- 1Key Laboratory of Freshwater Aquatic Genetic Resources, Ministry of Agriculture, Shanghai Ocean University, Shanghai, China
- 2Centre for Research on Environmental Ecology and Fish Nutrition, Ministry of Agriculture, Shanghai Ocean University, Shanghai, China
- 3National Demonstration Center for Experimental Fisheries Science Education, Shanghai Ocean University, Shanghai, China
As representatives of n-6 and n-3 fatty acids, many studies have analyzed the use of soybean oil and linseed oil rich in linoleic acid (18:2n-6, LA) and α-linolenic acid (18:3n-3, LNA) as better substitutes for fish oil. In aquatic animals, different dietary ratios of LA and LNA could have significant effects on growth, lipid metabolism, immune response, and reproduction. To assess the nutritive value of these two fatty acids in Chinese mitten crab (Eriocheir sinensis), we performed transcriptome analysis and label-free quantification proteomic analysis of the hepatopancreas from mitten crabs fed with LA or LNA diet. Parallel reaction monitoring was used to confirm the reliability of the proteomic analysis. A total of 186 proteins were differentially expressed with fold change ≥1.5 or ≤0.666. Among the 186 proteins, 116 were upregulated and 70 were downregulated in the LA than LNA. Most of these proteins participate in cellular process and metabolism process and have molecular functions such as binding and catalytic activity; the cellular component of these proteins are cell, cell part, membrane, and membrane part. A total of 18 proteins were identified to be related to lipid, carbohydrate, and protein metabolism, and they mainly participate in digestive enzyme activities, fatty acid transport, and glycolysis. Our results provide new insights for further investigation into the replacement of fish oil from mitten crabs with vegetable oils and enable us to better understand the different roles and nutrition value of LA and LNA in mitten crabs.
Introduction
The Chinese mitten crab, Eriocheir sinensis, is a native freshwater crab species in China, and it is widely distributed in the streams and rivers along the eastern coast of China (Sui et al., 2009). Because of their nutritional value, flavor, and taste, mitten crabs have become one of the most important economic crab species in China. In 2016, 812,103 tons of mitten crabs was produced by the Chinese aquaculture industry, and ranked first among freshwater crustaceans (China Fisheries Yearbook, 2017). Mitten crabs have a high content of highly unsaturated fatty acids (HUFAs), such as eicosapentaenoic acid (EPA, 20:5n-3) and docosahexaenoic acid (DHA, 22:6n-3), in the hepatopancreas (Tang et al., 2013). To date, most studies have indicated that freshwater fish have the ability to synthesize HUFAs, and supplementation of linoleic acid (LA) and α-linoleic acid (LNA) in diets can satisfy the need for HUFAs (Tocher, 2010). In mitten crabs, several enzymes responsible for the desaturation and elongation of the polyunsaturated fatty acid have been identified, including three desaturases and two elongases, namely, fatty acyl Δ6-desaturase (Yang et al., 2013), fatty acyl-CoA Δ6-b desaturase (Yang et al., 2016b), acyl-CoA Δ9-desaturase (Guo et al., 2013), elongase of very long chain fatty acids (ELOVL) (Yang et al., 2016a), and ELOVL6 (Shi et al., 2016). However, characterization of these desaturases and elongases in mitten crab has shown that these enzymes are not directly involved in the synthesis of HUFAs such as EPA and DHA; therefore, the demands of HUFAs in mitten crabs are mainly provided by the diets.
Fish oil extracted from marine fish contains a high content of HUFAs, such as EPA and DHA, and it has been considered the best lipid source in aquatic feed (Sargent et al., 2002). However, a sharp decline in wild fisheries has significantly increased the price of fish oil, followed by an increase in feed production costs (Naylor et al., 2000; Tacon and Metian, 2009). To reduce the use of fish oil in the diets of mitten crabs, other lipid sources should be found to substitute fish oil. Lipid sources that contain LA and LNA (n-6 and n-3 fatty acid representatives, respectively), such as soybean oil and linseed oil, are considered to be the best substitutes for fish oil, and blends of these oils may be better than using the oils individually (Brown and Hart, 2011; Turchini et al., 2011). However, there is limited information on the optimal LA/LNA ratio in aquatic animal feeds. Many studies have investigated the effects of different dietary LA/LNA ratios in fish (Tian et al., 2016; Zeng et al., 2016a,b, 2017). However, to the best of our knowledge, little studies have investigated the optimal LA/LNA ratio in the diets of the mitten crab. To identify an optimal LA/LNA ratio for mitten crabs, we need to understand the different nutrition values of LA and LNA in mitten crabs.
With developments in mass spectrometry, proteomics has become a powerful tool for the analysis of multidisciplinary scientific questions (Megger et al., 2013). The label-free quantification proteomic approach allows simultaneous identification and quantification and is applicable to samples from any source (Turck et al., 2007; Li et al., 2012; Merl et al., 2012). In this study, the label-free quantification proteomic approach was used to analyze the effects of LA and LNA diets on mitten crabs and identify the differentially expressed proteins in the hepatopancreas of the crabs fed with LA and LNA diets.
Parallel reaction monitoring (PRM) is a new method in targeted mass spectrometry (Du et al., 2015), and it has been widely used to quantify and detect target proteins (Peterson et al., 2012; Tsuchiya et al., 2013). PRM analysis has been successfully used in the confirmation of proteomic analysis. PRM is more specific and sensitive than other monitoring methods, and comparing with western blotting, PRM could simultaneously quantify multiple proteins between two group (Gallien et al., 2014). In this study, PRM was used to confirm the proteomic analysis.
Materials and Methods
Ethics Statement
All the animal experiments were approved by the Animal Care Committee of Shanghai Ocean University, and were carried out in accordance with the principles of the Animal Care Committee of Shanghai Ocean University.
Experimental Materials, Design, and Growth Performance
Two isonitrogenous and isolipidic purified experimental diets were formulated using different dietary lipid sources. The amounts of lipid and protein in the diets were 6 and 41%, respectively. The protein was provided by casein, and LA and LNA were the two lipid sources used in the study. The raw materials were blended and moistened with water and pelletized into 1.5 mm (diameter) pellets and stored at −20°C until use. The composition and formulation of the experimental diets are listed in Table 1.
All the mitten crabs used in the study were obtained from the Chongming Research Base of Shanghai Ocean University. All the crabs were separately acclimated in a single plastic box (36 cm × 18 cm × 18 cm) for a week. During the acclimation, all the crabs were fed with a commercial feed. After acclimation, 48 active male crabs with intact appendages were selected and randomly stocked into 48 plastic boxes (36 cm × 18 cm × 18 cm). Then, the 48 crabs were classified into two groups (24 crabs in each group) and fed with either the LA or LNA diet. Before the feeding experiment, all the crabs in each group were weighted, the carapace length and width were measured. From Table 2, the initial weight, carapace length, and width of crabs in each group were same.
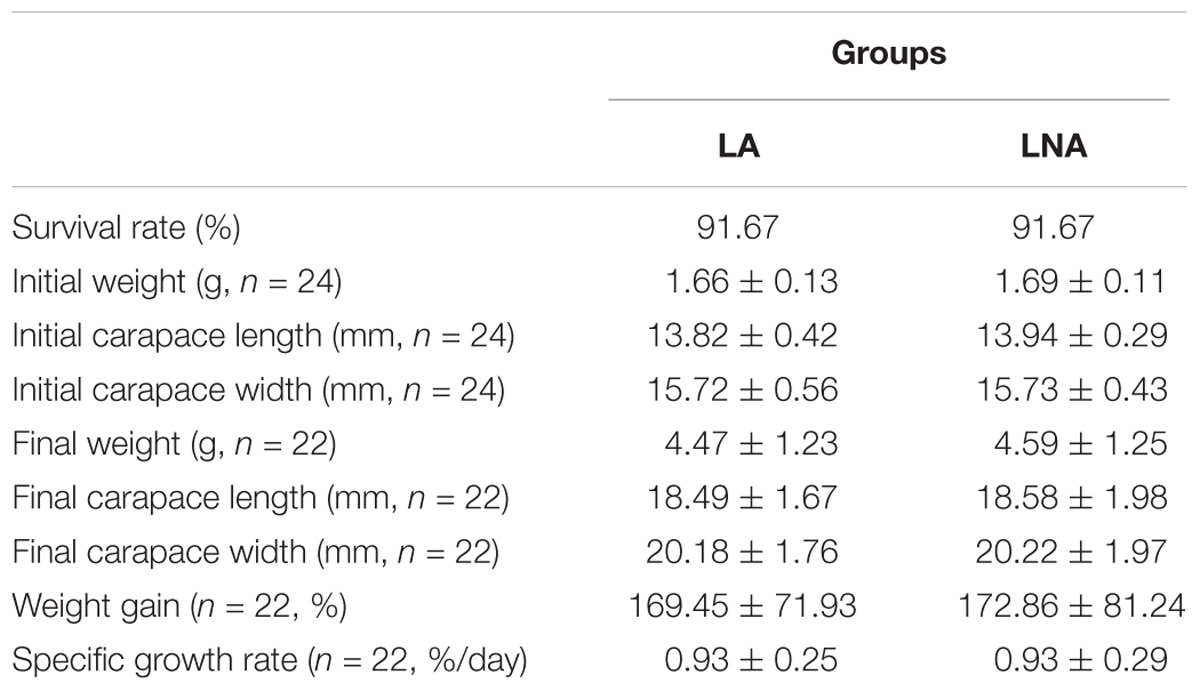
TABLE 2. Effects of different fatty acids diets on growth performance of juvenile Chinese mitten crab, Eriocheir sinensis.
