- 1Laboratory of Developmental and Evolutionary Biology, School of Life Sciences, Shandong University of Technology, Zibo, China
- 2College of Biological and Agricultural Engineering, Weifang Bioengineering Technology Research Center, Weifang University, Weifang, China
Vitamin C (VC) is an essential nutrient for most fish species because of the absence of L-gulonolactone oxidase in the bodies of fish. VC plays a significant role in maintaining the physiological functions and in improving the growth performance, immunity, and survival of fish. In this study, zebrafish (Danio rerio) were treated with 8.2, 509.6, and 1007.5 mg/kg VC diets for 2 weeks, and the muscle samples were collected for gene expression analysis and biochemical index analysis. The results indicated that 509.6 and 1007.5 mg/kg VC diets inhibited glycogen synthase kinase-3β (GSK-3β) expression and induced the expression of β-catenin in the muscle of zebrafish. The mRNA expression of CCAAT/enhancer-binding protein α (C/EBPα) and fatty acid synthase (FAS), FAS activity, and the content of glycerol and triglyceride (TG) were decreased in the muscle by 509.6 and 1007.5 mg/kg VC diets. In addition, GSK-3β RNA interference was observed in zebrafish fed with 8.2 and 1007.5 mg/kg VC diets. It was found that GSK-3β RNA interference induced the mRNA expression of β-catenin but decreased the mRNA expression of C/EBPα and FAS, FAS activity, as well as the content of glycerol and TG in the muscle of zebrafish. In ZF4 cells, the mRNA expression of GSK-3β, C/EBPα, and FAS was decreased, but β-catenin expression was increased by 0.1 and 0.5 mmol/L VC treatments in vitro. The glycerol and TG content, and FAS activity in ZF4 cells were decreased by 0.1 and 0.5 mmol/L VC treatments. Moreover, the result of western blot indicated that the protein expression level of GSK-3β was significantly decreased and that of β-catenin was significantly increased in ZF4 cells treated with 0.1 and 0.5 mmol/L VC. The results from in vivo and in vitro studies corroborated that VC exerted the lipid-lowering effect through GSK-3β/β-catenin signaling in zebrafish.
Introduction
Vitamin (VC) is an essential nutrient for most fish species because of the absence of L-gulonolactone oxidase in the fish body (Fracalossi et al., 2001). In the previous studies, it was found that the minimum requirement of VC was 10∼25 mg/kg diet for rainbow trout (Oncorhynchus mykiss) (Cho and Cowey, 1993), channel catfish (Ictalurus punctatus) (Mustin and Lovell, 1992), hybrid tilapia (Oreochromis niloticus ×O. aureus) (Shiau and Hsu, 1999), and hybrid striped bass (Morone chrysops ×M. saxatilis) (Sealey and Gatlin, 1999). For large yellow croaker (Pseudosciaena crocea), the VC requirement was 28.2 mg/kg diet based on the survival, whereas it was 87.0 mg/kg diet based on the VC content in liver (Ai et al., 2006). Dietary VC requirement of juvenile largemouth bass (Micropterus salmoides) based on growth was 102.6∼109.5 mg/kg (Chen et al., 2015), and it was 410.8 mg/kg diet based on the VC content in liver for Japanese eel (Anguilla japonica) (Shahkar et al., 2015).
As an essential nutrient for fish species, VC plays a significant role in maintaining the normal physiological functions (Khan et al., 2017). It has been observed that VC decreases the oxidative damage from lipid peroxidation and improves the growth performance, immunity, and survival of fish (Madhuban and Kaviraj, 2009; Norouzitallab et al., 2009; Gao et al., 2013; Wan et al., 2014; Barros et al., 2014). In mammals, the dietary VC is negatively associated with the occurrence of obesity and regulates adipocyte lipolysis, hyperglycemia, and glycosylation (Garcia-diaz et al., 2014; Christie-David et al., 2015; Ellulu et al., 2015; Park et al., 2015; Wilson et al., 2017). VC improves the plasma lipid profile, inhibits the lipid oxidation of high-density lipoprotein cholesterol (HDL-C), and prevents atherogenic modification of low-density lipoprotein cholesterol (LDL-C) (Karakilcik et al., 2014; Ashor et al., 2016). Since VC decreases the total serum cholesterol, LDL-C, and very-low-density lipoprotein (VLDL) and reduces the risk of coronary heart disease, it has been used to treat atherosclerosis by controlling cholesterol levels (Ashor et al., 2014; Zhang et al., 2014; Kobylecki et al., 2015; Polidori et al., 2015).
In the canonical Wnt signaling pathway, without Wnt ligands, β-catenin is continuously ubiquitinylated for degradation through glycogen synthase kinase-3β (GSK-3β). Upon Wnt ligand binding to receptors at membrane, a cascade of events impedes GSK-3β activity (Taelman et al., 2010; Arrázola et al., 2015). The ensuing accumulation and nuclear translocation of β-catenin leads to the transcription of a genetic program specific for the temporal, spatial, and tissue contexts (Valenta et al., 2012; Acebron and Niehrs, 2016). As a serine/threonine kinase, GSK-3β is involved in regulating numerous signaling pathways. The deregulation of GSK-3β is related to the development of diabetes, neurodegenerative disease, and bipolar disorder (Beurel et al., 2015; Maqbool et al., 2016). GSK-3β plays a significant role in regulating the canonical Wnt/β-catenin signaling pathway (Dema et al., 2016; Jain et al., 2017). Moreover, it has been found that Wnt/β-catenin signaling participates in regulating the cholesterol metabolism and triglyceride (TG) accumulation and affects the hepatic glucose, glycogen, and lipid metabolism (Chen et al., 2014; Kim et al., 2015; Popov et al., 2016; Liu et al., 2016b).
In fish species, muscle is one of the main sites for lipid storage (Sheridan, 1988). Adipocytes are present in the myoseptum of muscle, and lipid storage in the myoseptum is 40% of white muscle lipid content (Zhou et al., 1995, 1996; Nanton et al., 2007). It has been found that VC influenced lipid composition and peroxidation in the hepatopancreas and muscle of common carp (Cyprinus carpio) (Hwang and Lin, 2002). In our previous study, Wnt/β-catenin signaling participated in regulating lipogenesis in juvenile turbot (Scophthalmus maximus) (Liu et al., 2016b). However, the regulatory mechanism of VC on lipid deposition remains largely unknown in fish species. Thus, it is interesting to investigate whether VC affects lipid profile via GSK-3β/β-catenin signaling. In this study, the lipid-lowering effect of VC through GSK-3β/β-catenin signaling was investigated in zebrafish (Danio rerio) by an in vivo and in vitro study.
Materials and Methods
Diets for VC Experiment in vivo
The basal diet was formulated according to Table 1. Then the different contents of L-Ascorbate-2-phosphate (VC, Hangzhou Haijin Biology Technology Co., Ltd.) were added to the basal diets. The content of VC in the diet was measured with high efficiency liquid chromatography, and 8.2, 509.6, and 1007.5 mg/kg VC was detected in three diets, respectively.
Experimental Animals and VC Experiment in vivo
Zebrafish (AB strain) were maintained at a light:dark photoperiod (12-h:12-h) at 28°C in the dechlorinated water in the flow-through tanks. The zebrafish were, on average, ∼3.4 cm in length and 6 months of age. Twelve glass tanks were randomly distributed with 72 male zebrafish. Animals were fed twice daily with a commercial diet (Sanyou Beautification Feed Tech Co., Ltd, China) and were allowed to get acclimated for 15 days. Subsequently, the diet containing each level of VC was randomly assigned to four tanks. Fish were fed to apparent satiation twice daily (08:00 and 18:00).
Two weeks later, fish were anesthetized with 0.1 g/L MS222, and four independent white muscle samples were collected from four tanks, of which each sample was pooled from the dorsum of three fish. Four muscle samples were frozen in liquid nitrogen and stored at -80°C for molecular biology analysis. In addition, the muscle from the other three fish were sampled and pooled for the biochemical analysis. Four samples were homogenized in cold saline with a glass homogenizer and centrifuged at 4000 ×g for 15 min at 4°C, and the supernatants were collected for biochemical analysis. All animal experiments were approved by Shandong University of China’s Institutional Animal Care Committee in accordance with the Guidelines for Proper Conduct of Animal Experiments (Science Council of China).
GSK-3β RNA Interference in vivo
Firstly, the open reading frame (ORF) of GSK-3β gene (NM_131381.1) was cloned with the primers (F1: 5′-CTG GTG AGC AGT AGG GTG-3′; R1: 5′-CGG ATT CGT TCA AGA CAA). According to the method of Arockiaraj et al. (2014), the double-stranded RNA (dsRNA) was synthesized with the primers containing the T7 promoter RNA polymerase recognition sequence at the 5′ end (F2: 5′-GAT CAC TAA TAC GAC TCA CTA TAG GGC GGC ATT CGG CAG CAT GAA AG-3′; R2: 5′-GAT CAC TAA TAC GAC TCA CTA TAG GGG CAC GGC TGT GTC TGG GTC CA-3′). The reactions of in vitro transcription were performed in water at 37°C in a solution containing T7 RNA polymerase (Takara), 100 mM DTT, RNase Inhibitor, 5 × transcription buffer, 2.5 mM rNTP, and the template. A UV spectrophotometer (Nano View) was used to detect the concentration of dsRNA. Then 72 male zebrafish were randomly distributed into 12 glass tanks, which included four groups and each group included three tanks: group 1 (DEPC water + 8.2 mg/kg VC), group 2 (RNAi + 8.2 mg/kg VC), group 3 (DEPC water + 1007.5 mg/kg VC), and group 4 (RNAi + 1007.5 mg/kg VC). For the groups of GSK-3β RNA interference (group 2 and group 4), each fish received intraperitoneal injection of 25 μL dsRNA (8ng/μL). For the control groups (group 1 and group 3), each fish was injected with 25 μL DEPC water. The diet containing 8.2 mg/kg VC was fed to the fish in group 1 and group 2, and the diet containing 1007.5 mg/kg VC was fed to fish in group 3 and group 4, respectively. Seven days later, fish were sampled for VC treatment in vivo.
