- 1Department of Medicine, Cardiovascular Research Center, Icahn School of Medicine at Mount Sinai, New York, NY, United States
- 2Department of Genetics and Genomic Sciences, Icahn School of Medicine at Mount Sinai, New York, NY, United States
- 3Precision Immunology Institute, Icahn School of Medicine at Mount Sinai, New York, NY, United States
Cardiovascular health is a primary research focus, as it is a leading contributor to mortality and morbidity worldwide, and is prohibitively costly for healthcare. Atherosclerosis, the main driver of cardiovascular disease, is now recognized as an inflammatory disorder. Physical activity (PA) may have a more important role in cardiovascular health than previously expected. This review overviews the contribution of PA to cardiovascular health, the inflammatory role of atherosclerosis, and the emerging evidence of the microbiome as a regulator of inflammation.
An Overview of Atherosclerotic Cardiovascular Disease
Cardiovascular disease (CVD) is the worldwide leading cause of death and is a global economic burden (Cook et al., 2014). Most of the disease is driven by a process known as atherosclerosis—the buildup of plaque which occludes arterial vessels (Rajamani and Fisher, 2017). Throughout disease progression, the atherosclerotic plaque loses stability and becomes prone to rupture, a sudden event that can lead to arterial thrombosis and cause deleterious acute ischemic events like myocardial infarction (MI) and stroke (Gisterå and Hansson, 2017).
Atherosclerosis is influenced by a number of risk factors including, lifestyle choices (i.e., diet, physical activity, and cigarette smoking), advancing age, and associated disorders like hypertension, diabetes, obesity, and dyslipidemia (Tegos et al., 2001). However, traditional risk factors alone are inadequate at predicting atherosclerotic CVD. According to the Participants of Early Subclinical Atherosclerosis (PESA) study, subclinical atherosclerosis was detected in almost 50% of participants that were free of the conventional cardiovascular risk factors (Fernández-Friera et al., 2017). Statins, the gold standard treatment for lowering lipids (Grundy, 2016), have proven effective at reducing cardiovascular events, yet their contribution to reducing mortality remains questionable (Cholesterol Treatment Trialists’ [CTT] Collaborators, 2012; DuBroff and de Lorgeril, 2015). The recently developed proprotein convertase subtilisin-kexin type 9 (PCSK9) inhibitors effectively lowered cholesterol levels and reduced cardiovascular events when taken in conjunction with statins (Waters and Hsue, 2017). However, some of these studies failed to meet expectations based on the linear relationship between LDL reduction and percentage reduction of cardiovascular events based on data from 14 clinical trials using statins (Waters and Hsue, 2017). A possible explanation is that PCSK9 inhibitors failed to reduce C- reactive protein (CRP) levels, a clinical biomarker of inflammation and cardiovascular risk (Waters and Hsue, 2017).
Atherosclerosis is recognized as a chronic systemic inflammatory disease with focal manifestations at the vascular site (Ross, 1999; Conti and Shaik-Dasthagirisaeb, 2015). CVD is often pronounced in other immune disorders such as rheumatoid arthritis (RA) or systemic lupus erythematosus (SLE) (Gabriel, 2008; Abu-Shakra and Novack, 2012; Skeoch and Bruce, 2015; Henrot et al., 2018). Recent studies propose the gut microbiome as an emerging regulator of inflammatory conditions including atherosclerosis (Ridaura et al., 2013; Romano et al., 2015; Halfvarson et al., 2017; Kreznar et al., 2017; Slingerland et al., 2017).
The World Health Organization, American Heart Association, and European Society of Cardiology recommend several significant behavioral changes for the preventative care of CVD including smoking cessation, dietary changes, weight control, alcohol intake, and physical activity (PA) (Goff et al., 2014; Piepoli et al., 2016; Whelton et al., 2018). Of particular interest is the contribution of PA to CVD (Lin et al., 2015; Murtagh et al., 2015). Utilizing PA as therapy is particularly appealing because its implementation is cost-effective for the patient and the benefits can ease the global economic burden by reducing the cost of care (Valero-Elizondo et al., 2016). Recently, PA has been the focus of intense investigation for its ability to regulate the underlying immune system. This review will focus on the impact of PA as it relates to the regulation of systemic inflammation and the contribution of the microbiome.
