- 1Departamento de Fisiología, Facultad de Medicina, Universidad de Granada, Granada, Spain
- 2Departamento de Ciencias de la Salud, Universidad de Jaén, Jaén, Spain
- 3Servicio de Nefrología, Unidad Experimental, Hospital Universitario Virgen de las Nieves, Granada, Spain
- 4Departamento de Anatomía Patológica, Instituto de Biopatología y Medicina Regenerativa (IBIMER), Facultad de Medicina, Universidad de Granada, Granada, Spain
- 5Instituto de Investigación Biosanitaria de Granada (ibs.GRANADA), Granada, Spain
The aim of this study was to investigate if urinary glutamyl aminopeptidase (GluAp), alanyl aminopeptidase (AlaAp), Klotho and hydroxyproline can be considered as potential biomarkers of renal injury and fibrosis in an experimental model of obesity. Male Zucker lean (ZL) and obese (ZO) rats were studied from 2 to 8 months old. Kidneys from ZO rats at the end of the study (8 months old) developed mild focal and segmental glomerulosclerosis as well as moderate tubulointerstitial injury. Urinary excretion of Klotho was higher in ZO rats at 2, 5, and 8 months of study, plasma Klotho levels were reduced and protein abundance of Klotho in renal tissue was similar in ZL and ZO rats. GluAp and AlaAp urinary activities were also increased in ZO rats throughout the time-course study. ZO rats showed an augmentation of hydroxyproline content in renal tissue and a significant increase of tubulointerstitial fibrosis. Correlation studies demonstrated that GluAp, AlaAp, and Klotho are early diagnostic markers of renal lesions in Zucker obese rats. Proteinuria and hydroxyproline can be considered delayed diagnostic markers because their contribution to diagnosis starts later. Another relevant result is that GluAp, AlaAp, and Klotho are related not only with diagnosis but also with prognosis of renal lesions in Zucker obese rats. Moreover, strong predictive correlations of aminopeptidasic activities with the percentage of renal fibrosis or with renal hydroxyproline content at the end of the experiment were observed, indicating that an early increased excretion of these markers is related with a higher later extent of fibrosis in Zucker obese rats. In conclusion, GluAp, AlaAp, and Klotho are early diagnostic markers that are also related with the extent of renal fibrosis in Zucker obese rats. Therefore, they have a potential use not only in diagnosis, but also in prognosis of obesity-associated renal lesions.
Introduction
The Zucker obese rat is an experimental model that mimicked diabetes type II in human (de Artinano and Castro, 2009). This model results from an autosomal recessive mutation of the fa-gene, en coding the leptin receptor; and courses with obesity, insulin resistance, dyslipidemia, mild glucose intolerance and renal injury, whereas the Zucker lean rat is lacking of leptin receptor mutation and of metabolic and renal abnormalities.
Renal injury is characterized by an increased time related proteinuria, focal segmental glomerulosclerosis and tubulointerstitial damage that culminates ultimately in renal failure and death (Kasiske et al., 1985, 1992; Magil, 1995; Coimbra et al., 2000; Gassler et al., 2001; D’Agati et al., 2016).
Renal clinical practice requires highly sensitive and specific diagnostic and prognostic biomarkers for acute and chronic kidney disease (CKD). The early identification of renal disease onset and risk stratification in CKD progression are essential for early treatment of patients to ameliorate their comorbidity burden, particularly cardiovascular disease (CVD), and prevent the development of end stage renal disease (ESRD).
Enzymes released from damaged tubular cells and excreted into urine are the most promising biomarkers for an early detection of kidney injury. They have an obvious diagnostic benefit because their measurements may provide detailed information about the nature, size and site of the damage to tubular cells and their possible necrosis or dysfunction (Lisowska-Myjak, 2010). Alanyl aminopeptidase (AlaAp, EC3.4.11.2), and glutamyl aminopeptidase (GluAp, EC 3.4.11.7) are present in the renal tubular cells (Kenny and Maroux, 1982; Song et al., 1994; Albiston et al., 2011) and exert its aminopeptidasic activity in the renal angiotensin II metabolism, factor that is activated in renal injury of diabetic rodents and humans (Gagliardini et al., 2013).
In previous works, we determined the activity of these aminopeptidases as an index of renal damage in hypertensive hyperthyroid rats under normal, high and low saline intake (Pérez-Abud et al., 2011) and in cisplatin treated rats (Quesada et al., 2012). Urinary aminopeptidases, mainly AlaAp and GluAp, were revealed as early and predictive biomarkers of renal injury severity (Quesada et al., 2012; Montoro-Molina et al., 2015). More recently, we have reported that GluAp in microvesicular and exosomal fractions of urine is also related with renal dysfunction in cisplatin-induced nephrotoxicity and that serve to detect proximal tubular damage independently of glomerular filtration status (Quesada et al., 2017).
Schnackenberg et al. (2012) observed that in a model of melamine and cyanuric acid nephrotoxicity, urinary hydroxyproline was increased in male and female Fischer F344 rats; and that rats with the highest levels of urinary hydroxyproline exhibited renal fibrosis. These authors concluded that hydroxyproline may be a non-invasive urinary biomarker for detection of acute kidney injury associated with renal fibrosis.
Klotho is a transmembrane protein that is expressed mainly in the kidney. Parathyroid gland and choroid plexus are also sites of abundant Klotho expression (Kuro-o et al., 1997; Erben, 2016). The soluble extracellular domain is cleaved, presumably from renal cells, and secreted into the blood or other biological fluids (Ito et al., 2000). Thus, Klotho can be found in two forms tisular and secreted or soluble αKlotho. The kidney is probably the major source of the soluble form, since serum soluble Klotho was reduced by about 80% in kidney-specific Klotho knockout mice (Lindberg et al., 2014).
In the kidney, Klotho is expressed in the distal convoluted tubules, in the proximal convoluted tubule (Hu et al., 2010a; Ide et al., 2016) and the inner medullary collecting duct (Mitobe et al., 2005). Klotho mRNA expression in rat kidney is down regulated in various animal models of vascular and metabolic diseases such as hypertension, hyperlipidemia, renal failure, and inflammatory stress (Nagai et al., 2000).
Under physiological conditions, the kidney has dual effects over soluble αKlotho homeostasis, producing and cleaving αKlotho from the membrane of the renal tubular epithelial cells into the circulation and removing αKlotho from the blood into the tubular urinary lumen through transcytosis to cross renal tubules (Hu et al., 2016).
Soluble αKlotho is the main functional form in the circulation (Hu et al., 2016), cerebrospinal fluid (Semba et al., 2014; Chen et al., 2015), and urine (Hu et al., 2010a, 2011) acting as an endocrine factor on distant organs (Hu et al., 2013, 2015). Soluble Klotho is markedly decreased in CKD and ESRD (Lu and Hu, 2017). It has been proposed as a novel, early and sensitive biomarker for renal and CVDs and that can also serve as a predictor for risk of CVD and mortality in both CKD patients and the general population (Shimamura et al., 2012; Scholze et al., 2014). In CKD patients, the magnitude of decrease in urinary Klotho was correlated with the severity of decline in GFR (Hu et al., 2011).
In this work we characterized the histopathological manifestations of obesity –induced renal injury in obese Zucker (ZO) rats; and investigated the urinary enzymatic activities of AlaAp and GluAp, plasma, urinary and renal Klotho levels and urinary and renal hydroxyproline as potential biomarkers of the renal injury observed in ZO rats.
Materials and Methods
Ethics Statement
All experimental procedures were carried out in accordance with the principles of the Basel Declaration and recommendations of European Union Guidelines to the Care and Use of Laboratory Animals. The protocol was approved by the Ethical Committee of the University of Jaén and authorized by Junta de Andalucía with the approval identification number 450-5297 (02/21/2014).
Animals
Male Zucker lean and obese rats (ZL and ZO, n = 10 each group) at 2 months old were purchased from Charles River Laboratories (Wilmington, MA, United States) and studied up to 8 months old. These rats were kept in a room maintained at 24 ± 1°C and humidity of 55 ± 10%, with a 12 h light/dark cycle and had free access to rat chow and tap water.
Experimental Protocol
All rats were weighed and housed in metabolic cages (Panlab, Barcelona, Spain) once a month during 24 h with free access to food and drinking. Food and fluid intakes were measured and 24 h urine samples were collected. Urine samples were centrifuged for 15 min at 1000 g and frozen at -80°C until assay. We measured monthly urine volume, proteinuria, glucosuria, creatinine, hydroxyproline, GluAp, and AlaAp activities. Urinary Klotho was measured at 2, 5, and 8 months old.
After completion of the experimental period (at 8 months old), rats were anesthetized with pentobarbital, 50 mg/kg, i.p. Blood samples were then drawn by abdominal aortic puncture to determine plasma variables. Blood samples were centrifuged for 15 min at 1000 g and stored at -80°C. Finally, rats were killed by pentobarbital overdose (200 mg/kg, i.p.), and kidneys and heart were quickly removed and weighed.
Plasma variables were: glucose, urea, creatinine and Klotho. Tibial length was measured to normalize heart and kidney weight, since body weight cannot be used for this purpose in the present experimental setting. One kidney was fixed in 10% neutral-buffered formaldehyde solution for 48 h and subsequently placed in 70% ethanol for histological studies.
Analytical Procedures
Proteinuria and urine creatinine were determined in urine samples by an autoanalyzer Spin120. Plasma creatinine, urea and glucose were also measured in this instrument. Reagents for proteinuria, urea, and creatinine-Jaffé method were provided by Spinreact (Barcelona, Spain). Plasma and urinary Klotho levels were determined with an enzyme immunoassay kit, Bioassay Technology Laboratory (Shanghai, China). Renal and urinary hydroxyproline was measured with a Hydroxyproline Assay Kit (Sigma-Aldrich, Madrid, Spain). Protein content in renal tissue was analyzed with DC Bio-Rad protein assay (Madrid, Spain).
Measurement of Hydroxyproline in Urine and Renal Tissue
Hundred microliter of urine sample or 100 μl of renal tissue (100 mg/ml in water) were hydrolyzed with the same volume of 37% HCl at 120°C overnight. 5 mg of activated charcoal were added to samples, mixed and centrifuged at 13,000 g for 2 min. 50 μl of each supernatant were transferred to a 69-well plate and dried in a 60°C oven until dryness. 100 μl of chloramine T/oxidation buffer mixture, 50 μl of 4-(dimethylamino) benzaldehyde concentrate and 50 μl of perchloric acid/isopropanol solution were added to each well and plates were heated for 90 min at 60°C. Absorbance was measured at 560 nm. The amount of hydroxyproline in each well was calculated from a standard curve of hydroxyproline containing 0–1 μg/well.
