomes in community-dwelling older people an International Academy on Nutrition and Aging (IANA) task force. J. Nutr. Health Aging 10, 881–889. doi: 10.1007/s12603-009-0246-z
Aguiar, A. F., Grala, A. P., da Silva, R. A., Soares-Caldeira, L. F., Pacagnelli, F. L., Ribeiro, A. S., et al. (2017). Free leucine supplementation during an 8-week resistance training program does not increase muscle mass and strength in untrained young adult subjects. Amino Acids 49, 1255–1262. doi: 10.1007/s00726-017-2427-0
Anastasilakis, A. D., Polyzos, S. A., Saridakis, Z. G., Kynigopoulos, G., Skouvaklidou, E. C., Molyvas, D., et al. (2014). Circulating irisin in healthy, young individuals: day-night rhythm, effects of food intake and exercise, and associations with gender, physical activity, diet, and body composition. J. Clin. Endocrinol. Metab. 99, 3247–3255. doi: 10.1210/jc.2014-1367
Bird, S. P., Tarpenning, K. M., and Marino, F. E. (2006). Independent and combined effects of liquid carbohydrate/essential amino acid ingestion on hormonal and muscular adaptations following resistance training in untrained men. Eur. J. Appl. Physiol. 97, 225–238. doi: 10.1007/s00421-005-0127-z
Boström, P., Wu, J., Jedrychowski, M. P., Korde, A., Ye, L., Lo, J. C., et al. (2012). A PGC1-alpha-dependent myokine that drives brown-fat-like development of white fat and thermogenesis. Nature 481, 463–468. doi: 10.1038/nature10777
Brotto, M., and Abreu, E. L. (2012). Sarcopenia: pharmacology of today and tomorrow. J. Pharmacol. Exp. Ther. 343, 540–546. doi: 10.1124/jpet.112.191759
Burke, D. G., Chilibeck, P. D., Davidson, K. S., Candow, D. G., Farthing, J., and Smith-Palmer, T. (2001). The effect of whey protein supplementation with and without creatine monohydrate combined with resistance training on lean tissue mass and muscle strength. Int. J. Sport Nutr. Exerc. Metab. 11, 349–364. doi: 10.1123/ijsnem.11.3.349
Candow, D. G., Burke, N. C., Smith-Palmer, T., and Burke, D. G. (2006). Effect of whey and soy protein supplementation combined with resistance training in young adults. Int. J. Sport Nutr. Exerc. Metab. 16, 233–244. doi: 10.1123/ijsnem.16.3.233
Carvalho, L. P., Basso-Vanelli, R. P., Di Thommazo-Luporini, L., Mendes, R. G., Oliveira-Junior, M. C., Vieira, R. P., et al. (2018). Myostatin and adipokines: the role of the metabolically unhealthy obese phenotype in muscle function and aerobic capacity in young adults. Cytokine 107, 118–124. doi: 10.1016/j.cyto.2017.12.008
Chang, J. S., Kim, T. H., Nguyen, T. T., Park, K. S., Kim, N., and Kong, I. D. (2017). Circulating irisin levels as a predictiv biomarker for sarcopenia: a cross-sectional community-based study. Geriatr. Gerontol. Int. 11, 2266–2273. doi: 10.1111/ggi.13030
Christensen, K., Doblhammer, G., Rau, R., and Vaupel, J. W. (2009). Ageing populations: the challenges ahead. Lancet 37, 1196–1208. doi: 10.1016/S0140-6736(09)61460-4
Colaianni, G., Cinti, S., Colucci, C., and Grano, M. (2017). Irisin and musculoskeletal health. Ann. N. Y. Acad. Sci. 1402, 5–9. doi: 10.1111/nyas.13345
Colaianni, G., Cuscito, C., Mongelli, T., Pignataro, P., Buccoliero, C., Liu, P., et al. (2015). The myokine irisin increases cortical bone mass. Proc. Natl. Acad. Sci. U.S.A. 112, 12157–12162. doi: 10.1073/pnas.1516622112