ZF4 Cell Culture and VC Treatment in vitro
ZF4 cells of zebrafish were purchased from China Zebrafish Resource Center (CZRC) (Wuhan, China), and maintained in DMEM:F12 (Gibco) supplemented with 10% fetal bovine serum at 28°C (5% CO2). ZF4 cells were cultured in 0, 0.1, and 0.5 mmol/L VC diluted in the basal culture medium for 48 h. Then cells were collected for gene expression analysis and biochemical analysis. The experiment was repeated three times.
Assay the Content of Glycerol and TG, and the Activity of Fatty Acid Synthase (FAS), and the Protein Concentration
Muscle was homogenized and ZF4 cell samples were treated with 0.2% Triton X-100. The supernatants were collected for biochemical analysis after centrifugation at 4000 ×g for 10 min. According to the method of Liu et al. (2016a,b), the content of glycerol and TG, FAS activity, and the protein concentration were assayed with the glycerol, TG, FAS activity kits purchased from Nanjing Jiancheng Bioengineering Institute (Nanjing, China).
RNA Extraction and Real-Time Quantitative Polymerase Chain Reaction
Total RNA was extracted from muscle or ZF4 cells using Trizol reagent (Invitrogen, United States) and transcribed to cDNA by PrimeScriptTM RT Reagent Kit (Takara, Japan). The primer sequences for GSK-3β, β-catenin, FAS, CCAAT/enhancer-binding protein α (C/EBPα), and reference gene (β-actin) (Teng et al., 2014) were listed in Table 2. A quantitative thermal cycle (ROCHE, Lightcycler96, Switzerland) and SYBR® Premix Ex TaqTM II (Takara, Japan) were used to carry out real-time PCR. The real-time PCR program was set as follows: 50°C for 2 min, 95°C for 10 min, followed by 40 cycles of 95°C for 15 s, and 60°C for 1 min. The amplification efficiency was detected, and the 2-ΔΔCT method was employed to analyze the differences of relative gene expression in each sample by using β-actin as the internal reference gene (Livak and Schmittgen, 2001).
Immunofluorescent Microscopy of ZF4 Cells
ZF4 cells were cultured in basal culture medium containing 0, 0.1, and 0.5 mmol/L VC for 48 h. After the cells were fixed with paraformaldehyde and permeabilized with Triton X-100, cells were incubated with antibodies, anti-GSK-3β (Cat. No. 12456, Cell signaling, 1:200), and anti-β-catenin (Cat. No. 8480, Cell signaling, 1:200), respectively. Then cells were stained with the fluorescein isothiocyanate (FITC) dye secondary antibody (Santa-Cruz, 1:400). The negative controls with only secondary antibody were also performed. Finally, the samples were incubated with 5 μM DRAQ5™ (Cell signaling) nuclear stain, and a laser scanning confocal microscope (Leica TCS SP2, Germany) was used to detect the immunofluorescence.
Western Blot Analysis of ZF4 Cells
ZF4 cells were cultured in basal culture medium containing 0, 0.1, and 0.5 mmol/L VC for 48 h. The ZF4 cell samples were prepared using the method described by Tian et al. (2018). The membranes were probed with primary antibody and the appropriate horseradish peroxidase (HRP)-conjugated secondary antibody. Antibodies directed against β-catenin (Cat. No. 8480, 1:1000), GSK-3β (Cat. No. 12456, 1:1000), Phospho-GSK-3β (Ser9) (Cat. No. 9323, 1:1000), and Phospho-β-Catenin (Ser675) (Cat. No. 4176, 1:1000) were purchased from Cell Signaling Technology Inc. β-actin (sc-1615, 1:10000) was purchased from Santa-Cruz Inc. Densitometry analyses were performed with the Image J software (National Institutes of Health).
Statistical Analysis
Data were expressed as mean values ± standard error of mean (s.e.m). The statistical analyses were conducted using SPSS 16.0 (SPSS Inc, Chicago, IL, United States). The homogeneity of variances among groups and normality were tested, and the differences among samples were compared using one-way analysis of variance (ANOVA) followed by Tukey’s test. In addition, for GSK-3β RNA interference experiment, two-way ANOVA followed by the appropriate post hoc test was carried out to calculate the interaction of the two factors, “RNAi” and “VC content”, on the levels of gene expression and biochemical index. P < 0.05 was considered statistically significant.
Results
Effect of VC on the Levels of Gene Expression in the Muscle
Compared with the control group, the mRNA expression of GSK-3β in the muscle of zebrafish was significantly inhibited by 509.6 and 1007.5 mg/kg VC treatments for 2 weeks (P < 0.05) (Figure 1A). However, the mRNA expression of β-catenin was significantly induced by 1007.5 mg/kg VC treatment (P < 0.05) (Figure 1B). The mRNA expression of β-catenin in 509.6 mg/kg VC group was higher than the control but no significant difference was observed (Figure 1B). In addition, the mRNA expression of C/EBPα and FAS in the muscle of zebrafish was significantly decreased by 509.6 and 1007.5 mg/kg VC treatments (P < 0.05) (Figures 1C,D). No significant difference in the mRNA expression of C/EBPα and FAS was observed between the diets of 509.6 and 1007.5 mg/kg VC (Figures 1C,D).
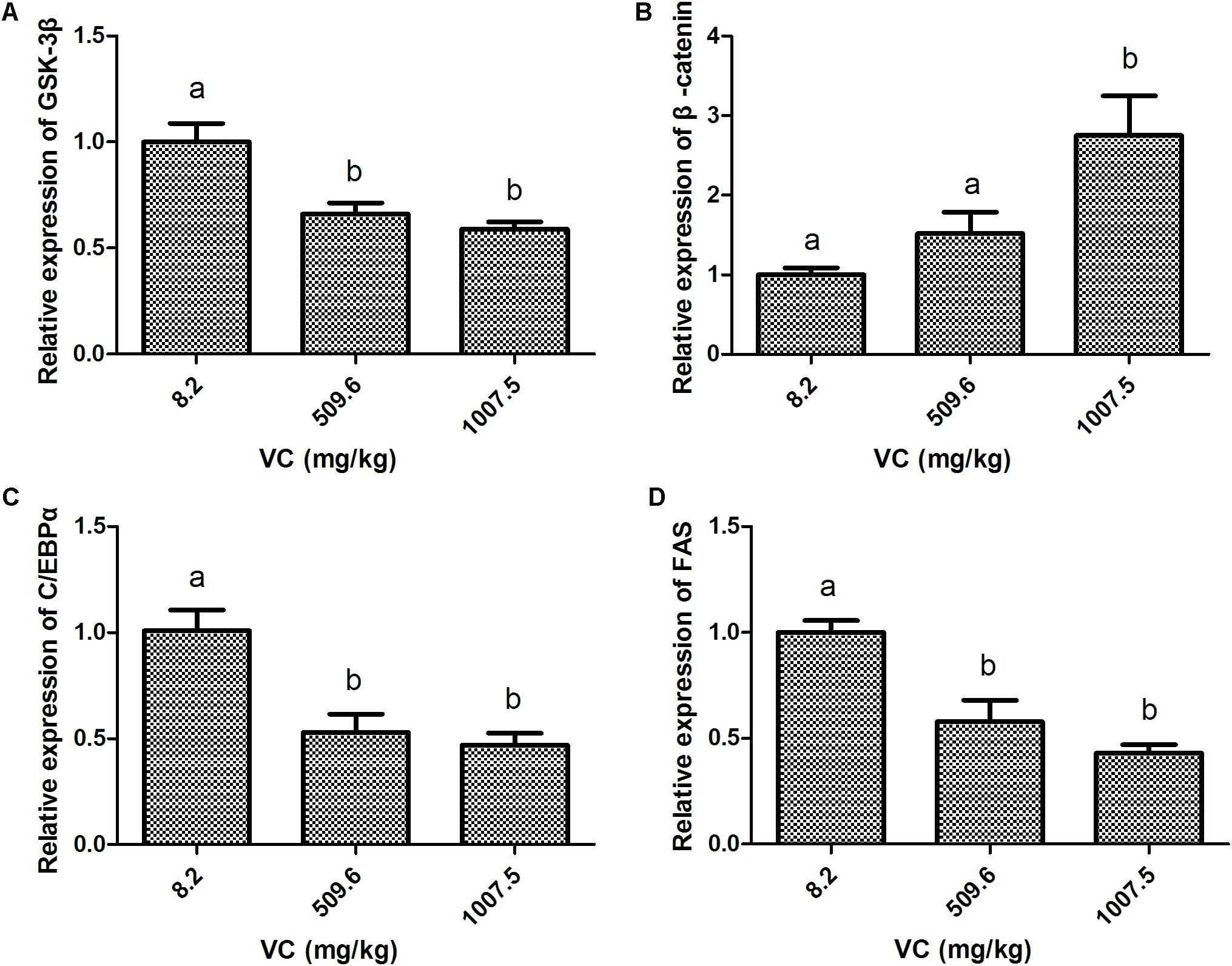
FIGURE 1. Effect of VC on the mRNA expression in the muscle of zebrafish. (A): GSK-3β; (B): β-catenin; (C): C/EBPα; (D): FAS. Values are expressed as means ± s.e.m. (n = 4). Statistically significant differences are denoted by different letters (P < 0.05).