Physical Activity and Cardiovascular Health
Regular PA is associated with many health benefits, including improving cholesterol levels, reducing body weight and blood pressure, increasing insulin sensitivity, and neuroprotective effects (Myers, 2003; Chieffi et al., 2017). Indeed, some of these benefits have been attributed to in part by the small neuropeptides called Orexins (Chieffi et al., 2017). Orexin A is released into plasma upon exercise, and contributes to regulating energy balance (Messina et al., 2016, 2017).
Numerous studies have established that PA is beneficial for reducing the risk and effect of CVD (Diaz et al., 2016; Vella et al., 2017; Florido et al., 2018). Physically fit individuals have a reduced risk of developing CVD (Kokkinos, 2012). A 10 year follow up study that surveyed senior participants found that leisure time PA reduced the risk of CVD incidents and mortality in a dose-dependent manner (Barengo et al., 2017). The Multi-Ethnic Study of Atherosclerosis (MESA) suggests that moderate to vigorous PA accompanies a more favorable inflammatory marker profile (Vella et al., 2017). The Atherosclerotic Risk in Communities (ARIC) Study evaluated participants with no history of CVD, and found that maintenance and engagement of PA were effective in decreasing heart failure risk (Florido et al., 2018). Additionally, the HUNT Study (Nord-Trondelag Health Study) showed that sustained PA, and not weight loss, substantially improved survival at 30 years follow up in individuals with coronary artery disease (Moholdt et al., 2018).
Physical activity has profound effects on vascular function and lumen dimension, and structural cardiac modifications (Green and Smith, 2017; Francavilla et al., 2018). For example PA regulates heart rate variability (HRV), a predictive factor for sudden cardiac death and MI (Sessa et al., 2018). Elderly athletes display less Carotid Intima Thickening and a more favorable HRV compared to their sedentary counterparts (Galetta et al., 2013; Soares-Miranda et al., 2016). Animal models have also demonstrated that old rats subjected to exercise training had reversed age-related microvascular dysfunction (Hotta et al., 2017).
Sedentary behavior (SB) is described as the lowest energy expenditure for waking activities (e.g., sitting or lying down), and is measured by metabolic equivalents (METs). Although uncoupling SB from associated illness and other risk factors like obesity and RA is difficult, SB promotes a pro-inflammatory status (Fenton et al., 2017). Biomarker analysis from a cross-sectional study of senior men found that higher levels of SB correlated with higher levels of pro-inflammatory markers IL-6, CRP, and tPA (Parsons et al., 2017). There is evidence that reallocation of SB with moderate to vigorous PA promotes a better inflammatory profile, with increased adiponectin levels and lower IL-6, C3, leptin, and leukocyte concentrations (Phillips et al., 2017). Moreover, patients with an inflammatory disease like RA, that have extended sedentary bouts have an increased risk of developing cardiovascular events and could benefit by interrupting sedentary time with leisurely PA (Fenton et al., 2017). Long-term studies, like the 15-years long Tanushimaru Study, confirm that decreased sitting time reduces the risk of mortality (Sakaue et al., 2017).
Inflammation and Atherosclerosis
The first critical step of atherosclerosis development is endothelial dysfunction and increased endothelial permeability that facilitates the build-up and deposition of low-density lipoproteins (LDLs) into the intima layer of the arterial wall where they become oxidized (oxLDL) (Lusis, 2000). Monocytes from the circulation infiltrate the arterial wall and differentiate into macrophages that engulf oxLDL becoming foam cells. Foam cells trapped in the intima layer become apoptotic and necrotic, which form the basis of a necrotic core (Ross, 1999; Gisterå and Hansson, 2017). Immune cells express cytokines and chemokines that are critical modulators of inflammatory signaling during atherogenesis (Turner et al., 2014; Ramji and Davies, 2015). Cytokines are also highly regulated during and as a consequence of exercise (Pedersen, 2017). Although there are many cytokines implicated in atherosclerosis, we will address the top key players, which are summarized in Table 1.
