- 1Life and Health Sciences Research Institute, School of Health Sciences, University of Minho, Braga, Portugal
- 2ICVS/3B’s – PT Government Associate Laboratory, Guimarães, Portugal
- 3Instituto de Tecnologia Química e Biológica António Xavier, Universidade NOVA de Lisboa, Oeiras, Portugal
- 4IINFACTS – Institute of Research and Advanced Training in Health Sciences and Technologies, Department of Sciences, CESPU, CRL, University Institute of Health Sciences, Gandra, Portugal
- 5UCIBIO, REQUIMTE, Laboratory of Toxicology, Department of Biological Sciences, Faculty of Pharmacy, University of Porto, Porto, Portugal
- 6Department of Public Health and Forensic Sciences, and Medical Education, Faculty of Medicine, University of Porto, Porto, Portugal
- 7Instituto de Investigação e Inovação em Saúde, Universidade do Porto, Porto, Portugal
- 8Instituto de Biologia Molecular e Celular, Universidade do Porto, Porto, Portugal
Dietary nutrients have emerged as potential therapeutic adjuncts for inflammatory bowel disease (IBD) given their impact on intestinal homeostasis through the modulation of immune response, gut microbiota composition and epithelial barrier stability. Several nutrients have already been associated with a protective phenotype. Yet, there is a lack of knowledge toward the most promising ones as well as the most adequate phase of action. To unveil the most prominent therapy candidates we characterized the colon metabolic profile during colitis development. We have observed a twofold decrease in threonine levels in mice subjected to DSS-induced colitis. We then assessed the effect of threonine supplementation in the beginning of the inflammatory process (DSS + Thr) or when inflammation is already established (DSS + Thr D8). Colitis progression was similar between the treated groups and control colitic mice, yet threonine had a surprisingly detrimental effect when administered in the beginning of the disease, with mice displaying a delayed recovery when compared to control mice and mice supplemented with threonine after day 8. Although no major changes were found in their metabolic profile, DSS + Thr mice displayed altered expression in mucin-encoding genes, as well as in goblet cell counts, unveiling an impaired ability to produce mucus. Moreover, IL-22 secretion was decreased in DSS + Thr mice when compared to DSS + Thr D8 mice. Overall, these results suggest that supplementation with threonine during colitis induction impact goblet cell number and delays the recovery period. This reinforces the importance of a deeper understanding regarding threonine supplementation in IBD.
Introduction
Inflammatory bowel disease (IBD) is a complex debilitating disorder of the gastrointestinal tract which comprises both Crohn’s disease and ulcerative colitis. Despite the unclear etiology of IBD, several factors have been accounted as key for the development of the disease, such as genetics, immune system and environmental factors, namely diet and gut microbiota composition (Khor et al., 2011).
Dietary supplementation has emerged as a promising therapeutic practice in the prevention and treatment of IBD (Durchschein et al., 2016). Recent evidence has revealed that fiber-enriched diets promote protection against IBD development, since dietary fiber is mainly fermented by intestinal microbiota into short-chain fatty acids (SCFAs), such as butyrate, acetate and propionate (den Besten et al., 2013). The protective properties of these metabolites are widely described by their impact on immune cell activation and epithelial barrier stability (Kelly et al., 2015; Macia et al., 2015), with decreased levels of SCFAs being found in colon samples from IBD patients (Huda-Faujan et al., 2010). Other studies have also pointed out several specific amino acids that can improve intestinal homeostasis, mainly by boosting mucosal healing and regeneration. For instance, glutamine is known to promote protection in dextran sulfate sodium (DSS)- and 2,4,6-trinitrobenzenesulfonic acid (TBNS)-induced intestinal inflammation, acting via NF-κB downregulation (Kretzmann et al., 2008). Colitic mice orally administered with glutamine displayed suppressive Th1/Th17 immune responses and subsequently decreased inflammation when compared to mice fed with regular diet (Hsiung et al., 2014). Other amino acids have been also associated with a protective phenotype against colitis. Using distinct animal models of colitis, diets enriched in threonine, serine, proline and cysteine, given before and throughout disease development, have been shown to restore mucin synthesis and stabilization of gut microbiota (Faure et al., 2006). Similar findings were observed with the administration of a mixture of threonine, methionine and monosodium glutamate after colitis induction (Liu et al., 2013).
Several studies have so far addressed whether administration of specific nutrients may arise as a prophylactic and/or therapeutic approach. However, there is a lack of knowledge toward the most promising and adequate phase of action. Thus, we investigated the metabolic profile of mice developing colitis, aiming to identify variation of metabolites during inflammation. The identification of the most attractive potential targets for therapy and the definition of a time range more prone to potentiate the effects of their supplementation may be relevant for future applications in IBD prophylaxis and therapy.
