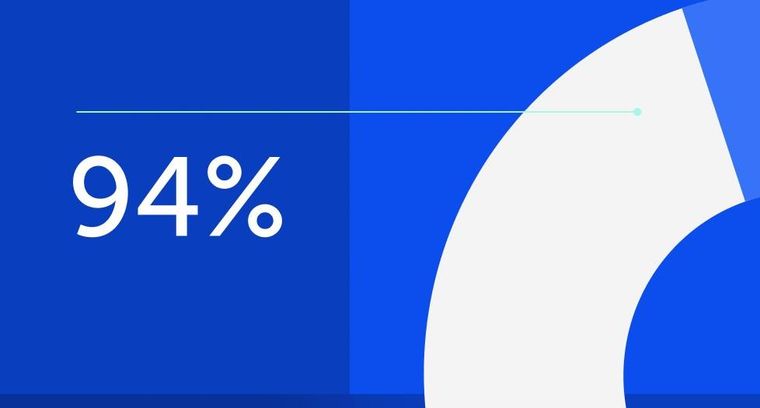
94% of researchers rate our articles as excellent or good
Learn more about the work of our research integrity team to safeguard the quality of each article we publish.
Find out more
ORIGINAL RESEARCH article
Front. Physiol., 28 January 2019
Sec. Exercise Physiology
Volume 10 - 2019 | https://doi.org/10.3389/fphys.2019.00035
This article is part of the Research TopicMuscle Recovery After Exercise, Training and Competition: Physiological Indicators and Non-invasive Monitoring TechniquesView all 16 articles
Purpose: We set out to investigate the effectiveness of grounded sleeping on the time course of recovery with respect to muscle soreness and athletic performance after intensive eccentric muscle loading.
Methods: Twenty-two healthy participants were recruited for this study and randomly assigned to an experimental group (GRD, grounded sleeping, n = 12) or control group (UGD, sham-grounded sleeping, n = 10) to evaluate the effects of 10 days recovery with GRD vs. UGD following a single intensive downhill treadmill intervention in a triple-blinded (participant, tester, and data analyst) manner. To operationalize recovery a test battery was performed at baseline and on days 1, 2, 3, 5, 7, and 10 post-intervention: (1) perception of muscle soreness (VAS), (2) creatine kinase blood levels (CK), (3) maximum voluntary isometric contraction (MVIC) for both legs, (4) counter movement jump (CMJ) and drop jump (DJ) performance. Furthermore, in four participants blood was sampled for detailed analysis of complete blood counts and serum-derived inflammation markers.
Results: The downhill treadmill running intervention led to distinct changes in all measured parameters related to fatigue. These changes were detectable already 5-min post intervention and were not fully recovered 10 days post intervention. GRD led to less pronounced decrease in performance (CMJ, MVIC) and less increase with respect to CK compared with UGD (all P < 0.05). Detailed blood samples demonstrated that grounded sleeping modulates the recovery process by (a) keeping a constant hemoconcentration, as represented by the number of erythrocytes, and the hemoglobin/hematocrit values; and (b) by the reduction of muscle damage-associated inflammation markers such as, IP-10, MIP-1α, and sP-Selectin.
Conclusion: The downhill running protocol is a feasible methodology to produce long term muscle soreness and muscular fatigue. GRD was shown to result in faster recovery and/or less pronounced markers of muscle damage and inflammation. GRD might be seen as a simple methodology to enhance acute and long-term recovery after intensive eccentric exercises.
Recovery following intense training bouts is crucial for both professional and recreational athletes. It is well understood that exercise-induced muscle damage (EIMD) usually follows novel, unaccustomed repetitive movements and/or strenuous eccentric contractions (Proske and Morgan, 2001; Proske et al., 2004; Mackey et al., 2008). This kind of external load induces muscle damage that is associated with delayed onset muscle soreness (DOMS) (Hough, 1902).
Exercise-induced muscle damage is often associated with temporary substantial drops in performance, local muscle soreness and enhanced risk for musculoskeletal injuries. In professional sports, athletes have to recover within a few days to regain their performance levels. In spite of both the frequency and monetary consequences of DOMS in elite athletes, the de facto underlying mechanisms and their impacts on performance and treatment strategies remain vague (Cheung et al., 2003). A number of conventional therapeutic strategies are applied to alleviate clinical symptoms of DOMS. Nonetheless, Cheung et al. (2003) and Seidel et al. (2012) concluded that, in fact, no treatment strategy consistently supported or enhanced muscle recovery, which indicates that there is actually a lack of compelling evidence-based and practical strategies to help prevent and/or alleviate DOMS.
Grounding, earthing, or grounded sleeping, is a process in which the athlete becomes grounded via an electrically conducted device. The person is grounded in an indirect way that corresponds to being barefoot with direct, continuous contact with the earth. Nowadays, it is almost impossible to be earthed, due to urbanization, insulating footwear or bituminization. There are various grounding systems available that permit contact with the surface of the Earth. This indirect way of earthing or grounding is based on trivial conductive systems like sheets, mats, wrist or ankle bands, adhesive patches that can be used during sleeping or working, or inside footwear. These devices get coupled to the Earth by a typical cord slotted into a grounded wall outlet (Oschman et al., 2015). Referring to Chevalier et al. (2006); Oschman (2007), and Oschman et al. (2015) the main hypothesis about earthing is based on the connection to the surface of the Earth, which is satiated with free electrons. This indirect or direct contact with the Earth enables “mobile” electrons to migrate into the body. Oschman (2007) suggests that these free electrons act as antioxidants in the organism and could neutralize reactive oxygen species (ROS). ROS are byproducts of mitochondrial metabolism of oxygen and delivered by the oxidative burst as part of the inflammation response. Harman (1956, 2009) postulated that these reactive chemical species are linked to the aging process, originally known as free-radical theory of aging, and in the 1970s was extended to the mitochondrial theory of aging. Over the past several years, an emerging body of evidence is starting to indicate that ROS are associated with tumorigenesis, cancer and chronic inflammatory systemic diseases (Reddy and Clark, 2004; Waris and Ahsan, 2006; Gupta et al., 2012). Consequently, Oschman (2007) suggests that the earthing based mobile electrons could also prevent or diminish inflammation.