The initial injury to endothelial cells (ECs) by LDL typically occurs at the arterial branching points where laminar flow becomes disturbed, which results in morphological and functional changes that promote the permeability of the EC layer and allow retention of LDL (Moore et al., 2013). Activated ECs recruit immune cells by enhancing expression of adhesion molecules (e.g., VCAM and ICAM) and chemokines (Gimbrone and García-Cardeña, 2016). Pro-inflammatory chemokines are crucial for atherogenesis, for example, inhibition of a three chemokine axis (CCL2, CCL5, and CX3CL1) in mouse models leads to almost a complete attenuation of atherosclerosis (Ramji and Davies, 2015). Although chemokine pathways have been exploited as therapeutic targets (Sheikine and Hansson, 2006), few drugs have been FDA approved, but none for the treatment of atherosclerosis. For instance, the CCR5 antagonist Maraviroc was developed for the treatment of HIV and the low molecular weight CXCR4 antagonist Plerixafor (AMD3100) for stem cell mobilization (Koenen and Weber, 2011). In fact, modulating these pathways in complex disorders like CVD may be difficult due to the high risk of side effects like increased infection rate (Koenen and Weber, 2010).
Interferon-γ (IFN-γ) is a pro-inflammatory cytokine that functions as the primary activator of macrophages and has been shown to influence many stages of atherogenesis (Gupta et al., 1997; Voloshyna et al., 2014). IFN-γ enhances Monocyte Chemotactic Protein-1 (MCP-1) levels, a chemokine that recruits monocytes (Struyf et al., 1998) and the expression of Interferon-induced protein 35 (IFI35), a protein that contributes to EC proliferation and migration (Jian et al., 2018). Moreover, IFN-γ acts synergistically with other pro-inflammatory cytokines, like TNF-α to enhance chemokine production in monocytes and T-cells (Mehta et al., 2017).
Interleukin-1β (IL-1β) is a pro-inflammatory cytokine and a prime mediator of inflammation in CVD (Moss and Ramji, 2016). Therapeutic targeting of IL-1β in the Canakinumab Anti-inflammatory Thrombosis Outcomes Study (CANTOS) trial has provided the first proof-of-concept that inflammation is a key driver of cardiovascular events in high risk populations (Ridker et al., 2017). Inhibition of IL-1β reduced the risk of non-fatal CV events by 17% in patients with recent MI and elevated high sensitivity CRP (hs-CRP) (Ridker et al., 2017). Remarkably, a subgroup analysis of the CANTOS revealed that patients who achieved hs-CRP levels less than 2 mg/dl had a 25% reduction in major adverse cardiovascular events (Ridker et al., 2018), suggesting that the residual CV risk in this subpopulation was due to inflammation.
Interleukin-6 is a pleiotropic cytokine that is induced by IL-1β (Cahill and Rogers, 2008) and modulated by Canakinumab (Ridker et al., 2012). IL-6 can act as both a pro- or anti-inflammatory molecule, and thus its function is context dependent (Reiss et al., 2017). It participates in two distinct signaling mechanisms, binding to either the membrane-tethered IL-6 receptor (classical pathway) or a soluble form of the IL-6 receptor (trans-pathway). Trans-signaling is regarded as pro-inflammatory, while classical signaling is as anti-inflammatory (Scheller et al., 2011). Coronary artery disease patients have high levels of IL-6 which are also a predictive biomarker of the disease (Wainstein et al., 2017). However, mouse studies show that IL-6 depletion in ApoE-/- mice promotes atherosclerosis (Schieffer et al., 2004). Physical activity regulates IL-6 levels and acute exercise induces the release of IL-6 from the skeletal muscles into the circulatory system (Pedersen, 2017). Of note, IL-6 induced during exercise stimulates the release of anti-inflammatory cytokines IL-10 and IL-1ra (Pedersen, 2006, 2017). IL-6 is also a mediator of lipid metabolism that stimulates lipolysis and fat oxidation (van Hall et al., 2003) and has been shown to increase after a meal (Payette et al., 2009).
Immune Cells and Physical Activity
Exercise has a profound effect on both the adaptive and innate immune system by regulating immune cell populations.