Western Blotting of Klotho in Renal Tissue
Kidneys were homogenized using RIPA lysis cell buffer containing protease inhibitors cocktail (05892791001, Roche, Germany). 30 μg of protein were subjected to SDS-PAGE electrophoresis using 50 μL/well ready gels purchased from Bio-Rad (Madrid, Spain). Proteins were transferred to a nitrocellulose membrane which was probed with 1 μg/mL rabbit anti-Klotho-antibody (Sigma-Aldrich, Madrid, Spain), and 20 ng/mL goat anti-rabbit IgG antibody HRP-conjugated (KPL Diagnostics, Gaithersburg, MD, United States). Bands were visualized with an enhanced chemiluminescence system (ECL, Amersham, United Kingdom) in a CCD camera image system and quantified with Image J software (v 1.48) (National Institute of Health, United States).
Measurement of Aminopeptidasic Activities
GluAp and AlaAp fluorimetric activities were determined in a kinetic fluorimetric assay using L-glutamic acid γ-2-naphthylamide or alanyl-2-naphthylamide (Sigma-Aldrich, Madrid, Spain) as substrates, respectively. 20 μl of urine, plasma or kidney homogenate were incubated during 30 min at 37°C with 80 μl of substrate solution (10 mM L-glutamic acid γ-2-naphthylamide or 10 mM alanyl-2-naphthylamide in pH 8.7 50 mM HCl-Tris). This buffer was also used to homogenate kidneys. Substrates had been previously dissolved in 1 ml of dimethyl sulfoxide and stored at -20°C. The amount of 2-naphthylamine released as a result of the aminopeptidase activities was measured fluorimetrically at an emission wavelength of 412 nm with an excitation wavelength of 345 nm, and quantified using a standard curve of 2-naphthylamine (0–200 nmol/ml). Fluorimetric data from samples and standard curve were taken each minute. Specific aminopeptidase activities were calculated from the slope of the linear portion of enzymatic assay, and expressed as nanomol of substrate hydrolyzed per minute per mg of urine creatinine (mU/mg Cr) per ml of plasma or per mg of total protein content in renal tissue.
Histopathological Study
For conventional morphology, buffered 10% formaldehyde-fixed, paraffin-embedded longitudinal rat kidney sections in sagittal plane were deparaffinized in xylol (three passes of 5 min) and re-hydrated in ethanol of decreasing gradation (absolute, 96%, and 70%, 2 passes of 3 min, respectively). Tissue sections were stained with hematoxylin-eosin, Masson’s trichrome and periodic acid-Schiff (PAS). The presence of glomerular lesions (glomerulosclerosis, glomerular hyperplasia, mesangium increase, glomerular cyst, and capsular fibrosis) was assessed in at least 200 glomeruli. Tubulointerstitial damage (tubular vacuolation, tubular atrophy, hyaline drops, tubular casts, and chronic inflammatory infiltrate) was also studied. Injury was graded according to Shih, Hines, and Neilson on a semiquantitative scale of 0–4 (0 = normal, 0.5 = small focal areas of damage, 1 = involvement of less than 10% of the cortex, 2 = involvement of 10–25% of the cortex, 3 = involvement of 25–75% of the cortex, 4 = extensive damage involving more than 75% of the cortex) (Shih et al., 1988). The morphological study was done in blinded fashion on 4-micrometer sections with light microscopy, using the most appropriate stain for each lesion.
Morphometrical Study
Samples were fixed in buffered 10% formalin, embedded in paraffin and serially sectioned at 5 μm thickness. Afterwards, they were stained with 1% picro Sirius red F3BA (Gurr, BDH Chemicals Ltd., Poole, United Kingdom) for image analysis quantification. To improve staining, tissue sections were kept after deparaffination for 3–5 days in 70% alcohol as mordent. Picro Sirius red stains connective fibers deep red and cell nuclei and cytoplasmatic structures light red/bright yellow (Sweat et al., 1964). To semiautomatically quantify interstitial connective tissue, 20 images of cortex per kidney were acquired using an IF 550 green optical filter with illumination intensity values slightly above those used for normal observation with a digital camera 3CCD (DP70) coupled to an Olympus BX-42 microscope (Olympus Optical Company). 20 images of corticomedullary junction per kidney were acquired using polarized light. Histologic images of kidney biopsies were convert in black and white at 8-bit intensity resolution (256 gray levels) with a global magnification of 200×, and normalized with Adobe Photoshop software (Adobe Systems Software, Ireland). To assess the fibrosis, we made a macro that included a semiautomatic thresholding of the total of the images per group of rats simultaneously with ImageJ software (v 1.48) (National Institute of Health, United States).
Statistical Analyses
To study the time course of biological variables and urinary markers, we used a factorial ANOVA for repeated measures, taking each rat as the subject and the group (ZL or ZO) as the between-subjects factor. Interactions between factors were analyzed using Bonferroni method. We used StatGraphics Centurion XVII software.
Morphological and biological variables measured at the end of the experiment were compared using a t-test for the analysis of variables with normal distribution and equal variances. Welch modification of t-test was used for data with normal distribution and unequal variances and Mann–Whitney W (Wilcoxon) test was used to analyze the differences when data did not correspond to a normal distribution. Shapiro–Wilk test was used to analyze the normality of distributions. Differences were considered statistically significant at p < 0.05 level. We used StatGraphics Centurion XVII software.
Simple linear regressions were analyzed with Statgraphics Centurion XVII software. Multiple linear regressions were also analyzed with this software to establish the optimal correlation between urinary markers as independent variables and each renal lesion as dependent variable. The model of regression with best information criteria (Akaike information criteria) was selected. This model eliminates the markers that do not significantly contribute to regression, obtaining an optimal fitted model that includes all markers with a significant contribution to regression (p < 0.05).
For histopathological results IBM SPSS-Windows 20.0 (SPSS Inc., Chicago, IL, United States) was used for the analyses. Results are presented as mean ± standard error in the case of fibrosis, or median and interquartilic range for categorical data. t-test was used to compare percentage of fibrosis. Non-parametric Mann–Whitney U-test were used to compared morphological and histomorphometrical variables. Results were considered statistically significance when p-values were below 0.05.
Results
Time Course of Biological Variables
A comparison of the biological variables between ZO and ZL rats was performed (data not shown). The variables analyzed were: body weight, food intake, water intake, diuresis and water balance. Body weight was obviously greater in ZO with respect to ZL rats. Food intake was increased in ZO rats, whereas water intake was reduced along the study. Unexpectedly, diuresis was higher in ZO rats; and consequently, water balance was found reduced in these animals. The time course of glucosuria was not significantly different in ZO and ZL rats when expressed in concentration, but it was significantly increased from 6 to 8 months old when data were normalized by creatinine excretion.
Morphological Variables
Final body weight was significantly increased in ZO rats by 1.35-fold when compared to ZL rats. Kidney weight and heart weight in absolute values or relative to tibial length were increased in ZO rats when compared with the ZL group (Table 1).
Histopathological Results
Renal lesions in ZL group were absent. No glomerular, tubulointerstitial, or vascular lesions were present in renal parenchyma. In ZO rats moderate segmental increases in glomerular matrix, segmental collapse and obliteration of capillary lumina, and accumulation of hyaline were found. Such changes were frequently associated with fibrosis and synechial attachments to Bowman’s capsule. Tubulointerstitial injury was defined as inflammatory cell infiltrates, tubular dilation and/or atrophy, tubular casts or interstitial fibrosis, and tubular atrophy, tubular casts, and chronic inflammatory infiltrate were statistically significant increased in ZO rats (Table 2 and Figure 1).
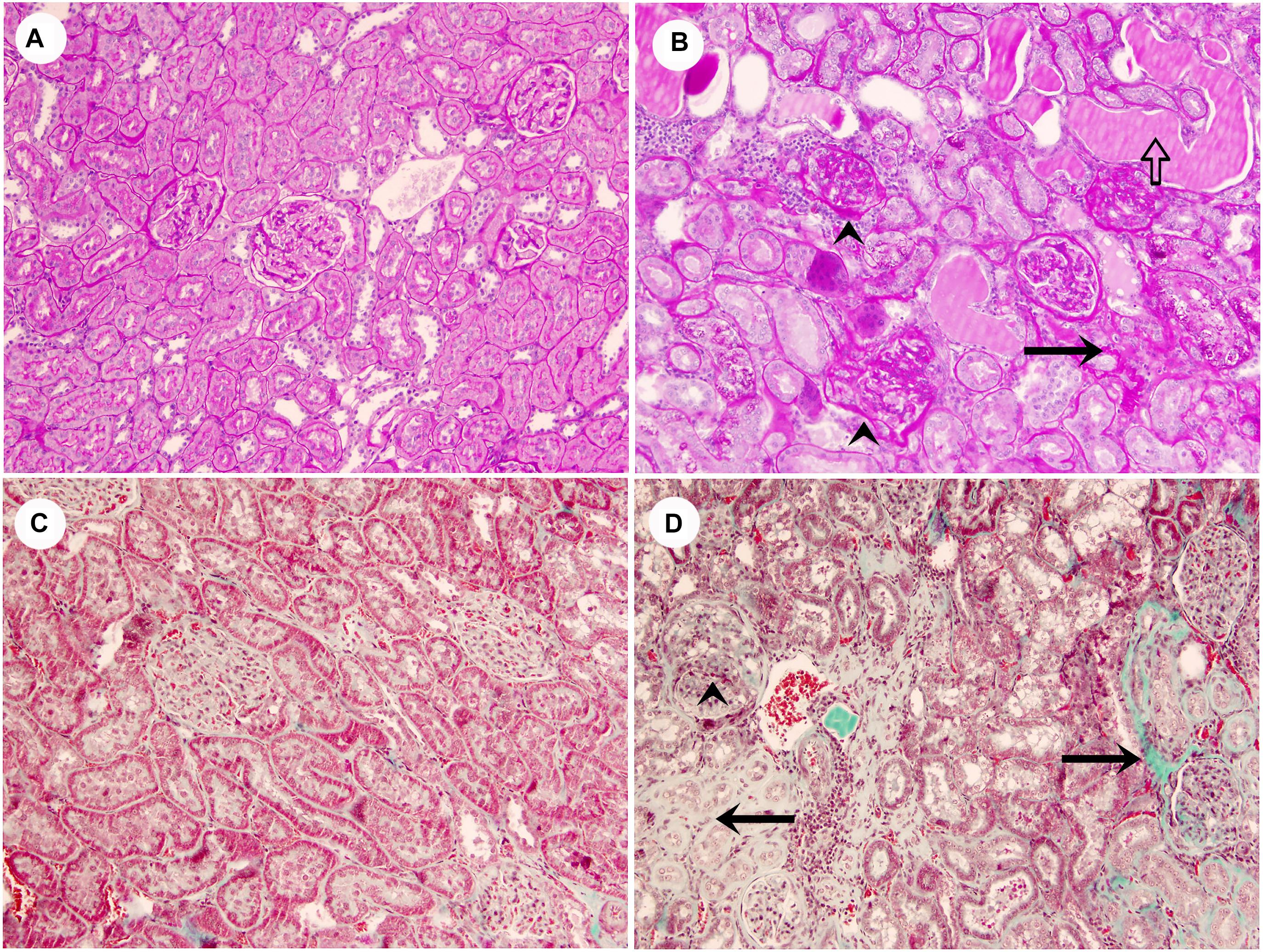
FIGURE 1. Representative images of lean rats without glomerular or tubulointerstitial injury (A,C), and obese rats with segmental glomerular sclerosis (ˆ), tubular atrophy (black arrows), tubular casts (white arrow) and inflammatory infiltrate (B,D) (A,B, PAS stain; C,D, Masson’s trichrome stain, original magnification 10×).