Actually, evidence-based research regarding the effectiveness of grounding is lacking. The narrative review of Chevalier et al. (2012) included grounding studies that indicated improvements in sleep (Ghaly and Teplitz, 2004), indices of DOMS (Brown et al., 2010; Brown et al., 2015), autonomic tone (Sinatra, 2011) and reduction in blood viscosity (Chevalier et al., 2013; Brown and Chevalier, 2015). Due to the potentially reduced blood viscosity, enhanced blood flow velocity, improved sleep quality and decreased muscle damage, it is suggested that grounding could be implemented as a viable, effective recovery tool after strenuous exercise. However, currently, the evidence of treatment strategies to accelerate recovery after EIMD is still inconsistent (Connolly et al., 2003).
Therefore, the aim of this study was to investigate the effectiveness of grounded sleeping on the time course of DOMS and athletic performance after intensive downhill running. We hypothesized that grounded sleeping would alleviate exercise induced muscle and accelerate the recovery of athletic performance after strenuous downhill running.
Twenty-two healthy sport science and physiotherapy students (mean ± SD; n = 22, 10 women, 12 men, age: 23.8 ± 3.2 years, weight: 67.2 ± 7.6 kg, height: 174.2 ± 6.3 cm) were recruited for this study. All Participants were free of musculoskeletal disorders, cardiovascular diseases, dietary supplements or prescription medicines that could potentially affect muscle recovery. All participants received detailed oral and written information about the procedures and the possible risks, and gave their written consent to participate. The study received approval from the local Ethics Committee of the University of Salzburg (Kapitelgasse 4, 5020 Salzburg, GZ 13/2017) and was conducted in accordance with the Declaration of Helsinki. Participants were given grounding equipment for compensation. Participants were randomly assigned to the experimental group (GRD, grounded sleeping, n = 12) or the control group (UGD, sham-grounded sleeping, n = 10). No difference in any baseline values between groups were detected (Table 1).
Table 1. Physical characteristics of the participants in the grounded sleeping group (GRD) versus the sham-grounded group (UGD) (mean ± SD).
A triple-blinded (participant, tester, and data analyst) randomized controlled design was used to evaluate the effects of 10 days recovery including grounded sleeping (GRD) or sham-grounded sleeping (UGD) after a single intensive downhill treadmill running intervention designed to induce DOMS. To operationalize recovery a test battery was performed before the intervention (baseline) and on days 1, 2, 3, 5, 7, and 10 post intervention. This test battery included: perception of muscle soreness via a visual analog scale (VAS), creatine kinase blood levels (CK), maximum voluntary isometric contraction (MVIC) for both legs, counter movement jump (CMJ) and drop jump (DJ) performance (see Figure 1). Furthermore, as a pilot study in four participants (GRD, n = 2; and UGD, n = 2), blood was sampled for detailed analysis of complete blood counts and serum-derived inflammation markers.
Figure 1. Schematic illustration of the procedure with a timeline: pretest (–30 min prior to intervention), intervention (downhill running), posttests (+5 min, 24 h, 48 h, 72 h, 5 days, 7 days, and 10 days post intervention) consisting determination of blood creatine kinase content (CK), visual analog scale (VAS) related to muscle soreness, drop jump (DJ) performance, counter movement jump performance (CMJ) and a maximum voluntary isometric contraction (MVIC) of both the left and right leg.
All participants underwent a 20-min exercise bout of intensive downhill running (-25% slope, 12 km ⋅ h-1) on a motorized treadmill (Saturn 300/100 rs, h/p/cosmos sports & medical GmbH, Germany). For determination of the physiological and psychological loading of the intervention, heart rate (Suunto Ambit 3.0, Helsinki, Finland), blood lactate (Biosen S-Line EFK Diagnostik, Germany) in the 1st, 3rd, and 5th minute post intervention and rating of perceived exertion (BORG, 6–20) in the last minute of running were collected.
During the 10-day post intervention, the GRD group slept grounded with a conductive sheet connected with a grounded wall outlet, while the UGD group slept sham-grounded. Both groups received identical sheets provided by BTZ (Badisches Therapie Zentrum, Baden-Baden, Germany) prior to the start of the study. These sheets (sized 90 cm × 200 cm) are made of 100% cotton with conductive silver fibers woven into the fabric. The sheet attaches to a grounded cord that connects at the other end to the ground of the wall socket. There is no direct connection to electricity; therefore, grounding via an indirect manner (e.g., cable) is safe and does not pose any danger to the individuals. To establish the control situation and subsequent blinding, the grounding plugs were manipulated and masked by an independent person. Neither the investigator, nor the participants were aware of this modification of the grounded system. The participants were instructed to sleep grounded at home on their sheets, ensuring as much skin contact as possible. Additionally, the participants were consistently reminded that it would be best if they slept either naked, or at most, wear no more than underwear. The participants were allowed to sleep with a pillow that was not grounded. In the event that a participant was not to sleep at home on any of the 10 days, they were advised to take the sheet with them.