On the innate arm, natural killer (NK) cells, neutrophils, and monocyte are all regulated by exercise (Koelwyn et al., 2015). NK cells are modulated during exercise by increasing in number and cytotoxic activity (Pedersen and Ullum, 1994; Na et al., 2000) and increased NK cell infiltration of tumors was observed upon exercise (Pedersen et al., 2016). Neutrophils, a hallmark of acute inflammation, in aging become dysfunctional (Jackaman et al., 2017). One study showed that PA promotes the migratory function of neutrophils in older adults (Bartlett et al., 2016). PA can also influence monocyte polarization with a decrease of classical monocytes, and an increase of intermediate and non-classical monocytes (Slusher et al., 2017). Another study found that strenuous, anaerobic exercise leads to the acute mobilization of intermediate monocytes by a catecholamine-dependent mechanism (Steppich et al., 2000). Particularly, acute exercise was associated with a reduction of monocyte-platelets aggregates, which are associated with increased risk of cardiovascular clinical events (Michelson et al., 2001).
Physical activity also regulates the expression of inflammatory markers and this effect is dependent on the exercise intensity. For example, studies in obese individuals subjected to either medium or high intensity exercise regimes demonstrated that the training intensity can modulate differently the expression of chemokine receptors. Moderate training promoted expression of CCR2 and CXCR2 on monocytes, while higher intensity training promoted CCR5 expression on monocytes, neutrophils, and T-cells (Barry et al., 2017).
On the adaptive side, either prolonged or strenuous exercise affects the balance of types of T-cells. Exercise promotes the predominance of Th2 cells versus Th1 cells. Th1 cells produce IL-2 and IFN-γ, while Th2 cells produce IL-4, IL-5, IL-6, and IL-10 (Sharif et al., 2018). Regulatory T-cells (Treg) belong to a subset of T-helper cells, are anti-inflammatory, atheroprotective (Foks et al., 2015), and are also affected by exercise. A study of elite Olympic athletes from various disciplines, found that athletes displayed higher frequencies of Tregs compared to age and sex-matched controls and this effect was associated with PA intensity (Weinhold et al., 2016). Tregs release immunosuppressive cytokines like IL-10, IL-35, and TGF-β (Olson et al., 2013), and decrease the production of pro-inflammatory cytokines like IFN-γ (Mallat et al., 2003; Ou et al., 2018).
The Microbiome and CVD: The Role of Physical Activity and Links to Inflammation
There is growing evidence indicating that the gut microbiome is a critical player in modulating host physiology (Clemente et al., 2012). Deviations from microbial homeostasis have been associated with various diseases, such as inflammatory bowel disease (Berg et al., 2015), arthritis (Clemente et al., 2018), or food allergies (Bunyavanich et al., 2016). Importantly, the microbiome has also been shown to play a critical role in obesity (Karlsson et al., 2012), atherosclerosis (Karlsson et al., 2012), and in the pathogenesis and progression of CVD (Kelly et al., 2016; Jie et al., 2017).
Karlsson et al. (2012) demonstrated that the genus Collinsella was enriched in patients with symptomatic atherosclerosis, while Roseburia and Eubacterium were enriched in healthy controls. These changes in bacterial composition were paralleled by enrichment in patients of bacterial genes encoding peptidoglycan synthesis (which might contribute to atherosclerosis by enhancing neutrophil function) and depletion of phytoene dehydrogenase and serum levels of b-carotene (hypothesized to have beneficial health effects) (Karlsson et al., 2012). A more recent study examined the association between gut microbiota and lifetime CVD risk in 112 participants in the Bogalusa Heart Study (Kelly et al., 2016). High risk participants had lower microbial diversity, as well as an increase in the abundance of Prevotella and Tyzzerella, and a decrease in Alloprevotella and Catenibacterium. A study in a cohort of 405 Chinese subjects found an enrichment in Enterobacteriaceae and Streptococcus spp. in atherosclerosis patients, suggesting that at least some of the associations between the gut microbiome and CVD might be population-specific (Jie et al., 2017).
The mechanisms by which the microbiome plays a role in CVD are however still poorly understood. One possible mechanism is the production of bacterial metabolites that induce the differentiation of pro- and anti-inflammatory cytokines, which has been demonstrated to be of critical importance in mouse models of colitis (Atarashi et al., 2013). Alternatively, the gut microbiota has also been shown to contribute to atherosclerosis through the conversion of choline or L-carnitine into TMAO (trimethylamine-N-oxide) (Koeth et al., 2013). Plasma TMAO is a biomarker of CVD risk associated with increased atherosclerotic stenosis, risk of major cardiovascular events, and mortality (Senthong et al., 2016). The deleterious effects of TMAO are hypothesized to be due to its promotion of platelet aggregation (Ridaura et al., 2013; Romano et al., 2015; Kreznar et al., 2017; Chen et al., 2018).