Effect of VC on FAS Activity, the Content of Glycerol and TG in the Muscle
Compared with the control, the content of glycerol and TG in the muscle was significantly decreased by 509.6 and 1007.5 mg/kg VC treatments (P < 0.05) (Figures 2A,B). However, there was no significant difference between 509.6 and 1007.5 mg/kg VC treatments (Figures 2A,B). The activity of FAS in the muscle was significantly decreased by 509.6 and 1007.5 mg/kg VC treatments (P < 0.05), and there was significant difference between these two groups (P < 0.05) (Figure 2C).
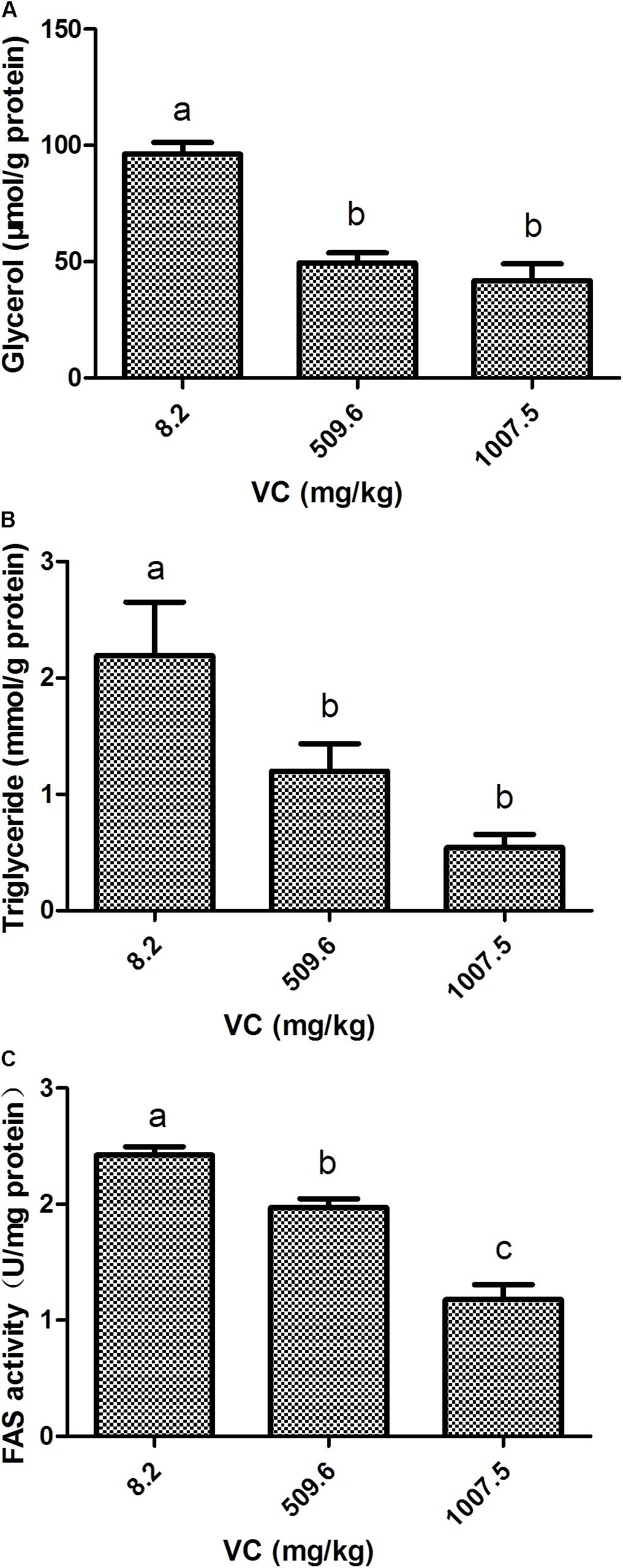
FIGURE 2. Effect of VC on glycerol, TG, and FAS activity in the muscle of zebrafish. (A): glycerol content; (B): TG content; (C): FAS activity. Values are expressed as means ± s.e.m. (n = 4). Statistically significant differences are denoted by different letters (P < 0.05).
Effect of GSK-3β RNA Interference on the Levels of Gene Expression in the Muscle
Compared with the control, the mRNA expression of GSK-3β was significantly decreased by GSK-3β RNA interference in the muscle of zebrafish fed with 8.2 and 1007.5 mg/kg VC diets a week later (P < 0.05) (Figure 3A). However, the mRNA expression of β-catenin was significantly increased by GSK-3β interference in the zebrafish fed with 8.2 and 1007.5 mg/kg VC diets (P < 0.05) (Figure 3B). Furthermore, the mRNA expression of C/EBPα and FAS was significantly decreased by GSK-3β interference fed with 1007.5 mg/kg VC diet (P < 0.05) (Figures 3C,D). Two-way ANOVA revealed that the factor RNAi had significant effect on the expression levels of GSK-3β, β-catenin, C/EBPα, and FAS (P < 0.05) (Supplementary Table 1). However, no significant difference was found on the factor VC content and the interaction factor (RNAi and VC content) (Supplementary Table 1).
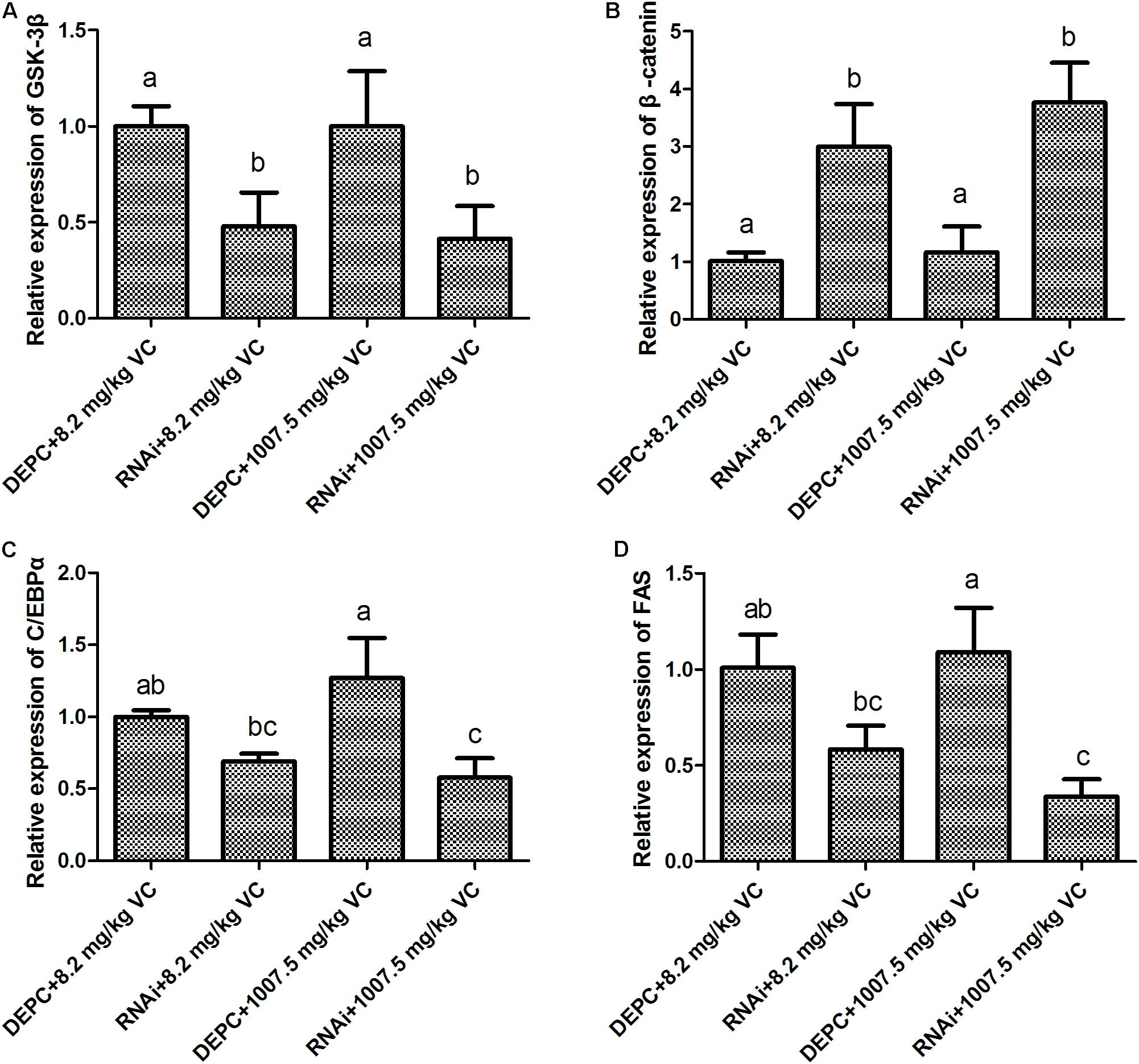
FIGURE 3. Effect of GSK-3β RNA interference on the mRNA expression in the muscle of zebrafish. (A): GSK-3β; (B): β-catenin; (C): C/EBPα; (D): FAS. Values are expressed as means ± s.e.m. (n = 3). Statistically significant differences are denoted by different letters (P < 0.05).