Plasma Variables and Creatinine Clearance at the End of the Study
Plasma urea and creatinine were decreased in ZO rats. Plasma glucose was not significantly different, probably because animals did not fast in order to obtain proper metabolic data. Creatinine clearance, expressed in absolute values or relative to g of kidney, was slightly increased in ZO rats, but did not reach statistical signification. These data are shown in Table 3.
Time-Course of Urinary Biomarkers
Figure 2 shows the evolution of urinary biomarkers along the study. Proteinuria and GluAp were augmented in ZO rat at 4, 6, 7, and 8 months old, when expressed relative to creatinine excretion (mg/mg of Cr), showing a progressive time-related increase. AlaAp was increased in ZO rats at 2, 4, 6, 7, and 8 months old, showing a U-shaped pattern of evolution. Hydroxyproline was significantly increased in ZO rats at 2, 4, 6, 7, and 8 months old.
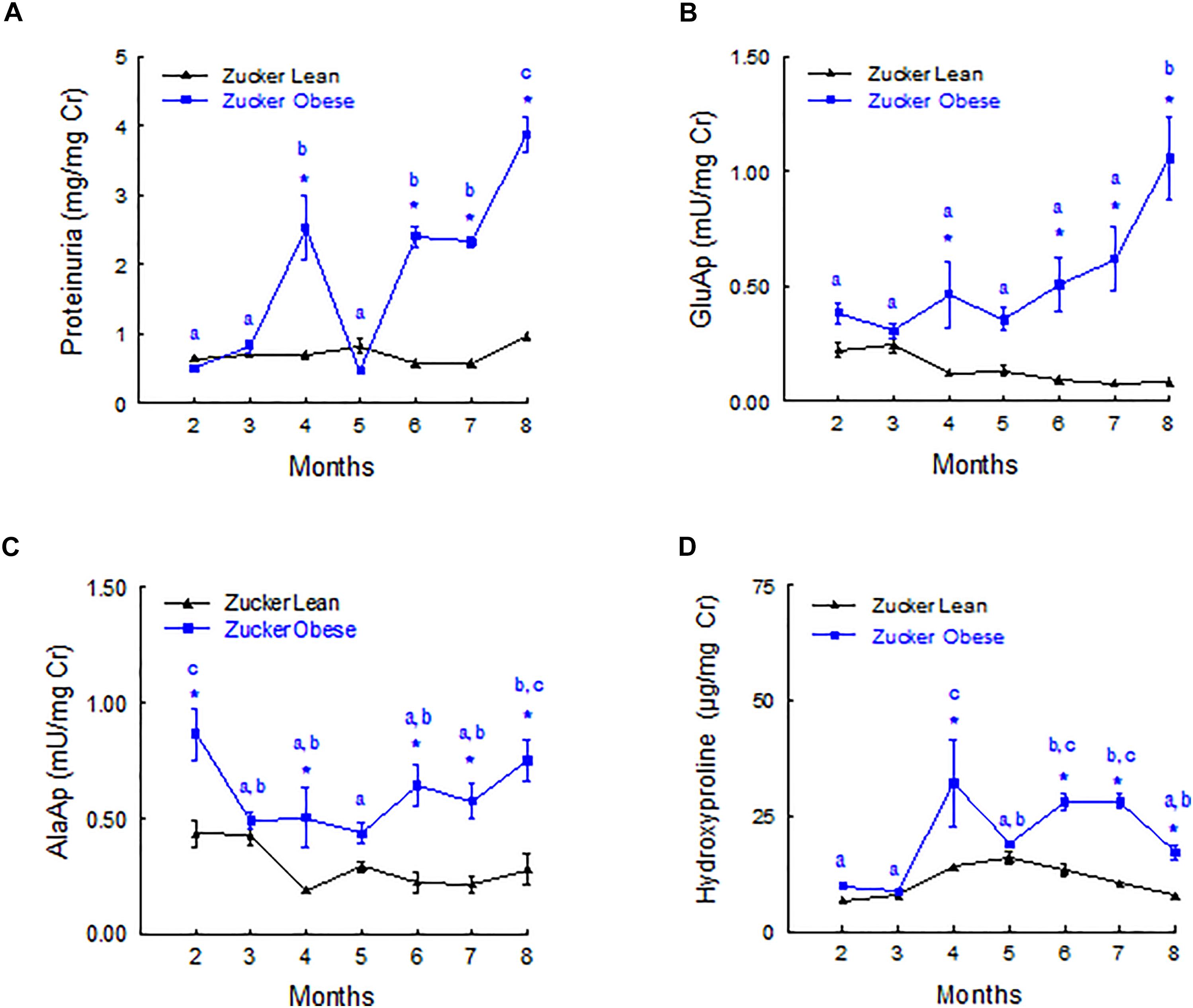
FIGURE 2. Time course of urinary biomarkers normalized by creatinine excretion. Proteinuria (A), GluAP (B), AlaAP (C), and hydroxyproline (D) in male Zucker lean and obese rats. Data are means ± SEM. ∗p < 0.05 compared with Zucker lean rats (n = 10, each group). Means with the same letter (a, b, or c) indicates sets of means within there are no significant difference at p < 0.05.
Plasma and Renal Aminopeptidasic Activities
GluAp and AlaAp enzymatic activities remained unchanged in the kidney of ZO rats at the end of the experiment. In plasma, there was a slight increase of GluAp activity in ZO rats, whereas plasmatic AlaAp activity remained unchanged (Table 4). The ratio of augmentation of GluAp activity in urine from ZO vs. ZL rats at 8 months old was 11.8 whereas the plasmatic ratio of augmentation was 1.43. Urinary AlaAp activity was nearly three times increased, even though there was no variation in its plasmatic activity.
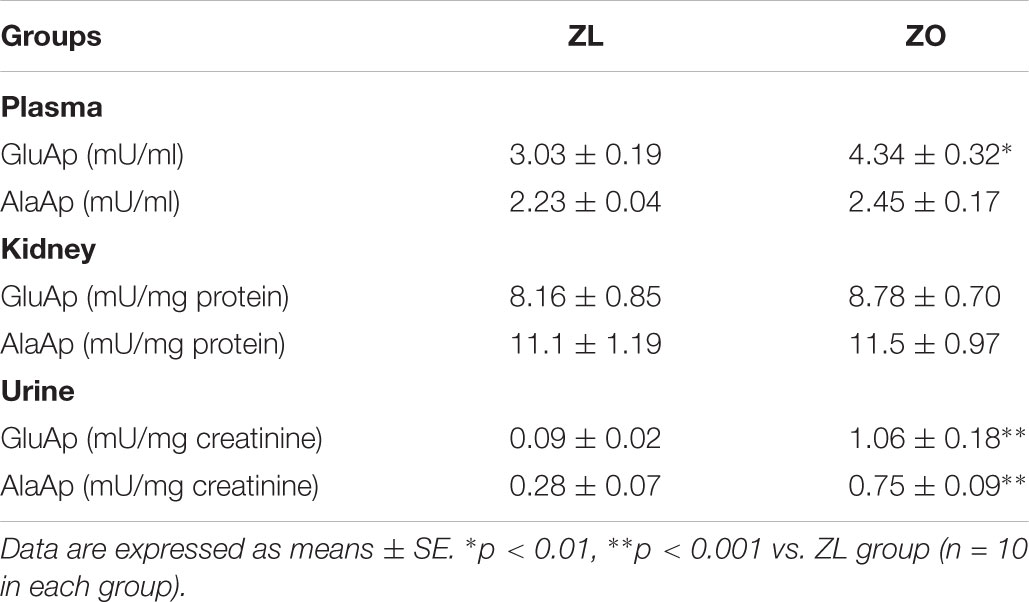
TABLE 4. Plasmatic, renal and urinary enzymatic activities of GluAp and AlaAp at 8 months old in male Zucker lean (ZL) and Zucker obese (ZO) rats.
Plasma, Urinary and Renal Klotho
The urinary excretion of Klotho was higher in ZO rats at 2, 5, and 8 months old when expressed as total daily excretion (ng/rat/day), urinary concentration (ng/ml) or relative to creatinine excretion (ng/mg Cr). The first two ways of expression are not presented. Plasma Klotho concentration was reduced to the half (p < 0.01) in ZO rats when compared with ZL group. The protein abundance of Klotho in renal tissue was similar in ZO and ZL rats. These data are shown in Figure 3 and Supplementary Figure S1.
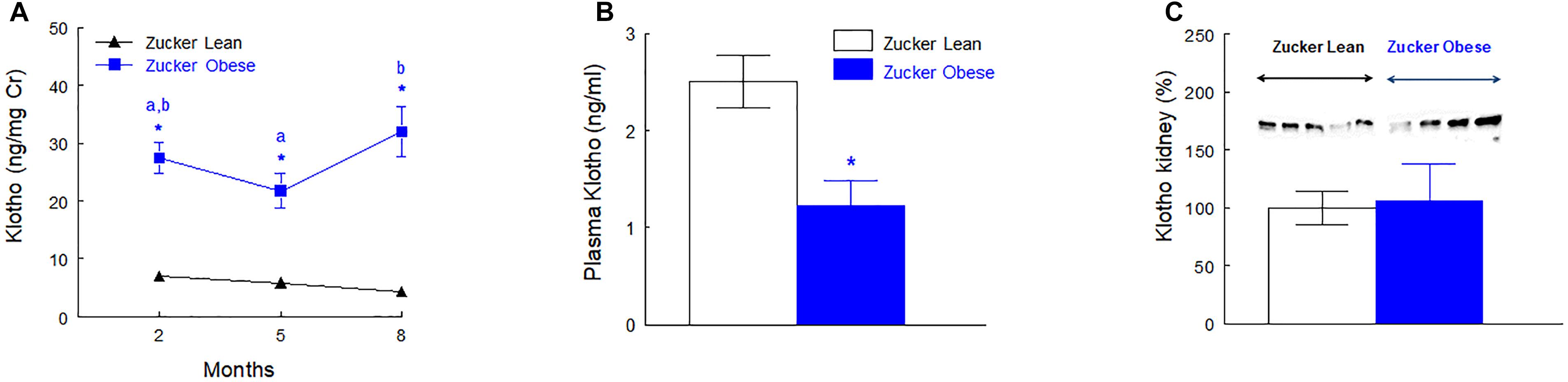
FIGURE 3. Urinary excretion of Klotho normalized by creatinine excretion (A), plasma levels of Klotho (B) at the end of the experiment (8 months old) and renal protein abundance of Klotho (C) in male Zucker lean and obese rats. Data are means ± SEM. ∗p < 0.05 compared with Zucker lean rats (n = 10, each group). Means with the same letter (a or b) indicates sets of means within there are no significant difference at p < 0.05.