The feeding experiment lasted for 107 days. During the experiment, the crabs were fed daily at 13:30. The experimental diets were administered at 5% body weight of the crabs. Leftover feed was removed using a siphon tube at 15:30. Throughout the experiment, all the boxes were aerated to maintain the dissolved oxygen at >5 mg/L. The photoperiod was 12 h light:12 h dark. Water in each box was exchanged once daily (1/3 to 1/2 of the tank volume). The water parameters in each box were maintained within the following ranges: temperature, 24.5–30°C; pH, 8.0 ± 0.4; and total ammonia nitrogen, <0.01 mg/L. At the end of feeding experiment, the weight, carapace length, and width of crabs in each group were also weighted and measured. The weight gain and specific growth rate were calculated in the following methods:
Sample Collection and Preparation
At the end of the feeding trial, all the crabs were fasted for 24 h. A total of six crabs that had the average weight were selected for the use of the proteomic analysis with three crabs in each group, and the hepatopancreas was collected from the three crabs in sterile centrifuge tubes and immediately frozen in liquid nitrogen. Then, the samples were stored at −80°C until use. Protein extraction was performed using the Mammalian Tissue Total Protein Extraction Reagent (AP0601-50) from Bangfei Bioscience. Before protein extraction, the hepatopancreas from three crabs in each group were pooled into one sample, and then two pooled hepatopancreas samples were ground in liquid nitrogen and rinsed twice with 1 mL of phosphate-buffered saline. Then, the samples were homogenized in the protein lysate containing a protease inhibitor. After centrifugation for 10 min, the supernatant was collected and stored at −80°C for further analysis.
Protein Quantification and Proteolysis
The extracted proteins were quantified using the Bradford method. The calibration curve was established using protein standards. Protein quantification was performed using the calibration curve and optical density values of the protein samples. After quantification, 60 μg of the protein samples was digested using trypsin (Promega, United States) in a ratio of protein:trypsin at 50:1. The protein samples were digested at 37°C for 12–16 h.
Liquid Chromatography-Tandem Mass Spectrometry Analysis
After proteolysis, two peptides samples were obtained, then the peptides in each group were divided into triplicate for the proteomic analysis to minimize equipment error. Gradient elution and separation were performed using Nano high-performance liquid chromatography Ultimate 3000 (Thermo Fisher Scientific, United States) with solutions A and B (A: 0.1% formic acid in water, B: 80% acetonitrile with 0.1% formic acid in water). Mass spectrometry analysis was performed using Q Exactive HF (Thermo Fisher Scientific, United States) coupled to the Nano high-performance liquid chromatography Ultimate 3000. Full MS spectra were obtained with a scan range of 300–1400 m/z, resolution of 120,000, automatic gain control target value of 3e6, maximum ion accumulation time of 80 ms, and one number of scan range. The MS2 scan was set at a resolution of 15,000, automatic gain control target value of 5e4, maximum ion accumulation time of 45 ms, and isolation window of 1.6 m/z.
Protein Identification and Quantification
As a transcriptome analysis was performed before the proteome analysis, the mass spectra was searched against the database from the transcriptome analysis by using Proteome Discoverer, version 2.0 (Thermo Fisher Scientific, United States) and in-house Mascot, version 2.2 (Matrix Science, United Kingdom). The search results were then filtered using a cutoff of 1% for the peptide false identification rate. Label-free quantification was used for quantification of the proteins. For quantitative changes, a cutoff of ≥1.5 or ≤0.666-fold change and p-value (t-test) <0.05 were set for differentially expressed proteins.
Bioinformatic Analysis
In this study, bioinformatic analysis of the proteome was performed by matching the accessions between the proteome and transcriptome. For the transcriptome analysis, the assembled unigenes were aligned with the non-redundant database (Nr), Gene Ontology (GO), euKaryotic Ortholog Groups (KOG), Kyoto Encyclopedia of Genes and Genomes (KEGG) by using BlastX to obtain annotation of the genes. Cluster enrichment of GO terms and KEGG pathways were analyzed using hypergeometric distribution.
PRM Analysis
Parallel reaction monitoring analysis was used to confirm the label-free quantification proteome analysis. Twelve proteins were selected for the PRM analysis. Protein extraction, proteolysis, mass spectrometry analysis, and protein identification were performed according to the methods described previously. Skyline 3.6 software was used for analysis of the raw data of PRM, and quantification of the selected proteins was performed using MSstat. The fold changes in the LNA and LA groups between PRM and label-free quantification proteome were compared for confirmation.
Statistical Analysis
Statistical analysis was performed using SPSS Statistics V22.0 (IBM Corporation, NY, United States). Growth performance was presented as means ± SD and was analyzed by Student’s t-test. Data were considered to be statistically significant when P < 0.05.
Results
Growth Performance
After the feeding experiment, 22 crabs in each group were survived with the survival rate of 91.67% (Table 2). And the final weight, carapace length, width, weight gain, and specific growth rate were same between the crabs fed with LA or LNA (Table 2).
Protein Identification
The mass spectrometry proteomics data have been deposited to the ProteomeXchange Consortium via the PRIDE partner repository with the dataset identifier PXD009864. The LC-MS/MS generated a total of 1,530 unique peptides, and 880 proteins were identified (Supplementary Table S1). The number of identified proteins with molecular weight in the range of 10–20 kD, 20–30 kD, 30–40 kD, 40–50 kD, and 50–60 kD was 183 (20.80%), 165 (18.75%), 138 (15.68%), 97 (11.02%), and 86 (9.77%), respectively, whereas 23.98% proteins had molecular weight >60 kD (Figure 1A). Most of the identified proteins (567) had unique peptides, and about 14.89% of the identified proteins had three or more peptides (Figure 1B). About 21.48% of the identified proteins showed more than 10% peptide coverage.
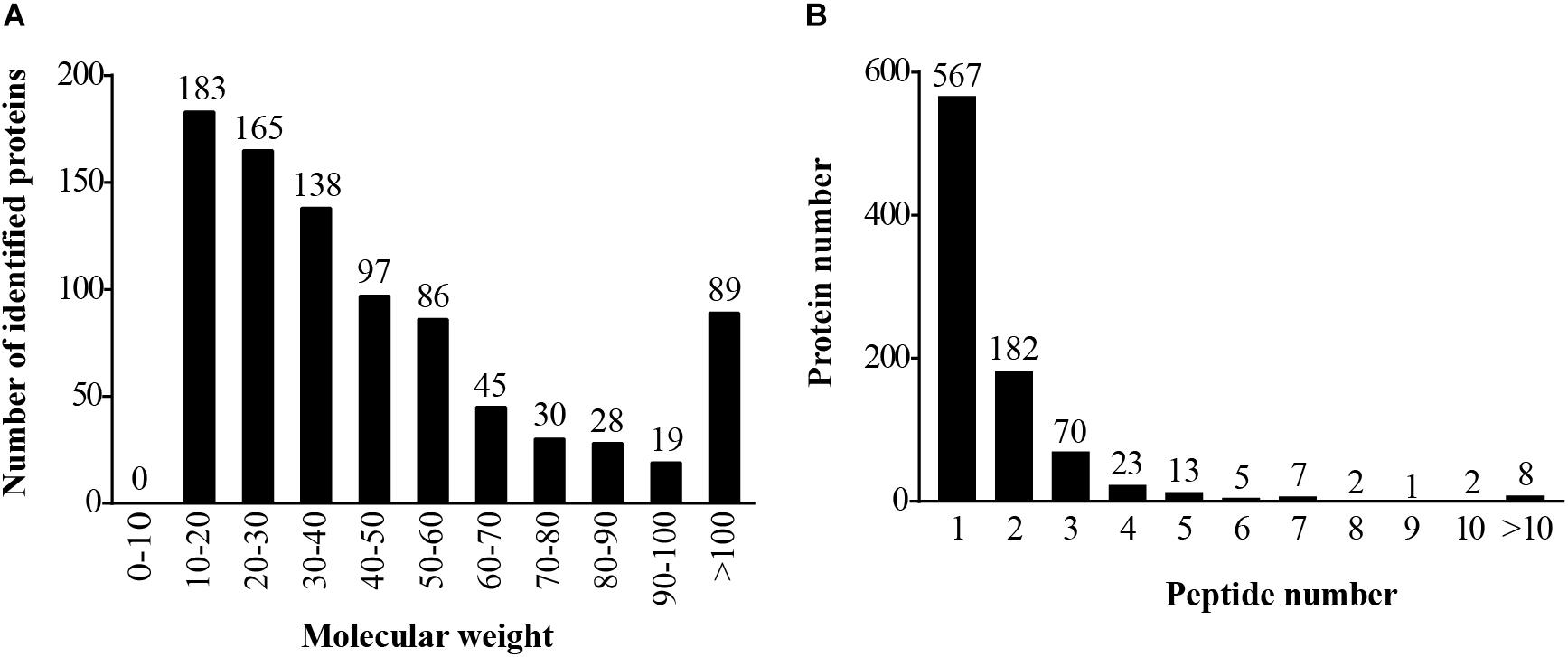
FIGURE 1. Identification and analysis of proteins in the hepatopancreas of mitten crabs fed with LA and LNA diets. (A) Distribution of the identified proteins among different molecular-weight classes. (B) Distribution of proteins containing different numbers of identified peptides.