A pilot study (n = 10) about the effects of earthing with the conductive sheets (grounded vs. sham-grounded) was performed prior to start of the main study. Participants were asked to lay down on a massage bed and relax on both the grounded139 or sham-grounded sheet for 5-min each wearing only underwear. Electrostatic charge was measured on the skin at the region of the vastus lateralis via an electrostatic voltmeter (ESVM 1000, Wolfgang Warmbier, Germany). The pilot study revealed values of -0.2 ± 0.1 V vs. -81.9 ± 25.6 V (P < 0.001) in the grounded vs. sham-grounded situation clearly demonstrating the effects of grounding via the conductive sheet.
The CMJ was executed without arm-swing and the DJ was performed from a 40 cm dropping height. Both jump modes were performed on a force plate (BP600900, AMTI, United States) to evaluate the maximum jumping height (CMJ and DJ) and the ground contact time and coefficient between jump height and ground contact time during the DJ. The best of three attempts were used for further analysis.
The MVIC of the left and the right leg was assessed in a self-constructed unilateral horizontal leg press with an integrated load cell (Hottinger Baldwin Messtechnik GmbH, Germany). The participants were seated with 110° knee flexion angle (180° equivalent to full knee extension) and were allowed to grab the handles. The individual set up of the knee angle was documented with a goniometer to ensure identical settings at baseline and at follow-ups. The best of three attempts was used for further analysis. The stronger leg at baseline was defined as the dominant leg. The researchers motivated verbally during the execution.
The CK enzyme is one of the most common objective reported indices of DOMS that is associated with muscle damage (Eston et al., 1994; Urhausen et al., 1995; Brown et al., 2015; Saw et al., 2016). For analysis of CK a capillary blood draw of 32 μl was taken and analyzed with the Reflotron Sprint system (Roche Diagnostics GmbH, Germany).
The VAS was used to evaluate the muscle soreness of the lower extremity. The length of the VAS was 100 mm, and the participants had to fill in the scale at the beginning of each test day. The VAS is commonly used to assess the muscle soreness and is a valid and reliable test (Bijur et al., 2001; Gallagher et al., 2001).
In four subjects venous blood was collected at each time point. All blood draws were performed by an experienced and certified phlebotomist. The analysis of blood counts (erythrocytes, hemoglobin, hematocrit, MCV, MCH, MCHC, leucocytes, monocytes, granulocytes, platelets and lymphocytes) was performed using a Celltac MEK-6500 (EuroLAB, Hallein, Austria). Serum-derived inflammation markers including sE-selectin, GM-CSF, sICAM-1/CD54, IFNα, IFNγ, IL-1α, IL-1β, IL-4, IL-6, IL-8, IL-10, IL-12p70, IL-13, IL-17A/CTLA-8, IP-10/CXCL10, MCP-1/CCL2, MIP-1α/CCL3, MIP-1β/CCL4, sP-selectin, and TNF alpha were analyzed with a Luminex® MAGPIX® System (Luminex Corporation, Austin, TX, United States) using a 20-Plex Human ProcartaPlex PanelTM (Thermo Fisher Scientific, Waltham, MA, United States). The C-reactive protein (CRP) value was determined from full blood using a EUROLyser CUBE-S (EUROLyser Diagnostica GmbH, Salzburg, Austria). Raw data were normalized to percentage difference based on the baseline value (day 0), equation: 100∗(value-baseline)/baseline.
All data exhibited a Gaussian distribution verified by the Shapiro–Wilk’s test and accordingly, the values are presented as means (±SD). The effects of the downhill treadmill running intervention on the various test parameters were analyzed with paired sampled t-tests between baseline and 5-min post intervention values. All post intervention test data where grounded or sham-grounded overnight sleeping has already taken place (days 1–10) were compared as how they changed from the baseline levels in per cent (day 0). A 2 × 6 ANOVA with repeated measures was used to compare both groups (GRD versus UGD) over the six-time points (days 1, 2, 3, 5, 7, and 10). The blood data were analyzed using the GraphPad Prism 7 software (La Jolla, CA, United States). Statistical analysis of blood values and inflammation markers was performed using an unpaired t-test with Welch’s correction. Alpha level of significance was set to 0.05. In addition, the values obtained were evaluated by calculating the effect size () and statistical power. The Statistical Package for the Social Sciences (Version 24.0; SPSS Inc., Chicago, IL, United States) was used for statistical analysis.
In both groups the intensive downhill treadmill protocol led to high physiological (e.g., heart rate of 200 bpm) and psychological loading (RPE between 18 and 19) with slightly higher response in UGD compared with GRD with respect to peak blood lactate (5.1 versus 3.2 mmol ⋅ L-1, P = 0.047) and RPE (18.9 vs. 18.3, P = 0.033) (Table 2). The intervention led to distinct reductions in jump performance (CMJ jump height, DJ jump height, DJ coefficient, all P < 0.001), MVIC during leg extension (both legs P < 0.001) and increases in VAS associated with muscle soreness (P < 0.001). CK levels were increased in both GRD (P = 0.003) and UGD (P = 0.024).
Table 2. Physical and psychological exertion during the treadmill downhill running intervention in the grounded sleeping (GRD) and sham-grounded sleeping (UGD) group (mean ± SD).
The time course of the absolute values in measured variables across the 10-days recovery period is presented in Table 3. With respect to the CMJ jump height there was a main effect of time (P < 0.001, = 0.50, power = 1.0) and group, with a systematically lower reduction in GRD compared with UGD (-8.2 ± 5.4% vs. -14.3 ± 5.4%, P = 0.017, = 0.25, power = 0.70) but no interaction in the time course of recovery between the two groups (P = 0.79). Lowest CMJ performance was achieved on the 1st day post intervention (Figure 2).
