- 1Department of Physiology and Pharmacology, Universidade Federal de Santa Maria, Santa Maria, Brazil
- 2Department of Microbiology and Parasitology, Universidade Federal de Santa Maria, Santa Maria, Brazil
- 3Department of Industrial Pharmacy, Universidade Federal de Santa Maria, Santa Maria, Brazil
- 4Department of Biology, Faculty of Marine and Environmental Sciences, Instituto Universitario de Investigación Marina, Campus de Excelencia Internacional del Mar, University of Cádiz, Cádiz, Spain
In fish, stressful events initiate a hormone cascade along the hypothalamus-pituitary-interrenal and hypothalamus-sympathetic-chromaffin (HSC) axis to evoke several physiological reactions in order to orchestrate and maintain homeostasis. Several biotic and abiotic factors, as well as aquaculture procedures (handling, transport, or stocking density), activated stress system inducing negative effects on different physiological processes in fish (growth, reproduction, and immunity). In order to reduce these consequences, the use of essential oils (EOs) derived from plants has been the focus of aquaculture studies due to their diverse properties (e.g., anesthetic, antioxidant, and antimicrobial), which have been shown to reduce biochemical and endocrine alterations and, consequently, to improve the welfare status. Recently, several studies have shown that biogenic compounds isolated from different EOs present excellent biological activities, as well as the nanoencapsulated form of these EOs may potentiate their effects. Overall, EOs presented less side effects than synthetic compounds, but their stress-reducing efficacy is related to their chemical composition, concentration or chemotype used. In addition, their species-specific actions must be clearly established since they can act as stressors by themselves if their concentrations and chemotypes used are not suitable. For this reason, it is necessary to assess the effect of these natural compound mixtures in different fish species, from marine to freshwater, in order to find the ideal concentration range and the way for their administration to obtain the desired biological activity, without any undesired side effects. In this review, the main findings regarding the use of different EOs as stress reducers will be presented to highlight the most important issues related to their use to improve fish welfare in aquaculture.
Introduction
The aquaculture industry deals with several stressful situations that can compromise the target species well-being, including handling, confinement, fertilization, transport, and other operations, from the hatchery to the final commercial stage (Ashley, 2007; Sampaio and Freire, 2016; Sneddon et al., 2016; Sánchez-Muros et al., 2017). Stress induced by such practices has long been suspected to cause mortality, affecting the success in fish production with the consequent economic loss for this sector. In addition, the impact of aquaculture-related stressors can also predispose fish to disease (Segner et al., 2012).
Stress response is usually triggered by a wide range of physiological mechanisms in order to compensate the imbalances produced by the stressor and recover the homeostatic status of fish. The stress response is initiated and controlled by two hormonal systems, which lead to the production of catecholamines (such as adrenaline and noradrenaline, and their precursor dopamine) by the hypothalamus-sympathetic-chromaffin (HSC) axis, and corticosteroids (mainly cortisol) by the hypothalamus-pituitary-interrenal (HPI) axis (Wendelaar Bonga, 1997, 2011; Flik et al., 2006; Martos-Sitcha et al., 2014). Behavioral changes are used by the organism to overcome this situation, subsequently generating several responses to the stressor, including gene, metabolic, energetic, immune, endocrine, and neural changes (Schreck and Tort, 2016; Figure 1). Long-term consequences of repeated or prolonged stressful exposures are maladaptive by negatively affecting other necessary life functions, such as growth, development, disease resistance, behavior or reproduction and may even culminate in fish death (Wendelaar Bonga, 1997; Schreck and Tort, 2016). Therefore, several studies assessed the use of sedatives and anesthetics in order to find alternatives to minimize stress effects caused by the intense management practices in aquaculture (Ross and Ross, 2008; Neiffer and Stamper, 2009; Sneddon, 2012; Zahl et al., 2012). Recent excellent reviews have focussed on this topic (Hoseini et al., 2018; Priborsky and Velisek, 2018; Vanderzwalmen et al., 2018).
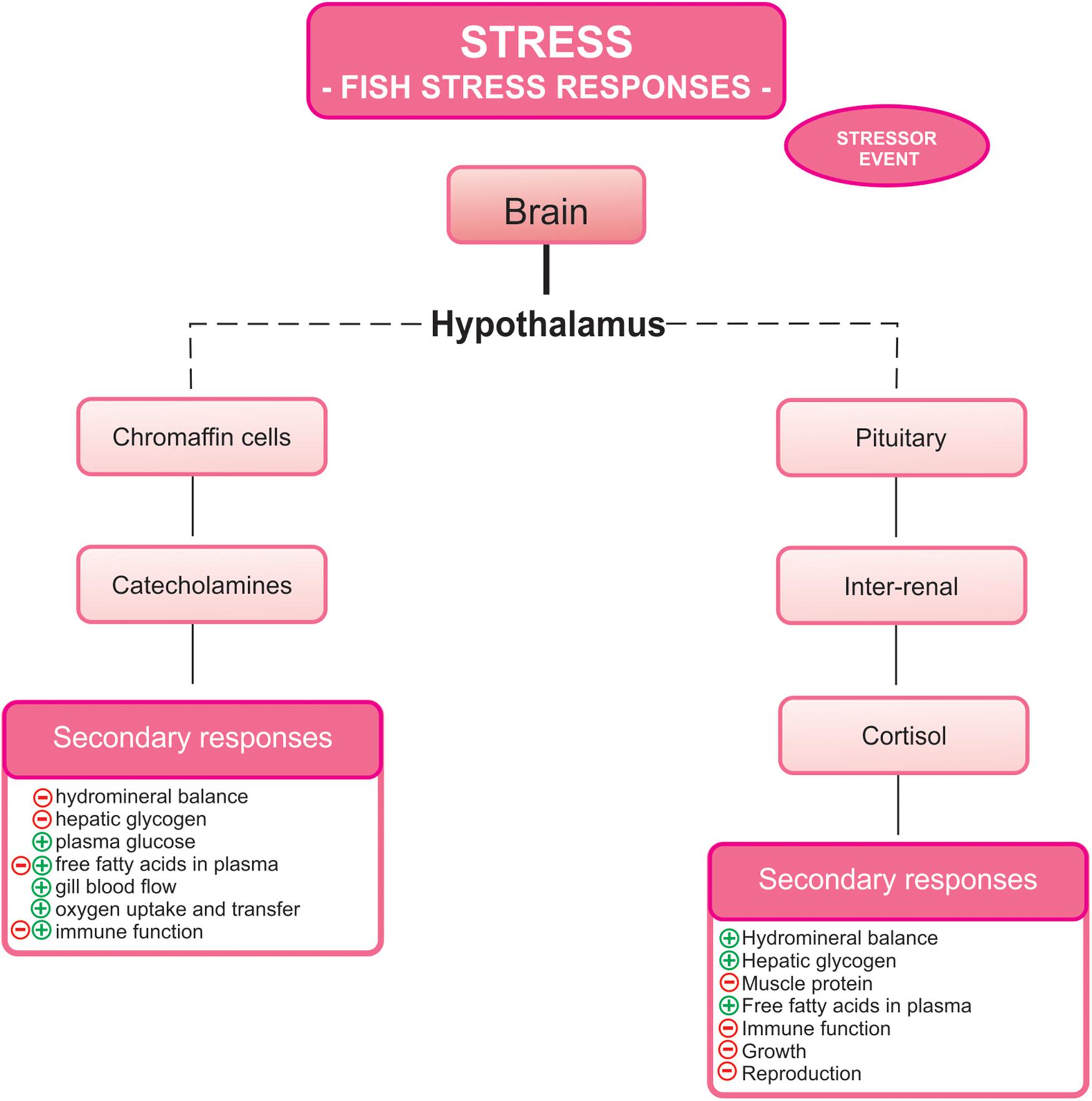
Figure 1. Responses to stress in fish. The activation of the hypothalamus-sympathetic-chromaffin and hypothalamus-pituitary-interrenal axes culminates in the release of catecholamines and cortisol, respectively. These hormones induced several stress secondary responses (modified from Wendelaar Bonga, 1997). (+) indicates activation and/or increase and (−) indicates inhibition and/or reduction.
In addition, essential oils (EOs) derived from plants have been used in aquaculture studies because of their diverse properties that can improve health, growth and welfare of animals (Azambuja et al., 2011; Zeppenfeld et al., 2014; Souza et al., 2018a), as well as reduce stress processes (Saccol et al., 2016, 2018a; Souza et al., 2017b). There are reviews of the effects of EOs as sedatives, anesthetics, antioxidants, and antimicrobials (Cunha et al., 2018; Hoseini et al., 2018; Sutili et al., 2018). Hoseini et al. (2018) focused on stress-reducing effects, but only related to anesthetic effects of the EOs, while the other two reviews did not deal with this subject. Therefore, the aim of the present review is to discuss the possible mechanisms by which the different ways of applying EOs (waterborne and dietary exposure) may minimize stress responses induced by aquaculture procedures, as well as a future perspective of their use.
Essential Oils
Essential oils extracted from plants contain compounds produced during plant secondary metabolism. They constitute one of the most important groups of raw materials for the food, hygiene, and cleaning products and pharmaceutical industries, perfumery, and others. They are complex mixtures of low molecular weight substances (Morais, 2009), with a wide variation in their chemical properties (Hussain et al., 2008). Several studies demonstrate that certain EOs, used as anesthetics and/or sedatives, reduced plasma cortisol levels and attenuated stress response (Cunha et al., 2010; Zeppenfeld et al., 2014; Souza et al., 2017b). Moreover, some EOs when added to therapeutic baths (concentrations lower than those that induce sedation) are able to prevent oxidative stress. The EO of Melaleuca alternifolia, for example, was also able to prevent the inhibition of splenic creatine kinase and pyruvate kinase activities caused by diseases (Baldissera et al., 2017c, 2018), thus demonstrating the possibility to be used as a stress-reducing agent in aquaculture practices. For example, anesthesia with the EO of Lippia alba (citral and linalool chemotypes) prevented plasma cortisol increase in silver catfish (Rhamdia quelen) exposed to air for biometry (Cunha et al., 2010; Souza et al., 2018a), and altered the expression of hormones and enzymes of the HPI axis, proving that it can directly influence this cascade (Souza et al., 2019). Furthermore, it is important to note that EOs have lipophilic properties and liposolubility, contributing to rapid dispersion through biological membranes, including the blood-brain barrier in the central nervous system (CNS), modulating brain function (Zahl et al., 2012; Manayi et al., 2016). It is known that several EOs exert their anesthetic effects by regulating the gamma-aminobutyric acid receptor complex (GABA), the main inhibitory neurotransmitter in the CNS (Bakkali et al., 2008; Chioca et al., 2013; Heldwein et al., 2014; Moreira et al., 2014; Raut and Karuppayil, 2015). Some EOs act in the benzodiazepine site of the GABAA receptor, but others do not (Heldwein et al., 2012; Silva et al., 2012; Garlet et al., 2016; Bianchini et al., 2017; Santos et al., 2017a). In addition, deep anesthesia with Cymbopogon nardus EO promotes a conspicuous depression on muscle contraction power with loss of muscle tonus and transient cardiorespiratory depression (Barbas et al., 2017b).
Definition
EOs are natural multicomponent systems of volatile, lipophilic, odoriferous and liquid substances, obtained from plant raw materials (Edris, 2007). The number of components of an EO generally ranges from 20 to 200, and they are named according to their concentration in the mixture, as (i) major constituents (from 20 to 95%), (ii) secondary constituents (1–20%), and (iii) trace components (below 1%). More than 3,000 distinct chemicals have been detected in EOs, with great variety of chemical structures (Sticher, 2015). Overall, they are classified as terpene hydrocarbons, simple and terpene alcohols, aldehydes, ketones, phenols, esters, ethers, oxides, peroxides, furans, organic acids, lactones, coumarins, or even sulfur compounds (Swamy et al., 2016).
Chemical Composition, Extraction Methods, and Stability of Essential Oils
The genetic characteristics of the producing plant are the most important factors determining the chemical composition of an EO and, consequently, interfere with their biological/pharmacological activities. In addition, the chemical composition is generally specific for a given organ and characteristic of its stage of development, but edaphic and environmental conditions, as well as the extraction method used may cause significant variations (Figueiredo et al., 2008; Reyes-Jurado et al., 2015). Since chemical variability is often high among plants from their natural habitat, whenever possible, EOs should be obtained from cultivated plants that have genetic homogeneity, to ensure the consistency of the composition. However, it is also necessary to observe other aspects that influence the composition of an oil (Sticher, 2015), already cited above, trying to keep them constant.