Given the reported associations between microbiome and CVD, several groups have investigated whether the beneficial effects of PA in CVD risk could be partially mediated by the changes induced in microbial composition. A study of professional rugby athletes and matched controls found that athletes had lower inflammatory status, and enrichment in bacterial diversity (Clarke et al., 2014). Differences were also observed in the abundance of 48 bacterial taxa, with notable enrichment in Ruminococcaceae, Succinivibrio, and Akkermansia in athletes compared to controls. However, diet was significantly distinct between the groups, and so the differences in microbial diversity and composition might be attributable both to PA and nutritional intake.
A more recent study aimed at disentangling the correlation between exercise, diet, and obesity status in shaping the gut microbiome. Eighteen lean and 14 obese subjects underwent 6 weeks of supervised endurance-based exercise training, followed by a washout period of 6 weeks in which they returned to a sedentary lifestyle (Allen et al., 2018b). Interestingly, changes in gut microbiome were dependent on obesity status, and short-chain fatty acids concentrations increased in lean but not in obese participants. Additionally, gut microbiome alterations disappeared once exercise ceased, suggesting that sustainment of PA is required for these changes to persist.
The causality of these associations is difficult to assess in human studies. Germ-free (GF) animals provide a suitable model to determine whether exercise-mediated changes in gut microbiome can induce specific phenotypes. Allen et al. (2018a) showed that transplanting the gut microbiome from either exercised or not exercised mice to GF mice induced specific distinct changes in the microbiome, metabolome, colonic inflammation, and body mass of the recipient mice (Allen et al., 2018a). Furthermore, colonization from exercised mice resulted in an attenuated response to DSS-induced colitis. These results demonstrate that exercise can alter gut microbiome and that those changes can result in beneficial health outcomes for the host.
Overall, current evidence points to an association between CVD and gut microbiome through the production of bacterial metabolites that induce potent host pro- or anti-inflammatory responses. Strategies to alter bacterial content are therefore of high interest for their potential therapeutic value. Together with diet (Llewellyn et al., 2017) and fecal transplants (Paramsothy et al., 2017), PA represents an additional approach through which the beneficial effects of gut microbiome modulation can be achieved.