Table 3. Measured test parameters during the 10 days recovery period after the strenuous downhill running intervention in the grounded sleeping (GRD) and sham-grounded sleeping (UGD) group (mean ± SD).
Figure 2. Time course of the % reductions with respect to baseline levels in the counter movement jump (CMJ) across the 10 days post intervention period. UGD, sham-grounded sleeping group; GRD, grounded sleeping group (mean ± SD).
For the DJ jump height, ground contact time and jump coefficient there was a main effect of time (all P < 0.001, = 0.28 to 0.88, power = 1.0). Both for jump height and ground contact time, no main effect for group was found. Lowest performance in the DJ was detected on day 2 (height and coefficient) and 3 (ground contact time) post intervention. For the jump coefficient a trend toward a group effect (P = 0.06) with a lower reduction in GRD compared with UGD (-12.2 ± 10.6% vs. -21.4 ± 10.6%) but no interaction between time x group (P = 0.18) was found.
For the MVIC in the dominant leg, a main effect of time (P < 0.001, = 0.49, power = 1.0) and group (P < 0.03, = 0.22, power = 0.61) with a less pronounced reduction in performance in GRD compared with UGD (-9.5 ± 16.8% vs. -17.3 ± 38.3%) was found. Day-to-day analysis revealed group × time interactions within the first 3 days with a more pronounced recovery in GRD compared with UGD (P < 0.05) (Figure 3). For the non-dominant leg, only a main effect of time was found (P < 0.001, = 0.49, power = 1.0) with no group differences. Lowest strength performance for both legs was found immediately after the intervention (day 0).
Figure 3. Time course of the % reductions with respect to baseline levels in the isometric maximal strength for the dominant leg across the 10 days post intervention period. UGD, sham-grounded sleeping group; GRD, grounded sleeping group (mean ± SD).
With respect to CK levels main effects of time (P < 0.001, = 0.30, power = 0.98) and group (P = 0.007, = 0.31, power = 0.81) and an interaction effect time × group (P = 0.001, = 0.26, power = 0.95) were found, demonstrating a lower increase GRD compared with UGD (310 ± 120% vs. 760 ± 380%) paralleled with a more pronounced increase in UGD at days 3, 5, and 7. Highest CK levels were measured on day 5 post intervention (Figure 4A). Individual response analysis revealed that within the GRD group none of the participants demonstrated a large increase in CK levels (i.e., change of >20% from baseline), while in UGD this was the case in 40% of the participants (n = 4). Furthermore in GRD even 25% (n = 3) demonstrated no increase (e.g., <3%) in CK levels while this was not the case in any participant of UGD (Figure 4B).
Figure 4. (A) Time course of the % increases with respect to baseline levels for CK-blood levels across the 10 days post intervention period. UGD, sham-grounded sleeping group; GRD, grounded sleeping group (mean ± SD). (B) Individual response analysis for increases in CK levels with respect to GRD (grounded sleeping) and UGD (sham-grounded sleeping). Percent differences are categorized as non-response: <3%, moderate response: 3–10%, large response: 10–20%, and very large response >20%.
Regarding VAS only a significant effect of time (P < 0.001, = 0.55, power = 1.0) with no main effect of group (P = 0.13) or interaction effect (P = 0.46) was found. VAS was highest at day 2.
The effects of grounded sleeping on the post-exercise recovery process of four participants were further analyzed at the cellular and molecular levels. With regard to the differential blood count (Figure 5, Supplementary Figure S1, and Supplementary Tables S1, S2), the most obvious divergence between both groups was observed for erythrocyte counts and for hemoglobin and hematocrit values, which significantly increased from recovery days 2–7 in the UGD group (P = 0.007, P = 0.029, and P = 0.017, respectively). These alterations were accompanied by a decrease in the average volume of red blood cells (MCV, P = 0.024). In contrast, these parameters remained at baseline level in the GRD group. No difference was found between the two groups regarding the average mass of hemoglobin per red blood cell (MCH), indicating that the ratio of hemoglobin to erythrocytes was not altered during the recovery process following intense exercise activity. A slight initial boost in the number of leucocytes (103 cells per μl) was observed for the GRD group between days 3 and 7 of the recovery phase (P = 0.079). Between recovery days 3 and 5, a similar trend was observed for granulocytes (P = 0.038), which represent the vast majority of white blood cells (Lacelle and Cameron, 2015). Interestingly, a significant decrease of blood-derived monocytes was detected in the recovery phase between days 2 and 7 in GRD group (P = 0.039). In both UGD and GRD groups, the amount of platelets (103/mm3) decreased in the recovery phase following intensive eccentric muscle loading. However, the amount of platelets in the UGD group returned to baseline levels earlier (between days 5 and 10, P = 0.01) than in the GRD group, suggesting that a prolonged recruitment of platelets to injured muscle tissue may have occurred. No significant alterations between both groups were observed for the mean corpuscular hemoglobin concentration (MCHC) and the overall lymphocyte count.
Figure 5. Parameters of the differential blood count observed over a defined time, including erythrocytes, hemoglobin, hematocrit, MCV, leucocytes, granulocytes, monocytes and platelets; represented as mean with standard deviation of the defined days of each group; nsP > 0.05, ∗P ≤ 0.05, ∗∗P ≤ 0.01, ∗∗∗P ≤ 0.001.
The C-reactive protein (CRP) value was monitored throughout the study and used as an indicator of unrelated inflammatory conditions (e.g., viral or bacterial infections), which could affect the evaluated blood parameters. The CRP values remained unaltered in all four subjects (Supplementary Tables S3, S4).