The EO extraction methods are among the aspects that most affect the chemical characteristics. The main methods of EO extraction are steam distillation and its variants, cold pressing, supercritical fluid extraction, and solvent extraction (Luque de Castro et al., 1999; Capuzzo et al., 2013; Reyes-Jurado et al., 2015). Another variability factor is the occurrence of chemotypes or chemical races, which is often related to geographical variations (Figueiredo et al., 2008). This phenomenon is characterized by botanically identical plants which differ chemically (Aprotosoaie et al., 2017). Consideration should also be given to the relative low stability of EOs, which may undergo chemical changes mainly due to loss of volatile compounds, oxidation reactions and/or polymerization. However, various strategies can be observed to prevent their deterioration, including storage in small volume glass containers, maintaining them under low temperatures and protected from light. Further information on the stability of EOs can be obtained in Turek and Stintzing (2013).
Effects of EOs During Different Stress Events
The interpretation of the general effects of EOs is somewhat complicated because only a few were tested in more than one fish species during different stress events. Furthermore, type and characteristics of stressors used may differ complicating the comparison between EOs. In addition, most studies were performed in freshwater species but not in marine species. Finally, other factors that hinder the comparisons are the existence of different chemotypes for the same plant and the variability of the composition of EOs even from the same chemotype.
Stress-Preventing Effects During Handling Procedures
Exhaustive swimming during attempts to escape from the capture induces changes in physiological parameters, such as increase in glucose and lactate values, when compared to resting animals (Brown et al., 2008; Olsen et al., 2013). Furthermore, previous studies showed that intense swimming activity during chase and capture can be sufficient to compromise flesh quality (Cole et al., 2003) and reproduction (Pankhurst and Van der Kraak, 1997). The handling procedure may also include exposure of fish to air, a situation that requires a short-term orchestration of different endocrine players from both HSC and HPI axes (Wendelaar Bonga, 2011; Skrzynska et al., 2018).
Despite the purpose of using an anesthetic being to mitigate stress, a common observation is that the substance itself may pose as a stressor, thus activating the stress response mechanism (Thomas and Robertson, 1991; Sladky et al., 2001; Bolasina, 2006) and these situations have been observed in fish exposed to MS-222, metomidate, quinaldine sulfate, benzocaine, and phenoxyethanol (Thomas and Robertson, 1991; Tort et al., 2002; Wagner et al., 2002). In addition, several synthetic anesthetics are aversive to fish even at low concentrations (Readman et al., 2013). In this sense, an increasing attention has been focused on the use of plant extracts in fish anesthesia due to a wide range of beneficial health benefits, such as antioxidant, antimicrobial, stress-relieving and immune-promoting effects (Zahl et al., 2010; Hoseini, 2011; Zeppenfeld et al., 2014; Souza et al., 2018b). Moreover, the EOs of Lippia alba (chemotype linalool) and Aloysia triphylla are not aversive to fish (Bandeira Junior et al., 2018), indicating another advantage compared to synthetic anesthetics.
The use of EOs as sedatives or anesthetics to minimize possible damage to fish resulting from handling was recently demonstrated in several species (Cunha et al., 2010; Benovit et al., 2012; Tondolo et al., 2013; Toni et al., 2015; Hashimoto et al., 2016; Pedrazzani and Ostrenski Neto, 2016; Ribeiro et al., 2016; Barbas et al., 2017a, b; Fogliarini et al., 2017; Bodur et al., 2018a, b; Hoseini et al., 2018; Souza et al., 2018a, b). For minor procedures such as biometry and collection of blood samples, lower concentrations of EOs can be used that induce tranquilization and light sedation, in order to minimize stress and reduce plasma cortisol levels (Table 1). The recommended concentration of clove oil (extracted from Syzygium aromaticum or Eugenia aromatica) in handling processes is 10–30 mg⋅L–1 (Javahery et al., 2012). However, exposure seawater gilthead seabream (Sparus aurata) at concentration of 44.5 μL⋅L–1 before air exposure for blood collection increased plasma cortisol and/or glucose values compared to control fish (handled as the anesthetized fish) (Bressler and Ron, 2004). Previous anesthesia with 10 mg⋅L–1 clove oil did not change plasma cortisol and glucose levels of the seawater meager manipulated for weighing compared to control fish (handled as the anesthetized fish) (Barata et al., 2016). On the other hand, for seawater Senegal sole (Solea senegalensis) anesthesia with 1000 mg⋅L–1 clove oil demonstrated a good ability of clove oil to prevent cortisol, lactate and glucose increases pre-mortem (Ribas et al., 2007). In addition, studies demonstrated that the faster anesthesia induction with eugenol and clove oil, the lower the stress response provoked by these anesthetics (Hoseini and Nodeh, 2013; Mirghaed et al., 2018) thus, low concentrations of eugenol besides causing slow anesthetic induction may cause disturbances in plasma glucose levels and plasma cortisol.
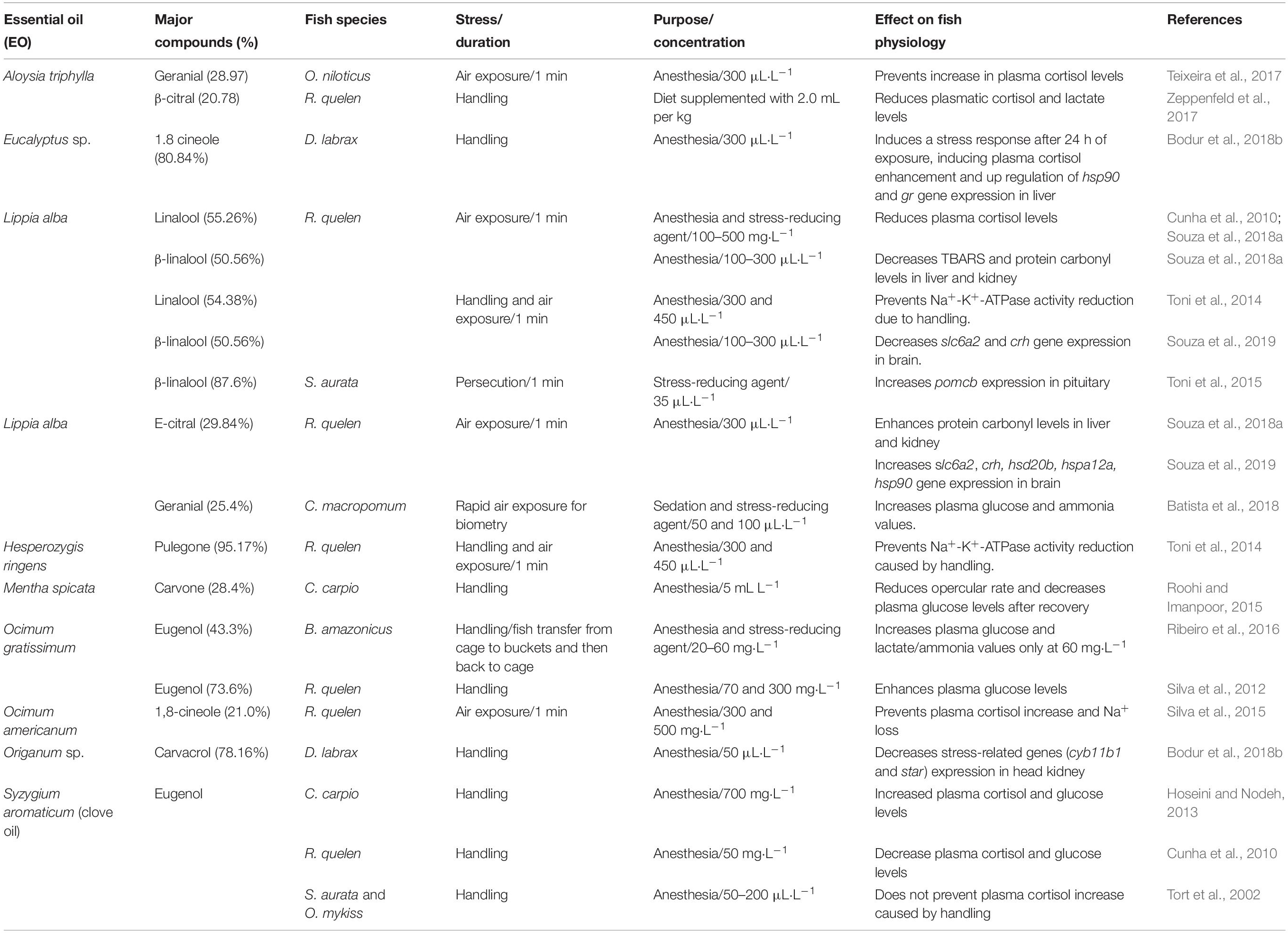
Table 1. The use of essential oils (EOs) as stress-reducing agents in fish exposed to handling and air exposure.
The effect of the EOs may also change according to the species and the type of application. For example, Nile tilapia anesthetized with 300 μL⋅L–1 of the EO of A. triphylla and then exposed to air for 1 min decreased plasma cortisol levels respect to control fish (handled as the anesthetized fish) (Teixeira et al., 2017). However, silver catfish anesthetized with 135 and 180 mg⋅L–1 and submitted to the same procedure did not change plasma cortisol values compared to control fish (Gressler et al., 2014). Interestingly, silver catfish fed diet supplemented with 2.0 mL per kg of this EO for 21 days and handled for blood collection presented lower plasma cortisol and lactate levels, but not glucose, than fish that received control feed and were submitted to the same procedure (Zeppenfeld et al., 2017; Table 1). However, the same dietary dose of this EO did not change whole body cortisol content in zebrafish handled for biometry (Zago et al., 2018).
The EO of Ocimum americanum used as anesthetic prevented plasma cortisol increase and Na+ loss induced by aerial exposure (1 min) in silver catfish compared to control fish (handled as the anesthetized fish) (Silva et al., 2015). However, the EO of a plant from the same genus, but different species (O. gratissimum, with eugenol and 1,8-cineole as the main compounds), did not prevent this cortisol enhancement from air exposure. In addition, hyperglycemia was verified in fish exposed to 70 and 300 mg⋅L–1 at 1 and 4 h after handling, indicating no effect on attenuation of stress axis activation (Silva et al., 2015). Matrinxãs (Brycon amazonicus) transferred from cage nets to 20 L buckets with 60 mg⋅L–1 of the EO of O. gratissimum for 10 min also increased plasma glucose levels and did not prevent plasma lactate enhancement compared to control fish (handled as the anesthetized fish) (Ribeiro et al., 2016; Table 1). Consequently, even having eugenol as one of the main compounds, the efficacy of these two EOs to reduce stress is species-specific.
Anesthesia of silver catfish with the EO L. alba (300 μL⋅L–1) before handling and air exposure (1 min) prevented plasma cortisol increase observed 1 and 4 h later in control fish (handled as the anesthetized fish) (Cunha et al., 2010). In addition, both linalool and citral chemotypes of this EO (300 μL⋅L–1) reduced plasma cortisol through anesthesia and the first 10 min of recovery compared to control fish (handled as the anesthetized fish) (Souza et al., 2017b). Finally, individuals of this species anesthetized with linalool chemotype of this EO (100 and 300 μL⋅L–1) decreased, at 4 h after anesthesia and air exposure for biometry and compared to control fish, expression of genes directly related to stress: corticotropin releasing hormone (crh), and solute carrier family 6 (neurotransmitter transporter, noradrenalin) member 2 (slc6a2) (Souza et al., 2019). However, the citral chemotype is stressful for silver catfish because there was an up regulation of slc6a2, crh, 20β-hydroxysteroid dehydrogenase (hsd20b) and heat shock proteins 70 member 12 (hspa12a) and heat shock protein 90 (hsp90) (Souza et al., 2019; Table 1).
Similarly to other EOs, the efficacy of the linalool chemotype of the L. alba EO as a stress reducing agent can change with the concentration and/or species. So, 200 μL⋅L–1 did not change plasma cortisol levels in the hybrid tambacu (Piaractus mesopotamicus × Colossoma macropomum) subjected to handling compared to control fish (handled as the anesthetized fish) (Sena et al., 2016). However, anesthesia of tambaqui (Colossoma macropomum) with 50 and 100 μL⋅L–1 EO of this plant, but of the chemotype citral, did not prevent plasma glucose and ammonia enhancement due to rapid air exposure for biometry (Batista et al., 2018; Table 1).
Others EOs from different plants showed variable effects depending on the species tested. Anesthesia with the EOs of Hesperozygis ringens (137–277 μL⋅L–1) and Ocotea acutifolia (300 μL⋅L–1) did not change blood glucose levels in silver catfish (Silva et al., 2013). The EOs of Myrcia sylvatica and Curcuma longa anesthetized matrinxã and reduced plasma cortisol levels compared to control fish (handled as the fish exposed to EOs) (Saccol et al., 2017). Finally, the use of 50 μL⋅L–1 of oregano EO (Origanum sp.) as anesthetic decreased the expression of steroidogenic genes at 2 and 24 h compared to clove oil, demonstrating the efficiency of oregano EO in reducing secondary stress in European sea bass (Bodur et al., 2018a, b; Table 1).