Urinary Klotho showed positive correlations with proteinuria, urinary GluAp and urinary AlaAP excreted at 2, 5, and 8 months old, when all animals (ZL and ZO rats) were pooled in a common regression line, reaching the strongest correlation with urinary GluAp (Figure 4).
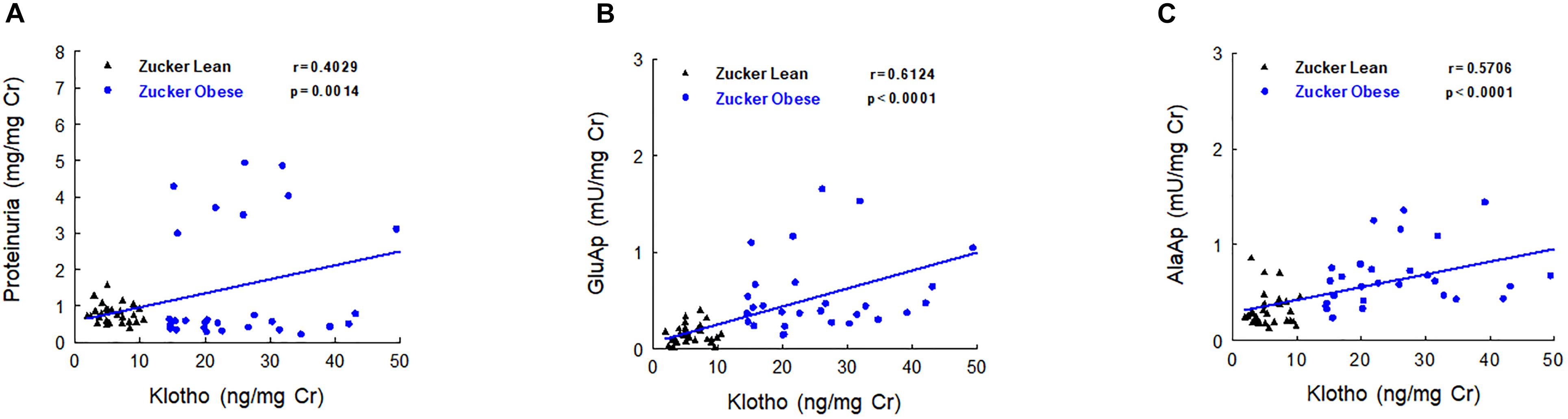
FIGURE 4. Correlations between urinary Klotho and proteinuria (A), urinary GluAp (B) and urinary AlaAP (C) excreted at 2, 5, and 8 months old, when all animals (lean and obese Zucker rats) were pooled in a common regression line.
Fibrosis Related Variables
Renal hydroxyproline content was increased in renal tissue of obese rats and morphometrical quantification with Sirius red stain and polarized light of renal cortex showed a significant increase of tubulointerstitial fibrosis in Zucker obese rats (p < 0.001). These data are displayed in Figure 5.
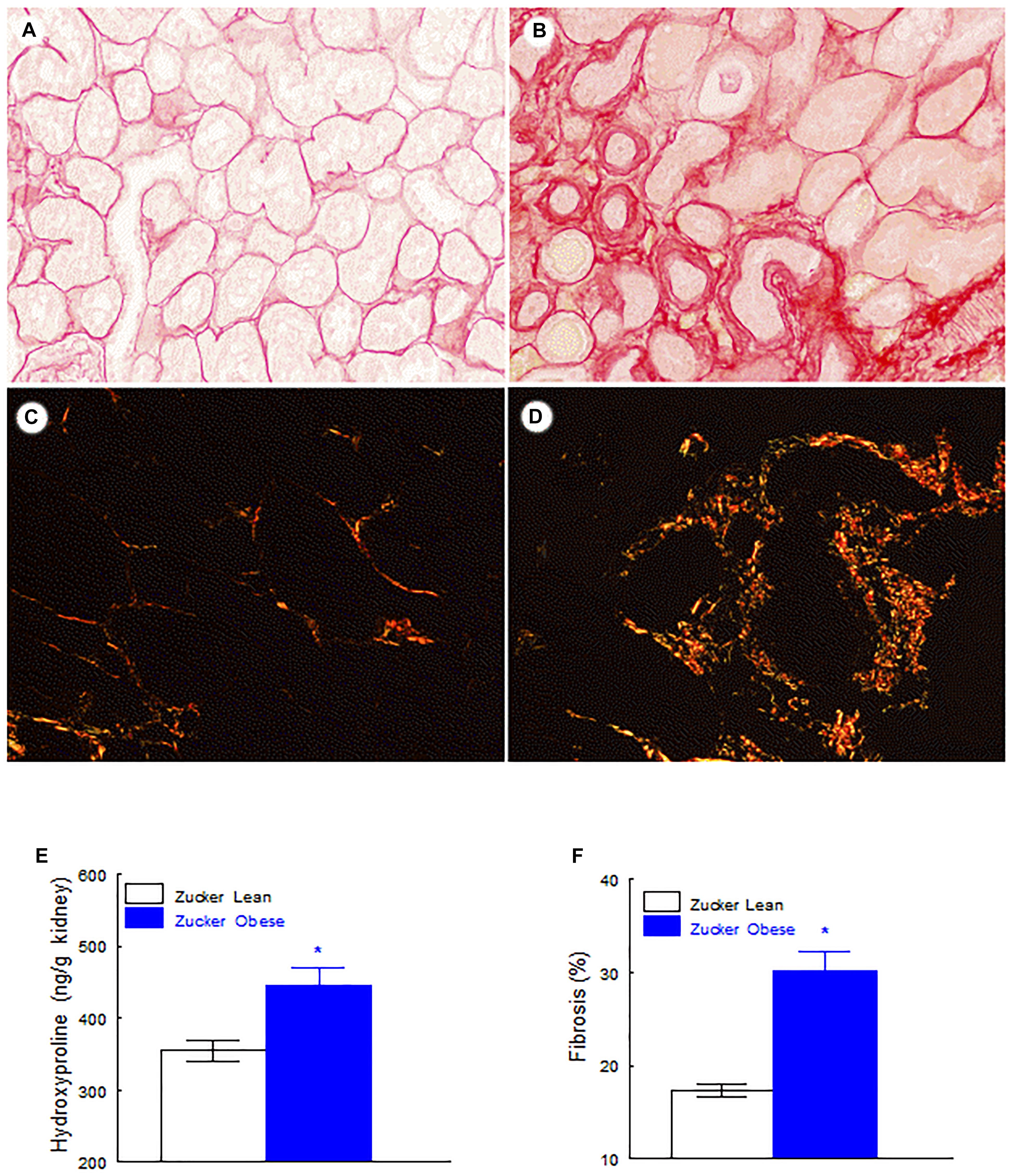
FIGURE 5. Fibrosis related variables. Renal level of fibrosis in Zucker lean rats (A,C) and Zucker obese rats (B,D), Note the significant increase of tubulointerstitial fibrosis in obese rats in comparison with lean rats (A,B Sirius red stain with light microscope, C,D Sirius red stain with polarized light, original magnification 20×). Renal hydroxyproline levels (E) and percentage of renal fibrosis (F). Data are means ± SEM. ∗p < 0.01 compared with Zucker lean rats (n = 10, each group).
Correlation Studies
Table 5 shows a multiple regression analysis that included GluAp, AlaAp, proteinuria, Klotho, and hydroxyproline excretion normalized per mg of creatinine in urine collected at 2 months old, GluAp, AlaAp, and Klotho or their combination were the markers that better predicted all renal lesions quantified at the end of the experiment. Results of multiple regression analysis at 5 months old were very similar to that of 2 months. GluAp, AlaAp, and Klotho were the best predictors of renal lesions (Table 6).
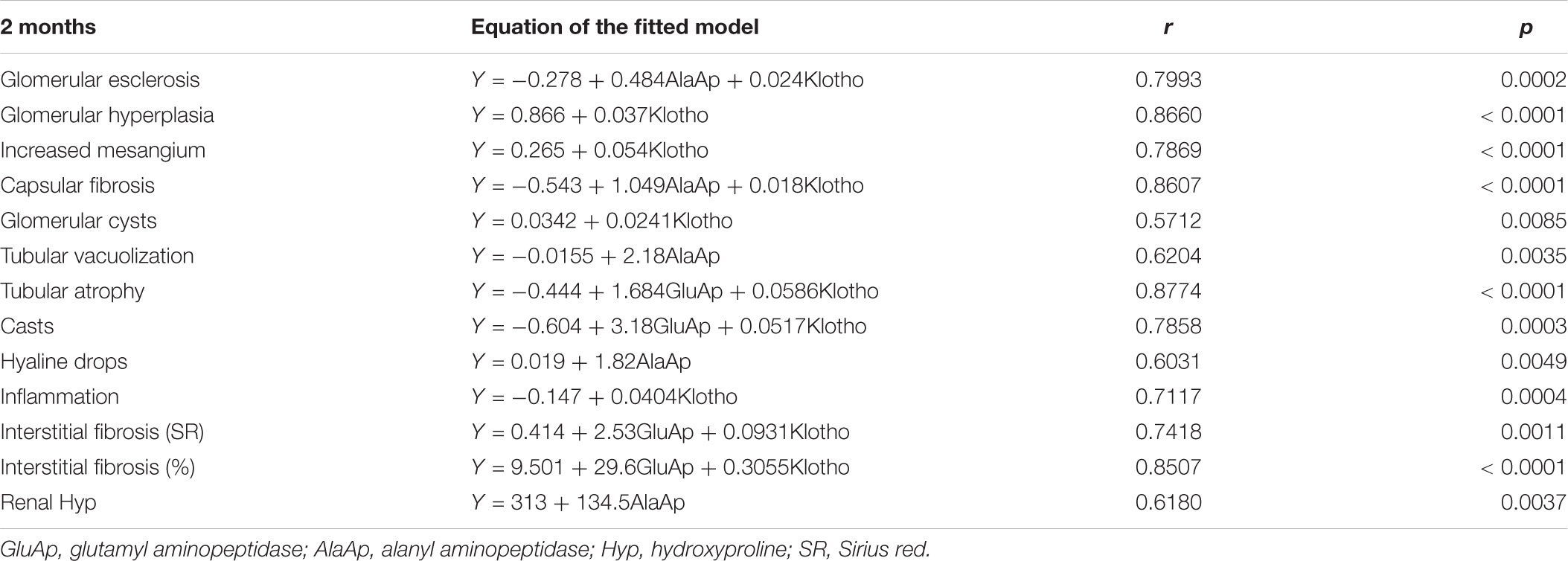
TABLE 5. Equation of the fitted model, correlation coefficient (r) and p-value of the multiple linear regression between urinary markers measured at 2 months and renal lesions at the end of the experiment (8 months old) in Zucker lean and Zucker obese rats (n = 20).