Cribb, P. J., Williams, A. D., Carey, M. F., and Hayes, A. (2006). The effect of whey isolate and resistance training on strength, body composition, and plasma glutamine. Int. J. Sport Nutr. Exerc. Metab. 16, 494–509. doi: 10.1123/ijsnem.16.5.494
Dzien, A., Winner, H., Theurl, E., Dzien-Bischinger, C., and Lechleitner, M. (2013). Fat-free mass and fasting glucose values in patients with and without statin therapy assigned to age groups between < 60 and > 75 years. Obes. Facts 6, 9–16. doi: 10.1159/000348573
Evans, W. J. (2010). Skeletal muscle loss: cachexia, sarcopenia, and inactivity. Am. J. Clin. Nutr. 91, 1123S–1127S. doi: 10.3945/ajcn.2010.28608A
Fried, L. P., Tangen, C. M., Walston, J., Newman, A. B., Hirsch, C., Gattdiener, J., et al. (2001). Frailty in older adults: evidence for a phenotype. J. Gerontol. A Biol. Sci. Med. Sci. 56, M146–M156. doi: 10.1093/gerona/56.3.M146
Hennebry, A., Berry, C., Siriett, V., O’Callaghan, P., Chau, L., Watson, T., et al. (2009). Myostatin regulates fiber-type composition of skeletal muscle by regulating MEF2 and MyoD gene expression. Am J Physiol Cell Physiol. 296, C525–C534. doi: 10.1152/ajpcell.00259.2007
Holloszy, J. O. (2000). The biology of aging. Mayo Clin. Proc. 75, 3–8. doi: 10.1080/13685530008500359
Hughes, V. A., Roubenoff, R., Wood, M., Frontera, W. R., Evans, W. J., and Fiatarone Singh, M. A. (2004). Anthropometric assessment of 10-y changes in body composition in the elderly. Am. J. Clin. Nutr. 80, 475–482. doi: 10.1093/ajcn/80.2.475
Huh, J. Y., Panagiotou, G., Mougios, V., Brinkoetter, M., Vamvini, M. T., Schneider, B. E., et al. (2012). FNDC5 and irisin in humans: I. Predictors of circulating concentrations in serum and plasma and II. mRNA expression and circulating concentrations in response to weight loss and exercise. Metabolism 61, 1725–1738. doi: 10.1016/j.metabol.2012.09.002
Hulmi, J. J., Kovanen, V., Selanne, H., Kraemer, W. J., Hakkinen, K., and Mero, A. A. (2009). Acute and long-term effects of resistance exercise with or without protein ingestion on muscle hypertrophy and gene expression. Amino Acids 37, 297–308. doi: 10.1007/s00726-008-0150-6
Johnston, A. P., De Lisio, M., and Parise, G. (2008). Resistance training, sarcopenia, and the mitochondrial theory of aging. Appl. Physiol. Nutr. Metab. 1, 191–199. doi: 10.1139/H07-141
Kalinkovichs, A., and Livshi, G. (2015). Sarcopenia – the search for emerging biomarkers. Ageing Res. Rev. 22, 58–71. doi: 10.1016/j.arr.2015.05.001
Kim, H. J., So, B., Choi, M., Kang, D., and Song, W. (2015). Resistance exercise training increases the expression of irisin concomitant with improvement of muscle function in aging mice and humans. Exp. Gerontol. 70, 11–17. doi: 10.1016/j.exger.2015.07.006
Kortebein, P., Ferrando, A., Lombeida, J., Wolfe, R., and Evans, W. J. (2007). Effect of 10 days of bed rest on skeletal muscle in healthy older adults. JAMA 297, 1769–1774.
Laksmi, P. W., Setiati, S., Tamin, T. Z., Soewondo, P., Rochmah, W., Nafrialdi, N., et al. (2017). Effect of metformin on handgrip strength, gait speed, myostatin serum level, and health- related quality of life: a double blind randomized controlled trial among non-diabetic pre-frail elderly patients. Acta Med. Indones. 49, 118–127.