Materials and Methods
Animals
Seven to nine-week old C57BL/6J male mice were purchased from Charles River Laboratories and housed in i3S animal facilities, under pathogen free conditions, with food and water ad libitum. All experimental procedures were approved by the i3S Animal Ethics Committee and licensed by the Portuguese National Authority for Animal Health (DGAV) with reference 014811/2016-07-13.
Colitis Induction
Dextran sulfate sodium (DSS; TdB Consultancy; 2% (w/v), molecular weight approximately 40000 Da) was administered in drinking water ad libitum for 5 days. Clinical signs of colitis were monitored daily and scored as a disease activity index (DAI; Supplementary Table S1).
L-Threonine Administration
Mice were divided into DSS (control), DSS with L-Threonine (DSS + Thr) and DSS followed by L-Threonine administration at day 8 (DSS + Thr D8), as shown in Figure 2A. L-Threonine [Thr; Sigma–Aldrich; 0.166% (w/v) corresponding to 250 mg/Kg/day] was given in the drinking water ad libitum. The dose was chosen according to the daily intake in previous studies with rodents (Faure et al., 2006; Liu et al., 2013) and to a high-threonine human supplementation study (Pencharz et al., 2008). The safety of our protocol measuring biomarkers of renal and liver damage was evaluated to confirm the absence of toxicity (Supplementary Figure S1). Similar fluid intake was found among all groups.
Metabolomic Analysis by Nuclear Magnetic Resonance (NMR)
Methanol/water extracts of colon were analyzed at an UltrashiedTM 800 Plus (Bruker) spectrometer as described in Graça et al. (2017). Metabolite concentrations were performed by integration of 1H-NMR resonances using TSP as reference.
Quantitative Real-Time PCR (qPCR)
Total RNA was isolated from colonic samples (TripleXtractor, Grisp). As DSS inhibits both polymerase and reverse transcriptase activities, RNA was purified with lithium chloride, as in Viennois et al. (2013). qPCR was performed as described in Correia et al. (2017). The list of primers used is in Supplementary Table S2.
Histology and Goblet Cell Count
Colons were fixed in 10% buffered formalin (Sigma–Aldrich) and embedded in paraffin. Sections of 5 μm were stained with hematoxylin/eosin and Alcian Blue/Periodic acid-Schiff. Goblet cell number was assessed for each experimental condition in a blinded fashion. Only crypts cut longitudinally from crypt opening to bottom were analyzed.
Cytokine Quantification
Colonic explant cultures were performed as previously described (McNamee et al., 2011). Cytokine quantification was performed in supernatants by ELISA (Biolegend). Tissue explants were homogenized and total protein was measured using Bradford assay. The concentration of secreted cytokines in the supernatant was normalized to total tissue protein and expressed as picogram of cytokine per μg of total tissue protein.
Statistical Analysis
Metabolite differences were evaluated by ANOVA in R statistical software. Partial least squares – discriminant analysis (PLS-DA) models were performed in SIMCA software. Other statistical analyses were carried out with GraphPad Prism (version 6.01). For multiple group comparisons one-way ANOVA test with a Tukey multiple-comparison posttest was performed, while for multiple group comparisons with repeated measures two-way ANOVA test with a Tukey multiple-comparison posttest was applied. Data are presented as mean ± standard deviation (SD). Statistically significant values are: ∗p < 0.05; ∗∗p < 0.01; ∗∗∗p < 0.001.
Results and Discussion
To characterize the colon metabolic profile during colitis development, metabolites present on colonic extracts of mice prior (day 0) and after 5 days of DSS-induced colitis were analyzed by NMR (Figure 1A). These time points were selected since it allows the comparison of a homeostatic profile (day 0) against a period with established inflammation and lesion, yet reversible and treatable (day 5). Multivariate analyses of the metabolomic data only demonstrated minor alterations between profiles for day 0 and day 5 of colitis development (Figure 1B). However, univariate analyses performed on the metabolites allow to discriminate ADP and particularly threonine as significantly altered from day 0 to day 5 (Figures 1C,D). Among essential amino acids, threonine has a prominent role in maintaining a healthy gut. Threonine is able to generate the main three SCFAs, namely acetate, butyrate and propionate (Neis et al., 2015). In fact, it has been previously identified several biosynthetic genes for threonine metabolism in the human gut microbiota, suggesting the relevance of this amino acid for microbiota biology (Abubucker et al., 2012). SCFAs are described as important modulators of immune response, since they are ligands for G-protein-coupled receptor 43 (GPR43) that is expressed by immune cells on the lamina propria, such as regulatory T cells, regulating the proinflammatory responses in the intestine (Bollrath and Powrie, 2013). Moreover, threonine is vastly metabolized in the intestine for mucin synthesis (Faure et al., 2005). These proteins are paramount in intestinal stability, since the mucus layer in the colonic outer layer prevents the direct contact of luminal microorganisms with the epithelium (Johansson et al., 2014). It has also been suggested that threonine requirements are increased under pathological settings to maintain proper intestinal function, such as production and formation of the mucus layer (Remond et al., 2009). Therefore, by participating in the mucus layer synthesis and production of anti-inflammatory SCFAs, threonine metabolism by gut microbiota proves to be essential for gut barrier integrity and function. Our data show that threonine levels drop 2-fold during colitis development until day 5 (Figures 1C–E). Therefore, we hypothesized that threonine supplementation during active inflammatory disease could help to restore the intestinal homeostasis and thus present some therapeutic potential.