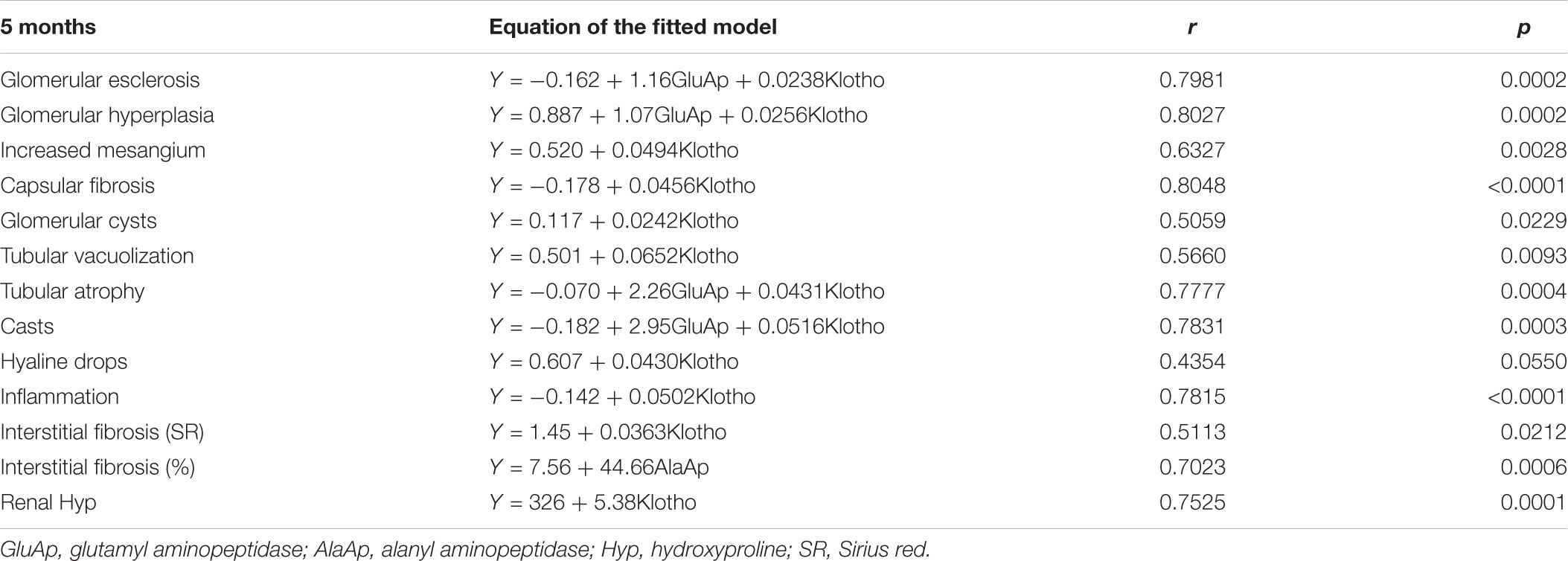
TABLE 6. Equation of the fitted model, correlation coefficient (r) and p-value of the multiple linear regression between urinary markers measured at 5 months and renal lesions at the end of the experiment (8 months old) in Zucker lean and Zucker obese rats (n = 20).
At 8 months old, proteinuria, hydroxyproline, AlaAp, and Klotho were the markers with the best correlations with renal lesions (Table 7). Moreover, we also found correlations with renal lesions at 2, 5, and 8 months old when urinary markers were normalized by total daily excretion, and even when they were expressed in urine concentration.
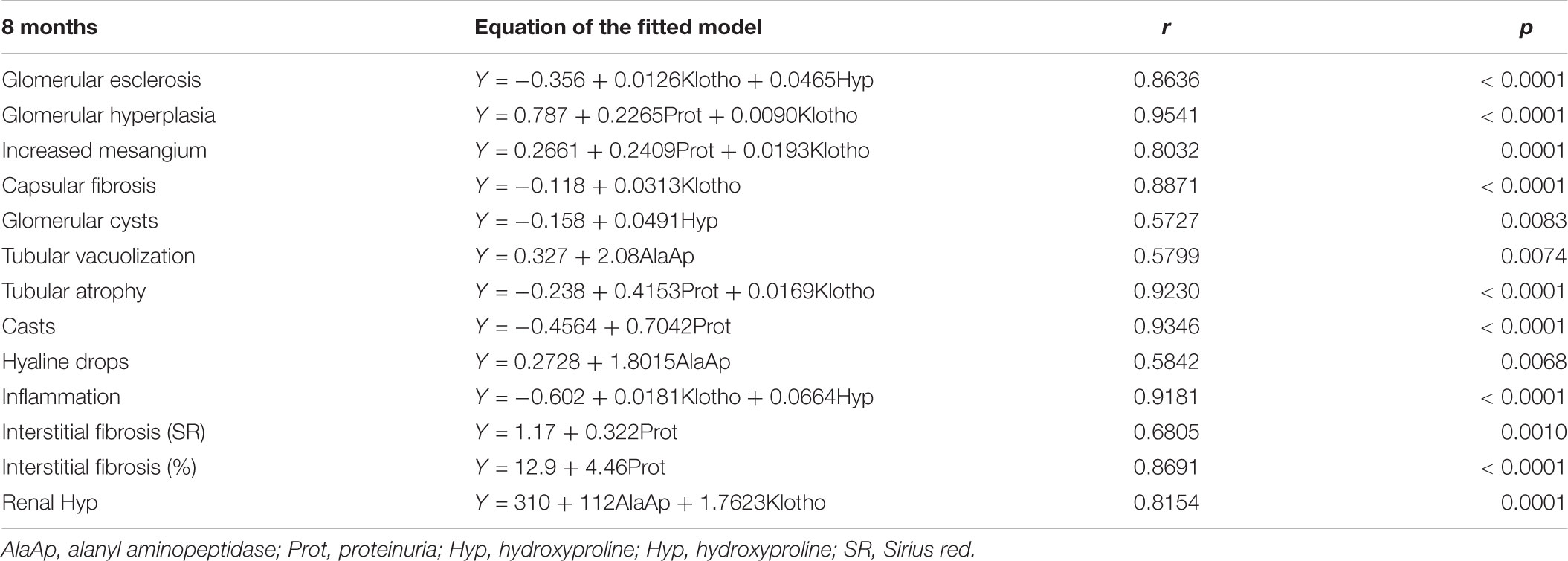
TABLE 7. Equation of the fitted model, correlation coefficient (r) and p-value of the multiple linear regression between urinary markers measured at 8 months and renal lesions at the end of the experiment (8 months old) in Zucker lean and Zucker obese rats (n = 20).
In order to study the prognostic value of urinary markers in ZO group, we carried out a multiple regression analysis between urinary markers at 2, 5, and 8 months old and the two continuous variables at the end of the experiment: renal fibrosis and renal hydroxyproline. At 2 months old, GluAp urinary activity was the marker that showed the highest predictive correlation with renal fibrosis in ZO group (Figure 6), indicating that this marker can have an added value as an early prognostic marker of the extent of fibrosis. Inclusion of ZL rats in the analysis made the correlation to be weaker, and no correlation was found if analysis was carried out using only ZL rats (Figure 6). These results can explain that urinary activity of GluAp at 2 months old was not found to be significantly increased in ZO rats, because some ZO rats have a low urinary activity, but they are the ones that exhibit lower levels of fibrosis at the end of the experiment. We did not find significant correlations with renal fibrosis for any other marker or their combinations at 5 or 8 months old when only ZO rats were used.
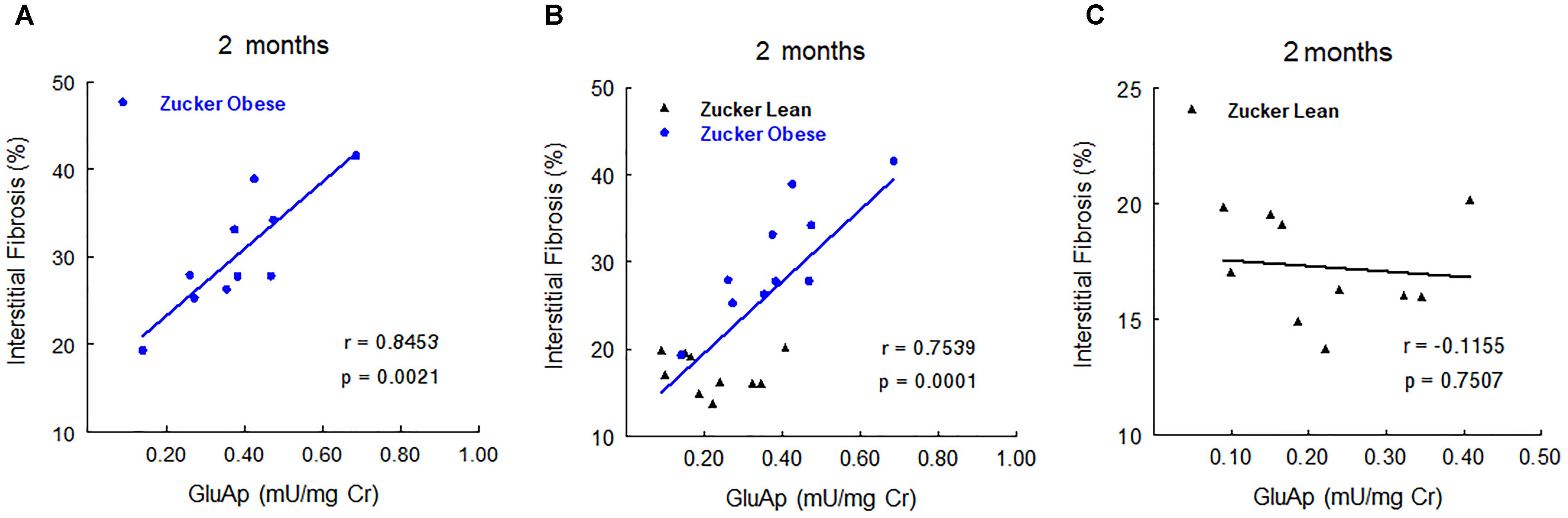
FIGURE 6. Predictive correlations of urinary GluAp activity at 2 months old with interstitial fibrosis at the end of the experiment in ZO rats (A, n = 10), in ZL and ZO rats (B, n = 20) and in ZL rats (C, n = 10).