In the marine dusky grouper the use of 50 and 300 μL⋅L–1 of the EO of Aloysia polystachya for, respectively, sedation and anesthesia, did not affect plasma glucose, Na+, and Cl– levels compared to control fish (handled as the anesthetized fish) (Fogliarini et al., 2017; Figure 2).
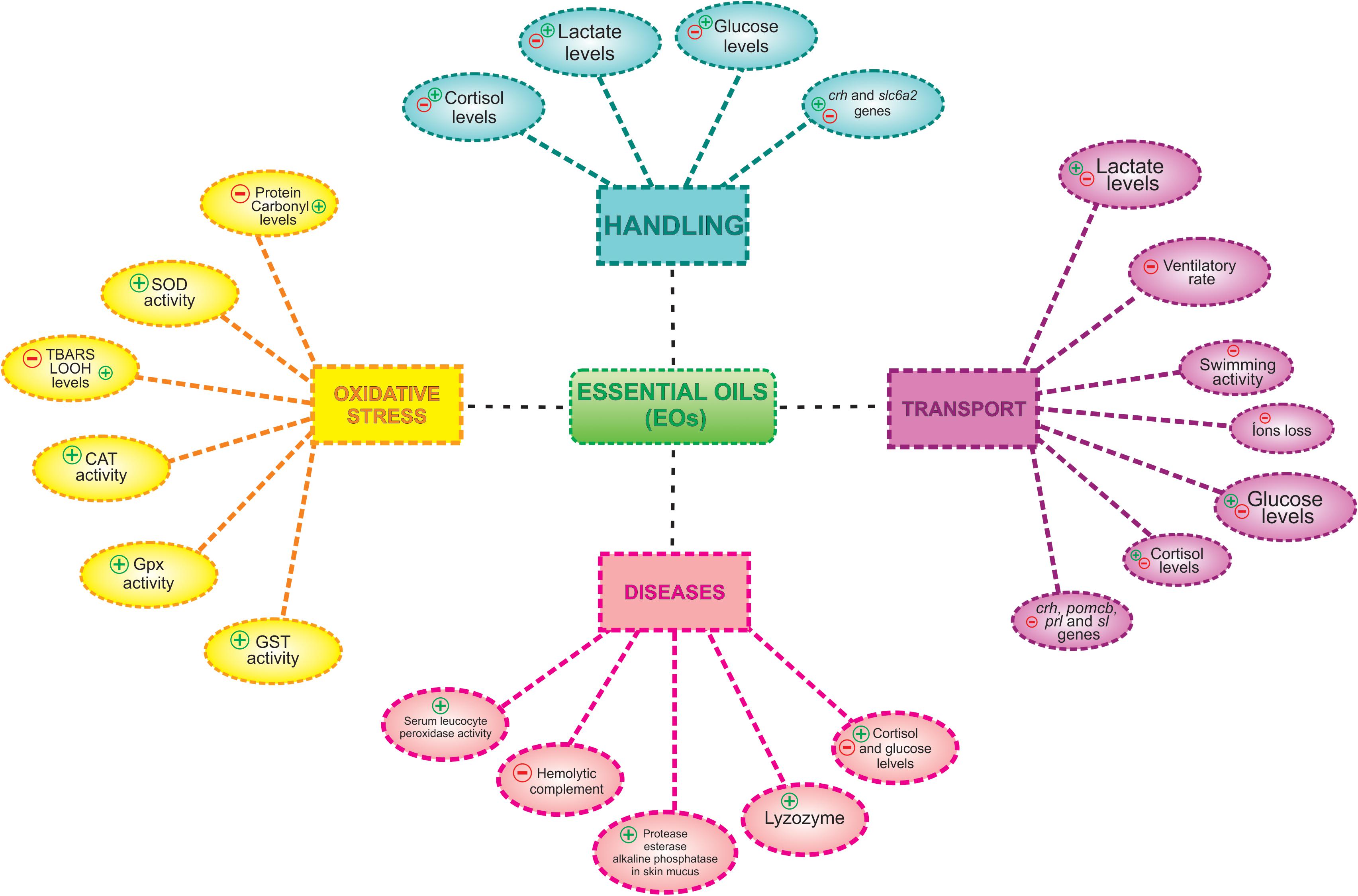
Figure 2. Effects of essential oils (EOs) on physiological changes induced by aquaculture procedures, handling, transport, and diseases. (+) indicates activation and/or increase and (−) indicates inhibition and/or reduction.
Usually, EOs are applied in water prior to handling. However, it would be interesting to test the efficacy of EOs if other means of administration were used. In this sense, dietary addition (0.5–2.0 mL⋅kg–1) of cinnamon (Cinnamomun sp.) EO did not alter plasma cortisol and glucose levels of Nile tilapia exposed to air for 3 min compared to control fish, which was fed diet without this EO and subjected to the same procedure) (Santos et al., 2016).
Stress-Preventing Effects During Transport Procedures
Live transportation of larvae, juvenile or broodstock is one of the major causes of stress in fish due to the capture, packing, high loading density, changes in water quality, the transport itself, unloading, and the final storage (Harmon, 2009; Sampaio and Freire, 2016). In all transport systems, including simple closed systems, such as sealed plastic bags partly filled with water and oxygen, homeostasis of the transported fish is mainly challenged by the build-up of ammonia (NH3 and NH4+) due to excretion, and carbon dioxide (CO2) from respiration (Lim et al., 2003; Paterson et al., 2003; Becker et al., 2016). These events cause changes in pH (Golombieski et al., 2003), which may alter fish metabolism and induce activation of the stress system. So, transportation can lead to a number of physiological responses such as catecholamines and corticosteroids release as well as blood glucose levels enhancement (Pottinger, 2008; Zeppenfeld et al., 2014). In addition, transport significantly decreased corticotrophin-releasing hormone (crh) and urotensin I (ui) expression levels in the brain of Coilia nasus and induced oxidative stress (Du et al., 2015). Furthermore, stress by transport induced an osmotic imbalance with ion loss in freshwater fish, showing a good correlation with transport stress (Becker et al., 2013, 2016; Parodi et al., 2014; Zeppenfeld et al., 2014; Garcia et al., 2015; Ostrensky et al., 2016; Teixeira et al., 2018; Table 2). Thus, during or prior to transport, sedation of the fish is desirable to reduce or prevent stress.
The EO of L. alba (linalool chemotype) at 10 or 20 μL⋅L–1 added to the transport water of silver catfish reduced net Na+, Cl–, and K+ loss compared to control fish subjected to the same procedure (Becker et al., 2012). The same chemotype of this EO (10 μL⋅L–1) prevented plasma cortisol (but not glucose) increase provoked by transport in tambacu (Sena et al., 2016; Table 2). A similar concentration of this EO (15 μL⋅L–1) inhibited plasma glucose enhancement in slender seahorses (Hippocampus reidi) transported in plastic bags (Cunha et al., 2011). However, meager submitted to simulated transport with 12 mg⋅L–1 of the EO of L. alba (linalool chemotype) presented higher plasma cortisol levels compared to control fish subjected to the same procedure (Cárdenas et al., 2016; Table 2). The transport of common carp in freshwater with 50–200 mg⋅L–1 linalool decreased ammonia excretion, but increased serum cortisol, glucose and urea values and did not prevent the decrease of serum Na+ and Cl– provoked by transport compared to control fish submitted to the same procedure (Mazandarani et al., 2017). Recent studies showed that EO of L. alba (chemotype linalool) at 5–10 μL⋅L–1 reduced swimming activity of the black piranha, Serrasalmus rhombeus, compared to control fish subjected to the same procedure and may also be considered as options for the transport of this species (Almeida et al., 2018). These results obtained so far with the use of the EO of L. alba (linalool chemotype) and linalool for the transport of fish do not lead to the conclusion whether the differences are related to species, concentrations, or water quality (fresh and seawater).
The EO of A. triphylla has also been tested for transport procedures in freshwater and marine species. The use of this EO (40 and 50 μL⋅L–1) during a 6 h transport reduced net ion loss (Parodi et al., 2014), ammonia excretion and plasma cortisol levels in silver catfish compared to control fish submitted to the same procedure (Zeppenfeld et al., 2014). Plasma cortisol enhancement in fat snook due to transport was reduced by adding 20 μL⋅L–1 of this EO to the water (Parodi et al., 2016), whereas 30 μL⋅L–1 decreased ventilatory rate, ion loss and plasma glucose levels, with no effects preventing cortisol increase in Nile tilapia (Teixeira et al., 2018), both compared to their respective control fish subjected to the same procedure. In addition, this EO (25 μL⋅L–1) also decreased ventilatory rate of pacamã, Lophiosilurus alexandri, as well as ammonia excretion during 4 h transport (Becker et al., 2017; Table 2), also reducing the swimming activity of black piranha at concentration of 10 μL⋅L–1 (Almeida et al., 2018) both compared to their respective control fish subjected to the same procedure. Overall, this EO seems to be effective in reducing stress of transport in fish, irrespective of whether they are fresh- or seawater-adapted.
Transport of the Brazilian flounder for 7 h with the EOs of Aloysia gratissima (90 mg⋅L–1) and Ocimum gratissimum (10–20 mg⋅L–1) increased blood glucose levels compared to control fish subjected to the same procedure. In addition, the EO of A. gratissima induced mortality. Consequently, both EOs are not effective in transport of this species (Benovit et al., 2012). Moreover, the EO of Nectandra megapotamica at 15 or 30 μL⋅L–1 was not able to prevent the stress of transport in fat snook because it did not prevent deterioration in water quality and the post-transport mortality compared to control fish subjected to the same procedure (Tondolo et al., 2013; Table 2). Studies of the effect of these EOs in freshwater species are still lacking. Simulated transport (6 h) of gilthead seabream with clove oil at 2.5 mg⋅L–1 increased plasma cortisol levels and expression of head kidney gene-expressions related to cortisol production (star and cyp11b1) and stimulated amino acids catabolism (Jerez-Cepa et al., 2019). The gill’s mRNA levels of gst3, a target gene related with the antioxidant response and cell-tissue repair, enhanced after transportation with clove oil, returning to control values after recovery. However, the rest of transcripts assessed (gpx1, cat, gr, mt, and hsp70) related to these responses did not present any alteration (Teles et al., 2019; Table 2). Authors proposed that alternative concentrations of this EO should be tested for the transport of gilthead seabream.
Transport of silver catfish with the EO of Myrcia sylvatica (25 or 35 μL⋅L–1) reduced plasma cortisol and lactate levels, concomitantly with the decrease in the gene expression of crh and proopiomelanocortin b (pomcb), prolactin and somatolactin mRNAs compared to control fish without EO administration and subjected to the same procedure (Saccol et al., 2018b). Exposure of silver catfish to the EOs of Citrus × aurantium, Citrus × latifolia (50–100 μL⋅L–1), increased ventilatory frequency, but reduced ion loss and ammonia excretion compared to control fish subjected to the same procedure (Lopes et al., 2018). The EOs of Cunila galioides (25 and 50 μL⋅L–1) and Origanum majorana (100 μL⋅L–1) also decreased ion loss (Cunha et al., 2017) and Lippia origanoides (5–10 μL⋅L–1) reduced ventilatory frequency in silver catfish after 6 and/or 8 h of exposure (Becker et al., 2018) both compared to their respective control fish subjected to the same procedure. For instance, these EOs apparently reduced stress and may also be useful for fish transport (Figure 2).
Stress-Preventing Effects During High Stocking Density
Recent studies demonstrated that EOs are capable of mitigating or preventing stress caused by different stocking densities. Dietary addition of 0.50 mL per kg EO L. alba (linalool chemotype) prevented the increase in cortisol levels in silver catfish submitted to a stressful condition of high stocking density (Souza et al., 2015). Similarly, a diet supplemented with M. sylvatica EO (2.0 mL per kg) for 90 days reduced cortisol levels in gilthead seabream after 22 days held at high stocking density (40 kg m–3) (Saccol et al., 2018a). These results may stimulate new studies on the influence of EOs on stress reduction caused by the different stocking densities of fish in culture systems.
Regulation of Oxidative Stress
Pro-oxidant compounds are the oxygen reactive species (ROS) that can damage DNA, proteins and lipids (Evans and Halliwell, 1999; Morel and Barouki, 1999), whereas the antioxidants are any substance that can prevent or reduce the oxidation of a target molecule (Halliwell and Gutteridge, 2007). To maintain homeostasis, fish eliminate ROS to counteract oxidative stress and prevent or repair oxidative damage by antioxidant defense system, which includes antioxidant enzymes such as superoxide dismutase (SOD), catalase (CAT), glutathione peroxidase (GPx), glutathione-S-transferase (GST), and also some non-enzymatic antioxidants such as non-protein thiol (NPSH) (Tu et al., 2012; Birnie-Gauvin et al., 2017), in order to avoid lipid peroxidation, and carbonylation of proteins, usually evaluated by the biomarkers thiobarbituric acid-reactive substances (TBARS) and carbonyl protein (PC), respectively. In this sense, the presence of ROS in the cells triggers biochemical reactions that will culminate in decreased cellular function due to oxidative damage caused in proteins, carbohydrates and lipids, which can lead to apoptosis and accumulation of oxidized molecular aggregates. Normally, an oxidative stress frame is triggered due to an imbalance between the oxidant and the antioxidant production, detected by low concentrations of antioxidant enzymes and higher prooxidant levels, culminating with a lower immune system response (Biller and Takahashi, 2018).
