- 1Department of Blood Transfusion, Chinese PLA General Hospital, Beijing, China
- 2Department of Blood Transfusion, Air Force General Hospital, PLA, Beijing, China
- 3Department of High Performance Computing Technology and Application Development, Computer Network Information Center, Chinese Academy of Sciences, Beijing, China
- 4University of Chinese Academy of Sciences, Beijing, China
- 5Guizhou University School of Medicine, Guiyang, China
- 6Clinical Laboratory, Hainan Branch of PLA General Hospital, Hainan, China
Background: The number of red blood cells (RBCs) increases significantly in response to high-altitude hypoxic environments, and the RBC microRNA (miRNA) expression pattern is similar to that in whole blood. Studies have shown that miRNA in plasma can act as a circulating hypoxia-associated marker, but the effect of a high-altitude hypoxic environment on RBC-derived miRNAs has not yet been reported.
Methods: Blood samples were collected from 20 Han Chinese individuals residing at 500 m (Sichuan Han), 10 migrant Han Chinese citizens residing at 3,658 m (Tibet Han) and 12 native Tibetans, and RBC indices measurements and miRNA sequencing analyses were performed for the three sample groups. The levels of some markedly altered miRNAs at high altitude were subsequently measured from 5 randomly selected samples of each group by real-time PCR. Bioinformatic analyses was performed to determine the potential target genes of selected hypoxia-associated miRNAs.
Results: Marked changes of several RBC indices were observed among the Tibet Han population, the Tibetan population and the Sichuan Han population. A total of 516 miRNAs derived from RBCs were initially identified by miRNA sequencing in the three sample groups. Compared with the Sichuan Han population, 49 miRNAs were differentially expressed in the Tibet Han population (17 upregulated and 32 downregulated). 12 upregulated and 21 downregulated miRNAs were observed in the Tibetan population compared with the Sichuan Han population. A total of 40 RBC miRNAs were differentially expressed in the Tibetan population (15 upregulated and 25 downregulated) compared with the Tibet Han population. Two significantly altered miRNAs with the highest expression levels (miRNA-144-5p and miR-30b-5p) were selected for real-time PCR analysis, and the results were consistent with those of miRNA sequencing. Furthermore, bioinformatic analyses showed that some potential target genes of miR-144-5p and miR-30b-5p are involved in the erythroid- hypoxia-, and nitric oxide (NO)-related signaling pathways in response to hypoxia.
Conclusion: Our findings provide clear evidence, for the first time, that a high-altitude hypoxic environment significantly affects human RBC miRNA profiles.
Introduction
The most extreme environment that people face is the hypoxic environment of the Tibet Plateau. The Tibet Plateau is the highest plateau in the world with a mean altitude of over 4,500 m. People who live at high altitude for a long time are prone to high-altitude polycythemia, high-altitude heart disease, plateau depression and other chronic altitude diseases (Simonson et al., 2010; He et al., 2013; Huertasánchez et al., 2014). Studies have shown that genotype variation at several unique genes (e.g., EGLN1, PPARA, and EPAS1) provide genetic contribution to an individual's adaptation to high-altitude hypoxic environments (Simonson et al., 2010; Yi et al., 2010). However, the epigenetic basis of this adaptation remains unknown.
The current literature shows that hypoxia is an important proximal regulator of miRNA production and function and that miRNAs can rapidly respond to hypoxia-induced physiological changes in the cell (Yan et al., 2009; Nallamshetty et al., 2013; Rupaimoole et al., 2014). Recently, some reports have revealed that circulating miRNA concentrations play an important role in the response to hypoxia at high altitudes. Yan et al. showed that the miRNA expression of plasma and peripheral blood cells is significantly different in high-altitude hypoxic environments (Yan et al., 2015). Their follow-up work confirmed that the miR-210-3p level is significantly altered in the peripheral blood cells of a Tibet Han group compared with a Han group (Yan et al., 2016). Most recent research suggests that miRNA expression levels in the plasma are associated with the occurrence of acute mountain sickness (Liu et al., 2017). In a high-altitude hypoxic environment, the number of RBCs changes significantly to enable adaptation to hypoxia. However, the impact of high-altitude hypoxia on RBC-derived miRNAs remains unclear.
Several studies have reported that the whole blood miRNA expression pattern is similar to that of RBCs but not leukocytes (Chen et al., 2008; Kannan and Atreya, 2010; Wu et al., 2017). Chen et al. also showed that miRNA profiles in human reticulocytes were very different from those in mature RBCs, indicating that a diverse population of miRNAs are selectively retained in RBCs, as opposed to being a random remnant (Chen et al., 2008; Hamilton, 2010). Among the hundreds of RBC miRNAs, the most abundant are miR-451a, miR-144-3p, miR-16, miR-92a, let-7, miR-486-5p (Doss et al., 2015). miR-451 and miR-144 are upregulated by GATA and play crucial roles in erythroid development (Rasmussen et al., 2010). miR-16-5p, miR-451a-5p, miR-486-5p, and miR-92a-3p are the most abundant intracellular Ago2-bound miRNAs observed in 24-h-stored RBCs, and functional enrichment analysis of mRNA targets of these major miRNAs have identified molecules related to various diseases, biological functions, and toxicity functions (e.g., cardio-, hepato-, and nephrotoxicity) (Vu et al., 2017). Consequently, selective retention of active miRNAs and the existence of Ago2-miRNA in RBCs indicate that RBC-derived miRNAs likely have specific functions.
Interestingly, several observations provide direct evidence defining the important function of miRNAs in RBCs (Regev-Rudzki et al., 2013; Mantel et al., 2016; Wang et al., 2017). For instance, studies have shown that the Ago2-miR-451 complex derived from infected RBCs is taken up by endothelial cells and can alter vascular function through RBC extracellular vesicles in malaria, even though RBCs lack the target mRNAs of miRNAs inside the cells (Mantel et al., 2016). Therefore, more attention should be directed toward understanding RBC miRNAs. It has been reported that RBC miRNAs not only inhibit the reproduction of Plasmodium falciparum in patients with sickle cell anemia but are also associated with the development of bodily disorders (Lamonte et al., 2012; Duan et al., 2016, 2017; Walzer and Chi, 2017; Wu et al., 2017). In this study, we hypothesized that high-altitude hypoxic environments likely impact RBC miRNA expression levels.