Conclusion
Ongoing research focusing on the immune system and CVD continues to probe the molecular intricacies that contribute to atherosclerosis, including the individual contribution of immune cells, cytokines, and the microbiome (summarized in Figure 1). While an association between systemic inflammation, gut microbiome, and CVD is emerging, there is a great need to further our understanding of what constitutes normal biological variation versus pathological changes.
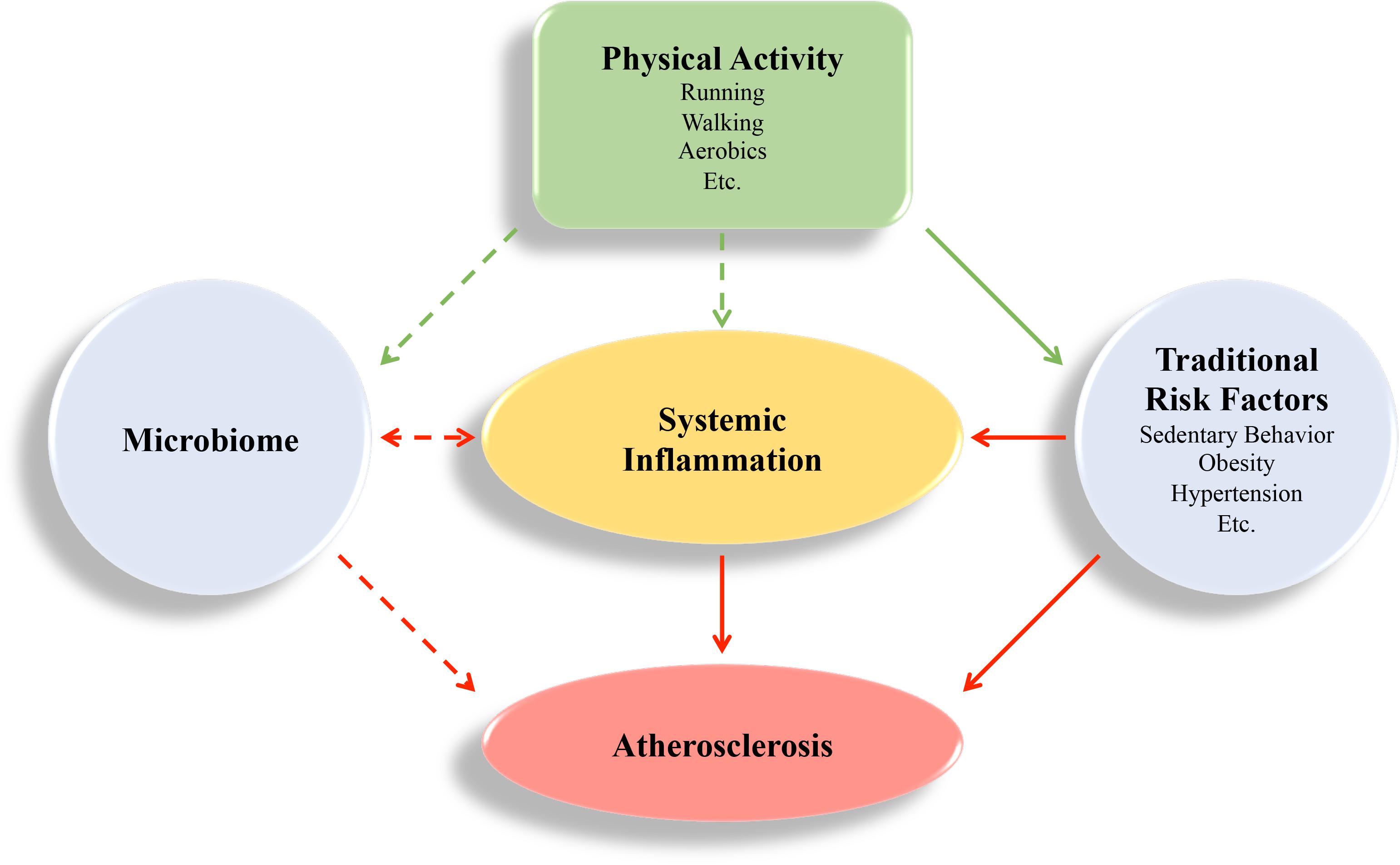
FIGURE 1. Visual Summary. Atherosclerosis is an inflammatory disease that is regulated by systemic inflammation. Physical activity can have beneficial effects (green arrows) by regulating systemic inflammation directly, and through behavioral and environmental factors that cause inflammation, like sedentary behavior, obesity, hypertension, and the microbiome. These risk factors contribute to deleterious effects (red arrows) and promote atherosclerosis development. The impact of the microbiome on atherogenesis is an emerging field, and the mechanism of how physical activity regulates the microbiome is not completely understood. Inflammation may also regulate the microbiome (van den Elsen et al., 2017; Chen et al., 2018).
Immune modulatory therapeutics like Canakinumab show great promise to aid in the treatment of atherosclerosis, yet, the development of molecularly targeted pharmacological intervention is a challenging process due to the multifactorial nature of atherosclerosis. Additionally, the use of drugs to control atherosclerosis can be very costly posing a growing economic burden on the society. PA has proven to be a general modulator of systemic inflammation with some emerging effects on the gut microbiome that may be beneficial to overall cardiovascular health. While more research is needed to understand the implications of these changes, improving healthy behaviors by incorporating PA as part of a healthy lifestyle is a promising way to combat CVD.
Author Contributions
DF, JC, and CG were responsible for writing and editing the manuscript.
Funding
DF was supported by the NIH (Grant No. 5T32HL007824-20). CG was funded by NIH (Grant Nos. K23HL111339, R03HL135289, R21TR001739, and UH2TR002067) and partially supported by The Leducq Foundation Consortium “CAD Genomics.” JC was partially supported by Grant No. R01MH110418.
Conflict of Interest Statement
The authors declare that the research was conducted in the absence of any commercial or financial relationships that could be construed as a potential conflict of interest.