Several EOs, when used as anesthetics or sedative for manipulation and/or transport, exhibit antioxidant capacity (Tables 1, 2). This antioxidant activity is also observed when the EOs are used at concentrations lower than those that induce sedation and as dietary supplements (Tables 1–3). Thus, the antioxidant effects of several EOs are not only due to the possibility of lower ROS production when fish are sedated or anesthetized.
We highlight the EO of L. alba because it presents different responses to oxidative stress, which varies according to its concentration, chemotype, and fish species. For example, the addition of this EO (10–20 μ⋅L–1, linalool chemotype) during transport suppressed oxidative stress in silver catfish (Azambuja et al., 2011). However, this EO (linalool chemotype) at higher concentrations (30–40 μ⋅L–1) induced physiological and oxidative stress in silver catfish and gilthead sea bream (Salbego et al., 2014; Toni et al., 2015). On the one hand, silver catfish anesthetized with the chemotypes linalool and citral did not increase renal and hepatic thiobarbituric acid reactive species levels after anesthesia, avoiding lipid damage. On the other hand, fish anesthetized with the citral chemotype showed higher protein carbonylation levels, superoxide dismutase, catalase, and glutathione S-transferase activities as well as non-protein thiol group values in both tissues compared to controls. Therefore, the EO of both chemotypes present antioxidant capacity, but anesthesia with higher concentrations of the linalool chemotype does not cause damage to lipids or proteins, being more effective in anesthetizing silver catfish (Souza et al., 2018a).
Interestingly, anesthesia of silver catfish with the EO of A. triphylla (135 and 180 mg⋅L–1), whose main compound is citral, was capable of preventing the formation of lipid peroxides in the liver, possibly due to the increase of catalase and glutathione-S-transferase activities (Gressler et al., 2014). Similarly, anesthesia and sedation of tambaqui with the EOs of M. sylvatica and C. longa resulted in lower levels of lipid peroxidation and higher activity of antioxidant enzymes (superoxide dismutase, catalase, glutathione peroxidase, glutathione reductase, and glutathione-S-transferase), the content of non-protein thiols and total reactive antioxidant potential in several tissues (brain, liver, gills, and kidney) compared to control (Saccol et al., 2016). Anesthesia of common carp with 30 mg⋅L–1 clove oil did not alter TBARS or carbonyl protein levels in several tissues, but reduced superoxide dismutase in the brain as well as glutathione reductase and glutathione peroxidase in the brain and gills (Velisek et al., 2011).
In addition, the use of EOs in water during transport is also useful in order to activate the antioxidant defense system. Silver catfish transported with the EOs of L. alba (chemotype linalool) (10 and 20 μL⋅L–1) and A. triphylla (30 and 40 μL⋅L–1) in the transport water showed improvement in the redox state (Azambuja et al., 2011; Zeppenfeld et al., 2014; Salbego et al., 2017). However, this effect is dependent on the concentrations used. So, specimens treated with the EO of L. alba (chemotype linalool) prior to transport (200 μL⋅L–1 for 3 min) and transported for 6 h (with 30 or 40 μL⋅L–1) decreased significantly hepatic catalase, glutathione-S-transferase, glutathione peroxidase, non-protein thiol groups, and ascorbic acid levels compared to the control group (Salbego et al., 2014). This study also revealed that hepatic TBARS, protein oxidation levels, and the lipid peroxidation/catalase+glutathione peroxidase ratio were significantly higher in fish transported with both concentrations of this EO, indicating the existence of oxidative stress at hepatic level.
Exposure to EO of Melaleuca alternifolia (25 μL⋅L–1 – light sedation) for 6 h decreased hepatic TBARS levels followed by an increase in glutathione-S-transferase activity (Souza et al., 2018b). Finally, a sedative concentration (30 μL⋅L–1) of N. grandiflora EO enhanced protection against oxidative damage mainly in muscle and gills of tambaqui transported for up to 10 h (Barbas et al., 2017b; Figure 2).
The dietary supplementation for 20–60 days with different EOs usually improved oxidative status of freshwater fish. Channel catfish (Ictalurus punctatus) fed with 0.5 mL per kg EO of Origanum vulgare increased plasma lysozyme, catalase, and superoxide dismutase compared to fish fed a control diet (Zheng et al., 2009). The dietary addition of the EOs of Cymbopogon citratus (0.2 g per kg) and Pelargonium graveolens (0.4 g per kg) enhanced catalase and lysozyme activities and glutathione reductase content and reduced malondialdehyde from the whole body of the Nile tilapia compared to the basal diet (Al-Sagheer et al., 2018). Silver catfish fed 2.0 mL per kg EO of A. triphylla presented lower TBARS, lipid hydroperoxide, superoxide dismutase, catalase, and non-protein thiols in the brain, liver, and muscle of fish fed a control diet (Zeppenfeld et al., 2017). The same species fed diets supplemented with EO of L. alba (linalool chemotype, around 0.5–2.0 mL per kg) stimulated superoxide dismutase, catalase and glutathione peroxidase activities and non-protein thiols content, whereas reduced lipoperoxidation and TBARS in several organs compared to those fed a control diet (Saccol et al., 2013). Finally, hepatic superoxide dismutase and glutathione peroxidase activities enhanced while catalase, glutathione reductase, glutathione-S-transferase and malondialdehyde decreased in rainbow trout fed 0.5–1.0 g per kg diet of EOs of Salvia officinalis, Mentha spicata, and Thymus vulgaris compared to that of the control-diet fed group (Sönmez et al., 2015).
Regulation of Immune System and/or Bactericidal Effects
The negative influence of stress on the immune system is well documented (Tort et al., 2003; Tort, 2011). In this regard, there has been a trend in the use of EOs for improving immune responses and disease resistance in fish (Bulfon et al., 2015). Often, the immunostimulants are administered as immersion or food additives, usually improving the innate (non-specific) defense mechanisms, increasing resistance to specific pathogens and promoting a recovery from immunosuppression states caused by stress (Sakai, 1999; Barman et al., 2013).
Some EOs used as anesthetics were also related as good immunostimulants for fish (Table 3). Anesthesia of gilthead seabream with 44.5 mg⋅L–1 clove oil did not change serum lysozyme activity, respiratory burst, and pinocytosis activity of head kidney (Bressler and Ron, 2004), but a concentration of 55 mg⋅L–1 increased serum leucocyte peroxidase activity and decreased hemolytic complement activity, which led to the conclusion that this EO did not change immune response in this species (Bahi et al., 2018). However, rainbow trout anesthetized with 25 mg⋅L–1 clove oil enhanced lysozyme and bactericidal activity, as well as protease, esterase, and alkaline phosphatase activities in the skin mucus, indicating potentiated skin mucosal immunity (Soltanian et al., 2018). Similarly, preventive baths with the EOs of H. ringens and O. americanum (20 mg⋅L–1) for 5 days increased survival in silver catfish infected with A. hydrophila (Sutili et al., 2015). Some EOs can have antimicrobial effects and, at the same time, act as immunostimulants. This is supported by the effects of the EO of Melaleuca alternifolia, which showed an antimicrobial effect against A. hydrophila, increased the non-specific immune system and prevented oxidative damage in silver catfish in fish exposed to 50 μL⋅L–1 for 7 days prior to infection (Baldissera et al., 2017a; Table 3).
The EOs may also have an immunostimulatory effect when added to the diet. For example, diet supplemented with the EO of Zataria multiflora enhanced common carp immunity to some extent even though fish could not express their potential immunity during stress caused by low temperature (Soltani et al., 2010). After 35 days of supplementation with EO O. gratissimum (0.5%), the Nile tilapia presented the lowest value of hematocrit and the highest numbers of neutrophils at 35 days. It can be inferred that this reduction is due to an energy offset for production of defense cells – in this case, neutrophils (Brum et al., 2017; Table 3). Adel et al. (2015) also observed a significant increase in neutrophils in the cyprinid kutum (Rutilus frisii) after dietary supplementation with the EO of Mentha piperita, asserting that there was stimulation of the innate immune response. However in spite of improving survival of silver catfish after A. hydrophila infection, the addition of 2.0 mL per kg diet of EO of A. triphylla showed an immunosuppressive activity because the total count of leukocytes, lymphocytes and neutrophils decreased (Santos et al., 2017b). In addition, supplementing the diet with EO of Citrus limon peels (0.5, 0.75, and 1%) improve non-specific immune parameters and decreased mortality rates in the Mozambique tilapia Oreochromis mossambicus challenged with Edwardsiella tarda (Baba et al., 2016; Table 3). These results indicate that diets enriched with EOs often present promising results for fish, and some EOs may even aid in improving the immune system as well as helping to prevent disease outbreaks in aquaculture systems.
Conclusion and Future Perspectives on the Use of EOs in Aquaculture
Some EOs demonstrated advantages when used as sedatives/anesthetics compared to synthetic compounds because they are not aversive to fish, and reduce the stress responses related to handling and transport. On the other hand, their effects may vary according to plant variables such as chemotype, place of collection, climate, anatomical part from which the EO was extracted, altering significantly their composition and consequently their effects. Consequently, EOs should be obtained from cultivated plants that have genetic homogeneity, to ensure the consistency of their composition. Another perspective to be followed by future studies and by the pharmaceutical industry would be isolating/purifying the active compounds present in
the EOs to obtain more refined products that combine their beneficial effects with a better control of composition and maybe avoid undesired effects of uncontrolled compounds. Some isolated compounds, as linalool, have proportional anesthetic effect to the EO of L. alba chemotype linalool in silver catfish, but eugenol, which is the main compound of O. gratissimum, presents a narrower safety range that this EO. Additional studies comparing the effects and side effects of EOs and their isolated compounds are necessary to evaluate the advantages of using a given EO or its main compound(s). In addition, it is important to emphasize that the use of different concentrations of the EOs and the different fish species used can alter behavioral and physiological responses, as observed in synthetic anesthetics. As explained in a previous section, the stress-reducing effect of eugenol is better the faster the anesthesia induction. Is this relationship concentration/time to induce anesthesia/stress-reducing effect similar for EOs and their isolated compounds? Could this possible relationship explain, at least partially, the species-specific effects of the EOs studied so far?
Most studies show that EO also have promising potential for maintaining and promoting health, as well as preventing and potentially treating some diseases, ameliorating growth and antioxidant status. However, besides the high volatility, EOs can easily decompose, owing to direct exposure to heat, humidity, light, or oxygen, which would imply the loss of their effectiveness (Turek and Stintzing, 2013). Thus, it is interesting to develop technology to protect EOs when exposed to temperature variations and even to protect from low pH during gastric absorption (Zhang et al., 2016). Studies regarding the use of diets supplemented with several EOs to minimize the stress caused by handling or air exposure remain scarce. In addition, due to the species-specific effects of EOs, it would be interesting to check the efficacy of these EOs on more fresh- and seawater species.
The use of nanotechnology can potentialize the EO effects. Lecithin-based nanoemulsion of clove oil showed faster anesthesia induction and recovery in goldfish, Carassius auratus. Serum glucose was not different, but serum urea was higher in goldfish anesthetized with the nanoemulsion than with free clove oil (Gholipourkanani et al., 2015). The nanoencapsulated EO of M. alternifolia exert potent bactericidal action, presenting 100% of curative efficacy in silver catfish infected with Pseudomonas aeruginosa, while free EO only showed 70% of curative efficacy (Souza et al., 2017c). Consequently, nanotechnology shows promising results that deserve further analysis.
Medicinal plants have broad antimicrobial properties against important fish pathogens. Further studies on chronic and acute toxicity and on the deleterious effects of herbal medicines on treated organisms, non-target organisms, and the environment are encouraged. Most studies on effectiveness have been based on in vitro testing or even conducted under laboratory conditions. Therefore, further practical and economic studies are needed to enable replacement of the current treatments. Joint work between the supply chain, industry, and researchers are paramount to studying medicinal plants that may present antimicrobial effects against important fish pathogens.
Author Contributions
CS wrote the manuscript and drew the figures. BH wrote from the section “Essential Oils.” BB, JM-S, JM, and MB carried out the corrections on the manuscript.