To test our hypothesis, RBC indices of people residing in the low- and high-altitude areas were measured. miRNA sequencing screening and real-time PCR confirmation assays were performed to systematically and comprehensively evaluate and compare RBC miRNA profiles between Han Chinese residing in Sichuan (at 500 m) and Han Chinese who migrated from the plain area to Tibet (Tibet Han, at 3,658 m) as well as the ethnic Tibetan population.
Methods
Participants and RBC Sample Processing
A total of 42 blood samples were collected from 20 Han Chinese residing in Chengdu, Sichuan Province (Sichuan Han) at an altitude of 500 m, 10 ethnic Han Chinese who migrated approximately 15 years ago from the plains to Lhasa (Tibet Han), and 12 native Tibetans who resided in Lhasa (Tibetan), a city in the Tibet Autonomous Region located at an altitude of 3,658 m. All of the participants were male and healthy, and written informed consent was obtained from all. Blood samples (~4 ml) were collected in tubes containing EDTA using a standard procedure. The study protocol was approved by the Ethics Committee of Chinese PLA General Hospital, in accordance with the Declaration of Helsinki.
Whole blood was centrifuged for 5 min at 2,500 rpm. The plasma and buffy coat layers were aspirated for initial removal of plasma, leukocytes and platelets. Mature RBCs were then passed through filter membranes for leukocyte removal and washed three times with phosphate-buffered saline (PBS) to obtain purified mature RBCs. We routinely assessed this material for residual platelet (PLT) and white blood cell (WBC) contamination using a hematology analyzer (BC-2800, MINDRAY, China). Typical readings indicating lack of contamination of these cells were approximately 0 WBCs/L and 0 PLTs/L. The purity of the resulting RBCs was verified by FACS for surface expression of CD235a (RBC, CD235a-FITC, Pharmingen, CA), CD45 (leukocyte, CD45-PE, Pharmingen, CA) and CD41a (platelet, CD41a-PE, Pharmingen, CA) using fluorescently labeled antibodies. We randomly selected purified RBCs for testing and the mean positive rates of CD235a, CD45 and CD41a were 98.1, 0.35, and 0.1%, respectively.
RNA Isolation and miRNA Sequencing Analysis
Total RNA was extracted from 100 μl purified RBCs of each sample using the TRIzol reagent (Invitrogen, CA, USA) according to the manufacturer's protocol. The Quantity and purity of the total RNA were measured using an RNA 6000 Nano Lab Chip Kit and Bioanalyzer 2100 (Agilent, CA, USA). Approximately 1 μg of total RNA was used to prepare a small RNA library according to the TruSeq Small RNA Sample Prep Kit protocol (Illumina, CA, USA), and single-end sequencing (36 bp) was performed on an Illumina HiSeq 2500 at LC-BIO (Hangzhou, China) according to the manufacturer's recommended guideline. All primary sequencing data have been deposited in the Gene Expression Omnibus (GEO dataset; GSE117436).
The raw reads were subjected to the Illumina pipeline filter (Solexa 0.3), and the dataset was further processed with an in-house program, ACGT101-miR (LC Sciences, Texas, USA), to remove adapter dimers, junk, low complexity molecules, common RNA families (rRNA, tRNA, snRNA, and snoRNA) and repeats. Unique sequences with 18–26 nucleotides in length were mapped to specific species precursors in miRBase 20.0 using a BLAST search to identify known miRNAs. miRNA differential expression based on normalized deep-sequencing counts was analyzed by selectively using Fisher's exact test, the Chi-squared 2X2 test, the Chi-squared nXn test, Student's t-test, and ANOVA depending on the experimental design. miRNAs with markedly altered expression levels between two groups were identified using fold change and p-value cutoffs of 2 and 0.05 for each test, respectively.
Real-Time PCR
Total RNA was extracted from RBCs using the miRcute miRNA isolation kit (TIANGEN, China) according to the manufacturer's instructions. RNA (1 μg) was reverse transcribed into cDNA using the miRcute Plus miRNA First-Strand cDNA Synthesis Kit (TIANGEN, China). Real-time PCR for miR-144-5p and miR-30b-5p was performed using the SYBR Green reagent (TIANGEN, China); U6 was used as the internal control. The relative amounts of miRNA or mRNA were determined using the comparative threshold cycle (CT) method.
Hematology Factor Analysis
Hematological indices, including RBC count, hemoglobin concentration (HGB), hematocrit (HCT), mean corpuscular volume (MCV), mean corpuscular hemoglobin (MCH), mean corpuscular hemoglobin concentration (MCHC), WBC counts and PLT levels, were measured immediately after blood collection using commercial reagents with a BC-2800 analyzer (MINDRAY, China).
Prediction of Target Genes of miRNAs
Two computational target prediction algorithms (TargetScan 50 and miRanda 3.3a) were used to identify miRNA binding sites to predict genes targeted by differentially expressed miRNAs. The data predicted by both algorithms were combined, and overlaps were calculated. GO (Gene Ontology) terms and KEGG (Kyoto Encyclopedia of Genes and Genomes) pathways of the differentially expressed miRNA targets were annotated.
Data Analysis
All analyses were performed using GraphPad Prism (version 5). Differences in variants among the groups were analyzed using one-way ANOVA or Student's t-test; p < 0.05 was considered statistically significant. Error bars indicate the standard deviation (SD). Data are expressed as the mean ± SD.
Results
Hematological Features of Participants
All participants in the Sichuan Han group, Tibet Han group, and Tibetan group were male; their ages were 24.3 ± 4.6 years, 40.1 ± 6.8 years, and 34.5 ± 12.3 years, respectively. The levels of RBCs (Figure 1A), HGB (Figure 1B), HCT (Figure 1C), and MCHC (Figure 1D) were significantly higher in the Tibet Han group than in the Sichuan Han group (p < 0.05). However, the values of RBCs, HGB and HCT were lower in the Tibetan group than in the Tibet Han group. No marked differences between the Tibet Han group and the Sichuan Han group were found for MCH (Figure 1E) and MCV (Figure 1F) values.