Differentially Expressed Proteins
Because a cutoff of ≥1.5 or ≤0.666-fold change was set for differentially expressed proteins, 186 proteins were found to be differentially expressed between the LA and LNA groups (Supplementary Table S2). When compared with the LA group, 116 proteins were downregulated and 70 proteins were significantly upregulated in the LNA group. Nr annotation of the differentially expressed proteins showed that the five most significantly downregulated proteins were elongation factor-1 alpha, histone H4, glyceraldehyde-3-phosphate dehydrogenase, nucleophosmin, and alpha-enolase, and the five most significantly upregulated proteins were fatty-acid-binding protein (FABP), RNA polymerase II transcription elongation factor, vitelline membrane outer layer 1, eukaryotic translation initiation factor 5A, and ribosomal protein S3a.
Functional Analysis of the Differentially Expressed Proteins
Function annotation of the differentially expressed proteins was performed to understand the function and bioprocess of the differentially expressed proteins. GO annotation of the differentially expressed proteins showed that both upregulated and downregulated proteins were mainly categorized into metabolism process and cellular process in biological processes, cell and cell part in cellular component, and catalytic activity and binding in molecular function (Figure 2).
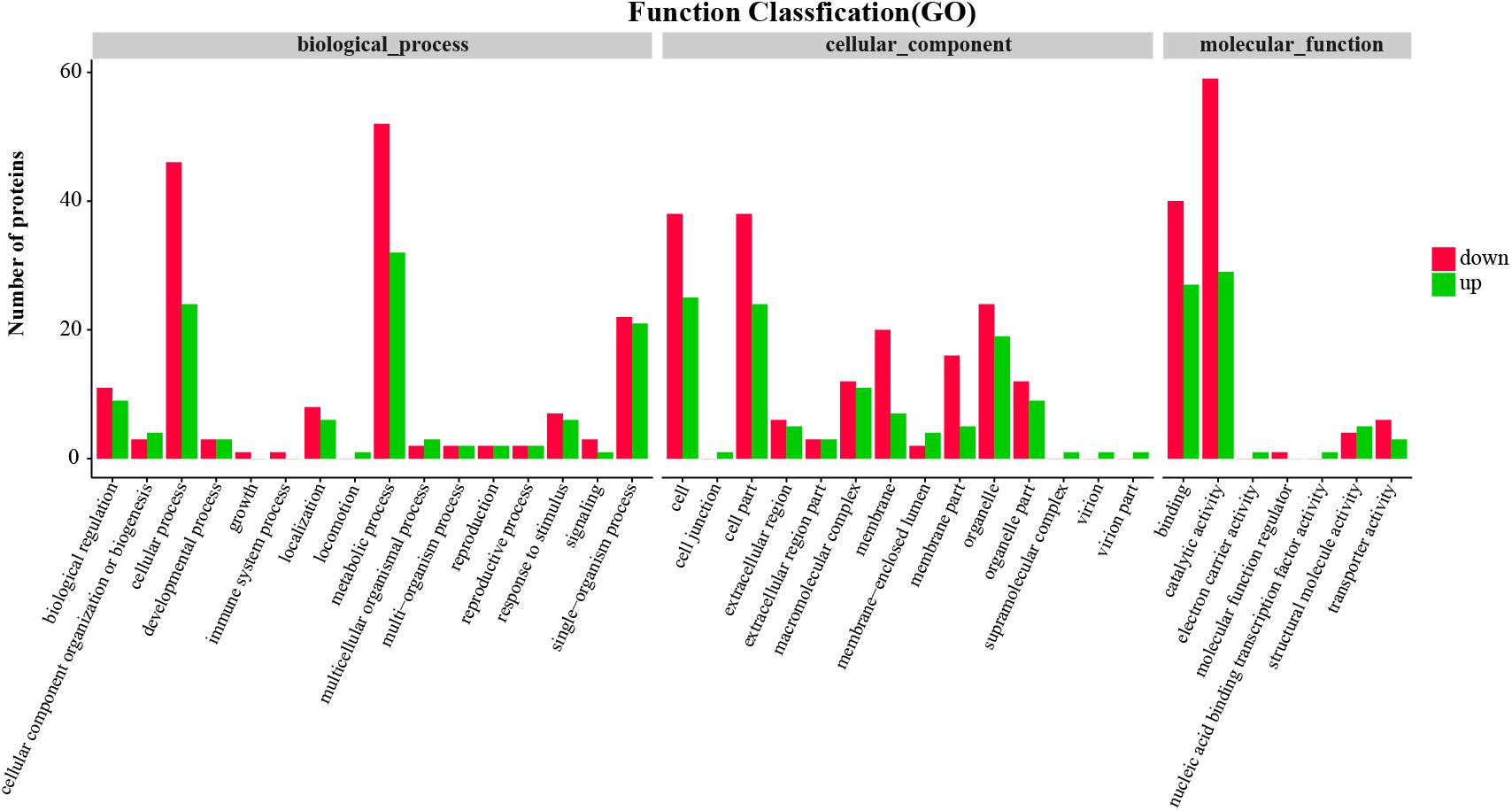
FIGURE 2. Gene Ontology (GO) function classification of differentially expressed proteins in the hepatopancreas of mitten crabs fed with LA and LNA diets.
Kyoto Encyclopedia of Genes and Genomes annotation showed that the downregulated proteins in the LNA participated in 60 KEGG pathways; the top five pathways were glutathione metabolism, ECM-receptor interaction, phagosome, protein processing in endoplasmic reticulum, and proteasome (Figure 3). The differentially expressed proteins that participated in these pathways were glutathione S-transferase, thioredoxin domain-containing protein, aminopeptidase, prostaglandin D synthase, collagen, laminin subunit, integrin, cathepsin L protein, V-type proton ATPase subunit G, calnexin, heat shock protein, and 26S protease regulatory subunit.