Funding
BH and BB received the Conselho Nacional de Desenvolvimento Tecnológico, Brazil (CNPq) research fellowships, and MB and CS received Ph.D. fellowships from the Coordenação de Aperfeiçoamento de Pessoal de Nível Superior, Brasil (CAPES) – Finance Code 001. This research was funded by the projects AGL2016-76069-C2-1-R of MICINN (Spain) with the support of the FEDER funds (European Union) and Fundação de Amparo à Pesquisa do Estado do Rio Grande do Sul (FAPERGS). JM-S and JM belong to the Fish Welfare and Stress Network (AGL2016-81808-REDT) supported by the MINECO.
Conflict of Interest Statement
The authors declare that the research was conducted in the absence of any commercial or financial relationships that could be construed as a potential conflict of interest.
References
Adel, M., Abedian Amiri, A., Zorriehzahra, J., Nematolahi, A., and Esteban, M. Á. (2015). Effects of dietary peppermint (Mentha piperita) on growth performance, chemical body composition and hematological and immune parameters of fry Caspian white fish (Rutilus frisii kutum). Fish Shellfish Immunol. 45, 841–847. doi: 10.1016/j.fsi.2015.06.010
Akar, A. M. (2011). Effects of clove oil on the response of blue tilapia (Oreochromis aureus) by transportation stress. J. Arabian Aquacult. Soc. 6, 77–86.
Almeida, A. P. G., Heinzmann, B. M., Val, A. L., and Baldisserotto, B. (2018). Essential oils and eugenol as anesthetics for Serrasalmus rhombeus. Bol. Inst. Pesca 44, 44–50. doi: 10.20950/1678-2305.2018.195
Al-Sagheer, A. A., Mahmoud, H. K., Reda, F. M., Mahgoub, S. A., and Ayyat, M. S. (2018). Supplementation of diets for Oreochromis niloticus with essential oil extracts from lemongrass (Cymbopogon citratus) and geranium (Pelargonium graveolens) and effects on growth, intestinal microbiota, antioxidant and immune activities. Aquacult. Nutr. 24, 1006–1014. doi: 10.1111/anu.12637
Aprotosoaie, A. C., Gille, E., Trifan, A., Luca, V. S., and Miron, A. (2017). Essential oils of Lavandula genus: a systematic review of their chemistry. Phytochem. Rev. 16, 761–799. doi: 10.1007/s11101-017-9517-1
Ashley, P. J. (2007). Fish welfare: current issues in aquaculture. Appl. Anim. Behav. Sci. 104, 199–235. doi: 10.1016/j.applanim.2006.09.001
Azambuja, C. R., Mattiazzi, J., Riffel, A. P. K., Finamor, I. A., Garcia, L. O., Heldwein, C. G., et al. (2011). Effect of the essential oil of Lippia alba on oxidative stress parameters in silver catfish (Rhamdia quelen) subjected to transport. Aquaculture 319, 156–161. doi: 10.1016/j.aquaculture.2011.06.002
Baba, E., Acar, U., Ontas, C., Kesbicß, O. S., and Ylmaz, S. (2016). Evaluation of Citrus limon peels essential oil on growth performance, immune response of Mozambique tilapia Oreochromis mossambicus challenged with Edwardsiella tarda. Aquaculture 465, 13–18. doi: 10.1016/j.aquaculture.2016.08.023
Bahi, A., Guardiola, F. A., and Esteban, M. A. (2018). A time course study of glucose levels and innate immune response in gilthead seabream (Sparus aurata L.) after exposure to clove oil-eugenol derived anaesthetic. Fish Shellfish Immun. 77, 280–285. doi: 10.1016/j.fsi.2018.03.057
Bakkali, F., Averbeck, S., Averbeck, D., and Idaomar, M. (2008). Biological effects of essential oils - a review. Food Chem. Toxicol. 46, 446–475.
Baldissera, M. D., Souza, C. F., and Baldisserotto, B. (2018). Melaleuca alternifolia essential oil prevents bioenergetics dysfunction in spleen of silver catfish naturally infected with Ichthyophthirius multifiliis. Microb. Pathog. 123, 47–51. doi: 10.1016/j.micpath.2018.06.042
Baldissera, M. D., Souza, C. F., Bandeira Junior, G., Vargas, A. C., Boligon, A. A., Campos, M. M. A., et al. (2017a). Melaleuca alternifolia essential oil enhances the non-specific immune system and prevents oxidative damage in Rhamdia quelen experimentally infected by Aeromonas hydrophila: effects on cholinergic and purinergic systems in liver tissue. Fish Shellfish Immun. 61, 1–8. doi: 10.1016/j.fsi.2016.12.016
Baldissera, M. D., Souza, C. F., Doleski, P. H., de Vargas, A. C., Duarte, M. M. M. F., Duarte, T., et al. (2017b). Melaleuca alternifolia essential oil prevents alterations to purinergic enzymes and ameliorates the innate immune response in silver catfish infected with Aeromonas hydrophila. Microb. Pathog. 109, 61–66. doi: 10.1016/j.micpath.2017.05.026
Baldissera, M. D., Souza, C. F., Moreira, K. L. S., Rocha, M. I. U. M., Veiga, M. L., and Baldisserotto, B. (2017c). Melaleuca alternifolia essential oil prevents oxidative stress and ameliorates the antioxidant system in the liver of silver catfish (Rhamdia quelen) naturally infected with Ichthyophthirius multifiliis. Aquaculture 480, 11–16. doi: 10.1016/j.aquaculture.2017.07.042
Bandeira Junior, G., Abreu, M. S., Rosa, J. G. D., Pinheiro, C. G., Heinzmann, B. M., Caron, B. O., et al. (2018). Lippia alba and Aloysia triphylla essential oils are anxiolytic without inducing aversiveness in fish. Aquaculture 482, 49–56. doi: 10.1016/j.aquaculture.2017.09.023
Barata, M., Soares, F., Aragão, C., Almeida, A. C., Pousão-Ferreira, P., and Ribeiro, L. (2016). Efficiency of 2-phenoxyethanol and clove oil for reducing handling stress in reared meagre, Argyrosomus regius (Pisces: Sciaenidae). J. World Aquacult. Soc. 47, 82–92. doi: 10.1111/jwas.12245
Barbas, L. A. L., Hamoy, M., de Mello, V. J., Barbosa, R. P. M., Lima, H. S. T., Torres, M. F., et al. (2017a). Essential oil of citronella modulates electrophysiological responses in tambaqui Colossoma macropomum: a new anaesthetic for use in fish. Aquaculture 479, 60–68. doi: 10.1016/j.aquaculture.2017.05.027
Barbas, L. A. L., Maltez, L. C., Stringhetta, G. R., Garcia, L. O., Monserrat, J. M., Silva, D. T., et al. (2017b). Properties of two plant extractives as anaesthetics and antioxidants for juvenile tambaqui Colossoma macropomum. Aquaculture 469, 79–87. doi: 10.1016/j.aquaculture.2016.12.012
Barman, D., Nen, P., Mandal, S. C., and Kumar, V. (2013). Immunostimulants for aquaculture health management. J. Mar. Sci. Res. Dev. 3:134.
Batista, E. D., Brandao, F. R., Majolo, C., Inoue, L. A. K. A., Maciel, P. O., Oliveira, M. R., et al. (2018). Lippia alba essential oil as anesthetic for tambaqui. Aquaculture 495, 545–549. doi: 10.1007/s10695-017-0410-z
Becker, A. G., Cunha, M. A., Garcia, L. O., Zeppenfeld, C. C., Parodi, T. V., Maldaner, G., et al. (2013). Efficacy of eugenol and the methanolic extract of Condalia buxifolia during the transport of the silver catfish Rhamdia quelen. Neotrop. Ichthyol. 11, 675–681. doi: 10.1590/s1679-62252013000300021
Becker, A. G., Luz, R. K., Mattioli, C. C., Nakayama, C. L., Silva, W. D. E., Leme, F. D. P., et al. (2017). Can the essential oil of Aloysia triphylla have anesthetic effect and improve the physiological parameters of the carnivorous freshwater catfish Lophiosilurus alexandri after transport? Aquaculture 481, 184–190. doi: 10.1016/j.aquaculture.2017.09.007
Becker, A. G., Parodi, T. V., Heldwein, C. G., Zeppenfeld, C. C., Heinzmann, B. M., and Baldisserotto, B. (2012). Transportation of silver catfish, Rhamdia quelen, in water with eugenol and the essential oil of Lippia alba. Fish Physiol. Biochem. 38, 789–796. doi: 10.1007/s10695-011-9562-4
Becker, A. G., Parodi, T. V., Zeppenfeld, C. C., Salbego, J., Cunha, M. A., Heldwein, C. G., et al. (2016). Pre-sedation and transport of Rhamdia quelen in water containing essential oil of Lippia alba: metabolic and physiological responses. Fish Physiol. Biochem. 42, 73–81. doi: 10.1007/s10695-015-0118-x
Becker, A. J., Fogliarini, C. O., Souza, C. F., Becker, A. G., Mourão, R. H. V., Silva, L. V. F., et al. (2018). Ventilatory frequency and anesthetic efficacy in silver catfish, Rhamdia quelen: a comparative approach between different essential oils. Rev. Bras. Zootec. 47:e20170185.
Benovit, S. C., Gressler, L. T., Silva, L. D., Garcia, L. D., Okamoto, M. H., Pedron, J. D., et al. (2012). Anesthesia and transport of Brazilian flounder, Paralichthys orbignyanus, with essential oils of Aloysia gratissima and Ocimum gratissimum. J. World Aquacult. Soc. 43, 896–900. doi: 10.1111/j.1749-7345.2012.00604.x
Bianchini, A. E., Garlet, Q. I., Cunha, J. A., Bandeira Junior, G., Brusque, I. C. M., Salbego, J., et al. (2017). Monoterpenoids (thymol, carvacrol and S-(+)-linalool) with anesthetic activity in silver catfish (Rhamdia quelen): evaluation of acetylcholinesterase and GABAergic activity. Braz. J. Med. Biol. Res. 50:e6346. doi: 10.1590/1414-431X20176346
Biller, J., and Takahashi, L. S. (2018). Oxidative stress and fish immune system: phagocytosis and leukocyte respiratory burst activity. An. Acad. Bras. Ciênc. 90, 3403–3414. doi: 10.1590/0001-3765201820170730
Birnie-Gauvin, K., Costantini, D., Cooke, S. J., and Willmore, W. G. (2017). A comparative and evolutionary approach to oxidative stress in fish: a review. Fish Fish. 18, 928–942. doi: 10.1111/faf.12215
Bodur, T., Afonso, J. M., Montero, D., and Navarro, A. (2018a). Assessment of effective dose of new herbal anesthetics in two marine aquaculture species: dicentrarchus labrax and Argyrosomus regius. Aquaculture 482, 78–82. doi: 10.1016/j.aquaculture.2017.09.029
Bodur, T., León-Bernabeu, S., Navarro, A., Tort, L., Afonso, J. M., and Montero, D. (2018b). Effects of new plant based anesthetics Origanum sp. and Eucalyptus sp. oils on stress and welfare parameters in Dicentrarchus labrax and their comparison with clove oil. Aquaculture 495, 402–408. doi: 10.1016/j.aquaculture.2018.06.021
Bolasina, S. N. (2006). Cortisol and hematological response in Brazilian codling, Urophycis brasiliensis (Pisces, Phycidae) subjected to anesthetic treatment. Aquacult. Int. 14, 569–575. doi: 10.1007/s10499-006-9055-0
Bressler, K., and Ron, B. (2004). Effect of anesthetics on stress and the innate immune system of gilthead seabream (Sparus aurata). Isr. J. Aquac. Bamid. 56, 5–13.