Lauretani, F., Russo, C. R., Bandinelli, S., Bartali, B., Cavazzini, C., Di Iorio, A., et al. (2003). Age-associated changes in skeletal muscles and their effect on mobility: an operational diagnosis of sarcopenia. J. Appl. Physiol. 95, 1851–1860. doi: 10.1152/japplphysiol.00246.2003
Lecker, S. H., Zavin, A., Cao, P., Arena, R., Allsup, K., Daniels, K. M., et al. (2012). Expression of the irisin precursor FNDC5 in skeletal muscle correlates with aerobic exercise performance in patients with heart failure. Circ. Heart Fail. 5, 812–818. doi: 10.1161/CIRCHEARTFAILURE.112.969543
Lee, S. J. (2004). Regulation of muscle mass by myostatin. Annu. Rev. Cell Dev. Biol. 20, 61–86. doi: 10.1146/annurev.cellbio.20.012103.135836
Lee, S. J., and McPherron, A. C. (2001). Regulation of myostatin activity and muscle growth. Proc. Natl. Acad. Sci. U.S.A. 31, 9306–9311. doi: 10.1073/pnas.151270098
Leenders, M., Verdijk, L. B., van der Hoeven, L., van Kranenburg, J., Nilwik, R., and van Loon, L. J. C. (2013). Elderly men and women benefit equally from prolonged resistance-type exercise training. J. Gerontol. A Biol. Sci. Med. Sci. 68, 769–779. doi: 10.1093/gerona/gls241
Li, F., Li, Y., Duan, Y., Hu, C. A., Tang, Y., and Yin, Y. (2017). Myokines and adipokines: involvement in the crosstalk between skeletal muscle and adipose tissue. Cytokine Growth Factor Rev. 33, 73–82. doi: 10.1016/j.cytogfr.2016.10.003
Liao, C. D., Tsauo, J. Y., Wu, Y. T., Cheng, C. P., Chen, H. C., Huang, Y. C., et al. (2017). Effects of protein supplementation combined with resistance exercise on body composition and physical function in older adults: a systematic review and meta-analysis. Am. J. Clin. Nutr. 106, 1078–1091. doi: 10.3945/ajcn.116.143594
Ling, C. H., Taekema, D., de Craen, A. J., Gussekloo, J., Westendorp, R. G., and Maier, A. B. (2010). Handgrip strength and mortality in the oldest old population: the Leiden 85-plus study. CMAJ 182, 429–435. doi: 10.1503/cmaj.091278
Löllgen, H., Böckenhoff, A., and Knapp, G. (2009). Physical activity and all-cause mortality: an updated meta-analysis with different intensity categories. Int. J. Sports Med. 30, 213–224. doi: 10.1055/s-0028-1128150
Lopez-Legarrea, P., de la Iglesia, R., Crujeiras, A. B., Pardo, M., Casanueva, F. F., Zulet, M. A., et al. (2014). Higher baseline irisin concentrations are associated with greater reductions in glycemia and insulinemia after weight loss in obese subjects. Nutr. Diabetes 4:e11. doi: 10.1038/nutd.2014.7
Malafarina, V., Uriz-Otano, F., Iniesta, R., and Gil-Guerrero, L. (2013). Effectiveness of nutritional supplementation on muscle mass in treatment of sarcopenia in old age: a systematic review. J. Am. Med. Dir. Assoc. 14, 10–17. doi: 10.1016/j.jamda.2012.08.001
McPherron, A. C., Lawler, A. M., and Lee, S. J. (1997). Regulation of skeletal muscle mass in mice by a new TGF-beta superfamily member. Nature 1, 83–90. doi: 10.1038/387083a0
Mendieta, L., Mendieta, R., Marcelo, J., and García, D. (2015). Improving the speed of up to the implementation of a program of muscle strength in elderly sixties. Ciencia y Desarrollo 2, 37–45.