At the molecular level, we found alterations in serological markers of inflammation, particularly for soluble cell adhesion molecules (sCAM) and chemokines (Figure 6, Supplementary Figure S2, and Supplementary Tables S3, S4). The intercellular adhesion molecule 1 (ICAM-1), which is expressed on epithelial cells and involved in cell adhesion and co-stimulation of macrophages, monocytes and granulocytes, either remained at baseline level or was higher in the GRD compared to the UGD group. From days 1 to 5, a clear decrease of the sICAM-1 levels (approximately 10%) was observed in the UGD group (P = 0.005). Furthermore, the cell adhesion molecule, sP-selectin, was downregulated in the GRD compared to the UGD and remained below baseline (approximately 20% reduction; P = 0.003) during the whole study period. In general, inflammation-associated chemokines, such as the interferon gamma-induced protein 10 (IP-10) and the macrophage inflammatory proteins (MIP-1α and MIP-1β), showed lower values (P = 0.014, P = 0.004, and P = 0.359, respectively) in the GRD group. No significant alterations were observed for the monocyte chemoattractant protein 1 (MCP-1), which is involved in the recruitment of monocytes, and the sCAM sE-selectin. It was not possible to detect chemokines/cytokines GM-CSF, IFNα, IFNγ, IL-1α, IL-1β, IL-4, IL-6, IL-8, IL-10, IL-12p70, IL-13, IL-17A/CTLA-8, and TNFα in the sera of the healthy athletes within the assay’s limit of detection.
Figure 6. Inflammation markers observed over a defined time, including sICAM-1, IP-10, MIP-1α, MIP-1β, and sP-selectin; represented as mean with standard deviation of the defined days of each group; nsP > 0.05, ∗P ≤ 0.05, ∗∗P ≤ 0.01, ∗∗∗P ≤ 0.001.
The main findings of the current study were sixfold: (1) the downhill treadmill running intervention led to distinct changes in measured parameters related to fatigue and were already detectable 5 min post intervention; (2) grounded sleeping led in various variables to less pronounced decrease in performance compared with sham-grounded sleeping; (3) grounded sleeping led to a much lower increase of CK compared with UGD during the whole recovery; (4) 10 days post intervention in none of the measured parameters full recovery has been stated; (5) highest CK levels were found on day 5, while for jump and strength performance the highest decrease was the case on day 1 and for VAS the highest increase happened on day 2 post intervention, and (6) detailed blood samples demonstrated that grounded sleeping modulates the recovery process by (a) keeping a constant hemoconcentration, as represented by the number of erythrocytes, and the hemoglobin/hematocrit values, and (b) by the reduction of muscle damage-associated inflammation markers such as IP-10, MIP-1α, and sP-Selectin.
Repetitive eccentric muscle activations like downhill running have repeatedly been shown to be responsible for DOMS. DOMS is associated with release of muscle proteins like CK into the blood stream, as well as with prolonged decreases in muscle performance capacity (Hoppeler, 2016). During eccentric exercises, the activated muscles are stretched which can create mechanical damage to sarcomeres, inflammation, disruption of the sarcolemma, as well as damage by reactive oxygen species (Lovering and Brooks, 2014). The applied 20-min downhill running protocol within the current study led to distinct decreases in performance and increases in measures of muscle damage and VAS, within the majority of analyzed parameters. No measured parameter returned to baseline levels after 10 days of recovery. Therefore, the applied downhill running intervention can be seen as a well-chosen method to provoke muscle damage resulting in distinct decrements in performance. This protocol can, therefore, be seen as suitable for analyzing the effectivity of different types of recovery interventions. In this specific case to analyze if grounded sleeping is able to enhance the ability to recover more quickly, or to dampen the decrements in performance when compared with an ungrounded situation. It is worth noting that Cheung et al. (2003) and Seidel et al. (2012) concluded that, in fact, no treatment strategy consistently supported or enhanced muscle recovery, which indicates that there is actually a lack of compelling evidence-based and practical strategies to help prevent and/or alleviate DOMS. Furthermore, the evidence of treatment strategies to accelerate recovery after EIMD is still inconsistent (Connolly et al., 2003).
However, the current study revealed that GRD showed lower decrements in performance with respect to CMJ, maximal leg strength (MVIC), a trend for DJ coefficient and less pronounced in increase in CK when compared with UGD. The less pronounced decrease in measures of performance (strength, jump performance) and less increase in CK levels within the GRD group might be attributed toward potentially reduced blood viscosity (Chevalier et al., 2013; Brown and Chevalier, 2015), enhanced blood flow velocity, improved sleep quality (Ghaly and Teplitz, 2004) and decreased muscle damage (Brown et al., 2010; Brown et al., 2015) as it is clearly demonstrated by the blood analyses of the present study. Referring to Chevalier et al. (2006); Oschman (2007), and Oschman et al. (2015) the main hypothesis about earthing is based on the connection to the surface of the Earth, which is satiated with free electrons. This indirect or direct contact with the Earth enables “mobile” electrons to migrate into the body. Consequently, Oschman (2007) suggests that the earthing based mobile electrons could also prevent or diminish inflammation, which could also be the reason for the unaffected hemoconcentration as well as the dampened CK response.