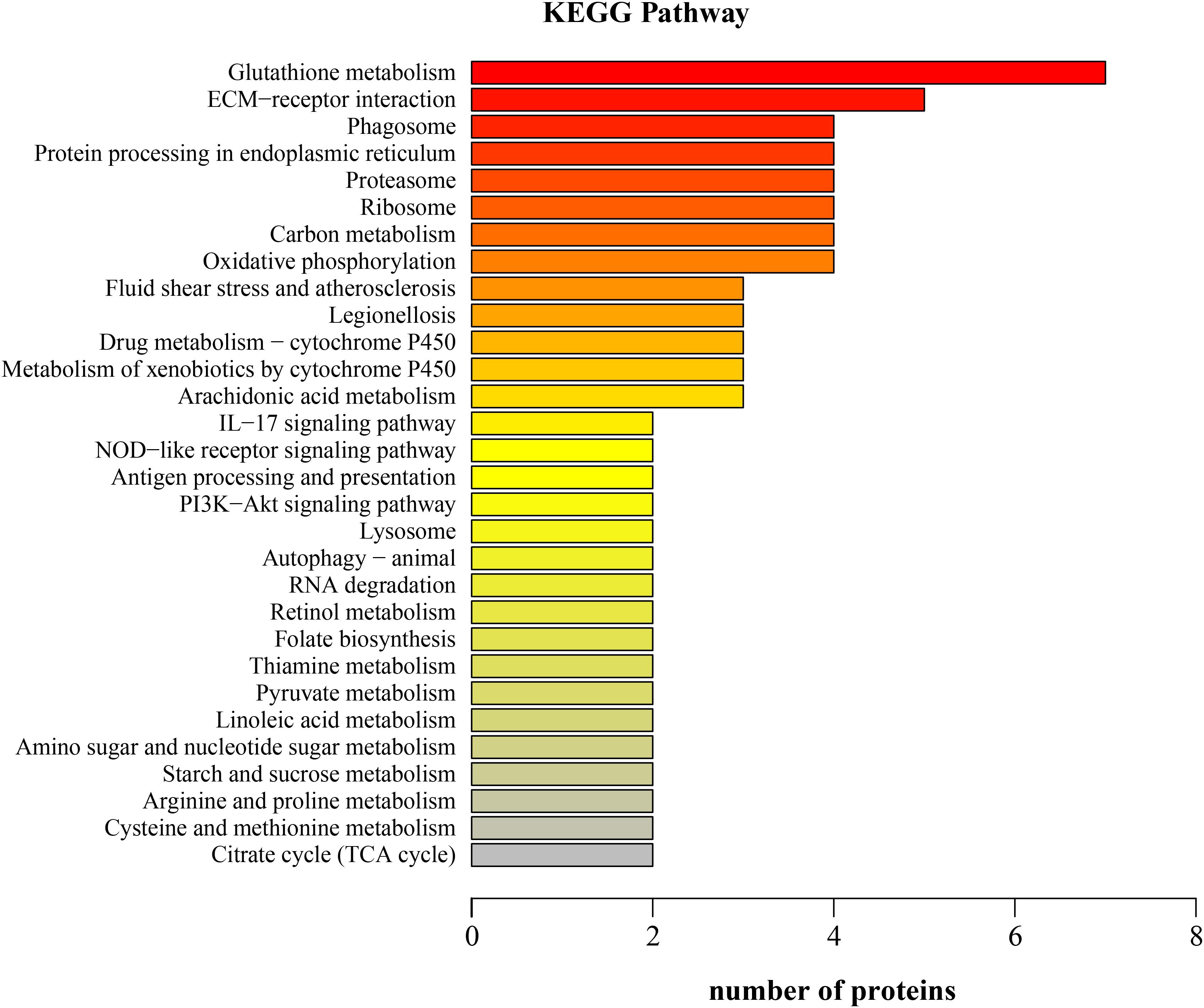
FIGURE 3. Kyoto Encyclopedia of Genes and Genomes (KEGG) function classification of the downregulated proteins in the hepatopancreas of mitten crabs fed with LA and LNA diets (LNA vs. LA).
Sixty-four KEGG pathways were annotated for the 70 upregulated proteins in the LNA. The top five KEGG pathways were protein processing in endoplasmic reticulum, phagosome, ribosome, biosynthesis of amino acids, and amino sugar and nucleotide sugar metabolism (Figure 4). The relevant proteins were protein-disulfide isomerase, translocon-associated protein subunit alpha, B-cell receptor-associated protein, actin, calreticulin, 60S ribosomal protein, ribosomal protein S3a, glyceraldehyde 3-phosphate dehydrogenase, pyruvate kinase 3, chitinase 1, N-acetylneuraminate lyase, and β-hexosaminidase subunit beta.
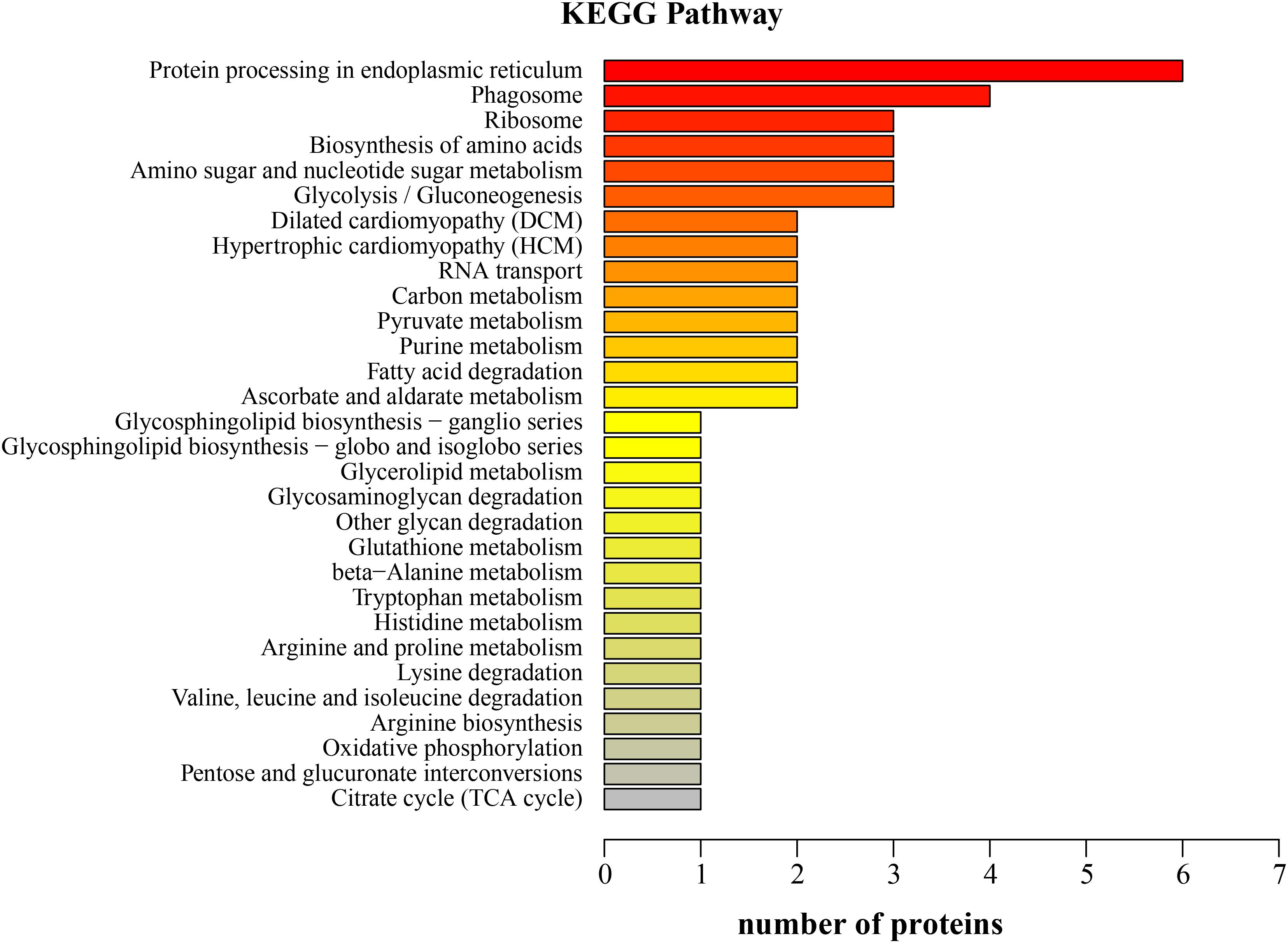
FIGURE 4. Kyoto Encyclopedia of Genes and Genomes function classification of the upregulated proteins in the hepatopancreas of mitten crabs fed with LA and LNA diets (LNA vs. LA).
Differentially Expressed Proteins Related to Metabolism
According to the KOG annotation of the differentially expressed proteins, many proteins related to metabolism were identified (Figure 5). A total of nine proteins were related to carbohydrate transport and metabolism. Metabolism of proteins and lipids was also significantly changed by the dietary fatty acids, as four and five proteins were related to amino acid transport and metabolism and lipid transport and metabolism, respectively. The relevant proteins are listed in Table 3.