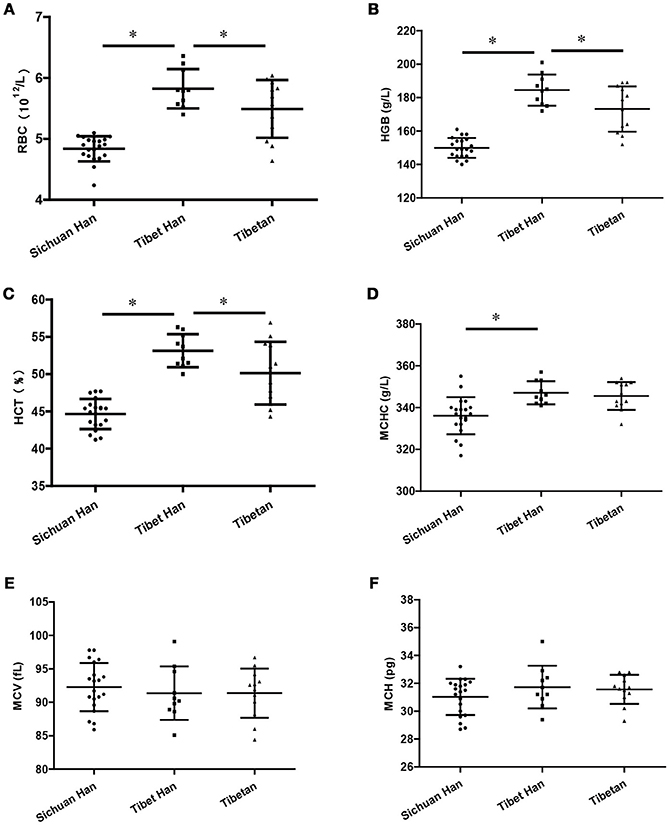
Figure 1. Hematological features of the Sichuan Han, Tibet Han and Tibetan groups. RBC, red blood cell counts (A); HGB, hemoglobin (B); HCT, hematocrit (C); MCHC, mean corpuscular hemoglobin concentration (D); MCV, mean corpuscular volume (E); MCH, mean corpuscular hemoglobin (F) (*p-value < 0.05; error bars indicate the SD).
miRNA Sequencing Analysis of RBC miRNA Profiles
The RBC samples were separately pooled to produce three sample pools of the Sichuan Han, Tibet Han and Tibetan populations. We globally analyzed RBC miRNA expression profiles from the three RBC samples using miRNA sequencing. miRNAs with more than 1 read in the sequencing data were considered expressed miRNAs and we detected 516 miRNAs in the purified RBC samples (Supplementary Table 1), of which 364 overlapped among the three RBC groups. These miRNAs are shown in a Venn diagram (Figure 2A). Of the 516 miRNAs tested, 49 RBC miRNAs were differentially expressed (17 upregulated and 32 downregulated miRNAs) in the Tibet Han group compared with the Sichuan Han group (fold change > 2 or < 0.5) (Figure 2B), 33 RBC miRNAs were differentially expressed (12 upregulated and 21 downregulated miRNAs) in the Tibetan group compared with the Sichuan Han group (fold change > 2 or < 0.5) (Figure 2C), and 40 RBC miRNAs were differentially expressed (15 upregulated and 25 downregulated miRNAs) in the Tibetan group compared with the Tibet Han group (fold change > 2 or < 0.5) (Figure 2D). It is worth mentioning that among the differentially expressed miRNAs between the Tibet Han group and the Sichuan Han group, the downregulated miRNAs still exhibited considerably higher expression levels than the upregulated ones did. miR-144-5p, miR-30b-5p, miR-423-5p, miR-16-2-3p, miR-3200-5p, miR-4732-5p, miR-200c-3p, miR-125a-5p, miR-195-5p, and miR-141-3p were the top ten miRNAs with the highest levels of expression, and their fold changes were less than 0.5 between the Tibet Han group and the Sichuan Han group (Fisher's exact test, p < 0.001) (Table 1). The miRNA sequencing results demonstrated that in addition to markedly different RBC miRNA patterns in the Tibet Han group and the Tibetan group compared to the Sichuan Han group, significant differences in RBC miRNA profiles were also observed between the Tibetan Han group and the Tibetan group.
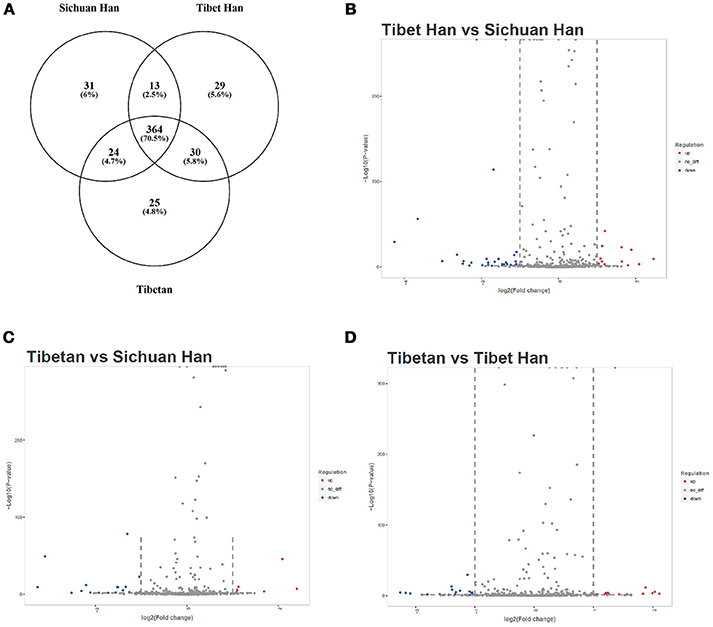
Figure 2. miRNA sequencing analysis of RBC miRNA profiles. miRNAs with more than 1 read in the sequencing data were considered expressed miRNAs. A Venn diagram (A) displays the overlapping miRNAs among the Sichuan Han group, Tibet Han group and Tibetan group. RBC miRNAs were compared between the Tibet Han group and the Sichuan Han group (B), between the Tibetan group and the Sichuan Han group (C), and between the Tibetan group and the Tibet Han group (D). The volcano plot displays the relationship between fold change and significance using a scatter plot view. The red dots in the plot represent significantly upregulated miRNAs, and the blue dots indicate significantly downregulated miRNAs.