The observed increase in the number of erythrocytes and in the hemoglobin and hematocrit values in the course of intensive eccentric muscle loading in the UGD group could be due to volumetric variation. Post-exercise hemoconcentration is typically resulting from water loss (Bloomer and Goldfarb, 2004; Del Coso et al., 2008). Hemoconcentration is also a possible explanation for the increased CK levels in the UGD group (Bassini-Cameron et al., 2007). Our preliminary results suggest that grounded sleeping might prevent the phenomenon of hemoconcentration via the reduction of blood viscosity and improved blood circulation (Chevalier et al., 2013). No alteration in the lymphocyte counts in the blood between both groups was expected since lymphocytes are mostly associated with adaptive immune responses to foreign antigens originating from bacteria, virus, and parasites (Paul and Seder, 1994).
Upon muscle injury, damaged myofibres and other muscle cells at lesion sites undergo necrosis, which in turn are removed by various infiltrating immune cells, mostly mast cells and neutrophils (Bentzinger et al., 2013). These early stages of muscle regeneration are further characterized by activation of the complement system and release of pro-inflammatory cytokines and chemokines such as, TNF-α, IFN-γ, IP-10, and sICAM-1 (Chazaud et al., 2003; Cheng et al., 2008). In response to INF-γ and TNF-α, several cell types secrete interferon gamma-induced protein 10 (IP-10) that can be measured in the blood. The significant decrease in IP-10 observed in the GRD group strongly suggests that grounded sleeping downregulates INF-γ-induced inflammatory responses as well as IP-10-associated (NK) cell-mediated cytolysis. Furthermore, a decrease in the levels of the anti-angiogenic IP-10 might favor angiogenesis and the subsequent influx of blood to the regenerating muscle (Gotsch et al., 2007).
Following these early events in muscle regeneration, muscle stem cells are activated and other immune cells, especially macrophages and T cells, are recruited to the injured muscle tissue. Accordingly, a significant increase in the number of macrophages was observed on day two post-injury (Yang and Hu, 2018). These observations are in line with our findings showing that both chemokines MIP-1α and MIP-1β, which are produced by macrophages (Petray et al., 2002), are increased in the UGD group starting at day 2 post-injury. In contrast, our pilot study showed a decrease in the levels of these chemokines in the GRD group, suggesting a lower attraction of macrophages and thus local inflammation.
Our assumption that grounded sleeping dampens inflammation is further supported by a general decrease of inflammation markers observed in the GRD group. Of note, the sCAM sP-selectin (Schrijver et al., 2017) remained below the baseline level in the GRD group throughout the observation period, suggesting that grounded sleeping has a beneficial and long-lasting effect on this inflammation marker. sP-selectin is found on activated endothelial cells and platelets and is a crucial factor for leukocyte recruitment to lesion sites (Cleator et al., 2006). In the GRD group, a downregulation of activation of sP-selectin on endothelial cells and platelets could result in diminished leukocyte recruitment at sites of damaged muscle tissue.
Our findings that another sCAM, sICAM-1, was found to decline in the UGD compared to the GRD group was not in line with other studies showing high plasma sICAM-1 levels after strenuous exercise. However, sICAM-1 levels seem to be altered depending on the type of exercise as no alterations were observed after bicycle ergometer exercise (Akimoto et al., 2002).
Taken together, grounded sleeping was shown to result in faster recovery and/or less pronounced markers of muscle damage and inflammation. Our preliminary results with respect to the detailed blood analysis strongly support the view that grounded sleeping modulates key events in the early stages of muscle regeneration at both cellular and molecular levels. Based on the investigated immunological parameters, the modulatory effects of grounded sleeping seem to dampen inflammatory responses triggered by EIMD. GRD might be seen as a simple methodology to enhance acute and long-term recovery after intensive exercises within the training process or following intensive competitions. Based on the results of the pilot study, more research is necessary to clearly point out the mechanisms behind possible effects of grounded sleeping on the cellular and molecular levels. Additionally, the magnitude of being grounded, that is how many “mobile” electrons migrate into the body while GRD vs. UGD sleeping, needs to be investigated further. Finally, whether or not grounded sleeping affects sleep patterns (e.g., sleep quality) also needs to be addressed in future studies. An improvement in the sleeping quality by grounded sleeping might result in alterations in performance and changes in stress markers in athletes.
EM, TS, PP, LA, and FF-B conception and designed the experiments. PP and LA performed the experiments. PP, TS, and LA analyzed data. TS, PP, EM, and LA prepared the manuscript. All authors read and approved the final manuscript.
The authors declare that the research was conducted in the absence of any commercial or financial relationships that could be construed as a potential conflict of interest.
The authors thank Dr. Angelika Stöcklinger (Department of Biosciences, University of Salzburg) for blood samples collection and Mag. Monika Stadlmann (Department of Sport and Exercise Science, University of Salzburg) for her assistance in the laboratory.