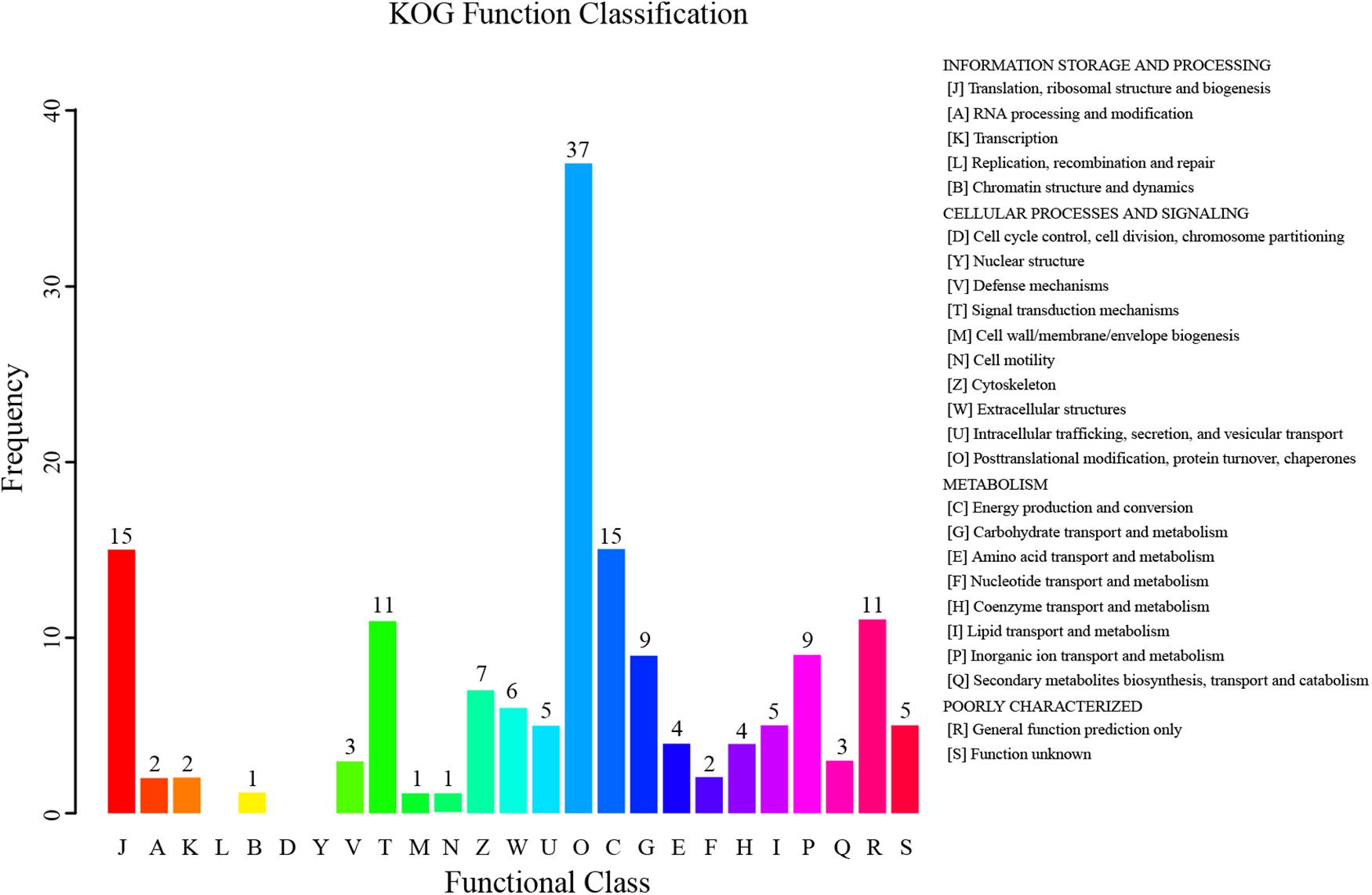
FIGURE 5. euKaryotic Ortholog Groups (KOG) function classification of differentially expressed proteins in the hepatopancreas of mitten crabs fed with LA and LNA diets.
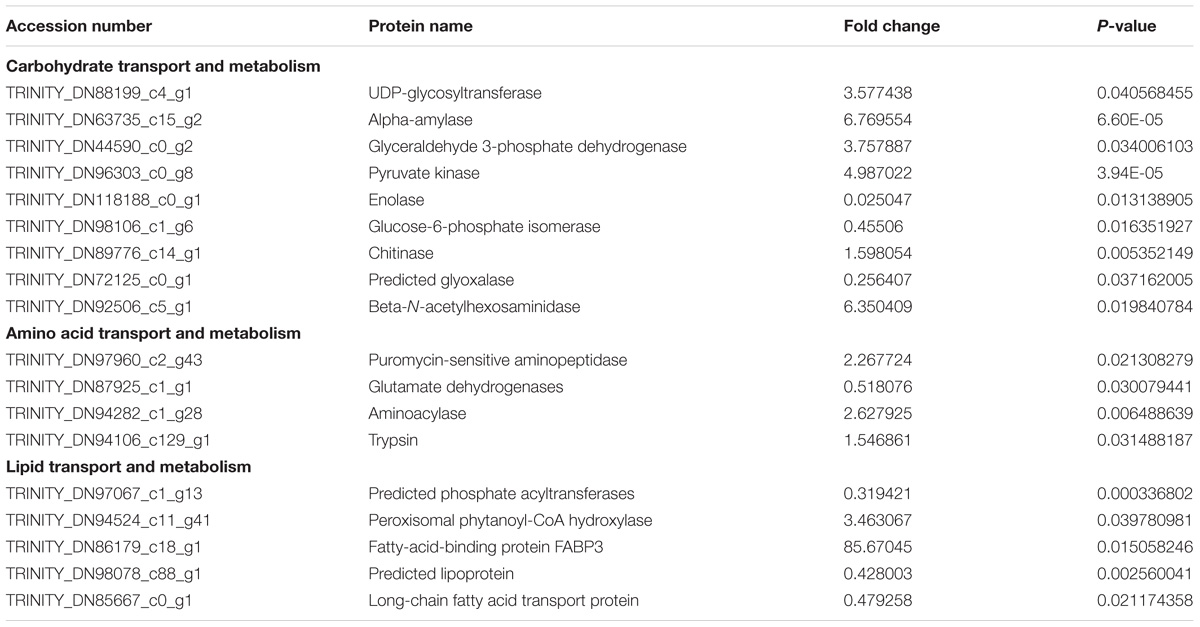
TABLE 3. Differentially expressed proteins related to metabolism in the mitten crabs fed with LA or LNA diet (LNA vs. LA).
PRM Analysis
Parallel reaction monitoring analysis was used to confirm the differentially expressed proteins in the proteome. Twelve differentially expressed proteins with a unique peptide were selected for the PRM analysis, namely, glyceraldehyde 3-phosphate dehydrogenase, hemocyanin, 60S ribosomal protein L5, eukaryotic translation initiation factor 5A, tubulin, adenosine kinase 1, chitinase, spectrin alpha chain, calreticulin, hemocyanin subunit 6, endo-beta-1,4-glucanase, and laminin subunit alpha. The fold changes in these proteins were consistent with the results of the label-free quantification proteome analysis (Figure 6).
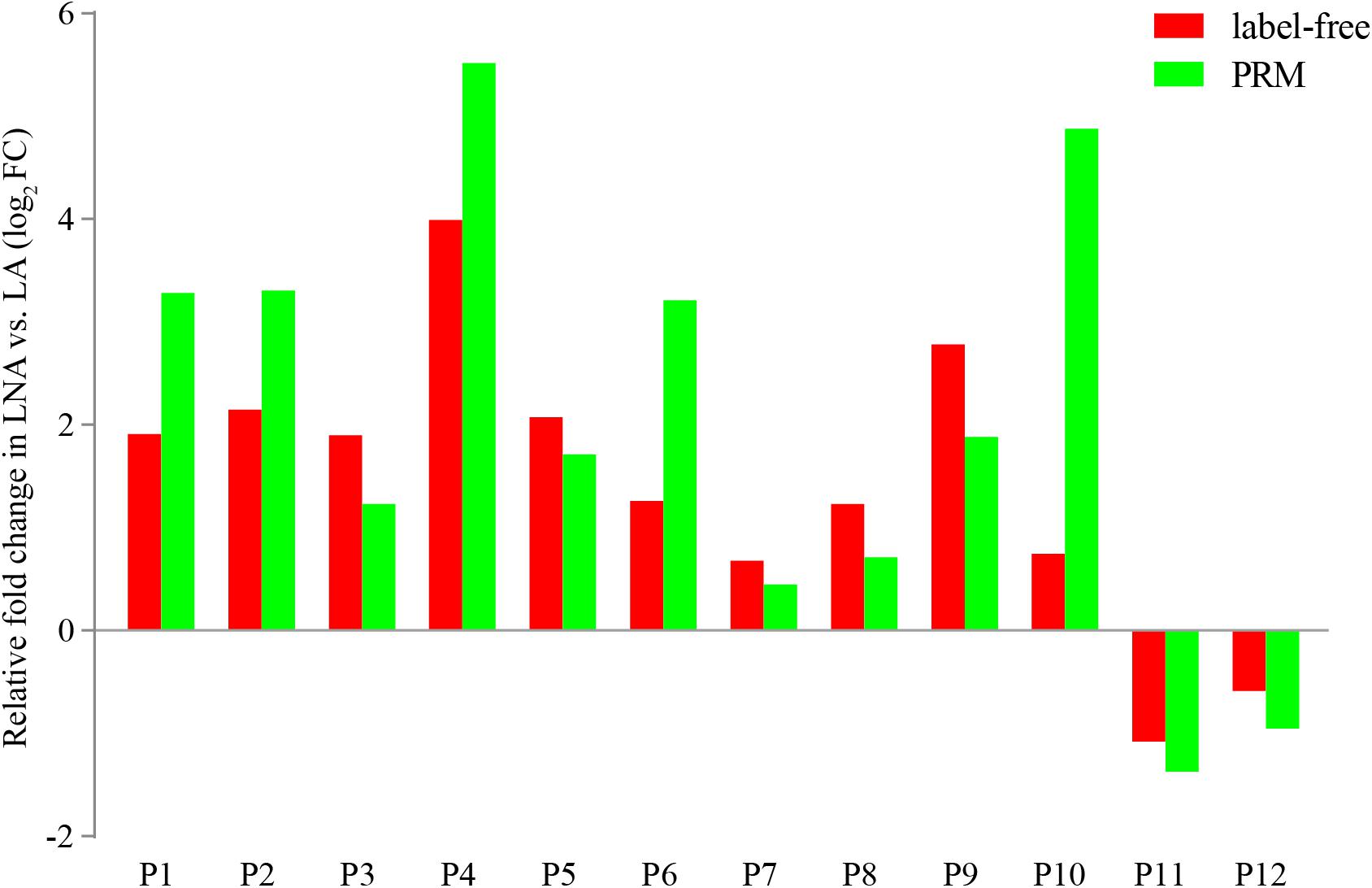
FIGURE 6. Confirmation of differentially expressed proteins detected in the proteome analysis by using PRM analysis. P1: glyceraldehyde 3-phosphate dehydrogenase, P2: hemocyanin, P3: 60S ribosomal protein L5, P4: eukaryotic translation initiation factor, P5: alpha tubulin, P6: adenosine kinase, P7: chitinase, P8: spectrin alpha chain, P9: calreticulin, P10: hemocyanin subunit 6, P11: endo-beta-1,4-glucanase, P12: laminin subunit alpha.