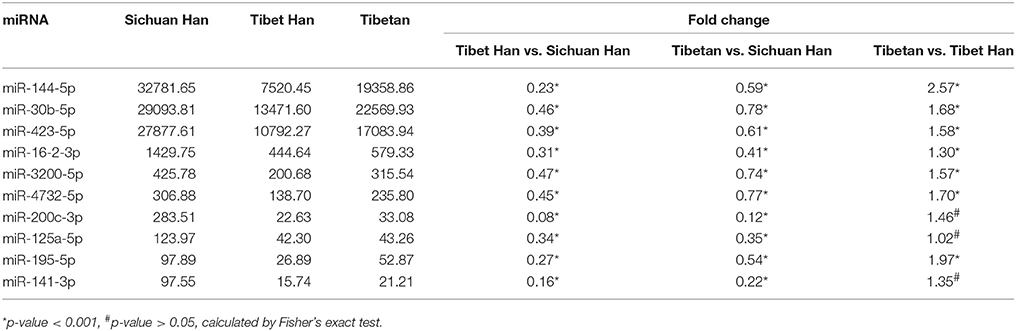
Table 1. Markedly altered miRNAs in pooled RBC samples from the Tibet Han group compared with the Sichuan group as determined by miRNA sequencing.
Verification of miRNA Sequencing Results by Real-Time PCR
Next, to verify the miRNA sequencing results, we performed real-time PCR of miRNAs in individual RBC samples. We selected two miRNAs with the top two highest expression levels and considerable variation (miR-144-5p and miR-30b-5p) for real-time PCR validation of 5 randomly selected RBC samples from each group. U6 was used as the negative control. The variation trends in miR-144-5p (Figure 3A) and miR-30b-5p (Figure 3B) are consistent with the miRNA sequencing results.
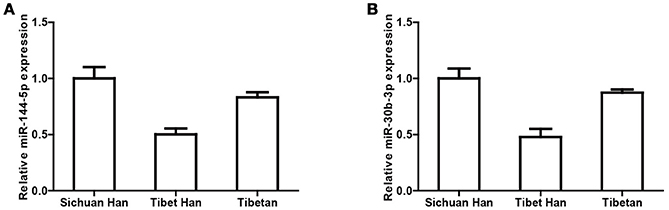
Figure 3. Verification of miRNA sequencing results by real-time PCR. Cq values of miR-144-5p (A) and miR-30b-5p (B) were converted to relative concentrations normalized to U6 values and were calculated using the comparative Cq method.
Target Analysis of miRNAs Altered by High-Altitude Hypoxia
To explore the possible roles and molecular basis of the miRNAs that were altered in response to high-altitude hypoxia, we performed a bioinformatic prediction of potential target genes of miR-144-5p and miR-30b-5p using two computational target prediction algorithms (TargetScan 50 and miRanda 3.3a). Based on GO terms and KEGG pathway analyses, the targets of miR-144-5p were found to be significantly associated with “ATPase activity” and “Calcium signaling pathway” (Figures 4A,B). Functional analysis of the potential targets of miR-30b-5p revealed a number of genes involved in “protein binding” and “regulation of actin skeleton” (Figures 4C,D). These results indicate that miR-144-5p and miR-30b-5p are involved in the regulation of cell energy and cell morphology. Further analysis demonstrated that the potential targets of both miRNAs are predicted to be associated with “hypoxia,” “erythroid,” and “NO”-related pathways (Table 2), Supplementary Table 2 exhibited a legend explaining the respective abbreviations of the target genes. Our findings suggest that miR-144-5p and miR-30b-5p may participate in adaptation to high-altitude hypoxic environments in humans.
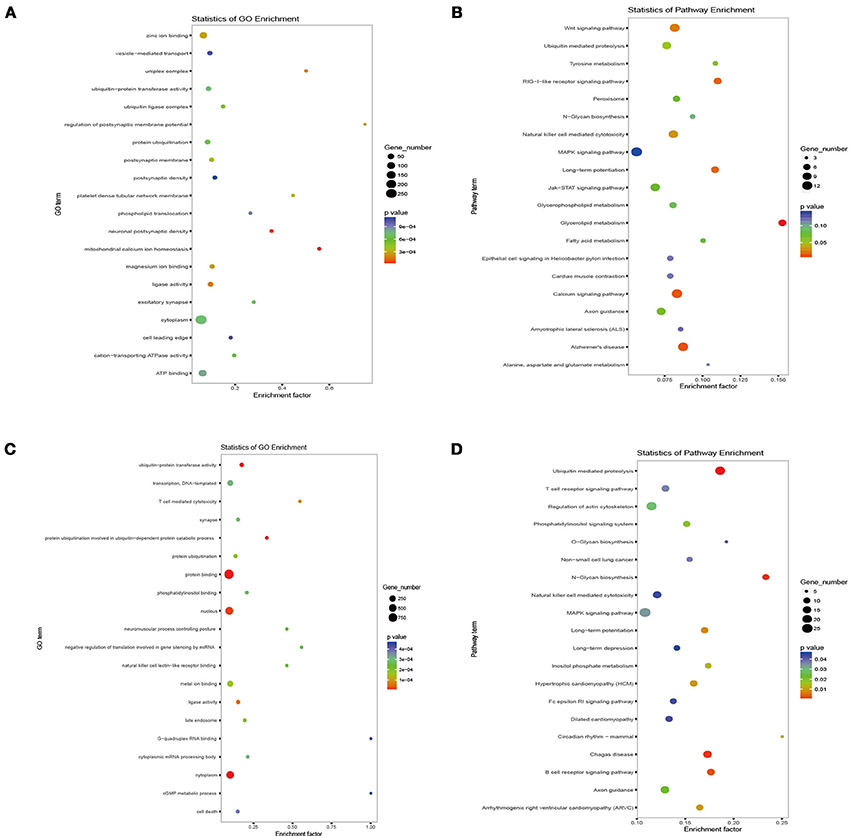
Figure 4. Target analysis of miRNAs altered by high-altitude hypoxia. The biological function prediction of miR-144-5p was performed by GO (A) and KEGG analysis (B). The potential targets of miR-30b-5p by GO and KEGG analysis are shown in (C,D).