The Supplementary Material for this article can be found online at: https://www.frontiersin.org/articles/10.3389/fphys.2019.00035/full#supplementary-material
Akimoto, T., Furudate, M., Saitoh, M., Sugiura, K., Waku, T., Akama, T., et al. (2002). Increased plasma concentrations of intercellular adhesion molecule-1 after strenuous exercise associated with muscle damage. Eur. J. Appl. Physiol. 86, 185–190. doi: 10.1007/s00421-001-0544-6
Bassini-Cameron, A., Sweet, E., Bottino, A., Bittar, C., Veiga, C., and Cameron, L. C. (2007). Effect of caffeine supplementation on haematological and biochemical variables in elite soccer players under physical stress conditions. Br. J. Sports Med. 41, 523–530; discussion 530. doi: 10.1136/bjsm.2007.035147
Bentzinger, C. F., Wang, Y. X., Dumont, N. A., and Rudnicki, M. A. (2013). Cellular dynamics in the muscle satellite cell niche. EMBO Rep. 14, 1062–1072. doi: 10.1038/embor.2013.182
Bijur, P. E., Silver, W., and Gallagher, E. J. (2001). Reliability of the visual analog scale for measurement of acute pain. Acad. Emerg. Med. 8, 1153–1157. doi: 10.1111/j.1553-2712.2001.tb01132.x
Bloomer, R. J., and Goldfarb, A. H. (2004). Anaerobic exercise and oxidative stress: a review. Can. J. Appl. Physiol. 29, 245–263. doi: 10.1139/h04-017
Brown, D., Chevalier, G., and Hill, M. (2010). Pilot study on the effect of grounding on delayed-onset muscle soreness. J. Altern. Complement. Med. 16, 265–273. doi: 10.1089/acm.2009.0399
Brown, R., and Chevalier, G. (2015). Grounding the human body during yoga exercise with a grounded yoga mat reduces blood viscosity. Open J. Preve. Med. 5, 159–168. doi: 10.4236/ojpm.2015.54019
Brown, R., Chevalier, G., and Hill, M. (2015). Grounding after moderate eccentric contractions reduces muscle damage. Open Access J. Sports Med. 6, 305–317. doi: 10.2147/OAJSM.S87970
Chazaud, B., Sonnet, C., Lafuste, P., Bassez, G., Rimaniol, A. C., Poron, F., et al. (2003). Satellite cells attract monocytes and use macrophages as a support to escape apoptosis and enhance muscle growth. J. Cell Biol. 163, 1133–1143. doi: 10.1083/jcb.200212046
Cheng, M., Nguyen, M. H., Fantuzzi, G., and Koh, T. J. (2008). Endogenous interferon-gamma is required for efficient skeletal muscle regeneration. Am. J. Physiol. Cell Physiol. 294, C1183–C1191. doi: 10.1152/ajpcell.00568.2007
Cheung, K., Hume, P., and Maxwell, L. (2003). Delayed onset muscle soreness: treatment strategies and performance factors. Sports Med. 33, 145–164. doi: 10.2165/00007256-200333020-00005
Chevalier, G., Mori, K., and Oschman, J. L. (2006). The effect of earthing (grounding) on human physiology. Eur. Biol. Bioelectro 2, 600–621. doi: 10.2466/06.PR0.116k21w5
Chevalier, G., Sinatra, S. T., Oschman, J. L., and Delany, R. M. (2013). Earthing (grounding) the human body reduces blood viscosity—a major factor in cardiovascular disease. J. Altern. Complement. Med. 19, 102–110. doi: 10.1089/acm.2011.0820
Chevalier, G., Sinatra, S. T., Oschman, J. L., Sokal, K., and Sokal, P. (2012). Earthing: health implications of reconnecting the human body to the Earth’s surface electrons. J. Environ. Public Health 2012:291541. doi: 10.1155/2012/291541
Cleator, J. H., Zhu, W. Q., Vaughan, D. E., and Hamm, H. E. (2006). Differential regulation of endothelial exocytosis of P-selectin and von Willebrand factor by protease-activated receptors and cAMP. Blood 107, 2736–2744. doi: 10.1182/blood-2004-07-2698
Connolly, D. A., Sayers, S. P., and Mchugh, M. P. (2003). Treatment and prevention of delayed onset muscle soreness. J. Strength Cond. Res. 17, 197–208.
Del Coso, J., Estevez, E., and Mora-Rodriguez, R. (2008). Caffeine effects on short-term performance during prolonged exercise in the heat. Med. Sci. Sports Exerc. 40, 744–751. doi: 10.1249/MSS.0b013e3181621336
Eston, R. G., Critchley, N., and Baltzopoulos, V. (1994). Delayed-onset muscle soreness, strength loss characteristics and creatine kinase activity following uphill and downhill running. J. Sports Sci. 12:135.
Gallagher, E. J., Liebman, M., and Bijur, P. E. (2001). Prospective validation of clinically important changes in pain severity measured on a visual analog scale. Ann. Emerg. Med. 38, 633–638. doi: 10.1067/mem.2001.118863
Ghaly, M., and Teplitz, D. (2004). The biologic effects of grounding the human body during sleep as measured by cortisol levels and subjective reporting of sleep, pain, and stress. J. Altern. Complement. Med. 10, 767–776. doi: 10.1089/acm.2004.10.767
Gotsch, F., Romero, R., Friel, L., Kusanovic, J. P., Espinoza, J., Erez, O., et al. (2007). CXCL10/IP-10: a missing link between inflammation and anti-angiogenesis in preeclampsia? J. Matern. Fetal Neonatal Med. 20, 777–792. doi: 10.1080/14767050701483298
Gupta, S. C., Hevia, D., Patchva, S., Park, B., Koh, W., and Aggarwal, B. B. (2012). Upsides and downsides of reactive oxygen species for cancer: the roles of reactive oxygen species in tumorigenesis, prevention, and therapy. Antioxid. Redox Signal. 16, 1295–1322. doi: 10.1089/ars.2011.4414
Harman, D. (1956). Aging: a theory based on free radical and radiation chemistry. J. Gerontol. 11, 298–300. doi: 10.1093/geronj/11.3.298
Harman, D. (2009). Origin and evolution of the free radical theory of aging: a brief personal history, 1954–2009. Biogerontology 10, 773–781. doi: 10.1007/s10522-009-9234-2