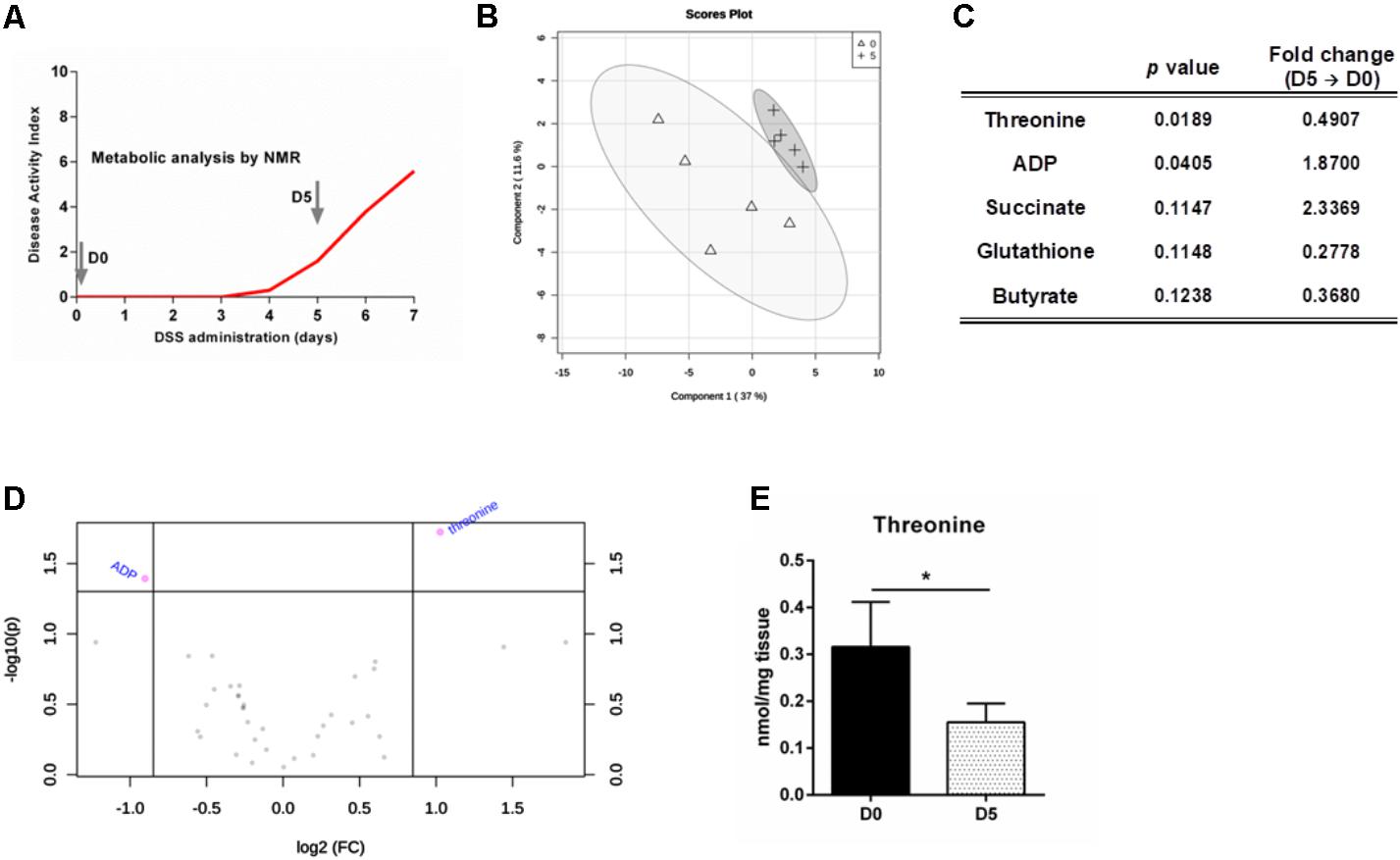
FIGURE 1. Threonine levels in the colon decrease with intestinal inflammation. (A) Mice were treated with DSS to induce colitis and colon metabolites were measured at day 0 and day 5 by NMR. (B) Partial least squares – discriminant analysis (PLS-DA) was obtained for samples of both days. (C) Top-five features identified by the combination of the p-value from t-test analysis and magnitude of the change (fold change from day 5 versus day 0). (D) Important metabolites selected by volcano plot with fold change threshold (x) 1.8 and t-test threshold (y) of 0.05. The purple circles represent features above the threshold. Both fold changes and p-values are log transformed. (E) Threonine levels in the colon at day 0 and day 5. Data is shown as mean ± SD; n = 5 mice/group. One representative experiment is shown out of two. ∗p < 0.05.