Discussion
To the best of our knowledge, the mitten crab has limited ability to synthesize HUFAs by LA or LNA, as a results, the HUFAs, such as EPA and DHA, are necessary in the diet of mitten crabs (Wu et al., 2007). The higher contents of EPA and DHA in mitten crabs (Chen et al., 2007), as well as the important roles of EPA and DHA in humans, have attracted a lot of research interest in the nutritional value of EPA and DHA in mitten crabs. The beneficial effects of LA and LNA have been ignored as the importance of EPA and DHA. In this study, we performed label-free quantification proteomic analysis to investigate the nutritional value of LA and LNA in the mitten crab.
The hepatopancreas plays an important role in the mitten crab; it is a major digestive gland and has a role in the synthesis and secretion of digestive enzymes as well as digestion and absorption of nutrients (Jiang et al., 2009). The hepatopancreas is also responsible for metabolism in crustaceans (Rosas et al., 1995; Li et al., 2008). Proteomics is a new system biology technique that theoretically presents all the proteins in an organism (Anderson and Anderson, 1998), and comparative proteomic analysis can identify the proteomic differences caused by different factors (Lemos et al., 2010). Thus, comparative proteomic analysis of the hepatopancreas is a practicable method for the analysis of the nutrition value of LA and LNA in the mitten crab.
In rates, LA and LNA have been observed to ameliorate the metabolic syndrome induced by high-fructose and high-fat diets (Zhang et al., 2016). Dietary LA and LNA could have significant effects on carbohydrate and lipid metabolism, and the effects depend on the dose of LA and LNA rather than the ratio; in other words, the roles of LA and LNA are different (Zhang et al., 2016). In the present study, the same results were obtained, several proteins that participated in the metabolism and transport of three major nutrients were significantly differently expressed in the crabs fed with LA and LNA diets.
Lipid Metabolism
A standard metabolism analysis has shown that lipid is the main source for energy metabolism in the mitten crab, followed by carbohydrate and protein (Wen et al., 2001); this indicated the important role of lipid metabolism in the mitten crab. In the present study, five proteins relative to lipid metabolism in the crabs fed with LA and LNA diets were significantly changed. The level of FABP3 was significantly higher in the crabs fed with LNA than in those fed with LA. FABPs belong to a gene family that helps in the transport of long-chain fatty acids across the plasma membrane (Kaikaus et al., 1990). FABP3 is responsible for the transport of fatty acids for β-oxidation (Jordal et al., 2006). Besides FABP3, two proteins, namely, a lipoprotein and long-chain fatty acid transport protein, were significantly changed in the LA and LNA groups. The lipoprotein and long-chain fatty acid transport protein have roles in the transport of lipid and fatty acid, respectively (Schaffer and Lodish, 1994; Rodenburg and Van der Horst, 2005). An optimal ratio of LA/LNA could inhibit fat deposition (Zeng et al., 2016b). In the present study, we observed that the effects of LA and LNA on fat deposition were dependent on two different parameters: LNA increased fatty acid transport for β-oxidation, whereas LA increased the levels of the lipoprotein and long-chain fatty acid transport protein to decrease lipid deposition. Because of the different roles of LA and LNA in lipid metabolism, an optimal blending of LA and LNA might be better than the use of either LA or LNA alone (Brown and Hart, 2011; Turchini et al., 2011).
Carbohydrate Metabolism
Chitin is a typical polysaccharide and exists in the cuticles of mitten crabs (Kurita, 2006). At the time of molting, the exoskeleton composed of chitin is cast off (Kono et al., 1995). Chitinase, which hydrolyzes chitin, has an important role in the molting of the mitten crab (Dahiya et al., 2006; Yao et al., 2015). Previous studies have indicated that the expression of chitinase is regulated by many factors, such as salinity (Zhang et al., 2015). However, to our knowledge, no study has investigated the effects of dietary fatty acids on chitinase. In the present study, the level of chitinase was significantly higher in the LNA group than in the LA group, which may indicate that LNA has a role in the regulation of molting in the mitten crab. The effects of LA and LNA on carbohydrate metabolism, which has a role in type 2 diabetes mellitus, have been thoroughly investigated in humans; however, further studies are needed to elucidate the effects of LA and LNA on glucose metabolism (Cabout et al., 2017). In this study, several proteins that participate in glycolysis were significantly changed in the LA and LNA groups, which indicates that LA and LNA have different roles in glucose metabolism in the mitten crab. Glycolysis is a universal pathway that exists in living cells. The end product of glycolysis is lactate under anaerobic conditions, while pyruvate is formed in the presence of oxygen and is then oxidized to CO2 and H2O (Akram, 2013). Glycolysis requires three key enzymes, one of which is pyruvate kinase (Li et al., 2015). The higher level of pyruvate kinase in the LNA group may indicate the more important role of LNA than LA in glycolysis. However, the levels of other enzymes that participate in glycolysis, such as enolase and glucose-6-phosphate isomerase, were higher in the LA group, suggesting that LA is also an important factor for the regulation of glycolysis in the mitten crab. Our results indicate that blends of LA and LNA may be better than the individual supplements. Alpha-amylase was also differentially expressed in the LA and LNA groups; it was expressed significantly higher in the LNA group than in the LA group. In grass carp, the activity of amylase could be significantly increased by increasing the LNA/LA ratio to a certain degree (Zeng et al., 2016b). On the basis of our results, we can conclude that LNA may have a more important role in the acceleration of amylase than LA. For omnivorous species, amylase is important for digestion. A previous study showed that mitten crabs fed with a linseed oil diet (2.03) had a lower feed coefficient than mitten crabs fed with a soybean oil diet (2.46) (Wang et al., 2017). We speculate that improvement of the feed coefficient in the linseed oil group is mainly due to the higher abundance of amylase, as well as trypsin, attributable to LNA.
Protein Metabolism
Besides trypsin, three proteins were significantly changed between the LNA and LA groups. In the mitten crab, glutamate dehydrogenases were isolated in 2012, and they have been proven to have an important role in controlling osmoregulation (Wang et al., 2012). Puromycin-sensitive aminopeptidase is an enzyme with roles in a number of physiological processes, such as normal cellular protein turnover, cell cycle regulation, processing of antigenic peptides for display on class I MHC and degradation of neuropeptides (Goldberg and Rock, 1992; Stoltze et al., 2000; Schulz et al., 2001; Lilley et al., 2005). In the present study, the levels of glutamate dehydrogenases were significantly higher in the LNA group than in the LA group, while puromycin-sensitive aminopeptidase level was higher in the LA group. However, further studies are required to investigate the effects of LNA and LA on osmoregulation and the above-mentioned physiological processes.
Conclusion
The present study demonstrated that dietary LA and LNA have different nutrition values in the mitten crab, particularly with respect to digestive enzyme activities, fatty acid transport, and glycolysis. The different roles of LA and LNA in mitten crabs are complementary, and blending LA and LNA may have better effects than the individual supplements. Our results provide new insights for the replacement of fish oil from mitten crabs with vegetable oils and enable us to better understand the different roles and nutrition value of LA and LNA in mitten crabs.
Author Contributions
BW, ZY, XY, and YC conceived and designed the study. BW, JZ, HY, and LZ collected the samples. BW and ZY acquired and analyzed the data. BW and ZY drafted the manuscript. All the authors have read and approved the final manuscript.
Funding
This work was supported by the National Natural Science Foundation of China (Grant No. 31472287) and the China Agriculture Research System (CARS-48).