Discussion
miRNAs are important epigenetic regulators of diverse cellular processes, and their dysregulation has been demonstrated in a range of bodily disorders or diseases (Lu et al., 2005; Ikeda et al., 2007). Recent studies have reported the effect of high-altitude hypoxia on human circulating miRNA profiles (Yan et al., 2015, 2016; Wang et al., 2017). It is known that, RBC counts in Han migrants increase markedly in response to the high-altitude hypoxic environment (Simonson et al., 2010), and abundant miRNAs can be found in mature RBCs (Chen et al., 2008; Kannan and Atreya, 2010; Wu et al., 2017). However, the effect of high-altitude hypoxia on RBC-derived miRNAs is still unclear. Here, we demonstrate that the RBC miRNA profiles of Han Chinese who migrated to highland Tibet over 10 years ago are dramatically different from those of Tibetans and those residing in low-altitude areas. These data suggest that human RBC miRNA patterns are significantly influenced by a high-altitude hypoxic environment.
In this study, only male samples were chosen for our analysis to reduce the number of confounding factors because Wu et al. reported that gender affects hemoglobin concentrations in Tibetans and Han Chinese (Wu et al., 2005). Several hematological indices were assessed, and RBC, HGB and HCT levels were notably higher in the Tibet Han group than in the Han group and the Tibetan group, which was consistent with previous reports (León-Velarde et al., 2000; Wu et al., 2005; Yan et al., 2015). A reasonable rise in RBC number and HGB levels is a beneficial adaptive response to high-altitude hypoxia, but their pathological increase can lead to elevated blood viscosity and aggravated tissue cell anoxia and result in high-altitude polycythemia accompanied by multiple organ dysfunction (Villafuerte and Corante, 2016). Therefore, the regulatory mechanisms of erythropoiesis in high-altitude environments need to be investigated thoroughly. Sufficient evidence proves that multiple miRNAs play key roles in the process of erythropoiesis (Felli et al., 2005; Sun et al., 2015; Li et al., 2017); thus, we sought to determine whether abundant RBC-derived miRNAs are involved in the regulation of erythropoiesis via transport pathways in high-altitude environments.
High-throughput miRNA sequencing was conducted, and the results showed that the expression profiles of RBC miRNAs of Tibet Han individuals were dramatically different from those of Sichuan Han individuals residing in the low-altitude area, with 49 differentially expressed miRNAs. Among them, miR-144-5p and miR-30b-5p, two miRNAs with the highest expression levels, were confirmed by real-time PCR in individual RBC samples. The consistent results of miRNA sequencing and real-time PCR indicated that RBC miR-144-5p and miR-30b-5p expression levels were significantly decreased in the Tibet Han group compared with the Sichuan Han group. Interestingly, the levels of both miRNAs in the Tibetan highlanders were also significantly lower than in the Han lowlanders, but higher than in the Tibet Han individuals. The expression trend of both miRNAs was negatively consistent with the variation trend of RBC and HGB levels, providing indirect evidence for the relationship between RBC miRNAs and erythropoiesis. In view of the stability of miRNA and its abundance in RBCs, further research on RBC-derived miRNAs may offer a new direction in the search for biomarkers of predicting adaptation to hypoxia. Subsequently, one question arises: Do erythrocyte-derived miRNAs function in high-altitude hypoxia, and if so, how?
To reveal the possible function and mechanism of RBC miRNAs, analysis of potential targets of miR-144-5p and miR-30b-5p was performed. GO and KEGG analyses indicated that the targets of both miRNAs are significantly associated with the “Calcium signaling pathway” and “Regulation of actin skeleton,” possibly indirectly affecting the formation and stability of RBCs. In addition, the potential target gene ERG of miR-30b-5p can regulate erythrocyte differentiation as an essential regulator of hematopoietic stem cell (HSC) function (Carmichael et al., 2012). Many studies indicate that miR-144 plays a crucial role in the process of erythropoiesis as an erythroid-specific regulator (Fu et al., 2009; Papapetrou et al., 2010; Rasmussen et al., 2010; Zhang et al., 2012; Undi et al., 2013). More encouragingly, biological experiments showed that miR-144 can increase the RBC number by inhibiting RAB14 transcription (Kim et al., 2015). Therefore, it is possible that RBC-derived miR-144-5p influences the process of erythropoiesis by targeting RAB14.
Many studies (Zhou et al., 2014; Thomou et al., 2017; Ying et al., 2017) confirm that extracellular miRNAs in microvesicles or exosomes may function in cell-cell communication. A similar transport mechanism of RBC miRNAs has been reported in Plasmodium infection research (Regev-Rudzki et al., 2013; Mantel et al., 2016; Wang et al., 2017). For example, Mantel et al. revealed that infected RBC-derived extracellular vesicles carrying the Ago2-miR-451 complex are taken up by endothelial cells and can alter vascular function in malaria. Wang et al. demonstrated that Ago2-miR-451 and miR-140 complexes in RBCs are abundantly released into parasites within infected RBCs, targeting the mRNAs of P. falciparum erythrocyte membrane protein-1 (pfEMP1, a critical parasite antigen). In addition, Kumar et al. demonstrated that bone marrow HSCs can take up exosomes derived from acute myeloid leukemia, and normal hematopoiesis is suppressed (Kumar et al., 2018). Papapetrou et al. observed that the expression level of miR-144 increases during erythropoiesis in HSCs and miR-144 can positively regulate erythrocyte differentiation (Papapetrou et al., 2010). Therefore, we speculated that miR-144-5p derived from erythrocyte vesicles may be taken up by the HSCs and inhibit the expression levels of target genes (e.g., RAB14), resulting in promotion of erythropoiesis.