Effect of GSK-3β RNA Interference on FAS Activity and the Content of Glycerol and TG in the Muscle
The content of glycerol and TG was significantly decreased by GSK-3β RNA interference in the muscle of zebrafish fed with 8.2 and 1007.5 mg/kg VC diets (P < 0.05) (Figures 4A,B), and GSK-3β interference significantly affected the activity of FAS in the muscle of zebrafish fed with 8.2 and 1007.5 mg/kg VC diets (P < 0.05) (Figure 4C). Two-way ANOVA analysis indicated that the factor RNAi had significant effect on FAS activity and the content of glycerol and TG (P < 0.05), but no significant difference was found on the interaction factor (RNAi and VC content) (Supplementary Table 2).
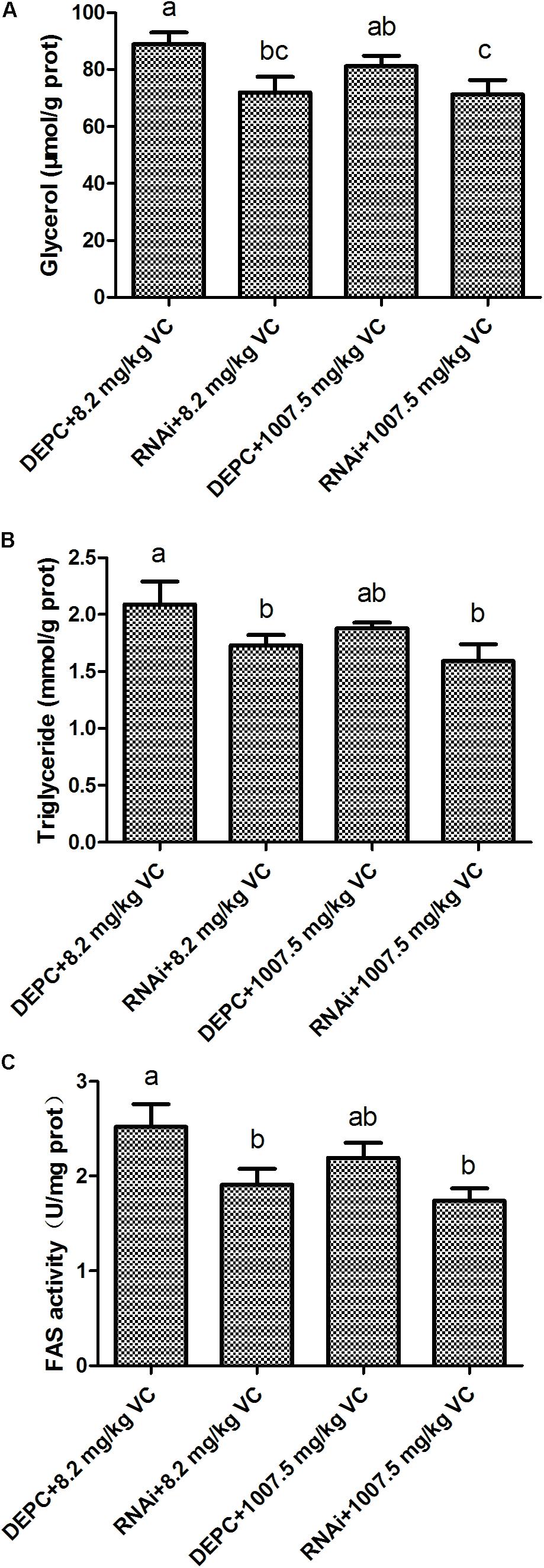
FIGURE 4. Effect of GSK-3β RNA interference on glycerol, TG, and FAS activity in the muscle of zebrafish. (A): glycerol content; (B): TG content; (C): FAS activity. Values are expressed as means ± s.e.m. (n = 3). Statistically significant differences are denoted by different letters (P < 0.05).
Effect of VC on the Levels of Gene Expression in the ZF4 Cells
Compared with the control group, the mRNA expression of GSK-3β was significantly decreased (P < 0.05), whereas β-catenin expression was significantly induced by 0.1 and 0.5 mmol/L VC treatments (P < 0.05) (Figures 5A,B). However, the mRNA expression of C/EBPα and FAS in the ZF4 cells of zebrafish was significantly decreased by 0.1 and 0.5 mmol/L VC treatments (P < 0.05) (Figures 5C,D).
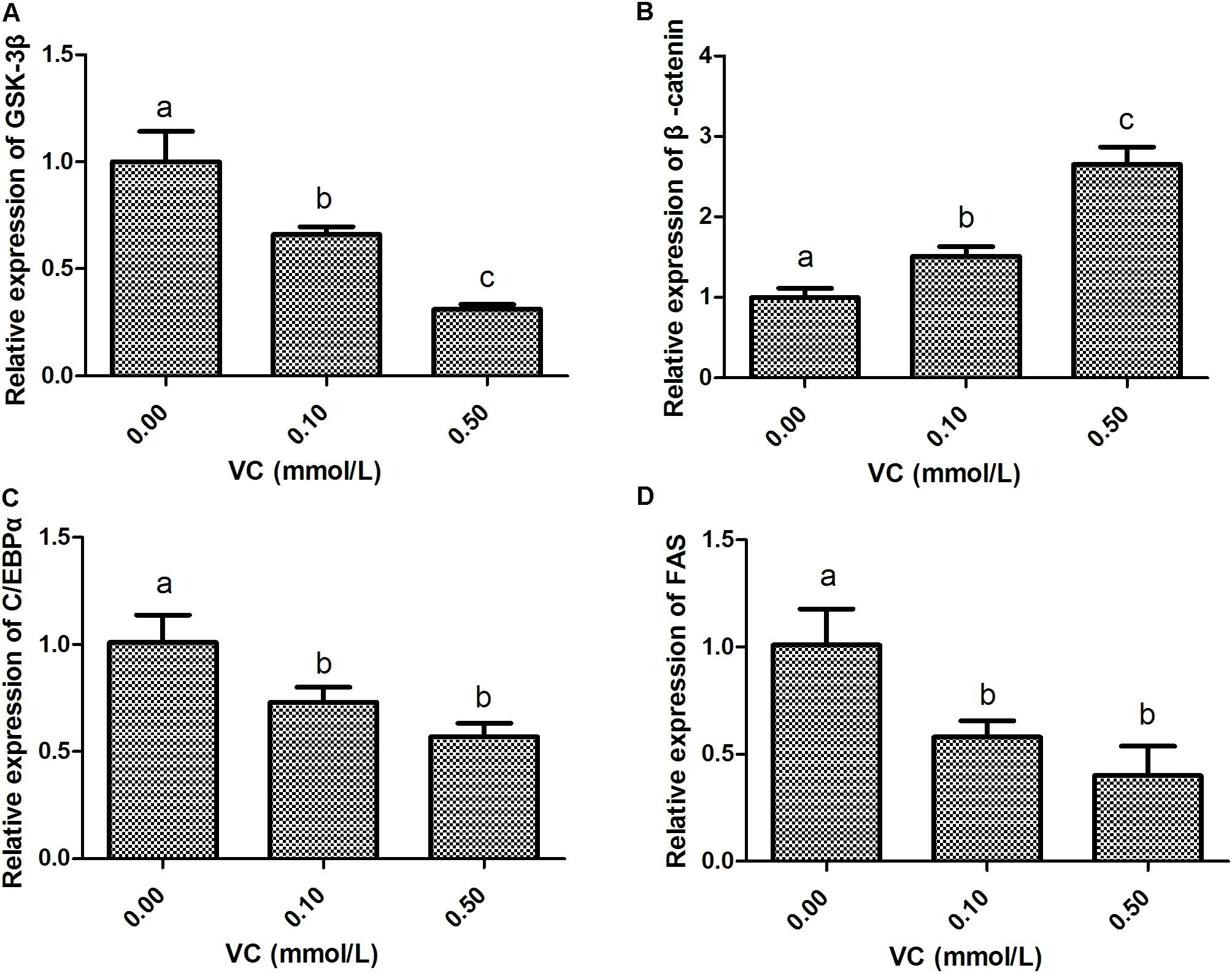
FIGURE 5. Effect of VC on the mRNA expression in ZF4 cells. (A): GSK-3β; (B): β-catenin; (C): C/EBPα; (D): FAS. Values are expressed as means ± s.e.m. (n = 3). Statistically significant differences are denoted by asterisk (P < 0.05).
Effect of VC on FAS Activity and the Content of Glycerol and TG in ZF4 Cells
Compared with the control group, the content of glycerol in ZF4 cells was significantly decreased by 0.5 mmol/L VC treatment (P < 0.05) (Figure 6A). The content of TG and FAS activity in ZF4 cells was significantly decreased by 0.1 and 0.5 mmol/L VC treatments (P < 0.05) (Figures 6B,C).