Previous studies have investigated threonine in combination with other amino acids as a potential candidate for therapy against colitis (Faure et al., 2006; Liu et al., 2013). Nevertheless, not only single threonine supplementation was not evaluated before, but also there is scarce evidence regarding the most adequate time frame for its supplementation in colitis treatment. Accordingly, we addressed threonine supplementation in two distinct phases: (1) in parallel with the initiation of the inflammatory process, i.e., simultaneously with DSS administration (DSS + Thr), and (2) on day 8, when inflammation is already established (DSS + Thr D8). Mice subjected to DSS-induced colitis but without threonine supply were used as control (DSS) (Figure 2A). We observed that the three groups developed colitis with a similar progression profile. Nevertheless, after this time point, the recovery profile of colitic mice supplemented with threonine in the drinking water (DSS + Thr) was slower than that of the other two groups, showing statistically significant differences from day 10 to the final day of experiment when compared to control group (DSS). Besides, DSS + Thr mice had also a distinctive DAI score at day 11 and 12 when compared with mice that only received threonine after day 8 (DSS + Thr D8) (Figure 2B). Despite the divergent phenotype, no major differences were found in colon length neither in the intestinal permeability (Supplementary Figure S1).
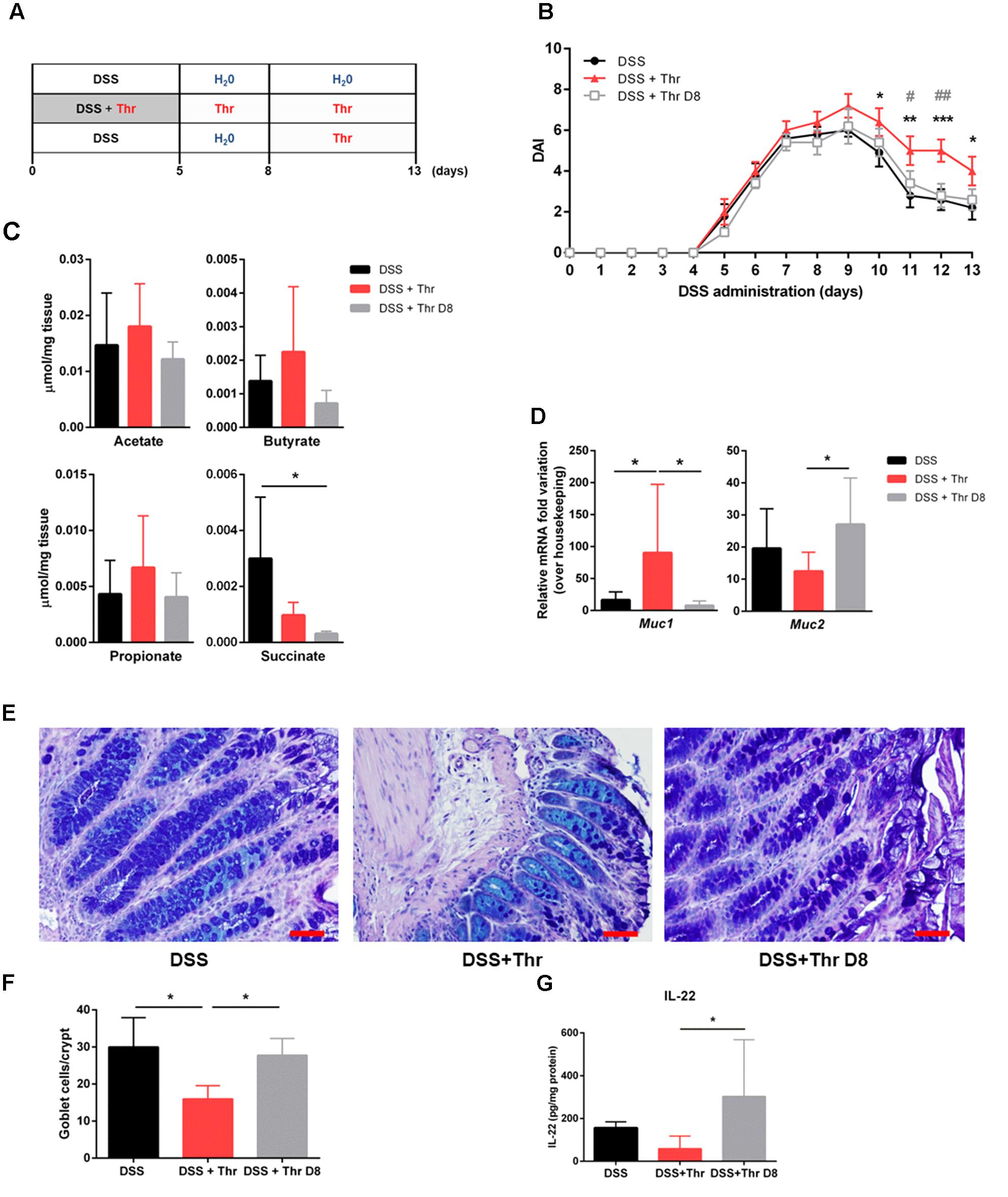
FIGURE 2. Threonine supplementation on the onset of colitis development delays recovery and decreases goblet cell number. (A) Mice were supplemented with threonine in the beginning of colitis induction (DSS + Thr) or after established inflammation (DSS + Thr D8). Mice treated only for colitis induction were used as control (DSS). (B) Disease progression was assessed by scoring the disease activity index (DAI) throughout the experiment. (C) Quantification of short-chain fatty acids (acetate, butyrate, propionate) and succinate by NMR was performed at day 13 of experiment. (D) Expression of mucin-encoding genes Muc1 and Muc2 was performed by qPCR at day 13. (E) Colonic tissue sections were stained with Alcian Blue/periodic acid-Schiff. (F) Quantification of goblet cell number per crypt. Scale bar = 50 μm. (G) IL-22 quantification on colonic explant cultures by ELISA. Data is shown as mean ± SD. n = 5 mice/group. One representative experiment is shown out of two. The symbol ∗ corresponds to statistical differences between DSS + Thr and DSS; while # is related to statistical differences between DSS + Thr and DSS + Thr D8. ∗p < 0.05; ∗∗p < 0.01; ∗∗∗p < 0.001.