The selective mechanism of miRNA loading into exosomes can be modulated by pathophysiological changes (Skog et al., 2008; Guduric-Fuchs et al., 2012; Bhome et al., 2018). Therefore, we infer that the amounts of miR-144-5p released by microvesicles might be affected by hypoxia and other factors; that is, the RBC vesicles released by the Tibet Han population may carry higher amounts of miR-144-5p to HSCs to promote erythropoiesis than the Sichuan Han population, resulting in significantly reduced miR-144-5p expression levels in RBCs, correspondingly, the RBCs of the Tibetan group release lower amounts of miR-144-5p than the Tibet Han group, which may partly contribute to a lower level of RBC production and prevent excessive RBC increase in Tibetans. However, further testing of this hypothesis should be conducted, to obtain more direct evidence supporting the physiological role of erythrocyte-derived miRNAs in hypoxia.
Furthermore, the expression level of PPAR (Peroxisome Proliferator Activated Receptor) family, as a potential target gene of miR-144-5p, is repressed under hypoxia (Slot et al., 2014) and negatively correlated with HGB concentration (Simonson et al., 2010), implying that miR-144-5p has the potential to regulate a high-altitude adaptation. In addition, potential target genes of miR-144-5p and miR-30b-5p, such as FOXO1 and MAPK9, are also associated with the NO synthesis-related signaling pathway, which can affect blood flow and the oxygen carrying capacity of individuals in response to hypoxia. Nonetheless, the targets are less specific for NO, meaning this may not be the main function of both miRNAs.
Conclusions
Collectively, we investigated for the first time that a high-altitude hypoxic environment has marked effects on human RBC miRNA expression profiles. By bioinformatic analyses, some potential target genes of affected miRNAs (miR-144-5p and miR-30b-5p) exhibited enrichment in the erythroid-, hypoxia- and NO- related signaling pathways in response to hypoxia. Further investigation of more individuals, including females and individuals of other ages, should be performed to confirm our results. Moreover, further studies are needed to clarify the potential of RBC miRNAs as biomarkers for predicting high-altitude hypoxic adaptation and the possible physiological role of miR-144-5p, miR-30b-5p and other RBC-derived miRNAs, the levels of which are altered under high-altitude hypoxic conditions.
Author Contributions
DW and CL conceived and designed the experiments. LS performed the experiments. FF contributed to sample and physical data collections. RL and BN analyzed part of the data. LZ, SY, and SW assisted in part of the experiments. LS wrote the manuscript.
Funding
This work was supported by Beijing Natural Science Foundation (7184246), Military Major Special Project (AWS11J007-08), and National Natural Science Foundation of China (81572059).
Conflict of Interest Statement
The authors declare that the research was conducted in the absence of any commercial or financial relationships that could be construed as a potential conflict of interest.
Acknowledgments
The authors thank Dr. Shufang Wang for the excellent suggestions.
Supplementary Material
The Supplementary Material for this article can be found online at: https://www.frontiersin.org/articles/10.3389/fphys.2018.01099/full#supplementary-material
Supplementary Table 1. Differentially expressed miRNAs in purified RBC samples from the Sichuan Han, Tibet Han and Tibetan groups determined by high-throughput miRNA sequencing.
Supplementary Table 2. Explanation for the abbreviation of the target genes showed in Table 2.
References
Bhome, R., Del, V. F., Lee, G. H., Bullock, M. D., Primrose, J. N., Sayan, A. E., et al. (2018). Exosomal micrornas (exomirs): small molecules with a big role in cancer. Cancer Lett. 420, 228–235. doi: 10.1016/j.canlet.2018.02.002
Carmichael, C. L., Metcalf, D., Henley, K. J., Kruse, E. A., Di Rago, L., Mifsud, S., et al. (2012). Hematopoietic overexpression of the transcription factor Erg induces lymphoid and erythro-megakaryocytic leukemia. Proc. Natl. Acad. Sci. U.S.A. 109, 15437–15442. doi: 10.1073/pnas.1213454109
Chen, S. Y., Wang, Y., Telen, M. J., and Chi, J. T. (2008). The genomic analysis of erythrocyte miRNA expression in sickle cell disease. PLoS ONE 3:e2360. doi: 10.1371/journal.pone.0002360
Doss, J. F., Corcoran, D. L., Jima, D. D., Telen, M. J., Dave, S. S., Chi, J. T., et al. (2015). A comprehensive joint analysis of the long and short RNA transcriptomes of human erythrocytes. BMC Genomics 16:952. doi: 10.1186/s12864-015-2156-2
Duan, Z. Y., Cai, G., Bu, R., Lu, Y., Hou, K., and Chen, X. M. (2016). Selection of urinary sediment miRNAs as specific biomarkers of IgA nephropathy. Sci. Rep. 6:23498. doi: 10.1038/srep23498
Duan, Z. Y., Cai, G. Y., Li, J. J., Bu, R., and Chen, X. M. (2017). Urinary erythrocytes-derived miRNAs: emerging role in IgA nephropathy. Kidney Blood Press. Res. 42, 738–748. doi: 10.1159/000481970