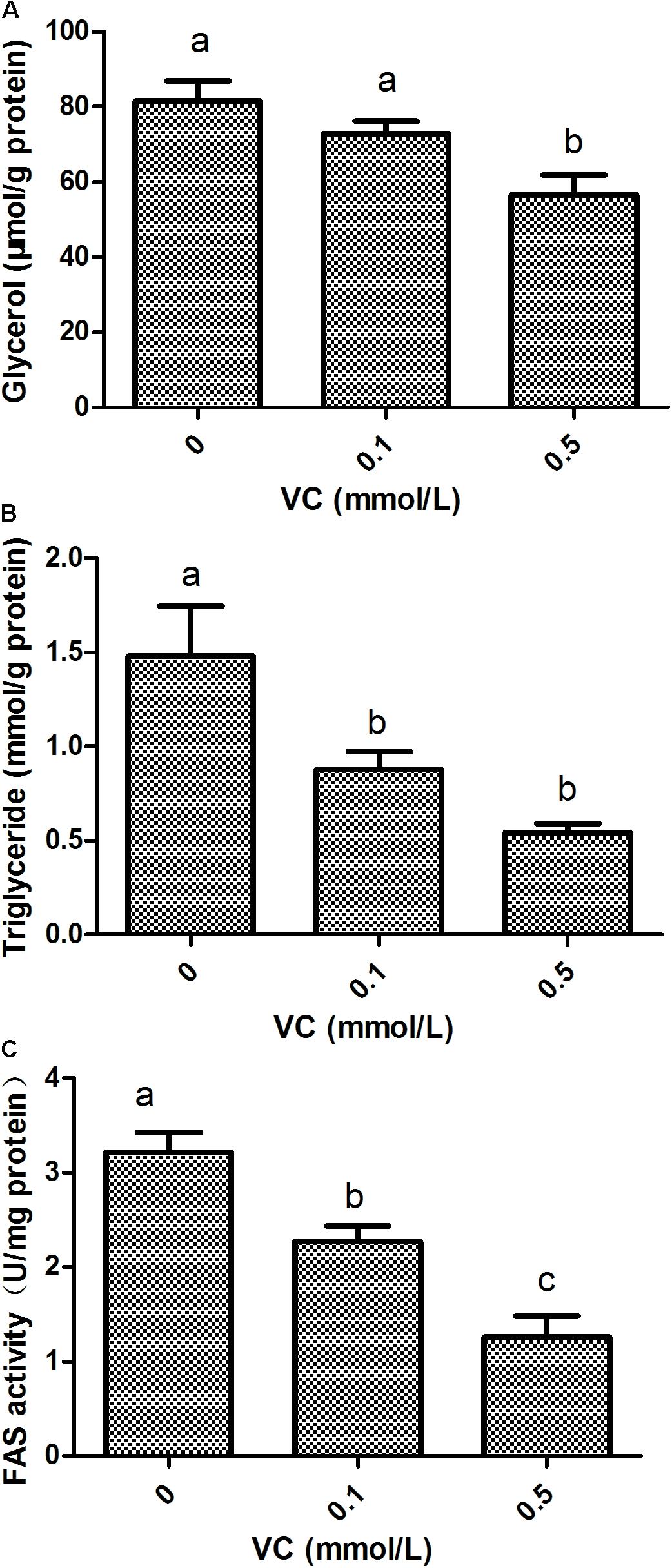
FIGURE 6. Effect of VC on glycerol, TG, and FAS activity in ZF4 cells. (A): glycerol content; (B): TG content; (C): FAS activity. Values are expressed as means ± s.e.m. (n = 3). Statistically significant differences are denoted by different letters (P < 0.05).
Effect of VC on the Protein Expression of GSK-3β and β-Catenin in ZF4 Cells
The immunofluorescent assay was carried out to detect the effect of VC on the protein expression of GSK-3β and β-catenin in ZF4 cells (Figures 7, 8). It was found that the immunofluorescence of β-catenin was increased, whereas the immunofluorescence of GSK-3β was decreased with increasing VC concentrations (Figures 7, 8). The result of western blot indicated that the protein expression level of total GSK-3β was significantly decreased (P < 0.05) and that of total β-catenin was significantly increased in ZF4 cells treated with 0.1 and 0.5 mmol/L VC (P < 0.05) (Figures 9A–C). Moreover, the phosphorylation level of GSK-3β was significantly increased (P < 0.05) and that of β-catenin was significantly decreased in ZF4 cells treated with 0.1 and 0.5 mmol/L VC (P < 0.05) (Figures 9A,D,E).
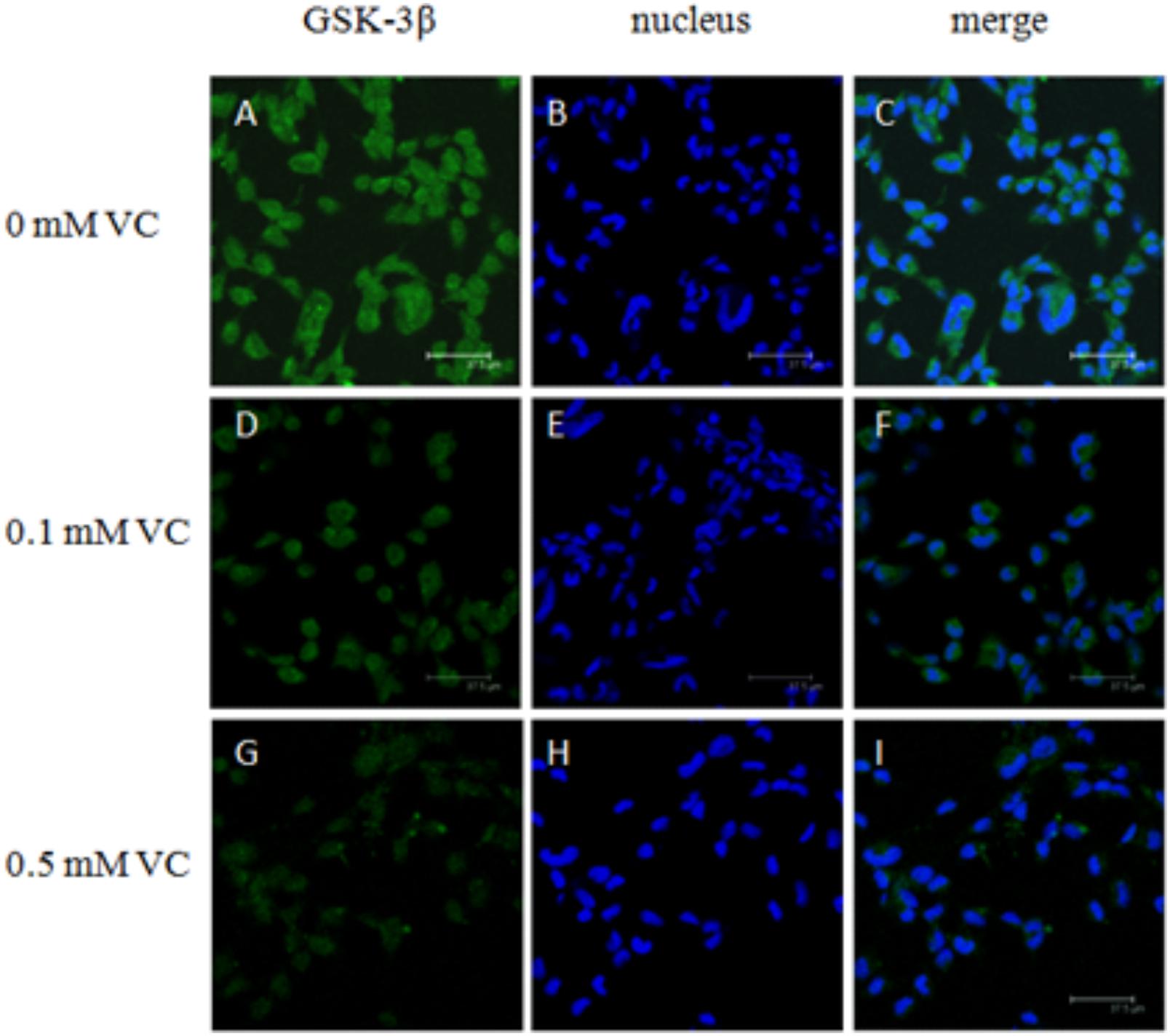
FIGURE 7. Effect of VC on the immunofluorescence of GSK-3β in the ZF4 cells of zebrafish. (A,D,G): GSK-3β, green; (B,E,H): nucleus, blue; (C,F,I): green and blue merger. Bar: 37.5 μm.