Moreno-Navarrete, J. M., Ortega, F., Serrano, M., Guerra, E., Pardo, G., Tinahones, F., et al. (2013). Irisin is expressed and produced by human muscle and adipose tissue in association with obesity and insulin resistance. J. Clin. Endocrinol. Metab. 98, E769–E778. doi: 10.1210/jc.2012-2749
Morgan, K. T. (2012). Nutrition, resistance training, and sarcopenia: their role in successful aging. Top. Clin. Nutr. 27, 114–123. doi: 10.1097/tin.0b013e318254217f
Morton, R. W., Murphy, K. T., McKellar, S. R., Schoenfeld, B. J., Henselmans, M., Helms, E., et al. (2017). A systematic review, meta-analysis and meta-regression of the effect of protein supplementation on resistance training-induced gains in muscle mass and strength in healthy adults. Br. J. Sports Med. 52, 376–384. doi: 10.1136/bjsports-2017-097608
Novelle, M. G., Contreras, C., Romero-Picó, A., López, M., and Diéguez, C. (2013). Irisin, two years later. Int. J. Endocrinol. 2013:746281. doi: 10.1155/2013/746281
Park, H. S., Kim, H. C., Zhang, D., Yeom, H., and Lim, S. K. (2018). The novel myokine irisin: clinical implications and potential role as a biomarker for sarcopenia in postmenopausal women. Endocrine 64, 341–348. doi: 10.1007/s12020-018-1814-y
Peng, L. N., Lee, W. J., Liu, L. K., Lin, M. H., and Chen, L. K. (2018). Healthy community-living older men differ from women in associations between myostatin levels and skeletal muscle mass. J. Cachexia Sarcopenia Muscle 9, 635–642. doi: 10.1002/jcsm.12302
Ruiz, J. R., Morán, M., Arenas, J., and Lucia, A. (2011). Strenuous endurance exercise improves life expectancy: it’s in our genes. Br. J. Sports Med. 45, 159–161. doi: 10.1136/bjsm.2010.075085
Shi, X., Lin, M., Liu, C., Xiao, F., Liu, Y., Huang, P., et al. (2016). Elevated circulating irisin is associated with lower risk of insulin resistance: association and path analyses of obese Chinese adults. BMC Endocr. Disord. 16:44. doi: 10.1186/s12902-016-0123-9
Studenski, S., Perera, S., Patel, K., Rosano, C., Faulkner, K., Inzitari, M., et al. (2011). Gait speed and survival in older adults. JAMA 305, 50–58. doi: 10.1001/jama.2010.1923
Szulc, P., Beck, T. J., Marchand, F., and Delmas, P. D. (2005). Low skeletal muscle mass is associated with poor structural parameters of bone and impaired balance in elderly men—the MINOS study. J. Bone Miner. Res. 20, 721–729. doi: 10.1359/jbmr.041230
Taekema, D. G., Gussekloo, J., Maier, A. B., Westendorp, R. G., and de Craen, A. J. (2010). Handgrip strength as a predictor of functional, psychological and social health. A prospective population-based study among the oldest old. Age Ageing 39, 331–337. doi: 10.1093/ageing/afq022
Teramoto, M., and Bungum, T. J. (2010). Mortality and longevity of elite athletes. J. Sci. Med. Sport 13, 410–416. doi: 10.1016/j.jsams.2009.04.010
Timmons, J. A., Baar, K., Davidsen, P. K., and Atherton, P. J. (2012). Is irisin a human exercise gene? Nature 488, E9–E10. doi: 10.1038/nature11364
Traylor, D. A., Gorissen, S. H. M., and Phillips, S. M. (2018). Perspective: protein requirements and optimal intakes in aging: are we ready to recommend more than the recommended daily allowance? Adv. Nutr. 1, 171–182. doi: 10.1093/advances/nmy003
Vieillevoye, S., Poortmans, J. R., Duchateau, J., and Carpentier, A. (2010). Effects of a combined essential amino acids/carbohydrate supplementation on muscle mass, architecture and maximal strength following heavy-load training. Eur. J. Appl. Physiol. 110, 479–488. doi: 10.1007/s00421-010-1520-9
Vinciguerra, M., Musaro, A., and Rosenthal, N. (2010). Regulation of muscle atrophy in aging and disease. Adv. Exp. Med. Biol. 694, 211–233. doi: 10.1007/978-1-4419-7002-2_15