Felli, N., Fontana, L., Pelosi, E., Botta, R., Bonci, D., Facchiano, F., et al. (2005). MicroRNAs 221 and 222 inhibit normal erythropoiesis and erythroleukemic cell growth via kit receptor down-modulation. Proc. Natl. Acad. Sci. U.S.A. 102, 18081–18086. doi: 10.1073/pnas.0506216102
Fu, Y. F., Du, T. T., Dong, M., Zhu, K. Y., Jing, C. B., Zhang, Y., et al. (2009). Mir-144 selectively regulates embryonic α-hemoglobin synthesis during primitive erythropoiesis. Blood 113, 1340–1349. doi: 10.1182/blood-2008-08-174854
Guduric-Fuchs, J., O'Connor, A., Camp, B., O'Neill, C. L., Medina, R. J., and Simpson, D. A. (2012). Selective extracellular vesicle-mediated export of an overlapping set of microRNAs from multiple cell types. BMC Genomics 13:357. doi: 10.1186/1471-2164-13-357
Hamilton, A. J. (2010). MicroRNA in erythrocytes. Biochem. Soc. Trans. 38, 229–231. doi: 10.1042/BST0380229
He, B., Wang, J., Qian, G., Hu, M., Qu, X., Wei, Z., et al. (2013). Analysis of high-altitude de-acclimatization syndrome after exposure to high altitudes: a cluster-randomized controlled trial. PLoS ONE 8:e62072. doi: 10.1371/journal.pone.0062072
Huertasánchez, E., Jin, X., Asan., Bianba, Z., Peter, B. M., Vinckenbosch, N., et al. (2014). Altitude adaptation in Tibet caused by introgression of Denisovan-like DNA. Nature 512, 194–197. doi: 10.1038/nature13408
Ikeda, S., Kong, S. W., Lu, J., Bisping, E., Zhang, H., Allen, P. D., et al. (2007). Altered microRNA expression in human heart disease. Physiol. Genomics 31, 367–373. doi: 10.1152/physiolgenomics.00144.2007
Kannan, M., and Atreya, C. (2010). Differential profiling of human red blood cells during storage for 52 selected miRNAs. Transfusion 50, 1581–1588. doi: 10.1111/j.1537-2995.2010.02585.x
Kim, M. J., Tan, Y. S., Cheng, W. C., Kingsbury, T. J., Heimfeld, S., and Civin, C. I. (2015). MIR144 and MIR451 regulate human erythropoiesis via RAB14. Br. J. Haematol. 168, 583–597. doi: 10.1111/bjh.13164
Kumar, B., Garcia, M., Weng, L., Jung, X., Murakami, J. L., Hu, X., et al. (2018). Acute myeloid leukemia transforms the bone marrow niche into a leukemia-permissive microenvironment through exosome secretion. Leukemia 32, 575–587. doi: 10.1038/leu.2017.259
Lamonte, G., Philip, N., Reardon, J., Lacsina, J. R., Majoros, W., Chapman, L., et al. (2012). Translocation of sickle cell erythrocytes micrornas into plasmodium falciparum inhibits parasite translation and contributes to malaria resistance. Cell Host Microbe 12, 187–199. doi: 10.1016/j.chom.2012.06.007
León-Velarde, F., Gamboa, A., Chuquiza, J. A., Esteba, W. A., Rivera-Chira, M., Monge, C. C., et al. (2000). Hematological parameters in high altitude residents living at 4,355, 4,660, and 5,500 meters above sea level. High. Alt. Med. Biol. 1, 97–104. doi: 10.1089/15270290050074233
Li, Y., Zhang, Q., Du, Z., Lu, Z., Liu, S., Zhang, L., et al. (2017). MicroRNA 200a inhibits erythroid differentiation by targeting PDCD4 and THRB. Br. J. Haematol. 176, 50–64. doi: 10.1111/bjh.14377
Liu, B., Huang, H., Wu, G., Xu, G., Sun, B. D., Zhang, E. L., et al. (2017). A signature of circulating micrornas predicts the susceptibility of acute mountain sickness. Front. Physiol. 8:55. doi: 10.3389/fphys.2017.00055
Lu, J., Getz, G., Miska, E. A., Alvarez-Saavedra, E., Lamb, J., Peck, D., et al. (2005). MicroRNA expression profiles classify human cancers. Nature 435, 834–838. doi: 10.1038/nature03702
Mantel, P. Y., Hjelmqvist, D., Walch, M., Kharoubi-Hess, S., Nilsson, S., Ravel, D., et al. (2016). Infected erythrocyte-derived extracellular vesicles alter vascular function via regulatory Ago2-miRNA complexes in malaria. Nat. Commun. 7:12727. doi: 10.1038/ncomms12727
Nallamshetty, S., Chan, S. Y., and Loscalzo, J. (2013). Hypoxia: a master regulator of miRNA biogenesis and activity. Free Radic. Biol. Med. 64, 20–30. doi: 10.1016/j.freeradbiomed.2013.05.022
Papapetrou, E. P., Korkola, J. E., and Sadelain, M. (2010). A genetic strategy for single and combinatorial analysis of miRNA function in mammalian hematopoietic stem cells. Stem Cells 28, 287–296. doi: 10.1002/stem.257
Rasmussen, K. D., Simmini, S., Abreugoodger, C., Bartonicek, N., Di Giacomo, M., Bilbao-Cortes, D., et al. (2010). The miR-144/451 locus is required for erythroid homeostasis. J. Exp. Med. 207, 1351–1358. doi: 10.1084/jem.20100458
Regev-Rudzki, N., Wilson, D. W., Carvalho, T. G., Sisquella, X., Coleman, B. M., Rug, M., et al. (2013). Cell-cell communication between malaria-infected red blood cells via exosome-like vesicles. Cell 153, 1120–1133. doi: 10.1016/j.cell.2013.04.029
Rupaimoole, R., Wu, S. Y., Pradeep, S., Ivan, C., Pecot, C. V., Gharpure, K. M., et al. (2014). Hypoxia mediated down regulation of mirna biogenesis promotes tumor progression. Nat. Commun. 5:5202. doi: 10.1038/ncomms6202
Simonson, T. S., Yang, Y., Huff, C. D., Yun, H., Qin, G., Witherspoon, D. J., et al. (2010). Genetic evidence for high-altitude adaptation in Tibet. Science. 329, 72–75. doi: 10.1126/science.1189406