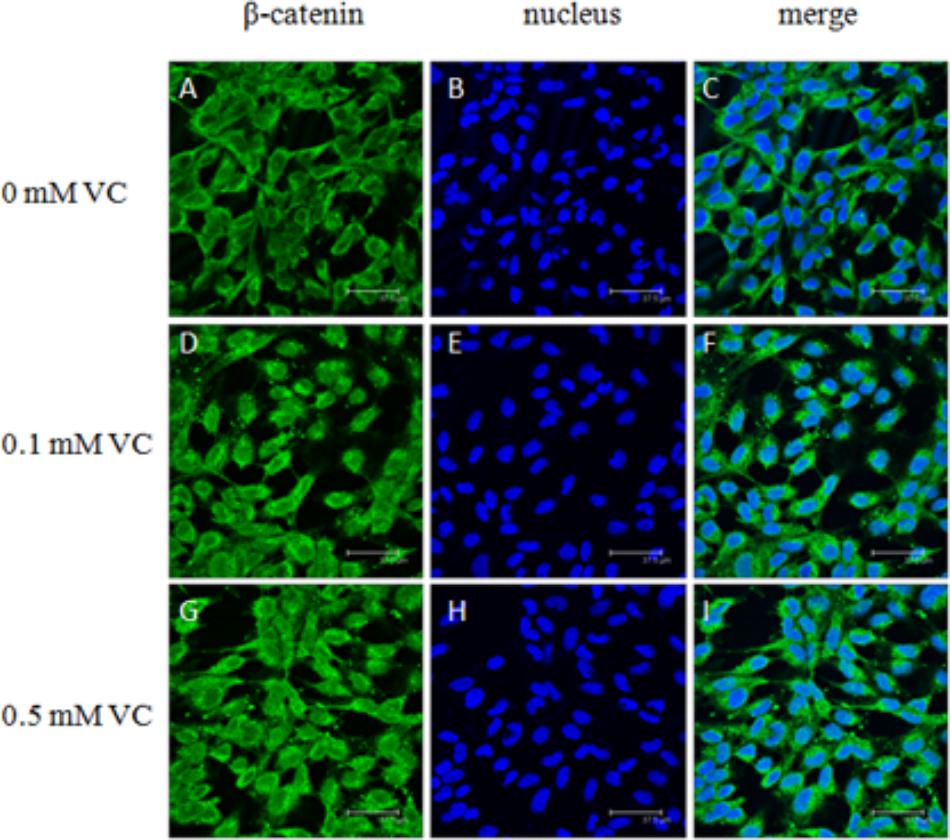
FIGURE 8. Effect of VC on the immunofluorescence of β-catenin in the ZF4 cells of zebrafish. (A,D,G): β-catenin, green; (B,E,H): nucleus, blue; (C,F,I): green and blue merger. Bar: 37.5 μm.
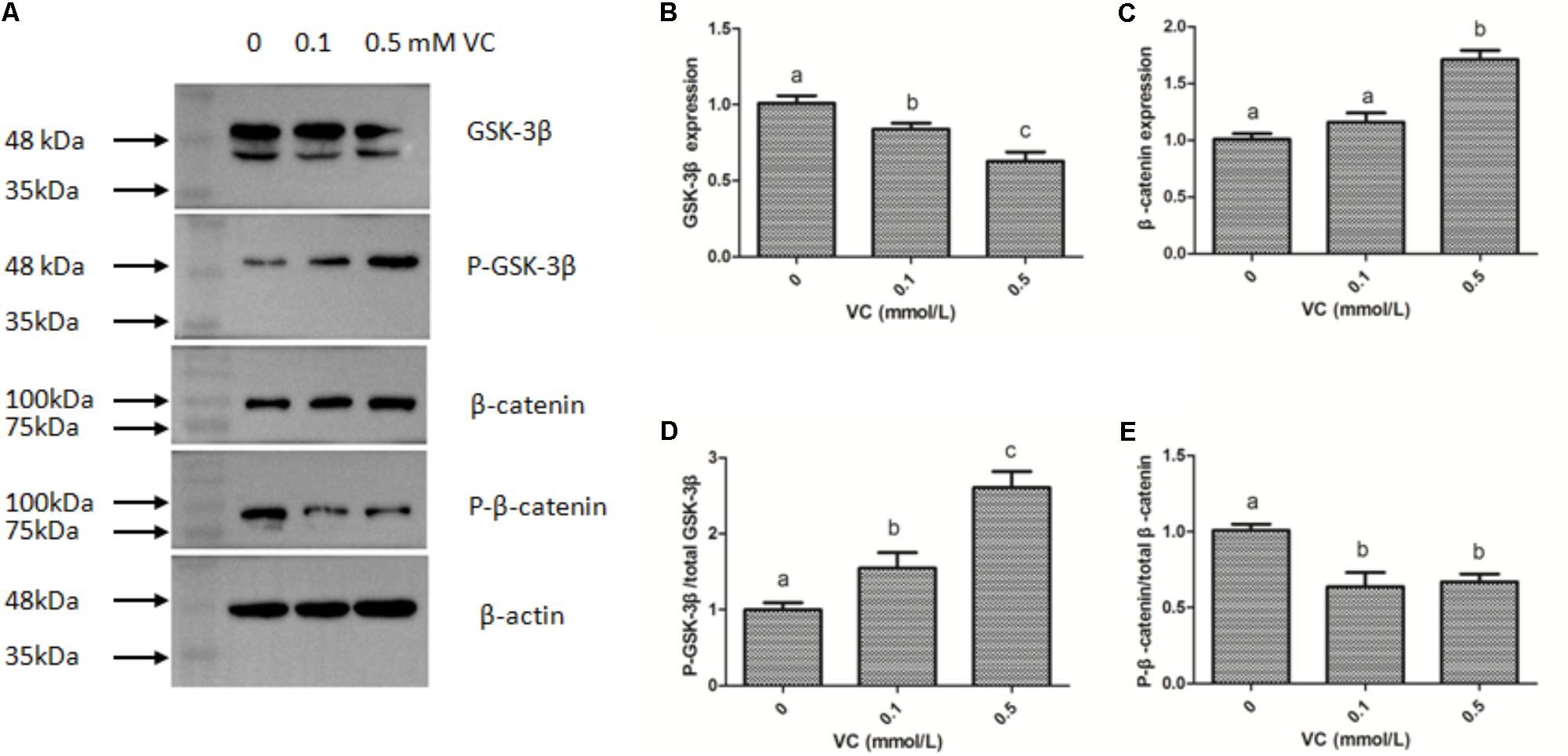
FIGURE 9. Effect of VC on the protein expression in the ZF4 cells of zebrafish. (A) Protein bands of western blot; (B) Effect of VC on GSK-3β; (C) Effect of VC on β-catenin; (D) Effect of VC on the phosphorylation level of GSK-3β; (E) Effect of VC on the phosphorylation level of β-catenin. Values are expressed as means ± s.e.m. (n = 3). Statistically significant differences are denoted by different letters (P < 0.05).
Discussion
GSK-3β plays a key role in regulating the canonical Wnt/β-catenin signaling pathway (Dema et al., 2016; Jain et al., 2017). The accumulation of β-catenin leads to the transcription of a genetic program specific for physiological activities (Valenta et al., 2012; Acebron and Niehrs, 2016). In this study, the mRNA expression of GSK-3β was significantly decreased, but the mRNA expression of β-catenin was significantly induced by VC treatments in the muscle and ZF4 cells. The result of western blot indicated that the expression level of total GSK-3β was significantly decreased and that of total β-catenin was significantly increased in ZF4 cells treated with 0.1 and 0.5 mmol/L VC. Moreover, the phosphorylation level of GSK-3β was significantly increased and that of β-catenin was significantly decreased by VC treatments in ZF4 cells. The phosphorylation of β-catenin could be degraded by ubiquitin protease and led to less β-catenin accumulation in the cytoplasm. The decrease of β-catenin phosphorylation level was beneficial to the accumulation of β-catenin. In addition, GSK-3β activity was involved in regulating the phosphorylation of β-catenin, and GSK-3β activity could be inhibited by the increase of GSK-3β phosphorylation and resulted in more β-catenin accumulation in the cytoplasm. The results of gene and protein expression indicated that VC treatments could induce Wnt/β-catenin signaling.
In a previous study, β-catenin signaling suppressed adipogenesis by inhibiting the expression of C/EBPα (Kim et al., 2014; Xu et al., 2014). C/EBPα inhibited adipogenesis by decreasing the expression of FAS in bone marrow (BM)-derived mesenchymal stromal cells and 3T3-L1 preadipocytes (Jang, 2016; Kim and Nam, 2017). Our study also showed that the mRNA expression of C/EBPα and FAS was significantly decreased by VC treatments in vitro and in vivo. Since β-catenin was activated by VC treatments, β-catenin may further inhibit the expression of C/EBPα and FAS, which was consistent with the previous studies.
It has been found that the dietary VC regulates adipocyte lipolysis, hyperglycemia, and glycosylation in obese diabetic mice (Garcia-diaz et al., 2014; Christie-David et al., 2015; Ellulu et al., 2015; Park et al., 2015; Wilson et al., 2017). Moreover, VC improves the plasma lipid profile (Garcia-diaz et al., 2014; Christie-David et al., 2015; Ellulu et al., 2015; Park et al., 2015; Wilson et al., 2017), and it has been used to treat atherosclerosis by controlling cholesterol levels (Ashor et al., 2014; Zhang et al., 2014; Kobylecki et al., 2015; Polidori et al., 2015). In this study, FAS activity and the content of glycerol and TG in the muscle was significantly decreased by 509.6 and 1007.5 mg/kg VC diets. In ZF4 cells, the content of glycerol was significantly decreased by 0.5 mmol/L VC treatments, and the content of TG and FAS activity was significantly decreased by 0.1 and 0.5 mmol/L VC treatments. Our results indicated that FAS activity and the content of glycerol and TG were related to the concentration of VC. The higher concentration of VC had a better effect on decreasing the content of glycerol and TG.