Nevertheless, at 5 and 8 months old we found significant correlations with renal hydroxyproline content. At 5 months old, the combination of urinary AlaAp activity and Klotho showed the maximal prognostic correlation (Figure 7), while the combination of GluAp and Klotho was the marker that best fitted with renal hydroxyproline content at 8 months old (Figure 8).
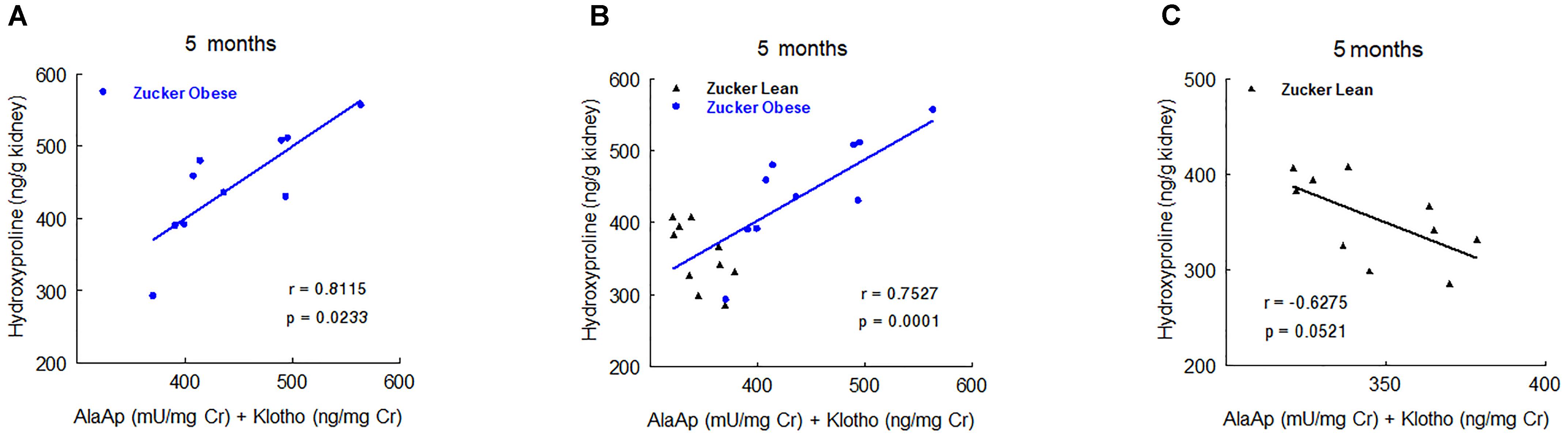
FIGURE 7. Predictive correlations of the combination of AlaAp and Klotho at 5 months old with renal hydroxyproline content at the end of the experiment in ZO rats (A, n = 10), in ZL and ZO rats (B, n = 20) and in ZL rats (C, n = 10).
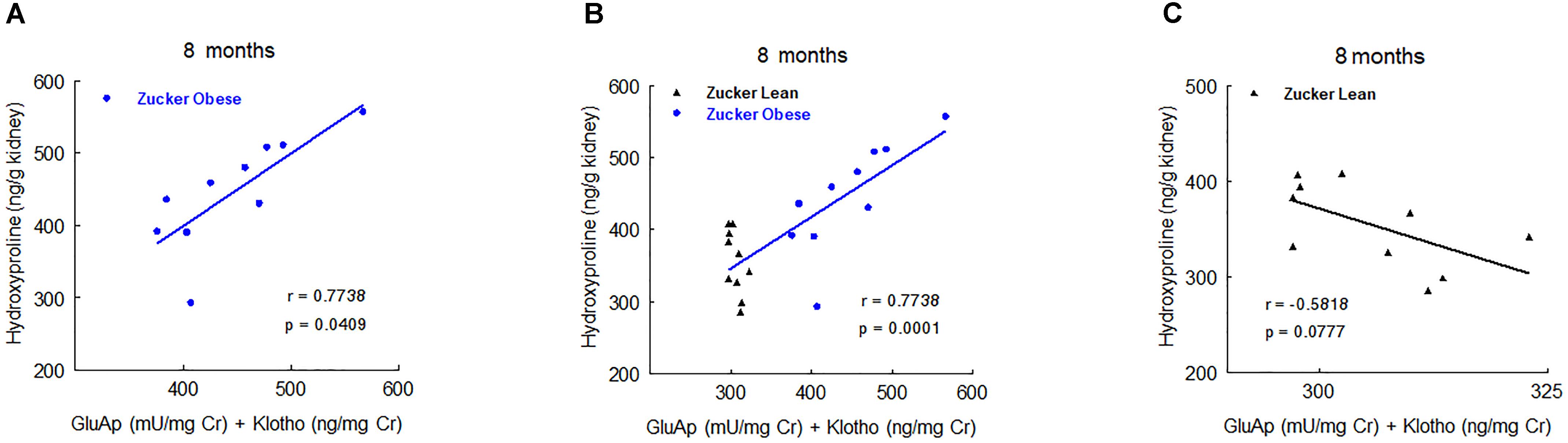
FIGURE 8. Predictive correlations of the combination of GluAp and Klotho at 8 months old with renal hydroxyproline content at the end of the experiment in ZO rats (A, n = 10), in ZL and ZO rats (B, n = 20) and in ZL rats (C, n = 10).
Altogether, these results demonstrate that GluAp, AlaAp, and Klotho are related with the severity of renal lesions even when animals are 2 months old, and they can serve not only to differentiate these lesions between ZO and ZL rats but also to establish a prognostic of these lesions inside ZO group.
Discussion
This study clearly shows for first time that obesity of Zucker rats is associated to increased urinary glutamyl and alanyl aminopeptidasic activities and to increased urinary excretion of Klotho, changes that were detected as early as 2 months old. This study also shows that urinary aminopeptidases and urinary Klotho correlates with the morphological changes observed in the renal injury of the ZO rats analyzed at the end of the experimental period. Moreover, renal hydroxyproline content and tubulointerstitial fibrosis were increased in the renal tissue of obese rats.
The present paper shows that kidneys from ZO rats at 8 months old developed mild focal and segmental glomerulosclerosis as well as moderate tubulointerstitial injury, data that are in consonance with those reported by other authors in this type of experimental obesity (Kasiske et al., 1985, 1992; Magil, 1995; Coimbra et al., 2000; Gassler et al., 2001; D’Agati et al., 2016). It is interesting to note that despite to these histopathological findings of renal injury in ZO rats, the classic biomarkers of renal disease –plasma urea and creatinine and creatinine clearance are normal or even better than normal in our study, as well as in the literature (Lavaud et al., 1996; Coimbra et al., 2000; Gassler et al., 2001), indicating that structural renal changes might be present before the manifestation of renal disease and that the classic biomarkers of renal disease are not useful tools for the evaluation of obesity induced renal damage. In fact, Coimbra et al. (2000) reported that plasma creatinine and urea remained normal during the observation period until week 40, and they started to increase at a later stage of renal injury, when Zucker obese rats were 60 weeks old.
The data concerning urinary aminopeptidasic activities are in consonance with previous papers, where we showed that urinary activity of these enzymes were associated to the development of renal damage in hypertensive hyperthyroid rats under high salt intake, showing that urinary aminopeptidasic activity correlates with the degree of renal injury but not with plasma renin activity or angiotensin II plasma levels (Pérez-Abud et al., 2011) and in cisplatin-treated rats (Quesada et al., 2012). In these studies, AlaAp and GluAp activities also were manifested as early and predictive biomarkers of the presence and severity of renal injury (Quesada et al., 2012; Montoro-Molina et al., 2015). These enzymes reach urine from damaged tubular cells, and they can detect proximal tubular injury regardless of the glomerular filtration status (Quesada et al., 2017).
Data obtained from the Prevention of Renal and Vascular End Stage Disease (PREVEND) study demonstrate that albuminuria augmented during the development of diabetic kidney disease and that, in addition, is a marker of the progression of diabetes (Brantsma et al., 2005). In our study, ZO rats also shows increased proteinuria that started at 4 months old, at 2 months of the observational period. However, other authors that also studied the time course of proteinuria in ZO rats reported the appearance of proteinuria in later stages of this type of obesity, e.g., 10 months of age (Michel et al., 1997; Coimbra et al., 2000; Gassler et al., 2001). Very recently, Mima et al. (2018) have observed that the appearance of albuminuria can be accelerated when ZO rats are fed with high-fat chow.
Proteinuria from the classic point of view is secondary to alterations of the glomerular barrier membrane, in fact podocyte injury underlies the progression of focal segmental glomerulosclerosis in the fa/fa Zucker rat (Gassler et al., 2001), but now it is also well known that, under physiological conditions, 7–9 g/day of proteins can cross the glomerular barrier membrane and appeared in primary urine in humans (Haraldsson and Sörensson, 2004). These proteins are uptaked by tubular cells (Haraldsson et al., 2008) by the megalin-cubulin complex (Christensen and Birn, 2001) and degraded and fragmented in the tubule (Eppel et al., 2001). Thus, lysine has been administered to produce proteinuria by inhibiting tubular cells (Tencer et al., 1998). Hence, it is possible that, at least, a part of the proteinuria observed in ZO rats may arise from a tubular injury, as indicated the increased urinary levels of aminopeptidases -localized in the brush border of tubular cells- that precede the appearance of proteinuria. Therefore, an early tubular injury could determine a defective tubular uptake of proteins. In consonance with this, Osicka et al. (2000) reported that the proteinuria that occurs with diabetic nephropathy reflects defects in the tubular system.
This study shows increased urinary levels of Klotho, a reduction in plasma and normal values of protein abundance in the renal tissue. These last data agree with the results of Lorenzi et al. (2010) that found no differences in klotho mRNA levels between obese Zucker and control rats, neither in kidneys nor in other organs; and that Klotho protein levels tested by Western blot were similar in kidneys from ZO and control rats. However, the present results contrast with the decreased renal gene expression of Klotho observed in various animal models of vascular and metabolic diseases (Nagai et al., 2000). Moreover, our results also contrast with previous reports in other renal diseases, where plasma, renal and urinary Klotho go in the same direction, considering that serum and urinary Klotho can be surrogate markers for renal Klotho production (Hu et al., 2011).