Visvanathan, R., and Chapman, I. (2010). Preventing sarcopaenia in older people. Maturitas 66, 383–388. doi: 10.1016/j.maturitas.2010.03.020
Volek, J. S., Volk, B. M., Gomez, A. L., Kunces, L. J., Kupchak, B. R., Freidenreich, D. J., et al. (2013). Whey protein supplementation during resistance training augments lean body mass. J. Am. Coll. Nutr. 32, 122–135. doi: 10.1080/07315724.2013.793580
Walsh, M. C., Hunter, G. R., and Livingstone, M. B. (2006). Sarcopenia in premenopausal and postmenopausal women with osteopenia, osteoporosis and normal bone mineral density. Osteoporos Int. 17, 61–67. doi: 10.1007/s00198-005-1900-x
White, T. A., and LeBrasseur, N. K. (2014). Myostatin and sarcopenia: opportunities and challenges - a mini-review. Gerontology 60, 289–293. doi: 10.1159/000356740
Wolfaman, N. M., McPherron, A. C., Pappano, W. N., Davies, M. V., Song, K., Tomkinson, K. N., et al. (2003). Activation of latent myostatin by the BMP-1/tolloid family of metalloproteinases. Proc. Natl. Acad. Sci. U.S.A. 100, 15842–15846. doi: 10.1073/pnas.2534946100
Wu, H., Esteve, E., Tremaroli, V., Khan, M. T., Caesar, R., Manneras-Holm, L., et al. (2017). Metformin alters the gut microbiome of individuals with treatment-naive type 2 diabetes, contributing to the therapeutic effects of the drug. Nat. Med. 23, 850–858. doi: 10.1038/nm.4345
Yarasheski, K. E., Bhasin, S., Sinha-Hikim, I., PakLoduca, J., and Gonzalez-Cadavid, N. F. (2002). Serum myostatin-immunoreactive protein is increased in 60-92 year old women and men with muscle wasting. J. Nutr. Health Aging 6, 343–348.
Yoshimura, Y., Wakabayashi, H., Yamada, M., Kim, H., Harada, A., and Arai, H. (2017). Interventions for treating sarcopenia: a systematic review and meta-analysis of randomized controlled studies. J. Am. Med. Dir. Assoc. 18, 553.e1–553.e16. doi: 10.1016/j.jamda.2017.03.019
Yu, J. (2015). The etiology and exercise implications of sarcopenia in the elderly. Int. J. Nurs. Sci. 2015, 199–203. doi: 10.1016/j.ijnss.2015.04.010
Zampieri, S., Pietrangelo, L., Loefler, S., Fruhmann, H., Vogelauer, M., Burggraf, S., et al. (2015). Lifelong physical exercise delays age-associated skeletal muscle decline. J. Gerontol. A Biol. Sci. Med. Sci. 70, 163–173. doi: 10.1093/gerona/glu006
Keywords: exercise, elderly, myokines, protein supplementation, irisin
Citation: Planella-Farrugia C, Comas F, Sabater-Masdeu M, Moreno M, Moreno-Navarrete JM, Rovira O, Ricart W and Fernández-Real JM (2019) Circulating Irisin and Myostatin as Markers of Muscle Strength and Physical Condition in Elderly Subjects. Front. Physiol. 10:871. doi: 10.3389/fphys.2019.00871
Received: 30 January 2019; Accepted: 21 June 2019;
Published: 10 July 2019.
Edited by:
Céline Aguer, Institut du Savoir Montfort (ISM), CanadaReviewed by:
Joo Young Huh, Chonnam National University, South KoreaGiacomina Brunetti, University of Bari Aldo Moro, Italy
Copyright © 2019 Planella-Farrugia, Comas, Sabater-Masdeu, Moreno, Moreno-Navarrete, Rovira, Ricart and Fernández-Real. This is an open-access article distributed under the terms of the Creative Commons Attribution License (CC BY). The use, distribution or reproduction in other forums is permitted, provided the original author(s) and the copyright owner(s) are credited and that the original publication in this journal is cited, in accordance with accepted academic practice. No use, distribution or reproduction is permitted which does not comply with these terms.
*Correspondence: José Manuel Fernández-Real, am1mcmVhbEBpZGliZ2kub3Jn