Brown, J. A., Watson, J., Bourhill, A., and Wall, T. (2008). Evaluation and use of the Lactate Pro, a portable lactate meter, in monitoring the physiological well-being of farmed Atlantic cod (Gadus morhua). Aquaculture 285, 135–140. doi: 10.1016/j.aquaculture.2008.08.027
Brum, A., Pereira, S. A., Owatari, M. S., Chagas, E. C., Chaves, F. C. M., Mourino, J. L. P., et al. (2017). Effect of dietary essential oils of clove basil and ginger on Nile tilapia (Oreochromis niloticus) following challenge with Streptococcus agalactiae. Aquaculture 468, 235–243. doi: 10.1016/j.aquaculture.2016.10.020
Bulfon, C., Volpatti, D., and Galeotti, M. (2015). Current research on the use of plant-derived products in farmed fish. Aquac. Res. 46, 513–551. doi: 10.1111/are.12238
Capuzzo, A., Maffei, M. E., and Occhipinti, A. (2013). Supercritical fluid extraction of plant flavors and fragrances. Molecules 18, 7194–7238. doi: 10.3390/molecules18067194
Cárdenas, C., Toni, C., Martos-Sitcha, J. A., Cardenas, S., de las Heras, V., Baldisserotto, B., et al. (2016). Effects of clove oil, essential oil of Lippia alba and 2-phe anaesthesia on juvenile meagre, Argyrosomus regius (Asso, 1801). J. Appl. Ichthyol. 32, 693–700. doi: 10.1111/jai.13048
Chioca, L. R., Ferro, M. M., Baretta, I. P., Oliveira, S. M., Silva, C. R., Ferreira, J., et al. (2013). Anxiolytic-like effect of lavender essential oil inhalation in mice: participation of serotonergic but not GABAA/benzodiazepine neurotransmission. J. Ethnopharmacol. 147, 412–418. doi: 10.1016/j.jep.2013.03.028
Cole, R., Alcock, N., Handley, S., Grange, K., Black, S., Cairney, D., et al. (2003). Selective capture of blue cod (Parapercis colias) by potting: behavioural observations and effects of capture method on peri-mortem fatigue. Fish. Res. 60, 381–392. doi: 10.1016/s0165-7836(02)00133-9
Cunha, J. A., Heinzmann, B. M., and Baldisserotto, B. (2018). The effects of essential oils and their major compounds on fish bacterial pathogens – a review. J. Appl. Microbiol. 125, 328–344. doi: 10.1111/jam.13911
Cunha, J. A., Scheeren, C. A., Salbego, J., Gressler, L. T., Madaloz, L. M., Bandeira Junior, G., et al. (2017). Essential oils of Cunila galioides and Origanum majorana as anesthetics for Rhamdia quelen: efficacy and effects on ventilation and ionoregulation. Neotrop. Ichthyol. 15:e160076.
Cunha, M. A., Barros, F. M. C., Garcia, L. O., Veeck, A. P. D., Heinzmann, B. M., Loro, V. L., et al. (2010). Essential oil of Lippia alba: a new anesthetic for silver catfish, Rhamdia quelen. Aquaculture 306, 403–406. doi: 10.1016/j.aquaculture.2010.06.014
Cunha, M. A., Silva, B. F., Delunardo, F. A. C., Benovit, S. C., Gomes, L. C., Heinzmann, B. M., et al. (2011). Anesthetic induction and recovery of Hippocampus reidi exposed to the essential oil of Lippia alba. Neotrop. Ichthyol. 9, 683–688. doi: 10.1590/s1679-62252011000300022
Du, F., Xu, G., Gao, J., Nie, Z., Xu, P., and Gu, R. (2015). Transport-induced changes in hypothalamic-pituitary-interrenal axis gene expression and oxidative stress responses in Coilia nasus. Aquac. Res. 47, 3599–3606.
Edris, A. E. (2007). Pharmaceutical and therapeutic potentials of essential oils and their individual volatile constituents: a review. Phytother. Res. 21, 308–323. doi: 10.1002/ptr.2072
Evans, P., and Halliwell, B. (1999). Free radicals and hearing: cause, consequence, and criteria. Ann. N. Y. Acad. Sci. 884, 19–40. doi: 10.1111/j.1749-6632.1999.tb08633.x
Figueiredo, A. C., Barroso, J. G., Pedro, L. G., Johannes, J. C., and Scheffer, J. J. C. (2008). Factors affecting secondary metabolite production in plants: volatile components and essential oils Factors affecting volatile and essential oil production in plants. Flavour Frag. J. 23, 213–226. doi: 10.1002/ffj.1875
Flik, G., Peter, H. M., Klaren, P. H. M., Erwin, H., Van den Burg, J. R., and Huising, M. O. (2006). CRF and stress in fish. Gen. Comp. Endocrinol. 146, 36–44. doi: 10.1016/j.ygcen.2005.11.005
Fogliarini, C. O., Garlet, Q. I., Parodi, T. V., Becker, A. G., Garcia, L. O., Heinzmann, B. M., et al. (2017). Anesthesia of Epinephelus marginatus with essential oil of Aloysia polystachya: an approach on blood parameters. An. Acad. Bras. Cienc. 89, 445–456. doi: 10.1590/0001-3765201720160457
Garcia, L. O., Barcellos, L. J. G., and Baldisserotto, B. (2015). Net ion fluxes and ammonia excretion during transport of Rhamdia quelen juveniles. Cienc. Rural 45, 1854–1858. doi: 10.1590/0103-8478cr20141420
Garlet, Q. I., Pires, L. C., Silva, D. T., Spall, S., Gressler, L. T., Bürger, M. E., et al. (2016). Effect of (+)-dehydrofukinone on GABA(A) receptors and stress response in fish model. Braz. J. Med. Biol. Res. 49:e4872. doi: 10.1590/1414-431X20154872
Gholipourkanani, H., Gholinasab-Omran, I., Ebrahimi, P., and Jafaryan, H. (2015). Anesthetic effect of clove oil loaded on lecithin based nano emulsions in gold fish, Carassius auratus. J. Fish. Aquatic Sci. 10, 553–561. doi: 10.3923/jfas.2015.553.561
Golombieski, J. I., Silva, L. V. F., Baldisserotto, B., and Silva, J. H. S. (2003). Transport of silver catfish, (Rhamdia quelen) fingerlings at different times, load densities, and temperatures. Aquaculture 216, 95–102. doi: 10.1016/s0044-8486(02)00256-9
Gressler, L. T., Riffel, A. P. K., Parodi, T. V., Saccol, E. M. H., Koakoski, G., Costa, S. T., et al. (2014). Silver catfish Rhamdia quelen immersion anaesthesia with essential oil of Aloysia triphylla (L’Herit) Britton or tricaine methanesulfonate: effect on stress response and antioxidant status. Aquacult. Res. 45, 1061–1072. doi: 10.1111/are.12043
Halliwell, B., and Gutteridge, J. M. C. (2007). Free Radicals in Biology and Medicine. Oxford: Clarendon.
Harmon, T. S. (2009). Methods for reducing stressors and maintaining water quality associated with live fish transport in tanks: a review of the basics. Rev. Aquac. 1, 58–66. doi: 10.1111/j.1753-5131.2008.01003.x
Hashimoto, G. S. O., Neto, F. M., Ruiz, M. L., Acchile, M., Chagas, E. C., Chaves, F. C. M., et al. (2016). Essential oils of Lippia sidoides and Mentha piperita against monogenean parasites and their influence on the hematology of Nile tilapia. Aquaculture 450, 182–186. doi: 10.1016/j.aquaculture.2015.07.029
Heldwein, C. G., Silva, L. L., Gai, E. Z., Roman, C., Parodi, T. V., Burger, M. E., et al. (2014). S-(+)-Linalool from Lippia alba: sedative and anesthetic for silver catfish (Rhamdia quelen). Vet. Anaesth. Analg. 41, 621–629. doi: 10.1111/vaa.12146
Heldwein, C. G., Silva, L. L., Reckziegel, P., Barros, F. M., Burger, M. E., Baldisserotto, B., et al. (2012). Participation of the GABAergic system in the anesthetic effect of Lippia alba (Mill.) N.E. Brown essential oil. Braz. J. Med. Biol. Res. 45, 436–443. doi: 10.1590/s0100-879x2012007500052
Hoseini, S. M. (2011). Efficacy of clove powder solution on stress mitigation in juvenile common carps, Cyprinus carpio (Linnaeus). Comp. Clin. Path. 20, 359–362. doi: 10.1007/s00580-010-1002-9
Hoseini, S. M., Mirghaed, A. T., and Yousefi, M. (2018). Application of herbal anaesthetics in aquaculture. Rev. Aquac. 0, 1–15. doi: 10.1111/raq.12245
Hoseini, S. M., and Nodeh, A. (2013). Changes in blood biochemistry of common carp Cyprinus carpio (Linnaeus), following exposure to different concentrations of clove solution. Comp. Clin. Pathol. 22, 9–13. doi: 10.1007/s00580-011-1362-9
Hussain, A. I., Anwar, F., Sherazi, S. T. H., and Przybylski, R. (2008). Chemical composition, antioxidant and antimicrobial activities of basil (Ocimum basilicum) essential oils depends on seasonal variations. Food Chem. 108, 986–995. doi: 10.1016/j.foodchem.2007.12.010
Javahery, S., Nekoubin, H., and Moradlu, A. H. (2012). Effect of anaesthesia with clove oil in fish (review). Fish Physiol. Biochem. 38, 1545–1552. doi: 10.1007/s10695-012-9682-5
Jerez-Cepa, I., Fernández-Castro, M., Del Santo, T. J., Martos-Sitcha, J. A., Martínez-Rodríguez, G., Mancera, J. M., et al. (2019). Transport and recovery of gilthead seabream (Sparus aurata L.) sedated with clove oil and MS-222: effects on stress axis regulation and intermediary metabolism. Frontiers Physiol 10:612. doi: 10.3389/fphys.2019.00612
Lim, L. C., Dhert, P., and Sorgeloos, P. (2003). Recent developments and improvements in ornamental fish packaging systems for air transport. Aquac. Res. 34, 923–935. doi: 10.1046/j.1365-2109.2003.00946.x
Lopes, J. M., Souza, C. F., Schindler, B., Pinheiro, C. G., Salbego, J., Siqueira, J. C., et al. (2018). Essential oils from Citrus x aurantium and Citrus x latifolia (Rutaceae) have anesthetic activity and are effective in reducing ion loss in silver catfish (Rhamdia quelen). Neotrop. Ichthyol. 16:e170152.
Luque de Castro, M. D., Jiménez-Carmona, M. M., and Fernández-Pérez, V. (1999). Towards more rational techniques for the isolation of valuable essential oils from plants. TRAC Trend. Anal. Chem. 18, 708–716. doi: 10.1016/s0165-9936(99)00177-6
Manayi, A., Nabavi, S. M., Daglia, M., and Jafari, S. (2016). Natural terpenoids as a promising source for modulation of GABAergic system and treatment of neurological diseases. Pharmacol. Rep. 68, 671–679. doi: 10.1016/j.pharep.2016.03.014
Martos-Sitcha, J. A., Wunderink, Y. S., Straatjes, J., Skrzynska, A. K., Mancera, J. M., and Martinez-Rodriguez, G. (2014). Different stressors induce differential responses of the CRH-stress system in the gilthead sea bream (Sparus aurata). Comp. Biochem. Physiol. A Mol. Integr. Physiol. 177, 49–61. doi: 10.1016/j.cbpa.2014.07.021
Mazandarani, M., Hoseini, S. M., and Ghomshani, M. D. (2017). Effects of linalool on physiological responses of Cyprinus carpio (Linnaeus, 1758) and water physico-chemical parameters during transportation. Aquac. Res. 48, 5775–5781. doi: 10.1111/are.13400
Mirghaed, A. T., Yasari, M., Mirzargar, S. S., and Hoseini, S. M. (2018). Rainbow trout (Oncorhynchus mykiss) anesthesia with myrcene: efficacy and physiological responses in comparison with eugenol. Fish Physiol. Biochem. 44, 919–926. doi: 10.1007/s10695-018-0481-5
Morais, L. A. S. (2009). Influence of abiotic factors on the chemical composition of essential oils. Hortic. Bras. 27, 4050–4063.
Moreira, M. R. C., Salvadori, M. G., De Almeida, A. A. C., De Sousa, D. P., Jordán, J., Satyal, P., et al. (2014). Anxiolytic-like effects and mechanism of (-)-myrtenol: a monoterpene alcohol. Neurosci. Lett. 579, 119–124. doi: 10.1016/j.neulet.2014.07.007
Morel, Y., and Barouki, R. (1999). Repression of gene expression by oxidative stress. Biochem. J. 342, 481–496. doi: 10.1042/bj3420481
Navarro, R. D., França, R. P., Paludo, G. R., Bizarro, Y. W. S., da Silva, R. F., and Pereira, F. K. S. (2016). Physiological and hematological responses of Nile tilapia (Oreochromis Niloticus) to different anesthetics during simulated transport conditions. Acta Sci. Technol. (Online) 38, 301–306. doi: 10.4025/actascitechnol.v38i3.28377
Neiffer, D. L., and Stamper, M. A. (2009). Fish sedation, analgesia, anesthesia, and euthanasia: considerations, methods, and types of drugs. ILAR J. 50, 343–360. doi: 10.1093/ilar.50.4.343
Olsen, S. H., Tobiassen, T., Akse, L., Evensen, T. H., and Midling, K. Ø. (2013). Capture induced stress and live storage of Atlantic cod (Gadus morhua) caught by trawl: consequences for the flesh quality. Fish. Res 147, 446–453. doi: 10.1016/j.fishres.2013.03.009
Ostrensky, A., Dal Pont, G., Westphal, G. G. C., and Pedrazzani, A. S. (2016). Use of clove, mint and camphor essential oils on confinement of clown anemonefish Amphiprion ocellaris (Cuvier 1830): anesthetic effects and influence on water quality. J. Aquac. Res. Dev. 7:457. doi: 10.4172/2155-9546.1000457
Pankhurst, N. M., and Van der Kraak, G. (1997). “Effects of stress on reproduction and growth of fish,” in Fish Stress and Health in Aquaculture, eds G. K. Iwama, A. D. Pickering, J. P. Sumpter, and C. B. Schreck (London: Cambridge University Press), 73–93.