Hoppeler, H. (2016). Moderate load eccentric exercise; a distinct novel training modality. Front. Physiol. 7:483. doi: 10.3389/fphys.2016.00483
Hough, T. (1902). Ergographic studies in muscular soreness. Am. Phys. Educ. Rev. 7, 1–17. doi: 10.1080/23267224.1902.10649879
Lacelle, C., and Cameron, A. (2015). Differences in blood composition between deceased and living donors: could it affect crossmatches? Hum. Immunol. 76:90. doi: 10.1016/j.humimm.2015.07.127
Lovering, R. M., and Brooks, S. V. (2014). Eccentric exercise in aging and diseased skeletal muscle: good or bad? J. Appl. Physiol. 116, 1439–1445. doi: 10.1152/japplphysiol.00174.2013
Mackey, A. L., Bojsen-Moller, J., Qvortrup, K., Langberg, H., Suetta, C., Kalliokoski, K. K., et al. (2008). Evidence of skeletal muscle damage following electrically stimulated isometric muscle contractions in humans. J. Appl. Physiol. 105, 1620–1627. doi: 10.1152/japplphysiol.90952.2008
Oschman, J. L. (2007). Can electrons act as antioxidants? A review and commentary. J. Altern. Complement. Med. 13, 955–967. doi: 10.1089/acm.2007.7048
Oschman, J. L., Chevalier, G., and Brown, R. (2015). The effects of grounding (earthing) on inflammation, the immune response, wound healing, and prevention and treatment of chronic inflammatory and autoimmune diseases. J. Inflamm. Res. 8, 83–96. doi: 10.2147/JIR.S69656
Paul, W. E., and Seder, R. A. (1994). Lymphocyte responses and cytokines. Cell 76, 241–251. doi: 10.1016/0092-8674(94)90332-8
Petray, P., Corral, R., Meckert, P., and Laguens, R. (2002). Role of macrophage inflammatory protein-1alpha (MIP-1alpha) in macrophage homing in the spleen and heart pathology during experimental infection with Trypanosoma cruzi. Acta Trop. 83, 205–211. doi: 10.1016/S0001-706X(02)00131-6
Proske, U., Gregory, J. E., Morgan, D. L., Percival, P., Weerakkody, N. S., and Canny, B. J. (2004). Force matching errors following eccentric exercise. Hum. Mov. Sci. 23, 365–378. doi: 10.1016/j.humov.2004.08.012
Proske, U., and Morgan, D. L. (2001). Muscle damage from eccentric exercise: mechanism, mechanical signs, adaptation and clinical applications. J. Physiol. 537, 333–345. doi: 10.1111/j.1469-7793.2001.00333.x
Reddy, M. B., and Clark, L. (2004). Iron, oxidative stress, and disease risk. Nutr. Rev. 62, 120–124. doi: 10.1301/nr.2004.mar.120-124
Saw, A. E., Main, L. C., and Gastin, P. B. (2016). Monitoring the athlete training response: subjective self-reported measures trump commonly used objective measures: a systematic review. Br. J. Sports Med. 50, 281–291. doi: 10.1136/bjsports-2015-094758
Schrijver, I. T., Kemperman, H., Roest, M., Kesecioglu, J., and De Lange, D. W. (2017). Soluble P-selectin as a biomarker for infection and survival in patients with a systemic inflammatory response syndrome on the intensive care unit. Biomark. Insights 12:1177271916684823. doi: 10.1177/1177271916684823
Seidel, E. J., Rother, M., Hartmann, J., Rother, I., Schaaf, T., Winzer, M., et al. (2012). Eccentric exercise and delayed onset of muscle soreness (DOMS)–an overview. Phys. Med. Rehabil. Kurortmedizin 22, 57–63. doi: 10.1055/s-0032-1304576
Sinatra, S. T. (2011). Emotional stress, heart rate variability, grounding, and improved autonomic tone: clinical applications. Integr. Med. 10, 16–21.
Urhausen, A., Gabriel, H., and Kindermann, W. (1995). Blood hormones as markers of training stress and overtraining. Sports Med. 20, 251–276. doi: 10.2165/00007256-199520040-00004
Waris, G., and Ahsan, H. (2006). Reactive oxygen species: role in the development of cancer and various chronic conditions. J. Carcinog. 5:14. doi: 10.1186/1477-3163-5-14
Keywords: creatine kinase (CK), downhill running, inflammation, muscle soreness, muscle strength
Citation: Müller E, Pröller P, Ferreira-Briza F, Aglas L and Stöggl T (2019) Effectiveness of Grounded Sleeping on Recovery After Intensive Eccentric Muscle Loading. Front. Physiol. 10:35. doi: 10.3389/fphys.2019.00035
Received: 30 August 2018; Accepted: 11 January 2019;
Published: 28 January 2019.
Edited by:
Fábio Yuzo Nakamura, Università degli Studi “G. d’Annunzio” Chieti - Pescara, ItalyReviewed by:
Júlio Alejandro Costa, Universidade do Porto, PortugalCopyright © 2019 Müller, Pröller, Ferreira-Briza, Aglas and Stöggl. This is an open-access article distributed under the terms of the Creative Commons Attribution License (CC BY). The use, distribution or reproduction in other forums is permitted, provided the original author(s) and the copyright owner(s) are credited and that the original publication in this journal is cited, in accordance with accepted academic practice. No use, distribution or reproduction is permitted which does not comply with these terms.
*Correspondence: Thomas Stöggl, dGhvbWFzLnN0b2VnZ2xAc2JnLmFjLmF0
Disclaimer: All claims expressed in this article are solely those of the authors and do not necessarily represent those of their affiliated organizations, or those of the publisher, the editors and the reviewers. Any product that may be evaluated in this article or claim that may be made by its manufacturer is not guaranteed or endorsed by the publisher.
Research integrity at Frontiers
Learn more about the work of our research integrity team to safeguard the quality of each article we publish.