Threonine administration was surprisingly detrimental for disease recovery when given at the setting of the inflammatory process. To understand this phenotype, we first evaluated the potential alterations in the colon metabolic profile among the different groups. No significant differences were found between most of the metabolites. Particularly, the levels of the major SCFAs (i.e., acetate, butyrate, and propionate), normally associated with a protective phenotype, were similar among groups. Only succinate levels, which is as an important marker of inflammation promoting IL-1β induction in inflammatory contexts (Tannahill et al., 2013), were found to be markedly decreased in DSS + Thr D8 mice (Figure 2C). No major alterations were observed in the inflammatory infiltrate profile, tissue organization and hepatic and renal toxicity serum biomarkers (Supplementary Figure S1).
Threonine plays a major role in mucin synthesis and consequently in the formation of the mucus layer. Indeed, lack of threonine is also known to impair intestinal paracellular permeability and is associated with fewer goblet cells and mucus synthesis (Faure et al., 2005; Mao et al., 2011). The mucus layer serves as a barrier against microbial translocation to the lamina propria and therefore its integrity is paramount for intestinal homeostasis. When we analyzed the expression of mucin-encoding genes, we found that Muc2 expression is decreased in DSS + Thr mice when compared to mice that only received threonine at day 8 (DSS + Thr D8) (Figure 2D). Muc2 encodes for the oligomeric mucus gel-forming mucin 2 protein that is the major responsible for mucus synthesis. Indeed, impairment or total absence of the mucus layer is associated with severe colitis, as observed in Muc2-deficient mice (Van der Sluis et al., 2006). We also found that DSS + Thr mice display higher expression of Muc1, which has shown to contribute to intestinal inflammation and colon cancer progression (Baldus et al., 2004; Takahashi et al., 2015). We next quantified the number of goblet cells of the colonic mucosa. These are a secretory epithelial cell lineage found in both the small and the large intestines, whose major function is the production of mucus. By analyzing colon slices stained with Alcian Blue/Periodic acid-Schiff, we observed that DSS + Thr mice displayed significantly fewer goblet cells when compared to both DSS and DSS + Thr D8 (Figures 2E,F). Therefore, our results suggest that an alteration in mucus synthesis due to threonine administration during the onset of disease may impact intestinal integrity, by delaying the recovery of disease. Faure et al. (2006) have demonstrated that supplementation with diet containing higher doses of amino acids, including L-threonine, lead to an increase in goblet cell number, regulated mucin production in the colon and restored microbiota composition after DSS treatment in rats. Notwithstanding, not only the animal model is different, but also L-threonine was given before colitis induction, which may be underlying the distinctive outcome.