GSK-3β participated in regulating numerous signaling pathways related to the development of diabetes, neurodegenerative disease, and bipolar disorder (Beurel et al., 2015; Maqbool et al., 2016). Since GSK-3β plays a key role in regulating the canonical Wnt/β-catenin signaling pathway (Dema et al., 2016; Jain et al., 2017), in this study, the gene of GSK-3β was interfered to confirm that GSK-3β/β-catenin was involved in regulating lipid deposition. It was found that the gene expression of β-catenin was increased, whereas the expression of C/EBPα and FAS was decreased by GSK-3β RNA interference. Two-way ANOVA revealed that the factor RNAi had significant effect on FAS activity, glycerol and TG content, as well as the gene expression levels of GSK-3β, β-catenin, C/EBPα, and FAS. However, no significant difference was observed on the factor VC content and the interaction factor in the experiment of GSK-3β RNA interference. For the muscle of zebrafish that was sampled a week after GSK-3β RNA interference, the shorter time with VC treatments may result in no significant difference on the factor VC content. The result of GSK-3β RNA interference indicated that GSK-3β played an important role in lipid-lowering effect by controlling the activity of FAS and the content of glycerol and TG. GSK-3β may be inactivated by VC, which results in β-catenin accumulation, and GSK-3β/β-catenin signaling exerted the lipid-lowering effect by controlling FAS activity and lipid profile in zebrafish.
Conclusion
The treatment of VC induced the expression of β-catenin by inhibiting GSK-3β expression. GSK-3β/β-catenin signaling exerted the lipid-lowering effect by controlling FAS activity and lipid profile in zebrafish (Danio rerio).
Author Contributions
DL contributed to the conception and design of the work. HY, DL, XZ, and QP contributed to data acquisition, analysis, and interpretation. DL, XZ, and QP drafted the work and revised it critically.
Conflict of Interest Statement
The authors declare that the research was conducted in the absence of any commercial or financial relationships that could be construed as a potential conflict of interest.
Acknowledgments
This study was supported by the Natural Science Foundation of Shandong Province (Grant No. ZR2016CM18), Key Science and Technology Project in Institutions of Higher Education of Shandong Province (J17KZ003), Key Technology Innovation Project of Shandong Province (Grant No. 2018CXGC0102), and Key Technology Innovation Project of Guangxi Province (Grant No. AA17204044).
Supplementary Material
The Supplementary Material for this article can be found online at: https://www.frontiersin.org/articles/10.3389/fphys.2018.01023/full#supplementary-material
References
Acebron, S. P., and Niehrs, C. (2016). β-Catenin-independent roles of Wnt/LRP6 signaling. Trends Cell Biol. 26, 956–967. doi: 10.1016/j.tcb.2016.07.009
Ai, Q., Mai, K., Tan, B., Xu, W., Zhang, W., Ma, H., et al. (2006). Effects of dietary vitamin c on survival, growth, and immunity of large yellow croaker, Pseudosciaena crocea. Aquaculture 261, 327–336. doi: 10.1016/j.aquaculture.2006.07.027
Arockiaraj, J., Palanisamy, R., Bhatt, P., Kumaresan, V., Gnanam, A. J., Pasupuleti, M., et al. (2014). A novel murrel Channa striatus mitochondrial manganese superoxide dismutase: gene silencing, SOD activity, superoxide anion production and expression. Fish Physiol. Biochem. 40, 1937–1955. doi: 10.1007/s10695-014-9981-0
Arrázola, M. S., Silva-Alvarez, C., and Inestrosa, N. C. (2015). How the wnt signaling pathway protects from neurodegeneration: the mitochondrial scenario. Front. Cell. Neurosci. 9:166. doi: 10.3389/fncel.2015.00166
Ashor, A. W., Lara, J., Mathers, J. C., and Siervo, M. (2014). Effect of vitamin C on endothelial function in health and disease: a systematic review and meta-analysis of randomized controlled trials. Atherosclerosis 235, 9–20. doi: 10.1016/j.atherosclerosis.2014.04.004
Ashor, A. W., Siervo, M., van der Velde, F., Willis, N. D., and Mathers, J. C. (2016). Systematic review and meta-analysis of randomized controlled trials testing the effects of vitamin C supplementation on blood lipids. Clin. Nutr. 35, 626–637. doi: 10.1016/j.clnu.2015.05.021
Barros, M. M., Falcon, D. R., de Oliveira Orsi, R., Pezzato, L. E., Fernandes, A. C., Guimarães, I. G., et al. (2014). Non-specific immune parameters and physiological response of Nile tilapia fed β-glucan and vitamin C for different periods and submitted to stress and bacterial challenge. Fish Shellfish Immunol. 39, 188–195. doi: 10.1016/j.fsi.2014.05.004
Beurel, E., Grieco, S. F., and Jope, R. S. (2015). Glycogen synthase kinase-3 (GSK3): regulation, actions, and diseases. Pharmacol. Ther. 148, 114–131. doi: 10.1016/j.pharmthera.2014.11.016
Chen, C., Xiang, H., Peng, Y. L., Peng, J., and Jiang, S. W. (2014). Mature miR-183, negatively regulated by transcription factor GATA 3, promotes 3T3-L1 adipogenesis through inhibition of the canonical Wnt/β-catenin signaling pathway by targeting LRP6. Cell. Signal. 26, 1155–1165. doi: 10.1016/j.cellsig.2014.02.003
Chen, Y. J., Yuan, R. M., Liu, Y. J., Yang, H. J., Liang, G. Y., and Tian, L. X. (2015). Dietary vitamin C requirement and its effects on tissue antioxidant capacity of juvenile largemouth bass. Micropterus salmoides. Aquaculture 435, 431–436. doi: 10.1016/j.aquaculture.2014.10.013
Cho, C. Y., and Cowey, C. B. (1993). “Utilization of monophosphate esters of ascorbic acid by rainbow trout (Oncorhynchus mykiss),” in Fish Nutriton in Practice, Vol. 61, eds S. J. Kaushik and P. Luquet (Biarritz: INRA), 149–156.
Christie-David, D. J., Girgis, C. M., and Gunton, J. E. (2015). Effects of vitamins C and D in type 2 diabetes mellitus. Nutr. Diet. Suppl. 7, 21–28.
Dema, A., Schröter, M. F., Perets, E., Skroblin, P., Moutty, M. C., Deàk, V. A., et al. (2016). The A-kinase anchoring protein (AKAP) glycogen synthase kinase 3β interaction protein (GSKIP) regulates β-catenin through its interactions with both protein kinase A (PKA) and GSK3β. J. Biol. Chem. 291, 19618–19630. doi: 10.1074/jbc.M116.738047
Ellulu, M. S., Rahmat, A., Patimah, I., Khaza’ai, H., and Abed, Y. (2015). Effect of vitamin C on inflammation and metabolic markers in hypertensive and/or diabetic obese adults: a randomized controlled trial. Drug Des. Devel. Ther. 9, 3405–3412. doi: 10.2147/DDDT.S83144
Fracalossi, D. M., Allen, M. E., Yuyama, L. K., and Oftedal, O. T. (2001). Ascorbic acid biosynthesis in Amazonian fishes. Aquaculture 192, 321–332. doi: 10.1016/S0044-8486(00)00455-5
Gao, J., Koshio, S., Ishikawa, M., Yokoyama, S., Gnuyen, B. T., and Mamauag, R. E. (2013). Effects of dietary oxidized fish oil and vitamin C supplementation on growth performance and reduction of oxidative stress in Red Sea Bream Pagrus major. Aquac. Nutr. 19, 35–44. doi: 10.1111/j.1365-2095.2011.00921.x
Garcia-diaz, D. F., Lopez-Legarrea, P., Quintero, P., and Martinez, J. A. (2014). Vitamin C in the treatment and/or prevention of obesity. J. Nutr. Sci. Vitaminol. 60, 367–379. doi: 10.3177/jnsv.60.367
Hwang, D. F., and Lin, T. K. (2002). Effect of temperature on dietary vitamin C requirement and lipid in common carp. Comp. Biochem. Physiol. B Biochem. Mol. Biol. 131, 1–7. doi: 10.1016/S1096-4959(01)00449-3
Jain, S., Ghanghas, P., Rana, C., and Sanyal, S. N. (2017). Role of GSK-3β in regulation of canonical Wnt/β-catenin signaling and PI3-K/Akt oncogenic pathway in colon cancer. Cancer Invest. 35, 473–483. doi: 10.1080/07357907.2017.1337783
Jang, B. C. (2016). Artesunate inhibits adipogeneis in 3T3-L1 preadipocytes by reducing the expression and/or phosphorylation levels of C/EBP-α, PPAR-γ, FAS, perilipin A, and STAT-3. Biochem. Biophys. Res. Commun. 474, 220–225. doi: 10.1016/j.bbrc.2016.04.109
Karakilcik, A. Z., Halat, R., Zerin, M., Celik, H., and Nazligul, Y. (2014). Effects of vitamin C and exercise on lipid profile, platelet and erythrocyte indices in young soccer players. J. Sports Med. Phys. Fitness 54, 665–671.