Conflict of Interest Statement
The authors declare that the research was conducted in the absence of any commercial or financial relationships that could be construed as a potential conflict of interest.
Acknowledgments
We would like to thank the native English speaking scientists of Elixigen Company (Huntington Beach, CA, United States) for editing our manuscript.
Supplementary Material
The Supplementary Material for this article can be found online at: https://www.frontiersin.org/articles/10.3389/fphys.2018.01430/full#supplementary-material
TABLE S1 | Proteins identified in the hepatopancreas of mitten crabs fed with LA and LNA diets by using the label-free quantification proteome approach.
TABLE S2 | Differentially expressed proteins identified in the hepatopancreas of mitten crabs fed with LA and LNA diets by using the label-free quantification proteome approach.
References
Akram, M. (2013). Mini-review on glycolysis and cancer. J. Cancer Educ. 28, 454–457. doi: 10.1007/s13187-013-0486-9
Anderson, N. L., and Anderson, N. G. (1998). Proteome and proteomics: new technologies, new concepts, and new words. Electrophoresis 19:1853. doi: 10.1002/elps.1150191103
Brown, P. B., and Hart, S. D. (2011). “Soybean oil and other n-6 polyunsaturated fatty acid-rich vegetable oils,” in Fish Oil Replacement and Alternative Lipid Sources in Aquaculture Feeds, eds G. M. Turchini, W. K. Ng and D. R. Tocher (Boca Raton, FL: Chemical Rubber CompanyPress).
Cabout, M., Alssema, M., Nijpels, G., Stehouwer, C. D. A., Zock, P. L., Brouwer, I. A., et al. (2017). Circulating linoleic acid and alpha-linolenic acid and glucose metabolism: the Hoorn Study. Eur. J. Nutr. 56, 2171–2180. doi: 10.1007/s00394-016-1261-6
Chen, D. W., Zhang, M., and Shrestha, S. (2007). Compositional characteristics and nutritional quality of Chinese mitten crab (Eriocheir sinensis). Food Chem. 103, 1343–1349. doi: 10.1016/j.foodchem.2006.10.047
Dahiya, N., Tewari, R., and Hoondal, G. S. (2006). Biotechnological aspects of chitinolytic enzymes: a review. Appl. Microbiol. Biotechnol. 71, 773–782. doi: 10.1007/s00253-005-0183-7
Du, C., Liu, H. F., Lin, Y. Z., Wang, X. F., Ma, J., Li, Y. J., et al. (2015). Proteomic alteration of equine monocyte-derived macrophages infected with equine infectious anemia virus. Proteomics 15, 1843–1858. doi: 10.1002/pmic.201400279
Gallien, S., Bourmaud, A., Kim, S. Y., and Domon, B. (2014). Technical considerations for large-scale parallel reaction monitoring analysis. J. Proteomics 100, 147–159. doi: 10.1016/j.jprot.2013.10.029
Goldberg, A. L., and Rock, K. L. (1992). Proteolysis, proteasomes and antigen presentation. Nature 357, 375–379. doi: 10.1038/357375a0
Guo, Z. H., Yang, Z. G., Cheng, Y. X., Ji, L. Y., Que, Y. Q., Liu, Z. W., et al. (2013). Molecular characterization, tissue expression of acyl-CoA (9-desaturase-like gene, and effects of dietary lipid levels on its expression in the hepatopancreas of the Chinese mitten crab (Eriocheir sinensis). Aquaculture 40, 58–65. doi: 10.1016/j.aquaculture.2013.03.033
Jiang, H., Cai, Y. M., Chen, L. Q., Zhang, X. W., Hu, S. N., and Wang, Q. (2009). Functional annotation and analysis of expressed sequence tags from the hepatopancreas of mitten crab (Eriocheir sinensis). Mar. Biotechnol. 11, 317–326. doi: 10.1007/s10126-008-9146-1
Jordal, A. E., Hordvik, I., Pelsers, M., Bernlohr, D. A., and Torstensen, B. E. (2006). FABP3 and FABP10 in Atlantic salmon (Salmo salar L.)–general effects of dietary fatty acid composition and life cycle variations. Comp. Biochem. Physiol. B Biochem. Mol. Biol. 145, 147–158. doi: 10.1016/j.cbpb.2006.05.007
Kaikaus, R. M., Bass, N. M., and Ockner, R. K. (1990). Functions of fatty acid binding proteins. Experimatia 46, 617–630. doi: 10.1007/BF01939701
Kono, M., Wilder, M. N., Matsui, T., Furukawa, K., Koga, D., and Aida, K. (1995). Chitinolytic enzyme activities in the hepatopancreas, tail fan and hemolymph of kuruma prawn Penaeus japonicus during the molt cycle. Fish. Sci. 61, 727–728. doi: 10.2331/fishsci.61.727
Kurita, K. (2006). Chitin and chitosan: functional biopolymers from marine crustaceans. Mar. Biotechnol. 8, 203–226. doi: 10.1007/s10126-005-0097-5
Lemos, M. F., Soares, A. M., Correia, A. C., and Esteves, A. C. (2010). Proteins in ecotoxicology - how, why and why not? Proteomics 10, 873–887. doi: 10.1002/pmic.200900470
Li, E. C., Chen, L. Q., Zeng, C., Yu, N., Xiong, Z. Q., Chen, X. F., et al. (2008). Comparison of digestive and antioxidant enzymes activities, haemolymph oxyhemocyanin contents and hepatopancreas histology of white shrimp, Litopenaeus vannamei, at various salinities. Aquaculture 274, 80–86. doi: 10.1016/j.aquaculture.2007.11.001
Li, X. B., Gu, J. D., and Zhou, Q. H. (2015). Review of aerobic glycolysis and its key enzymes - new targets for lung cancer therapy. Thoracic Cancer 6, 17–24. doi: 10.1111/1759-7714.12148
Li, Z., Adams, R. M., Chourey, K., Hurst, G. B., Hettich, R. L., and Pan, C. (2012). Systematic comparison of label-free, metabolic labeling, and isobaric chemical labeling for quantitative proteomics on LTQ Orbitrap Velos. J. Proteome Res. 11, 1582–1590. doi: 10.1021/pr200748h
Lilley, C. J., Goodchild, S. A., Atkinson, H. J., and Urwin, P. E. (2005). Cloning and characterisation of a Heterodera glycines aminopeptidase cDNA. Int. J. Parasitol. 35, 1577–1585. doi: 10.1016/j.ijpara.2005.07.017
Megger, D. A., Bracht, T., Meyer, H. E., and Sitek, B. (2013). Label-free quantification in clinical proteomics. Biochim. Biophys. Acta 1834, 1581–1590. doi: 10.1016/j.bbapap.2013.04.001
Merl, J., Ueffing, M., Hauck, S. M., and von Toerne, C. (2012). Direct comparison of MS-based label-free and SILAC quantitative proteome profiling strategies in primary retinal Muller cells. Proteomics 12, 1902–1911. doi: 10.1002/pmic.201100549
Naylor, R. L., Goldburg, R. J., Primavera, J. H., Kautsky, N., Beveridge, M. C., Clay, J., et al. (2000). Effect of aquaculture on world fish supplies. Nature 405, 1017–1024. doi: 10.1038/35016500
Peterson, A. C., Russell, J. D., Bailey, D. J., Westphall, M. S., and Coon, J. J. (2012). Parallel reaction monitoring for high resolution and high mass accuracy quantitative, targeted proteomics. Mol. Cell. Proteomics 11, 1475–1488. doi: 10.1074/mcp.O112.020131
Rodenburg, K. W., and Van der Horst, D. J. (2005). Lipoprotein-mediated lipid transport in insects: analogy to the mammalian lipid carrier system and novel concepts for the functioning of LDL receptor family members. Biochim. Biophys. Acta 1736, 10–29. doi: 10.1016/j.bbalip.2005.07.002
Rosas, C., Bolongaro-crevenna, A., Sanchez, A., Gaxiola, G., Soto, L., and Escobar, E. (1995). Role of digestive gland in the energetic metabolism of Penaeus setiferus. Biol. Bull. 189, 168–174.
Sargent, J. R., Tocher, D. R., and Bell, J. G. (2002). “The lipid,” in Fish Nutrition, eds J. E. Halver and R. W. Hardy (San Diego, CA: Academic Press), 181–257.