Previous studies have linked several cytokines to mucus production in the intestine (Parks et al., 2015). To examine the immunological profile of the three groups, cytokine levels in the colon were quantified. No major changes were observed between the groups for interleukin (IL)-1β, IL-12p70, IL-10, IL-17A/F and granulocyte-macrophage colony-stimulating factor (GM-CSF) levels (Supplementary Figure S2). Notwithstanding, the amount of IL-22 was significantly decreased in DSS + Thr mice when compared to DSS + Thr D8 mice (Figure 2G). IL-22 is a member of the IL-10 family of cytokines and has been vastly studied in the context of intestinal homeostasis. It can be produced by several cell types, such as T helper (Th1) 1, Th17, Th22 and innate lymphoid cells (ILCs), and present several roles in the gastrointestinal tract, such as tissue regeneration and maintenance of the intestinal epithelial barrier (Rutz et al., 2013). Thus, the decreased IL-22 levels may be associated with delayed recovery of the intestinal balance.
Overall, our data demonstrate that supplementation of threonine during colitis induction impairs goblet cell number, with concomitant decreased Muc2 expression and IL-22 production. These variations are likely to be the cause of delayed recovery observed in this situation. Interestingly, these effects are not seen when threonine is administered once colitis is established. Acute DSS-induced colitis is known to promote gut microbial dysbiosis (Munyaka et al., 2016). Threonine is metabolized by some intestinal commensal bacteria, leading to the production of several metabolites used for intestinal maintenance and to mediate immune responses (Neis et al., 2015). Thus, threonine supplementation during induction of colitis may impact differently the colonic microbiota populations present during the onset and upon the establishment of inflammation, having ultimately distinct effects in intestinal function. Further understanding of the mechanisms underlying threonine supplementation may give new insights on how dietary nutrients modulate the dynamic balance between microbiome, immune response and barrier function.
Ethics Statement
This study was carried out in accordance with the recommendations of European Council Directive (2010/63/EU) guidelines that where transposed into Portuguese law (Decree-Law n.°113/2013, August 7th), i3S Animal Ethics Committee and licensed by the Portuguese National Authority for Animal Health (DGAV). The protocol was approved by the i3S Animal Ethics Committee and licensed by the Portuguese National Authority for Animal Health (DGAV) with reference 014811/2016-07-13.
Author Contributions
JG, ET, CC, AgC, FR, MS, AnC, and RS designed the experiments. JG, LG, RD-O, and RS performed the experiments. JG, LG, RD-O, and RS analyzed the data. JG, LG, RD-O, and RS interpreted the results. JG and RS drafted the manuscript and prepared the tables and figures. JG, LG, RD-O, ET, CC, AgC, FR, MS, AnC, and RS revised the paper and approved the final version of the manuscript.
Funding
This work was supported by the Northern Portugal Regional Operational Programme (NORTE 2020), under the Portugal 2020 Partnership Agreement, through the European Regional Development Fund (FEDER) (NORTE-01-0145-FEDER-000013) and the Fundação para a Ciência e Tecnologia (FCT) (contracts PD/BD/106053/2015 to JG via Inter-University Doctoral Programme in Ageing and Chronic Disease – PhDOC, IF/00021/2014 to RS, IF/01390/2014 to ET, IF/01147/2013 to RD-O, IF/00735/2014 to AgC, SFRH/BPD/96176/2013 to CC, and SFRH/BPD/111100/2015 to LG). MS is a FCT investigator. The NMR data was acquired at CERMAX (Centro de Ressonância Magnética António Xavier) which is a member of the National NMR network with the support of Project LISBOA-01-0145-FEDER-007660.
Conflict of Interest Statement
The authors declare that the research was conducted in the absence of any commercial or financial relationships that could be construed as a potential conflict of interest.
The reviewer DS and handling Editor declared their shared affiliation at the time of the review.