Khan, K. U., Zuberi, A., Nazir, S., Ullah, I., Jamil, Z., and Sarwar, H. (2017). Synergistic effects of dietary nano selenium and vitamin C on growth, feeding, and physiological parameters of mahseer fish (Tor putitora). Aquac. Rep. 5, 70–75. doi: 10.1016/j.aqrep.2017.01.002
Kim, I. H., and Nam, T. J. (2017). Enzyme-treated Ecklonia cava extract inhibits adipogenesis through the downregulation of C/EBPα in 3T3-L1 adipocytes. Int. J. Mol. Med. 39, 636–644. doi: 10.3892/ijmm.2017.2869
Kim, M. B., Song, Y., Kim, C., and Hwang, J. K. (2014). Kirenol inhibits adipogenesis through activation of the Wnt/β-catenin signaling pathway in 3T3-L1 adipocytes. Biochem. Biophys. Res. Commun. 445, 433–438. doi: 10.1016/j.bbrc.2014.02.017
Kim, Y. M., Kim, I. H., Choi, J. W., Lee, M. K., and Nam, T. J. (2015). The anti-obesity effects of a tuna peptide on 3T3-L1 adipocytes are mediated by the inhibition of the expression of lipogenic and adipogenic genes and by the activation of the Wnt/β-catenin signaling pathway. Int. J. Mol. Med. 36, 327–334. doi: 10.3892/ijmm.2015.2231
Kobylecki, C. J., Afzal, S., Smith, G. D., and Nordestgaard, B. G. (2015). Genetically high plasma vitamin C, intake of fruit and vegetables, and risk of ischemic heart disease and all-cause mortality: a Mendelian randomization study. Am. J. Clin. Nutr. 101, 1135–1143. doi: 10.3945/ajcn.114.104497
Liu, D., Mai, K., Zhang, Y., Xu, W., and Ai, Q. (2016a). Tumour necrosis factor-α inhibits hepatic lipid deposition through GSK-3β/β-catenin signaling in juvenile turbot (Scophthalmus maximus L.). Gen. Comp. Endocrinol. 228, 1–8. doi: 10.1016/j.ygcen.2015.12.027
Liu, D., Mai, K., Zhang, Y., Xu, W., and Ai, Q. (2016b). Wnt/β-catenin signaling participates in the regulation of lipogenesis in the liver of juvenile turbot (Scophthalmus maximus L.). Comp. Biochem. Physiol. B Biochem. Mol. Biol. 191, 155–162. doi: 10.1016/j.cbpb.2015.11.002
Livak, K. J., and Schmittgen, T. D. (2001). Analysis of relative gene expression data using real time quantitative PCR and the 2ΔΔCT method. Methods 25, 402–408. doi: 10.1006/meth.2001.1262
Madhuban, B., and Kaviraj, A. (2009). Toxicity of the pyrethroid pesticide fenvalerate to fresh water catfish Clarias gariepinus: lethality, biochemical effects and role of dietary ascorbic acid. J. Environ. Sci. Health B 44, 578–583. doi: 10.1080/03601230903000602
Maqbool, M., Mobashir, M., and Hoda, N. (2016). Pivotal role of glycogen synthase kinase-3: a therapeutic target for Alzheimer’s disease. Eur. J. Med. Chem. 107, 63–81. doi: 10.1016/j.ejmech.2015.10.018
Mustin, W. G., and Lovell, R. T. (1992). Na-L-ascorbyl-2-monophosphate as a source of vitamin C for channel catfish. Aquaculture 105, 95–105. doi: 10.1016/0044-8486(92)90165-H
Nanton, D. A., Vegusdal, B., Rørå, A. M. B., Ruyter, B., Baeverfjord, G., and Torstensen, B. E. (2007). Muscle lipid storage pattern, composition, and adipocyte distribution in different parts of Atlantic salmon (Salmo salar) fed fish oil and vegetable oil. Aquaculture 265, 230–243. doi: 10.1016/j.aquaculture.2006.03.053
Norouzitallab, P., Farhangi, M., Babapour, M., Rahimi, R., Sinha, A. K., and Baruah, K. (2009). Comparing the efficacy of dietary a-tocopherol with that of DL-a-tocopheryl acetate, both either alone or in combination with ascorbic acid, on growth and stress resistance of angelfish, Pterophyllum scalare, juveniles. Aquac. Int. 17, 207–216. doi: 10.1007/s10499-008-9192-8
Park, S., Ham, J. O., and Lee, B. K. (2015). Effects of total vitamin A, vitamin C, and fruit intake on risk for metabolic syndrome in Korean women and men. Nutrition 31, 111–118. doi: 10.1016/j.nut.2014.05.011
Polidori, M. C., Ruggiero, C., Croce, M. F., Raichi, T., Mangialasche, F., Cecchetti, R., et al. (2015). Association of increased carotid intima–media thickness and lower plasma levels of vitamin C and vitamin E in old age subjects: implications for Alzheimer’s disease. J. Neural Transm. 122, 523–530. doi: 10.1007/s00702-014-1357-1
Popov, V. B., Jornayvaz, F. R., Akgul, E. O., Kanda, S., Jurczak, M. J., Zhang, D., et al. (2016). Second-generation antisense oligonucleotides against β-catenin protect mice against diet-induced hepatic steatosis and hepatic and peripheral insulin resistance. FASEB J. 30, 1207–1217. doi: 10.1096/fj.15-271999
Sealey, W. M., and Gatlin, M. I. I. I. (1999). Dietary vitamin C requirement of hybrid striped bass Morone chrysops ×M. saxatilis. J. World Aquac. Soc. 30, 297–301. doi: 10.1111/j.1749-7345.1999.tb00679.x
Shahkar, E., Yun, H., Kim, D. J., Kim, S. K., Lee, B. I., and Bai, S. C. (2015). Effects of dietary vitamin C levels on tissue ascorbic acid concentration, hematology, non-specific immune response and gonad histology in broodstock Japanese eel. Anguilla japonica. Aquaculture 438, 115–121. doi: 10.1016/j.aquaculture.2015.01.001
Sheridan, M. A. (1988). Lipid dynamics in fish: aspects of absorption, transportation, deposition and mobilization. Comp. Biochem. Physiol. B 90, 679–690. doi: 10.1016/0305-0491(88)90322-7
Shiau, S. Y., and Hsu, T. S. (1999). Quantification of vitamin C requirement for juvenile hybrid tilapia, Oreochromis niloticus ×Oreochromis aureus, with L-ascorbyl-2-monophosphate-Na and L-ascorbyl-2-monophosphate-Mg. Aquaculture 175, 317–326. doi: 10.1016/S0044-8486(99)00103-9
Taelman, V. F., Dobrowolski, R., Plouhinec, J. L., Fuentealba, L. C., Vorwald, P. P., Gumper, I., et al. (2010). Wnt signaling requires sequestration of glycogen synthase kinase 3 inside multivesicular endosomes. Cell 143, 1136–1148. doi: 10.1016/j.cell.2010.11.034
Teng, Z., Sun, C., Liu, S., Wang, H., and Zhang, S. (2014). Functional characterization of chitinase-3 reveals involvement of chitinases in early embryo immunity in zebrafish. Dev. Comp. Immunol. 46, 489–498. doi: 10.1016/j.dci.2014.06.008
Tian, J., Wang, K., Wang, X., Wen, H., Zhou, H., Liu, C., et al. (2018). Soybean saponin modulates nutrient sensing pathways and metabolism in zebrafish. Gen. Comp. Endocrinol. 257, 246–254. doi: 10.1016/j.ygcen.2017.10.010
Valenta, T., Hausmann, G., and Basler, K. (2012). The many faces and functions of beta-catenin. EMBO J. 31, 2714–2736. doi: 10.1038/emboj.2012.150
Wan, J., Ge, X., Liu, B., Xie, J., Cui, S., Zhou, M., et al. (2014). Effect of dietary vitamin C on non-specific immunity and mRNA expression of three heat shock proteins (HSPs) in juvenile Megalobrama amblycephala under pH stress. Aquaculture 434, 325–333. doi: 10.1016/j.aquaculture.2014.08.043
Wilson, R., Willis, J., Gearry, R., Skidmore, P., Fleming, E., Frampton, C., et al. (2017). Inadequate vitamin C status in prediabetes and type 2 diabetes mellitus: associations with glycaemic control, obesity, and smoking. Nutrients 9:997. doi: 10.3390/nu9090997
Xu, H., Wang, J., Chang, Y., Xu, J., Wang, Y., Long, T., et al. (2014). Fucoidan from the sea cucumber Acaudina molpadioides exhibits anti-adipogenic activity by modulating the Wnt/β-catenin pathway and down-regulating the SREBP-1c expression. Food Funct. 5, 1547–1555. doi: 10.1039/c3fo60716j
Zhang, P. Y., Xu, X., and Li, X. C. (2014). Cardiovascular diseases: oxidative damage and antioxidant protection. Eur. Rev. Med. Pharmacol. Sci. 18, 3091–3096.
Zhou, S., Ackman, R. G., and Morrison, C. (1995). Storage of lipids in the myosepta of Atlantic salmon (Salmo salar). Fish Physiol. Biochem. 14, 171–178. doi: 10.1007/BF00002460
Keywords: vitamin C, GSK-3β, β-catenin, C/EBPa, lipid-lowering effect, zebrafish
Citation: Liu D, Yu H, Pang Q and Zhang X (2018) Investigation of the Lipid-Lowering Effect of Vitamin C Through GSK-3β/β-Catenin Signaling in Zebrafish. Front. Physiol. 9:1023. doi: 10.3389/fphys.2018.01023
Received: 20 March 2018; Accepted: 10 July 2018;
Published: 14 August 2018.
Edited by:
Leonardo Julián Magnoni, Centro Interdisciplinar de Investigação Marinha e Ambiental (CIIMAR), PortugalReviewed by:
Ivan Viegas, University of Coimbra, PortugalEncarnación Capilla, University of Barcelona, Spain
Copyright © 2018 Liu, Yu, Pang and Zhang. This is an open-access article distributed under the terms of the Creative Commons Attribution License (CC BY). The use, distribution or reproduction in other forums is permitted, provided the original author(s) and the copyright owner(s) are credited and that the original publication in this journal is cited, in accordance with accepted academic practice. No use, distribution or reproduction is permitted which does not comply with these terms.
*Correspondence: Dongwu Liu, bGl1ZG9uZ3d1QHNkdXQuZWR1LmNu