Parodi, T. V., Cunha, M. A., Becker, A. G., Zeppenfeld, C. C., Martins, D. I., Koakoski, G., et al. (2014). Anesthetic activity of Aloysia triphylla and effectiveness in reducing stress during transport of albine and grey strains of silver catfish, Rhamdia quelen. Fish Physiol. Biochem. 40, 323–334. doi: 10.1007/s10695-013-9845-z
Parodi, T. V., Santos, C. A., Veronez, A., Gomes, L. C., Heinzmann, B. M., and Baldisserotto, B. (2016). Anesthetic induction and recovery time of Centropomus parallelus exposed to the essential oil of Aloysia triphylla. Cienc. Rural 46, 2142–2147. doi: 10.1590/0103-8478cr20160039
Paterson, B. D., Rimmer, M. A., Meikle, G. M., and Semmens, G. L. (2003). Physiological responses of the Asian sea bass, Lates calcarifer to water quality deterioration during simulated live transport: acidosis, red-cell swelling, and levels of ions and ammonia in the plasma. Aquaculture 218, 717–728. doi: 10.1093/dnares/dst022
Pedrazzani, A. S., and Ostrenski Neto, A. (2016). The anaesthetic effect of camphor (Cinnamomum camphora), clove (Syzygium aromaticum) and mint (Mentha arvensis) essential oils on clown anemonefish Amphiprion ocellaris (Cuvier 1830). Aquac. Res. 47, 769–776. doi: 10.1111/are.12535
Pottinger, T. G. (2008). “The stress response in fish: mechanisms, effects and measurement,” in Fish Welfare, ed. E. Branson (Oxford: Blackwell), 32–48. doi: 10.1002/9780470697610.ch3
Priborsky, J., and Velisek, J. (2018). A review of three commonly used fish anesthetics. Rev. Fish. Sci. Aquac. 26, 417–442. doi: 10.1080/23308249.2018.1442812
Raut, J. S., and Karuppayil, S. M. (2015). A status review on the medicinal properties of essential oils. Ind. Crops Prod. 62, 250–264. doi: 10.1016/j.indcrop.2014.05.055
Readman, G. D., Owen, S. F., Murrell, J. C., and Knowles, T. G. (2013). Do fish perceive anaesthetics as aversive? PLos One 8:e73773. doi: 10.1371/journal.pone.0073773
Reyes-Jurado, F., Franco-Veja, A., Ramírez-Corona, N., Palou, E., and López-Malo, A. (2015). Essential oils: antimicrobial activities extraction methods, and their modeling. Food Eng. Rev. 7, 275–297. doi: 10.1007/s12393-014-9099-2
Ribas, L., Flos, R., Reig, L., Mackenzie, S., Barton, B. A., and Tort, L. (2007). Comparison of methods for anaesthetizing Senegal sole (Solea senegalensis) before slaughter: stress responses and final product quality. Aquaculture 269, 250–258. doi: 10.1016/j.aquaculture.2007.05.036
Ribeiro, A. S., Batista, E. S., Dairiki, J. K., Chaves, F. C. M., and Inoue, L. A. (2016). Anesthetic properties of Ocimum gratissimum essential oil for juvenile matrinxã. Acta Sci. Anim. Sci. 38, 1–7.
Roohi, Z., and Imanpoor, M. R. (2015). The efficacy of the oils of spearmint and methyl salicylate as new anesthetics and their effect on glucose levels in common carp (Cyprinus carpio L., 1758) juveniles. Aquaculture 43, 327–332. doi: 10.1016/j.aquaculture.2014.12.019
Ross, L. G., and Ross, B. (2008). Anaesthetic and Sedative Techniques for Aquatic Animals. London: Blackwell Publishing Ltd.
Saccol, E. M. H., Jerez-Cepa, I., Ourique, G. M., Pês, T. S., Gressler, L. T., Mourão, R. H. V., et al. (2018a). Myrcia sylvatica essential oil mitigates molecular, biochemical and physiological alterations in Rhamdia quelen under different stress events associated to transport. Res. Vet. Sci. 117, 150–160. doi: 10.1016/j.rvsc.2017.12.009
Saccol, E. M. H., Londero, E. P., Bressan, C. A., Salbego, J., Gressler, L. T., Silva, L. V. F., et al. (2017). Oxidative and biochemical responses in Brycon amazonicus anesthetized and sedated with Myrcia sylvatica (G. Mey.) DC. and Curcuma longa L. essential oils. Vet. Anaesth. Analg. 44, 555–566. doi: 10.1016/j.vaa.2016.08.005
Saccol, E. M. H., Parrado-Sanabria, Y. A., Gagliardi, L., Jerez-Cepa, I., Mourão, R. H. V., Heinzmann, B. M., et al. (2018b). Myrcia sylvatica essential oil in the diet of gilthead sea bream (Sparus aurata L.) attenuates the stress response induced by high stocking density. Aquac. Nutr. 24, 1381–1392. doi: 10.1111/anu.12675
Saccol, E. M. H., Toni, C., Pês, T. S., Ourique, G. M., Gressler, L. T., Silva, V. F., et al. (2016). Anaesthetic and antioxidant effects of Myrcia sylvatica (G. Mey.) DC. and Curcuma longa L. essential oils on tambaqui (Colossoma macropomum). Aquac. Res. 48, 2012–2031.
Saccol, E. M. H., Uczay, J., Pês, T. S., Finamor, I. A., Ourique, G. M., Riffel, A. P. K., et al. (2013). Addition of Lippia alba (Mill) N. E. Brown essential oil to the diet of the silver catfish: an analysis of growth, metabolic and blood parameters and the antioxidant response. Aquaculture 416, 244–254. doi: 10.1016/j.aquaculture.2013.09.036
Sakai, M. (1999). Current research status of fish immunostimulants. Aquaculture 172, 63–92. doi: 10.1016/s0044-8486(98)00436-0
Salbego, J., Becker, A. G., Gonçalves, J. F., Menezes, C. C., Heldwein, C. G., Spanevello, R. M., et al. (2014). The essential oil from Lippia alba induces biochemical stress in the silver catfish (Rhamdia quelen) after transportation. Neotrop. Ichthyol. 12, 811–818. doi: 10.1590/1982-0224-20130178
Salbego, J., Toni, C., Becker, A. G., Zeppenfeld, C. C., Menezes, C. C., Loro, V. L., et al. (2017). Biochemical parameters of silver catfish (Rhamdia quelen) after transport with eugenol or essential oil of Lippia alba added to the water. Braz. J. Biol. 77, 696–702. doi: 10.1590/1519-6984.16515
Sampaio, F. D., and Freire, C. A. (2016). An overview of stress physiology of fish transport: changes in water quality as a function of transport duration. Fish Fish. 17, 1055–1072. doi: 10.1111/faf.12158
Sánchez-Muros, M. J., Sánchez, B., Barroso, F. G., Toniolo, M., Trenzado, C. E., and Rus, A. S. (2017). Effects of rearing conditions on behavioural responses, social kinetics and physiological parameters in gilthead sea bream Sparus aurata. Appl. Anim. Behav. Sci. 197, 120–128. doi: 10.1016/j.applanim.2017.08.004
Santos, A. C., Bandeira Junior, G., Zago, D. C., Zeppenfeld, C. C., Silva, D. T., Heinzmann, B. M., et al. (2017a). Anesthesia and anesthetic action mechanism of essential oils of Aloysia triphylla and Cymbopogon flexuosus in silver catfish (Rhamdia quelen). Vet. Anaest. Analg. 44, 106–113. doi: 10.1111/vaa.12386
Santos, A. C., Sutili, F. J., Heinzmann, B. M., Cunha, M. A., Brusque, I. C. M., Baldisserotto, B., et al. (2017b). Aloysia triphylla essential oil as additive in silver catfish diet: blood response and resistance against Aeromonas hydrophila infection. Fish Shellfish Immun. 62, 213–216. doi: 10.1016/j.fsi.2017.01.032
Santos, W. M., Brito, T. S., Prado, S. A., Oliveira, C. G., Paula, A. C., Melo, D. C., et al. (2016). Cinnamon (Cinnamomum sp.) inclusion in diets for Nile tilapia submitted to acute hypoxic stress. Fish Shellfish Immun. 54, 551–555. doi: 10.1016/j.fsi.2016.04.135
Schreck, C. B., and Tort, L. (2016). “The concept of stress in fish,” in Biology of Stress in Fish - Fish Physiology, Vol. 35, eds C. B. Schreck, L. Tort, A. P. Farrell, and C. J. Brauner (Cambridge, MA: Academic Press) 1–34. doi: 10.1371/journal.pone.0106523
Segner, H., Sundh, H., Buchmann, K., Douxfils, J., Sundell, K. S., Mathieu, C., et al. (2012). Health of farmed fish: its relation to fish welfare and its utility as welfare indicator. Fish Physiol. Biochem. 38, 85–105. doi: 10.1007/s10695-011-9517-9
Sena, A. C., Teixeira, R. R., Ferreira, E. L., Heinzmann, B. M., Baldisserotto, B., Caron, B. O., et al. (2016). Essential oil from Lippia alba has anaesthetic activity and is effective in reducing handling and transport stress in tambacu (Piaractus mesopotamicus x Colossoma macropomum). Aquaculture 465, 374–379. doi: 10.1016/j.aquaculture.2016.09.033
Silva, L. L., Garlet, Q. I., Koakoski, G., Abreu, M. S., Mallmann, C. A., Baldisserotto, B., et al. (2015). Anesthetic activity of the essential oil of Ocimum americanum in Rhamdia quelen (Quoy & Gaimard, 1824) and its effects on stress parameters. Neotrop. Ichthyol. 13, 715–722. doi: 10.1590/1982-0224-20150012
Silva, L. L., Parodi, T. V., Reckziegel, P., Garcia, V. O., Bürger, M. E., Baldisserotto, B., et al. (2012). Essential oil of Ocimum gratissimum L.: anesthetic effects, mechanism of action and tolerance in silver catfish, Rhamdia quelen. Aquaculture 35, 91–97. doi: 10.1016/j.aquaculture.2012.04.012
Silva, L. L., Silva, D. T., Garlet, Q. I., Cunha, M. A., Mallmann, C. A., Baldisserotto, B., et al. (2013). Anesthetic activity of Brazilian native plants in silver catfish (Rhamdia quelen). Neotrop. Ichthyol. 11, 443–451. doi: 10.1590/s1679-62252013000200014
Skrzynska, A. K., Maiorano, E., Bastaroli, M., Naderi, F., Miguez, J. M., Martinez-Rodriguez, G., et al. (2018). Impact of air exposure on vasotocinergic and isotocinergic systems in gilthead sea bream (Sparus aurata): new insights on fish stress response. Front. Physiol. 9:96. doi: 10.3389/fphys.2018.00096
Sladky, K. K., Swanson, C. R., Stoskopf, M. K., Loomis, M. R., and Lewbart, G. A. (2001). Comparative efficacy of tricaine methanesulfonate and clove oil for use as anesthetics in red pacu (Piaractus brachypomus). Am. J. Vet. Res. 62, 337–342. doi: 10.2460/ajvr.2001.62.337
Sneddon, L., Wolfenden, D., and Thomson, J. (2016). “Stress management and welfare,” in Fish Physiology: Biology of Stress in Fish, Vol. 35, eds C. B. Schreck, L. Tort, A. P. Farrell, and C. J. Brauner (Cambridge, MA: Academic Press), 463–539. doi: 10.2460/ajvr.2001.62.337
Soltani, M., Sheikhzadeh, N., Mousavi, H. A. E., and Zargar, A. (2010). Effects of Zataria multiflora essential oil on innate immune responses of common carp (Cyprinus carpio). J. Fish. Aquat. Sci. 5, 191–199. doi: 10.3923/jfas.2010.191.199
Soltanian, S., Hoseinifar, S. H., and Gholamhosseini, A. (2018). Modulation of rainbow trout (Oncorhynchus mykiss) cutaneous mucosal immune responses following anesthesia: a comparative study on different anesthetic agents. Fish Shellfish Immun. 80, 319–324. doi: 10.1016/j.fsi.2018.06.032
Sönmez, A. Y., Bilen, S., Alak, G., Hisar, O., Yanik, T., and Biswas, G. (2015). Growth performance and antioxidant enzyme activities in rainbow trout (Oncorhynchus mykiss) juveniles fed diets supplemented with sage, mint and thyme oils. Fish Physiol. Biochem. 41, 165–175. doi: 10.1007/s10695-014-0014-9
Souza, C. F., Baldissera, M. D., Bianchini, A. E., Silva, E. G., Mourão, R. H. V., Silva, L. V. F., et al. (2018a). Citral and linalool chemotypes of Lippia alba essential oil as anesthetics for fish: a detailed physiological analysis of side effects during anesthetic recovery in silver catfish (Rhamdia quelen). Fish Physiol. Biochem. 44, 21–34. doi: 10.1007/s10695-017-0410-z
Souza, C. F., Baldissera, M. D., Guarda, N. S., Bollick, Y. S., Moresco, R. N., Brusque, I. C. M., et al. (2017a). Melaleuca alternifolia essential oil nanoparticles ameliorate the hepatic antioxidant/oxidant status of silver catfish experimentally infected with Pseudomonas aeruginosa. Microb. Pathog. 108, 61–65. doi: 10.1016/j.micpath.2017.05.016
Souza, C. F., Baldissera, M. D., Salbego, J., Lopes, J., Vaucher, R. A., Mourão, R., et al. (2017b). Physiological responses of silver catfish to anesthesia with essential oils from two different chemotypes of Lippia alba. Neotrop. Ichthyol. 15:e160083. doi: 10.1590/1982-0224-20160083
Souza, C. F., Baldissera, M. D., Santos, R. C. V., Raffin, R. P., and Baldisserotto, B. (2017c). Nanotechnology improves the therapeutic efficacy of Melaleuca alternifolia essential oil in experimentally infected Rhamdia quelen with Pseudomonas aeruginosa. Aquaculture 473, 169–171. doi: 10.1016/j.aquaculture.2017.02.014
Souza, C. F., Baldissera, M. D., Silva, L. L., Geihs, M. A., and Baldisserotto, B. (2018b). Is monoterpene terpinen-4-ol the compound responsible for the anesthetic and antioxidant activity of Melaleuca alternifolia essential oil (tea tree oil) in silver catfish? Aquaculture 486, 217–223. doi: 10.1016/j.aquaculture.2017.12.025
Souza, C. F., Descovi, S., Baldissera, M. D., Bertolin, K., Bianchini, A. E., Mourão, R. H. V., et al. (2019). Involvement of HPI-axis in anesthesia with Lippia alba essential oil citral and linalool chemotypes: gene expression in the secondary responses in silver catfish. Fish Physiol. Biochem. 45, 155–166. doi: 10.1007/s10695-018-0548-3
Souza, C. F., Salbego, J., Gressler, L. T., Golombieski, J. I., Ferst, J. G., Cunha, M. A., et al. (2015). Silver catfish, Rhamdia quelen (Quoy & Gaimard, 1824), submitted to a stressful condition: effect of dietary addition of the essential oil of Lippia alba (Mill.) N. E. Brown on metabolism, osmoregulation and endocrinology. Neotrop. Ichthyol. 13, 707–714.