Supplementary Material
The Supplementary Material for this article can be found online at: https://www.frontiersin.org/articles/10.3389/fphys.2018.01247/full#supplementary-material
References
Abubucker, S., Segata, N., Goll, J., Schubert, A. M., Izard, J., Cantarel, B. L., et al. (2012). Metabolic reconstruction for metagenomic data and its application to the human microbiome. PLoS Comput. Biol. 8:e1002358. doi: 10.1371/journal.pcbi.1002358
Baldus, S. E., Monig, S. P., Huxel, S., Landsberg, S., Hanisch, F. G., Engelmann, K., et al. (2004). MUC1 and nuclear beta-catenin are coexpressed at the invasion front of colorectal carcinomas and are both correlated with tumor prognosis. Clin. Cancer Res. 10, 2790–2796. doi: 10.1158/1078-0432.CCR-03-0163
Bollrath, J., and Powrie, F. (2013). Immunology. Feed your Tregs more fiber. Science 341, 463–464. doi: 10.1126/science.1242674
Correia, C. R., Gaifem, J., Oliveira, M. B., Silvestre, R., and Mano, J. F. (2017). The influence of surface modified poly(l-lactic acid) films on the differentiation of human monocytes into macrophages. Biomater. Sci. 5, 551–560. doi: 10.1039/c6bm00920d
den Besten, G., van Eunen, K., Groen, A. K., Venema, K., Reijngoud, D. J., and Bakker, B. M. (2013). The role of short-chain fatty acids in the interplay between diet, gut microbiota, and host energy metabolism. J. Lipid Res. 54, 2325–2340. doi: 10.1194/jlr.R036012
Durchschein, F., Petritsch, W., and Hammer, H. F. (2016). Diet therapy for inflammatory bowel diseases: the established and the new. World J. Gastroenterol. 22, 2179–2194. doi: 10.3748/wjg.v22.i7.2179
Faure, M., Mettraux, C., Moennoz, D., Godin, J. P., Vuichoud, J., Rochat, F., et al. (2006). Specific amino acids increase mucin synthesis and microbiota in dextran sulfate sodium-treated rats. J. Nutr. 136, 1558–1564. doi: 10.1093/jn/136.6.1558
Faure, M., Moennoz, D., Montigon, F., Mettraux, C., Breuille, D., and Ballevre, O. (2005). Dietary threonine restriction specifically reduces intestinal mucin synthesis in rats. J. Nutr. 135, 486–491. doi: 10.1093/jn/135.3.486
Graça, G., Desterro, J., Sousa, J., Fonseca, C., Silveira, M., Serpa, J., et al. (2017). Identification of putative biomarkers for leptomeningeal invasion in B-cell non-Hodgkin lymphoma by NMR metabolomics. Metabolomics 13:136. doi: 10.1007/s11306-017-1269-9
Hsiung, Y. C., Liu, J. J., Hou, Y. C., Yeh, C. L., and Yeh, S. L. (2014). Effects of dietary glutamine on the homeostasis of CD4+ T cells in mice with dextran sulfate sodium-induced acute colitis. PLoS One 9:e84410. doi: 10.1371/journal.pone.0084410
Huda-Faujan, N., Abdulamir, A. S., Fatimah, A. B., Anas, O. M., Shuhaimi, M., Yazid, A. M., et al. (2010). The impact of the level of the intestinal short chain Fatty acids in inflammatory bowel disease patients versus healthy subjects. Open Biochem. J. 4, 53–58. doi: 10.2174/1874091x01004010053
Johansson, M. E., Gustafsson, J. K., Holmen-Larsson, J., Jabbar, K. S., Xia, L., Xu, H., et al. (2014). Bacteria penetrate the normally impenetrable inner colon mucus layer in both murine colitis models and patients with ulcerative colitis. Gut 63, 281–291. doi: 10.1136/gutjnl-2012-303207
Kelly, C. J., Zheng, L., Campbell, E. L., Saeedi, B., Scholz, C. C., Bayless, A. J., et al. (2015). Crosstalk between microbiota-derived short-chain fatty acids and intestinal epithelial HIF augments tissue barrier function. Cell Host Microbe 17, 662–671. doi: 10.1016/j.chom.2015.03.005
Khor, B., Gardet, A., and Xavier, R. J. (2011). Genetics and pathogenesis of inflammatory bowel disease. Nature 474, 307–317. doi: 10.1038/nature10209
Kretzmann, N. A., Fillmann, H., Mauriz, J. L., Marroni, C. A., Marroni, N., Gonzalez-Gallego, J., et al. (2008). Effects of glutamine on proinflammatory gene expression and activation of nuclear factor kappa B and signal transducers and activators of transcription in TNBS-induced colitis. Inflamm. Bowel Dis. 14, 1504–1513. doi: 10.1002/ibd.20543
Liu, X., Beaumont, M., Walker, F., Chaumontet, C., Andriamihaja, M., Matsumoto, H., et al. (2013). Beneficial effects of an amino acid mixture on colonic mucosal healing in rats. Inflamm. Bowel Dis. 19, 2895–2905. doi: 10.1097/01.MIB.0000435849.17263.c5
Macia, L., Tan, J., Vieira, A. T., Leach, K., Stanley, D., Luong, S., et al. (2015). Metabolite-sensing receptors GPR43 and GPR109A facilitate dietary fibre-induced gut homeostasis through regulation of the inflammasome. Nat. Commun. 6:6734. doi: 10.1038/ncomms7734
Mao, X., Zeng, X., Qiao, S., Wu, G., and Li, D. (2011). Specific roles of threonine in intestinal mucosal integrity and barrier function. Front. Biosci. 3:1192–1200.