The possible explanation for the absence of uniformity of our Klotho data is that tubular injury determines an increased urinary excretion of Klotho as happens with aminopeptidases that are released to urine. This wasting of Klotho through the urine, might contributes to the reduced plasma level, since urinary αKlotho, at least in part, may arise from the plasma. Thus, exogenously injected labeled αKlotho was detected in the urine of rats (Hu et al., 2016). In support of our hypothesis is the positive correlation between urinary aminopeptidases and urinary Klotho, indicating that Klotho and aminopeptidases probably are augmented in urine due to the presence of damaged tubular cells.
As reported in the Introduction section, plasma soluble Klotho may operate as an endocrine agent on distant organs (Hu et al., 2013, 2015), with many cardiovascular and protective effects (Hu et al., 2013, 2015; Lu and Hu, 2017). Thus, the reduced plasma levels of Klotho might contribute to the cardiovascular and renal abnormalities observed in ZO rats, since reduced circulating Klotho also have been reported in other renal (Hu et al., 2010b, 2011, 2012; Akimoto et al., 2012; Scholze et al., 2014) or CVDs (Wang and Sun, 2009; Yu et al., 2010), including diabetes in humans and mice (Zhao et al., 2011; Asai et al., 2012).
Urinary hydroxyproline excretion has been related in previous works with the development of different renal lesions including fibrosis in a murine model of nephrotoxicity evoked with melamine and cyanuric acid (Schnackenberg et al., 2012). In our study, urinary hydroxiproline was increased in ZO rats from two 2 months old, although our data demonstrate that the excretion of Klotho, GluAp, and AlaAp activities at 2 and 5 months old correlate better than hydroxyproline even with renal fibrosis or renal hydroxyproline content. At 8 months old, urinary hydroxyproline excretion takes relevance as a diagnostic marker of glomerular sclerosis, glomerular cysts and inflammation, but not for renal fibrosis or renal hydroxyproline content. Furthermore, in our study, urinary hydroxyproline was not a prognostic marker, because it did not show any correlation with these variables in ZO rats. This lack of correlation might be due to the fact that urinary hydroxyproline mainly reflects plasmatic levels of this amino acid, because it is freely filtered in glomerulus, although hydroxyproline derived from collagen of renal tissue can also contribute to increase its content in urine, explaining the higher excretion of this marker in ZO rats when compared with ZL rats.
The correlation studies shown in Tables 5, 6 demonstrate that GluAp, AlaAp, and Klotho are early diagnostic markers of renal lesions in ZO rats. Proteinuria and hydroxyproline can be considered delayed diagnostic markers because their contribution to diagnosis starts later, probably when glomerular and fibrotic lesions are more extended. It is interesting to note that all urinary markers were normalized per creatinine excretion because it allows analyzing spot samples in clinical practice without the needing of collecting 24-h urine and it is the most used way to quantify urinary markers, but we have obtained similar correlations when markers were expressed in total daily excretion or even in urinary concentration.
Another relevant result is that GluAp, AlaAp and Klotho are related not only with diagnosis but also with prognosis of renal lesions in Zucker obese rats. Results reported in Figures 6–8 show strong predictive correlations with percentage of renal fibrosis or with renal hydroxyproline content at the end of the experiment, indicating that an early increased excretion of these markers is related with a higher later extent of fibrosis in ZO rats. Finally, we also want to pointed out that the correlations observed between tubular urinary biomarkers and the morphological signs of renal injury, do not establish any causal relationship among them.
Conclusion
Urinary aminopeptidases and Klotho are early diagnostic biomarkers of renal injury, and urinary levels of these biomarkers are also related with the extent of renal fibrosis in Zucker obese rats. Measurements of GluAp, AlaAp, and Klotho may represent a novel, specific and non-invasive diagnostic approach to assess kidney fibrosis and may have a potential use not only in diagnosis but also in prognosis of obesity-associated renal lesions.
Author Contributions
AO, FV, and RW: conception and design of the experiments. AL-C, AQ, FO’V, NM-M, SM-M, and RW: collection, analysis and interpretation of the data. FO’V, AO, FV, and RW: drafting the article or revising it critically for important intellectual content. All authors reviewed and approved the final version of the manuscript.
Funding
This study was supported by the grants PI13/02743 and PI13/02384 from the Carlos III Health Institute of Spain, and the Red de Investigación Renal REDinREN RD16/0009/0033 “FEDER una manera de hacer Europa”.
Conflict of Interest Statement
RW, FV, and AO are co-authors of the patent “Glutamyl aminopeptidase as a marker of renal damage” with publication number 2382960. There is not any other relevant declaration related to employment, consultancy, patents, products in development or modified products.
The remaining authors declare that the research was conducted in the absence of any commercial or financial relationships that could be construed as a potential conflict of interest.
Acknowledgments
Technical and human support provided by CICT of Universidad de Jaén (UJA, MINECO, Junta de Andalucía, FEDER) was gratefully acknowledged. We thank the technical assessment of María Dolores Rodríguez. We also thank to Richard Davies for help with the English version.
Supplementary Material
The Supplementary Material for this article can be found online at: https://www.frontiersin.org/articles/10.3389/fphys.2018.01599/full#supplementary-material
References
Akimoto, T., Yoshizawa, H., Watanabe, Y., Numata, A., Yamazaki, T., Takeshima, E., et al. (2012). Characteristics of urinary and serum soluble Klotho protein in patients with different degrees of chronic kidney disease. BMC Nephrol. 13:155. doi: 10.1186/1471-2369-13-155
Albiston, A. L., Yeatman, H. R., Pham, V., Fuller, S. J., Diwakarla, S., Fernando, R. N., et al. (2011). Distinct distribution of GLUT4 and insulin regulated aminopeptidase in the mouse kidney. Regul. Pept. 166, 83–89. doi: 10.1016/j.regpep.2010.09.003
Asai, O., Nakatani, K., Tanaka, T., Sakan, H., Imura, A., Yoshimoto, S., et al. (2012). Decreased renal a-Klotho expression in early diabetic nephropathy in humans and mice and its possible role in urinary calcium excretion. Kidney Int. 81, 539–547. doi: 10.1038/ki.2011.423
Brantsma, A. H., Bakker, S. J., Hillege, H. L., de Zeeuw, D., de Jong, P. E., Gansevoort, R. T., et al. (2005). Urinary albumin excretion and its relation with C-reactive protein and the metabolic syndrome in the prediction of type 2 diabetes. Diabetes Care 28, 2525–2530. doi: 10.2337/diacare.28.10.2525
Chen, C. D., Li, H., Liang, J., Hixson, K., Zeldich, E., and Abraham, C. R. (2015). The anti-aging and tumor suppressor protein klotho enhances differentiation of a human oligodendrocytic hybrid cell line. J. Mol. Neurosci. 55, 76–90. doi: 10.1007/s12031-014-0336-1
Christensen, E. I., and Birn, H. (2001). Megalin and cubilin: synergistic endocytic receptors in renal proximal tubule. Am. J. Physiol. Renal Physiol. 280,F562–F573. doi: 10.1152/ajprenal.2001.280.4.F562
Coimbra, T. M., Janssen, U., Grone, H. J., Ostendorf, T., Kunter, U., Schmidt, H., et al. (2000). Early events leading to renal injury in obese Zucker (fatty) rats with type II diabetes. Kidney Int. 57, 167–182. doi: 10.1046/j.1523-1755.2000.00836.x
de Artinano, A. A., and Castro, M. M. (2009). Experimental rat models to study the metabolic syndrome. Br. J. Nutr. 102, 1246–1253. doi: 10.1017/S0007114509990729
D’Agati, V. D., Chagnac, A., de Vries, A. P., Levi, M., Porrini, E., Herman-Edelstein, M., et al. (2016). Obesity-related glomerulopathy: clinical and pathologic characteristics and pathogenesis. Nat. Rev. Nephrol. 12, 453–471. doi: 10.1038/nrneph.2016.75
Eppel, G. A., Osicka, T. M., Pratt, L. M., Jablonski, P., Howden, B., Glasgow, E. F., et al. (2001). The return of glomerular filtered albumin to the rat renal vein—the albumin retrieval pathway. Renal Failure 23, 347–363. doi: 10.1081/JDI-100104719
Erben, R. G. (2016). Update on FGF23 and klotho signaling. Mol. Cell. Endocrinol. 432, 56–65. doi: 10.1016/j.mce.2016.05.008
Gagliardini, E., Perico, N., Rizzo, P., Buelli, S., Longeretti, L., Perico, L., et al. (2013). Angiotensin II contributes to diabetic renal dysfunction in rodents and humans via Notch1/Snail pathway. Am. J. Pathol. 183, 119–130. doi: 10.1016/j.ajpath.2013.03.025
Gassler, N., Elger, M., Kränzlin, B., Kriz, W., Gretz, N., Hähnel, B., et al. (2001). Podocyte injury underlies the progression of focal segmental glomerulosclerosis in the fa/fa Zucker rat. Kidney Int. 60, 106–116. doi: 10.1046/j.1523-1755.2001.00777.x
Haraldsson, B., Nystrom, J., and Deen, W. M. (2008). Properties of the glomerular barrier and mechanisms of proteinuria. Physiol. Rev. 88, 451–487. doi: 10.1152/physrev.00055.2006
Haraldsson, B., and Sörensson, J. (2004). Why do we not all have proteinuria? An update of our current understanding of the glomerular barrier. News Physiol. Sci. 19, 7–10. doi: 10.1152/nips.01461.2003
Hu, M. C., Kuro, O. M., and Moe, O. W. (2012). Secreted Klotho and chronic kidney disease. Adv. Exp. Med. Biol. 728, 126–157. doi: 10.1007/978-1-4614-0887-1_9
Hu, M. C., Kuro-o, M., and Moe, O. W. (2013). Renal and extrarenal actions of Klotho. Semin. Nephrol. 33, 118–129. doi: 10.1016/j.semnephrol.2012.12.013
Hu, M. C., Shi, M., Cho, H. J., Adams-Huet, B., Paek, J., Hill, K., et al. (2015). Klotho and phosphate are modulators of pathologic uremic cardiac remodeling. J. Am. Soc. Nephrol. 26, 1290–1302. doi: 10.1681/ASN.2014050465
Hu, M. C., Shi, M., Zhang, J., Addo, T., Cho, H. J., Barker, S. L., et al. (2016). Renal production, uptake, and handling of circulating αKlotho. J. Am. Soc. Nephrol. 27, 79–90. doi: 10.1681/ASN.2014101030
Hu, M. C., Shi, M., Zhang, J., Pastor, J., Nakatani, T., Lanske, B., et al. (2010a). Klotho: a novel phosphaturic substance acting as an autocrine enzyme in the renal proximal tubule. FASEB J. 24, 3438–3450. doi: 10.1096/fj.10-154765
Hu, M. C., Shi, M., Zhang, J., Quiñones, H., Griffith, C., Kuro-o, M., et al. (2011). Klotho deficiency causes vascular calcification in chronic kidney disease. J. Am. Soc. Nephrol. 22, 124–136. doi: 10.1681/ASN.2009121311
Hu, M. C., Shi, M., Zhang, J., Quiñones, H., Kuro-o, M., and Moe, O. W. (2010b). Klotho deficiency is an early biomarker of renal ischemia- reperfusion injury and its replacement is protective. Kidney Int. 78, 1240–1251. doi: 10.1038/ki.2010.328
Ide, N., Olauson, H., Sato, T., Densmore, M. J., Wang, H., Hanai, J. I., et al. (2016). In vivo evidence for a limited role of proximal tubular Klotho in renal phosphate handling. Kidney Int. 90, 348–362. doi: 10.1016/j.kint.2016.04.009
Ito, S., Kinoshita, S., Shiraishi, N., Nakagawa, S., Sekine, S., Fujimori, T., et al. (2000). Molecular cloning and expression analyses of mouse beta klotho, which encodes a novel Klotho family protein. Mech. Dev. 98, 115–119. doi: 10.1016/S0925-4773(00)00439-1
Kasiske, B. L., Cleary, M. P., O’Donnell, M. P., and Keane, W. F. (1985). Effects of obesity on renal structure and function in the Zucker rat. J. Lab. Clin. Med. 106, 598–604.