Schaffer, J. E., and Lodish, H. F. (1994). Expression cloning and characterization of a novel adipocyte long chain fatty acid transport protein. Cell 79, 427–436. doi: 10.1016/0092-8674(94)90252-6
Schulz, C., Perezgasga, L., and Fuller, M. T. (2001). Genetic analysis of dPsa, the Drosophila orthologue of puromycin-sensitive aminopeptidase, suggests redundancy of aminopeptidases. Dev. Genes Evol. 211, 581–588. doi: 10.1007/s00427-001-0194-z
Shi, Q. Y., Yang, Z. G., Yao, Q. Q., Cheng, Y. X., Yang, Q., and Wei, B. H. (2016). Full-length cDNA cloning of ELOVL6 and its tentative study in Chinese mitten crab (Eriocheir sinensis). J. Fish. China 40, 344–355. doi: 10.11964/jfc.20151210193
Stoltze, L., Schirle, M., Schwarz, G., Schroter, C., Thompson, M. W., Hersh, L. B., et al. (2000). Two new proteases in the MHC class I processing pathway. Nat. Immunol. 1:413. doi: 10.1038/80852
Sui, L. Y., Zhang, F. M., Wang, X. M., Bossier, P., Sorgeloos, P., and Hänfling, B. (2009). Genetic diversity and population structure of the Chinese mitten crab Eriocheir sinensis in its native range. Mar. Biol. 156, 1573–1583. doi: 10.1007/s00227-009-1193-2
Tacon, A. G. J., and Metian, M. (2009). Fishing for aquaculture: non-food use of small pelagic forage fish-a global perspective. Rev. Fish. Sci. 17, 305–317.
Tang, C. J., Songqian, C. H., Fu, N., Liu, Y., Tao, N. P., and Wang, X. C. (2013). Lipid content and fatty acid composition of Eriocheir sinensis at different stages of growth. Food Sci. 34, 174–178.
Tian, J. J., Lei, C. X., and Ji, H. (2016). Influence of dietary linoleic acid (18:2n-6) and α-linolenic acid (18:3n-3) ratio on fatty acid composition of different tissues in freshwater fish Songpu mirror carp, Cyprinus Carpio. Aquac. Res. 47, 3811–3825. doi: 10.1111/are.12832
Tocher, D. R. (2010). Fatty acid requirements in ontogeny of marine and freshwater fish. Aquac. Res. 41, 717–732. doi: 10.1111/j.1365-2109.2008.02150.x
Tsuchiya, H., Tanaka, K., and Saeki, Y. (2013). The parallel reaction monitoring method contributes to a highly sensitive polyubiquitin chain quantification. Biochem. Biophys. Res. Commun. 436, 223–229. doi: 10.1016/j.bbrc.2013.05.080
Turchini, G. M., Francis, D. S., Senadheera, S. P. S. D., Thanuthong, T., and De Silva, S. S. (2011). Fish oil replacement with different vegetable oils in Murray cod: evidence of an “omega-3 sparing effect” by other dietary fatty acids. Aquaculture 315, 250–259. doi: 10.1016/j.aquaculture.2011.02.016
Turck, C. W., Falick, A. M., Kowalak, J. A., Lane, W. S., Lilley, K. S., Phinney, B. S., et al. (2007). The association of biomolecular resource facilities proteomics research group 2006 study: relative protein quantitation. Mol. Cell. Proteomics 6, 1291–1298. doi: 10.1074/mcp.M700165-MCP200
Wang, J. Y., Yang, Z. G., Wei, B. H., Shi, Q. Y., Yang, X. Z., and Cheng, Y. X. (2017). Effects of different lipid sources on growth, digestive enzyme activity, and fatty acid composition in juvenile Chinese mitten crab, Eriocheir sinensis. J. Fish. China 24, 1213–1222.
Wang, Y. R., Li, E. C., Yu, N., Wang, X. D., Cai, C. F., Tang, B. P., et al. (2012). Characterization and expression of glutamate dehydrogenase in response to acute salinity stress in the Chinese mitten crab, Eriocheir sinensis. PLoS One 7:e37316. doi: 10.1371/journal.pone.0037316
Wen, X. B., Chen, L. Q., Ai, C. X., and Jiang, H. B. (2001). Standard metabolism of the juvenile crab Eriocheir sinensis. Zool. Res. 22, 425–428.
Wu, X. G., Cheng, Y. X., Sui, L. Y., Zeng, C. S., Southgate, P. C., and Yang, X. Z. (2007). Effect of dietary supplementation of phospholipids and highly unsaturated fatty acids on reproductive performance and offspring quality of Chinese mitten crab, Eriocheir sinensis (H. Milne-Edwards), female broodstock. Aquaculture 273, 602–613. doi: 10.1016/j.aquaculture.2007.09.030
Yang, Z. G., Guo, Z. H., Ji, L. Y., Zeng, Q. T., Wang, Y., Yang, X. Z., et al. (2013). Cloning and tissue distribution of a fatty acyl (6-desaturase-like gene and effects of dietary lipid levels on its expression in the hepatopancreas of Chinese mitten crab (Eriocheir sinensis). Comp. Biochem. Physiol. B Biochem. Mol. Biol. 165, 99–105. doi: 10.1016/j.cbpb.2013.03.010
Yang, Z. G., Shi, Q. Y., Cheng, Y. X., Que, Y. Q., Yang, Q., Xu, L., et al. (2016a). Full-length cDNA cloning and expression analysis of the fatty acid elongase gene from Chinese mitten crab (Eriocheir sinensis). J. Fish. China 23,53–63.
Yang, Z. G., Yao, Q. Q., Cheng, Y. X., Shi, Q. Y., Yang, Q., He, J., et al. (2016b). The full length cDNA cloning and prokaryotic expression of fatty acyl-CoA (6-b desaturase in Eriocheir sinensis. J. Fish. China 40, 1–13. doi: 10.11964/jfc.20150309791
Yao, Q. Q., Yang, Z. G., Wang, Y., Guo, Z. H., Liu, Q. B., Shi, Q. Y., et al. (2015). Full length cDNA cloning of the chitinase gene (HXchit) and analysis of expression during the molting cycle of the Chinese mitten crab, Eriocheir sinensis. J. Fish. China 22, 185–195.
Zeng, Y. Y., Feng, L., Jiang, W. D., Liu, Y., Wu, P., Jiang, J., et al. (2017). Dietary alpha-linolenic acid/linoleic acid ratios modulate immune response, physical barrier and related signaling molecules mRNA expression in the gills of juvenile grass carp (Ctenopharyngodon idella). Fish Shellfish Immunol. 62, 1–12. doi: 10.1016/j.fsi.2017.01.003
Zeng, Y. Y., Jiang, W. D., Liu, Y., Wu, P., Zhao, J., Jiang, J., et al. (2016a). Dietary alpha-linolenic acid/linoleic acid ratios modulate intestinal immunity, tight junctions, anti-oxidant status and mRNA levels of NF-kappaB p65, MLCK and Nrf2 in juvenile grass carp (Ctenopharyngodon idella). Fish Shellfish Immun. 51, 351–364. doi: 10.1016/j.fsi.2015.11.026
Zeng, Y. Y., Jiang, W. D., Liu, Y., Wu, P., Zhao, J., Jiang, J., et al. (2016b). Optimal dietary alpha-linolenic acid/linoleic acid ratio improved digestive and absorptive capacities and target of rapamycin gene expression of juvenile grass carp (Ctenopharyngodon idellus). Aquac. Nutr. 22, 1251–1266. doi: 10.1111/anu.12337
Zhang, F., Lv, J. J., Liu, P., Gao, B. Q., Li, J., and Chen, P. (2015). Cloning and expression of chitinase under low salinity stress during molting in Portunus trituberculatus. Oceanol. Limnol. Sin. 46, 948–957.
Keywords: Eriocheir sinensis, proteome, hepatopancreas, linoleic acid, α-linolenic acid, label-free quantification
Citation: Wei B, Yang Z, Cheng Y, Zhou J, Yang H, Zhang L and Yang X (2018) Proteomic Analysis of the Hepatopancreas of Chinese Mitten Crabs (Eriocheir sinensis) Fed With a Linoleic Acid or α-Linolenic Acid Diet. Front. Physiol. 9:1430. doi: 10.3389/fphys.2018.01430
Received: 25 March 2018; Accepted: 20 September 2018;
Published: 10 October 2018.
Edited by:
Nour Eissa, University of Manitoba, CanadaReviewed by:
Maria Violetta Brundo, Università degli Studi di Catania, ItalyErchao Li, Hainan University, China
Wang Ai Min, Yancheng Institute of Technology, China
Copyright © 2018 Wei, Yang, Cheng, Zhou, Yang, Zhang and Yang. This is an open-access article distributed under the terms of the Creative Commons Attribution License (CC BY). The use, distribution or reproduction in other forums is permitted, provided the original author(s) and the copyright owner(s) are credited and that the original publication in this journal is cited, in accordance with accepted academic practice. No use, distribution or reproduction is permitted which does not comply with these terms.
*Correspondence: Zhigang Yang, emd5YW5nQHNob3UuZWR1LmNu Yongxu Cheng, Y2hlbmd5b25neHVjcmFibGFiNkBob3RtYWlsLmNvbQ==
†These authors have contributed equally to this work