McNamee, E. N., Masterson, J. C., Jedlicka, P., McManus, M., Grenz, A., Collins, C. B., et al. (2011). Interleukin 37 expression protects mice from colitis. Proc. Natl. Acad. Sci. U.S.A. 108, 16711–16716. doi: 10.1073/pnas.1111982108
Munyaka, P. M., Rabbi, M. F., Khafipour, E., and Ghia, J. E. (2016). Acute dextran sulfate sodium (DSS)-induced colitis promotes gut microbial dysbiosis in mice. J. Basic Microbiol. 56, 986–998. doi: 10.1002/jobm.201500726
Neis, E. P., Dejong, C. H., and Rensen, S. S. (2015). The role of microbial amino acid metabolism in host metabolism. Nutrients 7, 2930–2946. doi: 10.3390/nu7042930
Parks, O. B., Pociask, D. A., Hodzic, Z., Kolls, J. K., and Good, M. (2015). Interleukin-22 signaling in the regulation of intestinal health and disease. Front. Cell Dev. Biol. 3:85. doi: 10.3389/fcell.2015.00085
Pencharz, P. B., Elango, R., and Ball, R. O. (2008). An approach to defining the upper safe limits of amino acid intake. J. Nutr. 138, 1996s–2002s. doi: 10.1093/jn/138.10.1996S
Remond, D., Buffiere, C., Godin, J. P., Mirand, P. P., Obled, C., Papet, I., et al. (2009). Intestinal inflammation increases gastrointestinal threonine uptake and mucin synthesis in enterally fed minipigs. J. Nutr. 139, 720–726. doi: 10.3945/jn.108.101675
Rutz, S., Eidenschenk, C., and Ouyang, W. (2013). IL-22, not simply a Th17 cytokine. Immunol. Rev. 252, 116–132. doi: 10.1111/imr.12027
Takahashi, H., Jin, C., Rajabi, H., Pitroda, S., Alam, M., Ahmad, R., et al. (2015). MUC1-C activates the TAK1 inflammatory pathway in colon cancer. Oncogene 34, 5187–5197. doi: 10.1038/onc.2014.442
Tannahill, G. M., Curtis, A. M., Adamik, J., Palsson-McDermott, E. M., McGettrick, A. F., Goel, G., et al. (2013). Succinate is an inflammatory signal that induces IL-1beta through HIF-1alpha. Nature 496, 238–242. doi: 10.1038/nature11986
Van der Sluis, M., De Koning, B. A., De Bruijn, A. C., Velcich, A., Meijerink, J. P., Van Goudoever, J. B., et al. (2006). Muc2-deficient mice spontaneously develop colitis, indicating that MUC2 is critical for colonic protection. Gastroenterology 131, 117–129. doi: 10.1053/j.gastro.2006.04.020
Keywords: IBD, threonine, DSS-induced colitis, goblet cells, metabolomics, IL-22, mucin
Citation: Gaifem J, Gonçalves LG, Dinis-Oliveira RJ, Cunha C, Carvalho A, Torrado E, Rodrigues F, Saraiva M, Castro AG and Silvestre R (2018) L-Threonine Supplementation During Colitis Onset Delays Disease Recovery. Front. Physiol. 9:1247. doi: 10.3389/fphys.2018.01247
Received: 09 March 2018; Accepted: 17 August 2018;
Published: 05 September 2018.
Edited by:
Maria Teresa Cruz, University of Coimbra, PortugalReviewed by:
Vicente Lahera, Complutense University of Madrid, SpainDiana Jurado Serra, University of Coimbra, Portugal
Copyright © 2018 Gaifem, Gonçalves, Dinis-Oliveira, Cunha, Carvalho, Torrado, Rodrigues, Saraiva, Castro and Silvestre. This is an open-access article distributed under the terms of the Creative Commons Attribution License (CC BY). The use, distribution or reproduction in other forums is permitted, provided the original author(s) and the copyright owner(s) are credited and that the original publication in this journal is cited, in accordance with accepted academic practice. No use, distribution or reproduction is permitted which does not comply with these terms.
*Correspondence: Ricardo Silvestre, cmljYXJkb3NpbHZlc3RyZUBtZWQudW1pbmhvLnB0