Sticher, O. (2015). “Ätherische Öle und Drogen, die ätherisches Öl enthalten,” in Sticker Pharmacognosie Phytopharmazie, eds O. Sticher, J. Heilmann, I. Zúndorf, and I. Hänsel (Stuttgart: Wissenschaftliche Verlagsgesellschaft), 663–740.
Sutili, F. J., Gatlin, D. M., Heinzmann, B. M., and Baldisserotto, B. (2018). Plant essential oils as fish diet additives: benefits on fish health and stability in feed. Rev. Aquac. 10, 716–726. doi: 10.1111/raq.12197
Sutili, F. J., Kreutz, L. C., Noro, M., Gressler, L. T., Heinzmann, B. M., de Vargas, A. C., et al. (2014). The use of eugenol against Aeromonas hydrophila and its effect on hematological and immunological parameters in silver catfish (Rhamdia quelen). Vet. Immunol. Immunopathol. 157, 142–148. doi: 10.1016/j.vetimm.2013.11.009
Sutili, F. J., Silva, L. L., Gressler, L. T., Battisti, E. K., Heinzmann, B. M., Vargas, A. P. C., et al. (2015). Plant essential oils against Aeromonas hydrophila in vitro activity and their use in experimentally infected fish. J. Appl. Microbiol. 119, 47–54. doi: 10.1111/jam.12812
Swamy, M. K., Akhtar, M. S., and Sinniah, U. R. (2016). Antimicrobial properties of plant essential oils against human pathogens and their mode of action: an updated review. Evid Based Complement. Alternat. Med. 2016:3012462. doi: 10.1155/2016/3012462
Teixeira, R. R., Souza, R. C., Sena, A. C., Baldisserotto, B., Heinzmann, B. M., and Copatti, C. E. (2018). Essential oil of Aloysia triphylla is effective in Nile tilapia transport. Bol. Inst. Pesca 44, 17–24. doi: 10.20950/1678-2305.2018.263
Teixeira, R. R., Souza, R. C., Sena, A. C., Baldisserotto, B., Heinzmann, B. M., Couto, R. D., et al. (2017). Essential oil of Aloysia triphylla in Nile tilapia: anaesthesia, stress parameters and sensory evaluation of fillets. Aquac. Res. 48, 3383–3392. doi: 10.1111/are.13165
Teles, M., Oliveira, M., Jerez-Cepa, I., Franco-Martínez, L., Tvarijonaviciute, A., Tort, L., et al. (2019). Transport and recovery of gilthead sea bream (Sparus aurata L.) sedated with clove oil and ms222: effects on oxidative stress status. Front. Physiol. 10:523. doi: 10.3389/fphys.2019.00523
Thomas, P., and Robertson, L. (1991). Plasma cortisol and glucose stress responses of red drum (Sciaenops ocellatus) to handling and shallow water stressors and anesthesia with MS-222, quinaldine sulfate and metomidate. Aquaculture 1, 69–86. doi: 10.1016/0044-8486(91)90140-3
Tondolo, J. S. M., Amaral, L. P., Simões, L. N., Garlet, Q. I., Schindler, B., Oliveira, T. M., et al. (2013). Anesthesia and transport of fat snook Centropomus parallelus with the essential oil of Nectandra megapotamica (Spreng.) Mez. Neotrop. Ichthyol. 11, 667–674. doi: 10.1590/s1679-62252013000300020
Toni, C., Becker, A. G., Simões, L. N., Pinheiro, C. G., Silva, L. L., Heinzmann, B. M., et al. (2014). Fish anesthesia: effects of the essential oils of Hesperozygis ringens and Lippia alba on the biochemistry and physiology of silver catfish (Rhamdia quelen). Fish Physiol Biochem 40, 701–714. doi: 10.1007/s10695-013-9877-4
Toni, C., Martos-Sitcha, J. A., Baldisserotto, B., Heinzmann, B. M., Silva, L. L., Martínez-Rodríguez, G., et al. (2015). Sedative effect of 2-phenoxyethanol and essential oil of Lippia alba on stress response in gilthead sea bream (Sparus aurata). Res. Vet. Sci. 103, 20–27. doi: 10.1016/j.rvsc.2015.09.006
Tort, L. (2011). Stress and immune modulation in fish. Dev. Comp. Immunol. 35, 1366–1375. doi: 10.1016/j.dci.2011.07.002
Tort, L., Balasch, J. C., and Mackenzie, S. (2003). Fish immune system. A crossroads between innate and adaptive responses. Inmunología 22, 277–286.
Tort, L., Puigcerver, M., Crespo, S., and Padros, F. (2002). Cortisol and haematological response in sea bream and trout subjected to the anesthetics clove oil and 2- phenoxyethanol. Aquac. Res. 33, 907–910. doi: 10.1046/j.1365-2109.2002.00741.x
Tu, W. Y., Pohl, S., Summpunn, P., Hering, S., Kerstan, S., and Harwood, C. R. (2012). Comparative analysis of the responses of related pathogenic and environmental bacteria to oxidative stress. Microbiology 158, 636–647. doi: 10.1099/mic.0.057000-0
Turek, C., and Stintzing, F. C. (2013). Stability of essential oils: a review. Compr. Rev. Food Sci. Food Saf. 12, 40–53. doi: 10.1111/1541-4337.12006
Vanderzwalmen, M., Eaton, L., Mullen, C., Henriquez, F., Carey, P., Snellgrove, D., et al. (2018). The use of feed and water additives for live fish transport. Rev. Aquac. 11, 263–278. doi: 10.1111/raq.12239
Velisek, J., Stejskal, V., Kouril, J., and Svobodova, Z. (2011). Comparison of the effects of four anaesthetics on biochemical blood profiles of perch. Aquac. Res. 40, 354–361. doi: 10.1111/j.1365-2109.2008.02102.x
Wagner, E., Arndt, R., and Hilton, B. (2002). Physiological stress responses, egg survival and sperm motility for rainbow trout broodstock anaesthetized with clove oil, tricaine methanesulfonate or carbon dioxide. Aquaculture 211, 353–366. doi: 10.1016/s0044-8486(01)00878-x
Wendelaar Bonga, S. E. (2011). “Hormonal responses to stress,” in Encyclopedia of Fish Physiology, ed. P. F. Anthony (San Diego, CA: Academic Press), 1515–1523. doi: 10.1016/b978-0-12-374553-8.00183-0
Zago, D. C., Santos, A. C., Lanes, C. F. C., Almeida, D. V., Koakoski, G., Abreu, M. S., et al. (2018). Aloysia triphylla in the zebrafish food: effects on physiology, behavior, and growth performance. Fish Physiol. Biochem. 44, 465–474. doi: 10.1007/s10695-017-0446-0
Zahl, I. H., Kiessling, A., Samuelsen, O. B., and Olsen, R. E. (2010). Anaesthesia induces stress in Atlantic salmon (Salmo salar), Atlantic cod (Gadus morhua) and Atlantic halibut (Hippoglossus hippoglossus). Fish Physiol. Biochem. 36, 719–730. doi: 10.1007/s10695-009-9346-2
Zahl, I. H., Samuelsen, O., and Kiessling, A. (2012). Anaesthesia of farmed fish: implications for welfare. Fish Physiol. Biochem. 38, 201–218. doi: 10.1007/s10695-011-9565-1
Zeppenfeld, C. C., Saccol, E. M. H., Pês, T. S., Salbego, J., Koakoski, G., Santos, A. C., et al. (2017). Aloysia triphylla essential oil as food additive for Rhamdia quelen - Stress and antioxidant parameters. Aquac. Nutr. 23, 1362–1367. doi: 10.1111/anu.12511
Zeppenfeld, C. C., Toni, C., Becker, A. G., Miron, D. S., Parodi, T. V., Heinzmann, B. M., et al. (2014). Physiological and biochemical responses of silver catfish, Rhamdia quelen, after transport in water with essential oil of Aloysia triphylla (L’Herit) Britton. Aquaculture 418-419, 101–107. doi: 10.1016/j.aquaculture.2013.10.013
Zhang, Y., Wang, Q. C., Yu, H., Zhu, J., Lange, K., Yin, Y., et al. (2016). Evaluation of alginate-whey protein microcapsules for intestinal delivery of lipophilic compounds in pigs. J. Sci. Food Agric. 96, 2674–2681. doi: 10.1002/jsfa.7385
Zheng, Z. L., Tan, J. Y. W., Liu, H. Y., Zhou, X. H., Xiang, X., and Wang, K. Y. (2009). Evaluation of oregano essential oil (Origanum heracleoticum L.) on growth, antioxidant effect and resistance against Aeromonas hydrophila in channel catfish (Ictalurus punctatus). Aquaculture 292, 214–218. doi: 10.1016/j.aquaculture.2009.04.025
Keywords: aquaculture, natural compounds, fish stress, fish health, fish immundogy
Citation: Souza CdF, Baldissera MD, Baldisserotto B, Heinzmann BM, Martos-Sitcha JA and Mancera JM (2019) Essential Oils as Stress-Reducing Agents for Fish Aquaculture: A Review. Front. Physiol. 10:785. doi: 10.3389/fphys.2019.00785
Received: 15 February 2019; Accepted: 04 June 2019;
Published: 20 June 2019.
Edited by:
José Luis Soengas, University of Vigo, SpainReviewed by:
Jose Fernando Lopez-Olmeda, University of Murcia, SpainManuel Gesto, Technical University of Denmark, Denmark
Copyright © 2019 Souza, Baldissera, Baldisserotto, Heinzmann, Martos-Sitcha and Mancera. This is an open-access article distributed under the terms of the Creative Commons Attribution License (CC BY). The use, distribution or reproduction in other forums is permitted, provided the original author(s) and the copyright owner(s) are credited and that the original publication in this journal is cited, in accordance with accepted academic practice. No use, distribution or reproduction is permitted which does not comply with these terms.
*Correspondence: Juan Miguel Mancera, anVhbm1pZ3VlbC5tYW5jZXJhQHVjYS5lcw==