Kasiske, B. L., O’Donnell, M. P., and Keane, W. F. (1992). The Zucker rat model of obesity, insulin resistance, hyperlipidemia, and renal injury. Hypertension 19, I110–I115. doi: 10.1161/01.HYP.19.1_Suppl.I110
Kenny, A. J., and Maroux, S. (1982). Topology of microvillar membrane hydrolases of kidney, and intestine. Physiol. Rev. 62, 91–128. doi: 10.1152/physrev.1982.62.1.91
Kuro-o, M., Matsumura, Y., Aizawa, H., Kawaguchi, H., Suga, T., Utsugi, T., et al. (1997). Mutation of the mouse klotho gene leads to a syndrome resembling ageing. Nature 390, 45–51. doi: 10.1038/36285
Lavaud, S., Michel, O., Sassy-Prigent, C., Heudes, D., Bazin, R., Bariety, J., et al. (1996). Early influx of glomerular macrophages precedes glomerulosclerosis in the obese Zucker rat model. J. Am. Soc. Nephrol. 7, 2604–2615.
Lindberg, K., Amin, R., Moe, O. W., Hu, M. C., Erben, R. G., Ostman, W. A., et al. (2014). The kidney is the principal organ mediating klotho effects. J. Am. Soc. Nephrol. 25, 2169–2175. doi: 10.1681/ASN.2013111209
Lisowska-Myjak, B. (2010). Serum and urinary biomarker of acute kidney injury. Blood Purif. 29, 357–365. doi: 10.1159/000309421
Lorenzi, O., Veyrat-Durebex, C., Wollheim, C. B., Villemin, P., Rohner-Jeanrenaud, F., Zanchi, A., et al. (2010). Evidence against a direct role of klotho in insulin resistance. Pflugers Arch. Eur. J. Physiol. 459, 465–473. doi: 10.1007/s00424-009-0735-2
Lu, X., and Hu, M. C. (2017). Klotho/FGF23 Axis in chronic kidney disease and cardiovascular disease. Kidney Dis. 3, 15–23. doi: 10.1159/000452880
Magil, A. B. (1995). Tubulointerstitial lesions in young Zucker rats. Am. J. Kidney Dis. 25, 478–485. doi: 10.1016/0272-6386(95)90112-4
Michel, O., Heudes, D., Lamarre, I., Masurier, C., Lavau, M., Bariety, J., et al. (1997). Reduction of insulin and triglycerides delays glomerulosclerosis in obese Zucker rats. Kidney Int. 52, 1532–1542. doi: 10.1038/ki.1997.483
Mima, A., Yasuzawa, T., King, G. L., and Ueshima, S. (2018). Obesity-associated glomerular inflammation increases albuminuria without renal histological changes. FEBS Open Bio 8, 664–670. doi: 10.1002/2211-5463.12400
Mitobe, M., Yoshida, T., Sugiura, H., Shirota, S., Tsuchiya, K., and Nihei, H. (2005). Oxidative stress decreases klotho expression in a mouse kidney cell line. Nephron Exp. Nephrol. 101, e67–e74. doi: 10.1159/000086500
Montoro-Molina, S., Quesada, A., Zafra-Ruiz, P. V., O’Valle, F., Vargas, F., de Gracia, M. C., et al. (2015). Immunological detection of glutamyl aminopeptidase in urine samples from cisplatin-treated rats. Proteomics Clin. Appl. 9, 630–635. doi: 10.1002/prca.201400096
Nagai, R., Saito, Y., Ohyama, Y., Aizawa, H., Suga, T., Nakamura, T., et al. (2000). Endothelial dysfunction in the klotho mouse and downregulation of klotho gene expression in various animal models of vascular and metabolic diseases. Cell Mol. Life Sci. 57, 738–746. doi: 10.1007/s000180050038
Osicka, T. M., Houlihan, C. A., Chan, J. G., Jerums, G., and Comper, W. D. (2000). Albuminuria in patients with type 1 diabetes is directly linked to changes in the lysosome-mediated degradation of albumin during renal passage. Diabetes Metab. Res. Rev. 49, 1579–1584. doi: 10.2337/diabetes.49.9.1579
Pérez-Abud, R., Rodríguez-Gómez, I., Villarejo, A. B., Moreno, J. M., Wangensteen, R., Tassi, M., et al. (2011). Salt sensitivity in experimental thyroid disorders in rats. Am. J. Physiol. Endocrinol. Metab. 301, E281–E287. doi: 10.1152/ajpendo.00690.2010
Quesada, A., Segarra, A. B., Montoro-Molina, S., de Gracia, M. C., Osuna, A., O’Valle, F., et al. (2017). Glutamyl aminopeptidase in microvesicular and exosomal fractions of urine is related with renal dysfunction in cisplatin-treated rats. PLoS One 12:e0175462. doi: 10.1371/journal.pone.0175462
Quesada, A., Vargas, F., Montoro-Molina, S., O’Valle, F., and Rodríguez-Martínez, M. D. (2012). Urinary aminopeptidase activities as early and predictive biomarkers of renal dysfunction in cisplatin-treated rats. PLoS One 7:e40402. doi: 10.1371/journal.pone.0040402
Schnackenberg, L. K., Sun, J., Pence, L. M., Bhattacharyya, S., Gamboa da Costa, G., and Beger, R. D. (2012). Metabolomics evaluation of hydroxyproline as a potential marker of melamine and cyanuric acid nephrotoxicity in male and female Fischer F344 rats. Food Chem. Toxicol. 50, 3978–3983. doi: 10.1016/j.fct.2012.08.010
Scholze, A., Liu, Y., Pedersen, L., Xia, S., Roth, H. J., Hocher, B., et al. (2014). Soluble α-klotho and its relation to kidney function and fibroblast growth factor-23. J. Clin. Endocrinol. Metab. 99, E855–E861. doi: 10.1210/jc.2013-4171
Semba, R. D., Moghekar, A. R., Hu, J., Sun, K., Turner, R., Ferrucci, L., et al. (2014). Klotho in the cerebrospinal fluid of adults with and without Alzheimer’s disease. Neurosci. Lett. 558, 37–40. doi: 10.1016/j.neulet.2013.10.058
Shih, W., Hines, W. H., and Neilson, E. G. (1988). Effects of cyclosporin A on the development of immune-mediated interstitial nephritis. Kidney Int. 33, 1113–1118. doi: 10.1038/ki.1988.119
Shimamura, Y., Hamada, K., Inoue, K., Ogata, K., Ishihara, M., Kagawa, T., et al. (2012). Serum levels of soluble secreted alpha-Klotho are decreased in the early stages of chronic kidney disease, making it a probable novel biomarker for early diagnosis. Clin. Exp. Nephrol. 303, F689–F699. doi: 10.1007/s10157-012-0621-7
Song, L., Ye, M., Troyanovskaya, M., Wilk, E., Wilk, S., and Healy, D. P. (1994). Rat kidney glutamyl aminopeptidase (aminopeptidase A): molecular identity and cellular localization. Am. J. Physiol. 267, F546–F557. doi: 10.1152/ajprenal.1994.267.4.F546
Sweat, F., Puchtler, H., and Rosenthal, S. I. (1964). Sirius red F3BA as a stain for connective tissue. Arch. Pathol. 78, 69–72.
Tencer, J., Frick, I. M., Öquist, B. W., Alm, P., and Rippe, B. (1998). Size-selectivity of the glomerular barrier to high molecular weight proteins: upper size limitations of shunt pathways. Kidney Int. 53, 709–715. doi: 10.1046/j.1523-1755.1998.00797.x
Wang, Y., and Sun, Z. (2009). Klotho gene delivery prevents the progression of spontaneous hypertension and renal damage. Hypertension 54, 810–817. doi: 10.1161/HYPERTENSIONAHA.109.134320
Yu, J., Deng, M., Zhao, J., and Huang, L. (2010). Decreased expression of Klotho gene in uremic atherosclerosis in apolipoprotein E-deficient mice. Biochem. Biophys. Res. Commun. 391, 261–266. doi: 10.1016/j.bbrc.2009.11.046
Keywords: Zucker rats, aminopeptidases, Klotho, hydroxyproline, renal injury
Citation: Montoro-Molina S, López-Carmona A, Quesada A, O’Valle F, Martín-Morales N, Osuna A, Vargas F and Wangensteen R (2018) Klotho and Aminopeptidases as Early Biomarkers of Renal Injury in Zucker Obese Rats. Front. Physiol. 9:1599. doi: 10.3389/fphys.2018.01599
Received: 16 July 2018; Accepted: 24 October 2018;
Published: 13 November 2018.
Edited by:
Hui Y. Lan, The Chinese University of Hong Kong, ChinaReviewed by:
Ana Belen Sanz, Instituto de Investigación Sanitaria Fundación Jiménez Díaz, SpainZhanjun Jia, Nanjing Medical University, China
Copyright © 2018 Montoro-Molina, López-Carmona, Quesada, O’Valle, Martín-Morales, Osuna, Vargas and Wangensteen. This is an open-access article distributed under the terms of the Creative Commons Attribution License (CC BY). The use, distribution or reproduction in other forums is permitted, provided the original author(s) and the copyright owner(s) are credited and that the original publication in this journal is cited, in accordance with accepted academic practice. No use, distribution or reproduction is permitted which does not comply with these terms.
*Correspondence: Rosemary Wangensteen, rwangens@ujaen.es