Skog, J., Würdinger, T., van Rijn, S., Meijer, D. H., Gainche, L., Sena-Esteves, M., et al. (2008). Glioblastoma microvesicles transport RNA and proteins that promote tumour growth and provide diagnostic biomarkers. Nat. Cell. Biol. 10, 1470–1476. doi: 10.1038/ncb1800
Slot, I. G., Schols, A. M., Vosse, B. A., Kelders, M. C., and Gosker, H. R. (2014). Hypoxia differentially regulates muscle oxidative fiber type and metabolism in a HIF-1α-dependent manner. Cell Signal. 26, 1837–1845. doi: 10.1016/j.cellsig.2014.04.016
Sun, Z., Wang, Y., Han, X., Zhao, X., Peng, Y., Li, Y., et al. (2015). miR-150 inhibits terminal erythroid, proliferation and differentiation. Oncotarget 6, 43033–43047. doi: 10.18632/oncotarget.5824
Thomou, T., Mori, M. A., Dreyfuss, J. M., Konishi, M., Sakaguchi, M., Wolfrum, C., et al. (2017). Adipose-derived circulating miRNAs regulate gene expression in other tissues. Nature 542, 450–455. doi: 10.1038/nature21365
Undi, R. B., Kandi, R., and Gutti, R. K. (2013). MicroRNAs as haematopoiesis regulators. Adv. Hematol. 2013:695754. doi: 10.1155/2013/695754
Villafuerte, F. C., and Corante, N. (2016). Chronic mountain sickness: clinical aspects, etiology, management, and treatment. High. Alt. Med. Biol. 17, 61–69. doi: 10.1089/ham.2016.0031
Vu, L., Ragupathy, V., Kulkarni, S., and Atreya, C., (2017). Analysis of Argonaute 2-microRNA complexes in ex vivo stored red blood cells. Transfusion 57, 2995–3000. doi: 10.1111/trf.14325
Walzer, K. A., and Chi, J. T. (2017). Trans-kingdom small RNA transfer during host-pathogen interactions: The case of P. falciparum and erythrocytes. RNA. Biol. 14, 442–449. doi: 10.1080/15476286.2017.1294307
Wang, Z., Xi, J., Hao, X., Deng, W., Liu, J., Wei, C., et al. (2017). Red blood cells release microparticles containing human argonaute 2 and miRNAs to target genes of Plasmodium falciparum. Emerg. Microbes Infect. 6:e75. doi: 10.1038/emi.2017.63
Wu, C. W., Cao, X., Berger, C. K., Foote, P. H., Mahoney, D. W., Simonson, J. A., et al. (2017). Novel approach to fecal occult blood testing by assay of erythrocytes-specific microRNA markers. Dig. Dis. Sci. 62, 1985–1994. doi: 10.1007/s10620-017-4627-6
Wu, T., Wang, X., Wei, C., Cheng, H., Wang, X., Li, Y., et al. (2005). Hemoglobin levels in Qinghai-Tibet: different effects of gender for Tibetans vs. Han. J. Appl. Physiol. 98, 598–604. doi: 10.1152/japplphysiol.01034.2002
Yan, H. L., Xue, G., Mei, Q., Wang, Y. Z., Ding, F. X., Liu, M. F., et al. (2009). Repression of the miR-17-92 cluster by p53 has an important function in hypoxia-induced apoptosis. EMBO J. 28, 2719–2732. doi: 10.1038/emboj.2009.214
Yan, Y., Shi, Y., Wang, C., Guo, P., Wang, J., Zhang, C. Y., et al. (2015). Influence of a high-altitude hypoxic environment on human plasma miRNA profiles. Sci. Rep. 5:15156. doi: 10.1038/srep15156
Yan, Y., Wang, C., Zhou, W., Shi, Y., Guo, P., Liu, Y., et al. (2016). Elevation of circulating miR-210-3p in high-altitude hypoxic environment. Front. Physiol. 7:84. doi: 10.3389/fphys.2016.00084
Yi, X., Liang, Y., Huerta-Sanchez, E., Jin, X., Cuo, Z. X., Pool, J. E., et al. (2010). Sequencing of 50 human exomes reveals adaptation to high altitude. Science 329, 75–78. doi: 10.1126/science.1190371
Ying, W., Riopel, M., Bandyopadhyay, G., Dong, Y., Birmingham, A., Seo, J. B., et al. (2017). adipose tissue macrophage-derived exosomal miRNAs can modulate in vivo and in vitro insulin sensitivity. Cell 171, 372–384. doi: 10.1016/j.cell.2017.08.035
Zhang, L., Sankaran, V. G., and Lodish, H. F. (2012). MicroRNAs in erythroid and megakaryocytic differentiation and megakaryocyte-erythroid progenitor lineage commitment. Leukemia 26, 2310–2316. doi: 10.1038/leu.2012.137
Keywords: high-altitude hypoxia, Tibet, Han Chinese, Tibetan, erythrocyte-derived microRNAs
Citation: Sun L, Fan F, Li R, Niu B, Zhu L, Yu S, Wang S, Li C and Wang D (2018) Different Erythrocyte MicroRNA Profiles in Low- and High-Altitude Individuals. Front. Physiol. 9:1099. doi: 10.3389/fphys.2018.01099
Received: 09 March 2018; Accepted: 23 July 2018;
Published: 14 August 2018.
Edited by:
Wassim El Nemer, Institut National de la Santé et de la Recherche Médicale (INSERM), FranceReviewed by:
Heimo Mairbäurl, Universitätsklinikum Heidelberg, GermanyBalaji Krishnamachary, Johns Hopkins University, United States
Giel Bosman, Radboud University Nijmegen, Netherlands
Copyright © 2018 Sun, Fan, Li, Niu, Zhu, Yu, Wang, Li and Wang. This is an open-access article distributed under the terms of the Creative Commons Attribution License (CC BY). The use, distribution or reproduction in other forums is permitted, provided the original author(s) and the copyright owner(s) are credited and that the original publication in this journal is cited, in accordance with accepted academic practice. No use, distribution or reproduction is permitted which does not comply with these terms.
*Correspondence: Deqing Wang, deqingw@vip.sina.com
Cuiying Li, licuiying2013@qq.com