References
Abu-Shakra, M., and Novack, V. (2012). Mortality and multiple causes of death in systemic lupus erythematosus – role of the death certificate. J. Rheumatol. 39, 458–460. doi: 10.3899/jrheum.111556
Allen, J. M., Mailing, L. J., Cohrs, J., Salmonson, C., Fryer, J. D., Nehra, V., et al. (2018a). Exercise training-induced modification of the gut microbiota persists after microbiota colonization and attenuates the response to chemically-induced colitis in gnotobiotic mice. Gut Microbes 9, 115–130. doi: 10.1080/19490976.2017.1372077
Allen, J. M., Mailing, L. J., Niemiro, G. M., Moore, R., Cook, M. D., White, B. A., et al. (2018b). Exercise alters gut microbiota composition and function in lean and obese humans. Med. Sci. Sports Exerc. 50, 747–757. doi: 10.1249/MSS.0000000000001495
Apostolakis, S., Krambovitis, E., Vlata, Z., Kochiadakis, G. E., Baritaki, S., and Spandidos, D. A. (2007). CX3CR1 receptor is up-regulated in monocytes of coronary artery diseased patients: impact of pre-inflammatory stimuli and renin–angiotensin system modulators. Thromb. Res. 121, 387–395. doi: 10.1016/j.thromres.2007.04.005
Atarashi, K., Tanoue, T., Oshima, K., Suda, W., Nagano, Y., Nishikawa, H., et al. (2013). Treg induction by a rationally selected mixture of Clostridia strains from the human microbiota. Nature 500, 232–236. doi: 10.1038/nature12331
Barengo, N. C., Antikainen, R., Borodulin, K., Harald, K., and Jousilahti, P. (2017). Leisure-time physical activity reduces total and cardiovascular mortality and cardiovascular disease incidence in older adults. J. Am. Geriatr. Soc. 65, 504–510. doi: 10.1111/jgs.14694
Barry, J. C., Simtchouk, S., Durrer, C., Jung, M. E., and Little, J. P. (2017). Short-term exercise training alters leukocyte chemokine receptors in obese adults. Med. Sci. Sport Exerc. 49, 1631–1640. doi: 10.1249/MSS.0000000000001261
Bartlett, D. B., Fox, O., McNulty, C. L., Greenwood, H. L., Murphy, L., Sapey, E., et al. (2016). Habitual physical activity is associated with the maintenance of neutrophil migratory dynamics in healthy older adults. Brain Behav. Immun. 56, 12–20. doi: 10.1016/j.bbi.2016.02.024
Baturcam, E., Abubaker, J., Tiss, A., Abu-Farha, M., Khadir, A., Al-Ghimlas, F., et al. (2014). Physical exercise reduces the expression of RANTES and its CCR5 receptor in the adipose tissue of obese humans. Mediators Inflamm. 2014:627150. doi: 10.1155/2014/627150
Berg, D., Clemente, J. C., and Colombel, J.-F. (2015). Can inflammatory bowel disease be permanently treated with short-term interventions on the microbiome? Expert Rev. Gastroenterol. Hepatol. 9, 781–795. doi: 10.1586/17474124.2015.1013031
Branen, L., Hovgaard, L., Nitulescu, M., Bengtsson, E., Nilsson, J., and Jovinge, S. (2004). Inhibition of tumor necrosis factor- reduces atherosclerosis in apolipoprotein e knockout mice. Arterioscler. Thromb. Vasc. Biol. 24, 2137–2142. doi: 10.1161/01.ATV.0000143933.20616.1b
Bunyavanich, S., Shen, N., Grishin, A., Wood, R., Burks, W., Dawson, P., et al. (2016). Early-life gut microbiome composition and milk allergy resolution. J. Allergy Clin. Immunol. 138, 1122–1130. doi: 10.1016/j.jaci.2016.03.041
Cahill, C. M., and Rogers, J. T. (2008). Interleukin (IL) 1β induction of IL-6 is mediated by a novel phosphatidylinositol 3-kinase-dependent AKT/IκB kinase α pathway targeting activator protein-1. J. Biol. Chem. 283, 25900–25912. doi: 10.1074/jbc.M707692200
Chen, J., Guo, Y., Gui, Y., and Xu, D. (2018). Physical exercise, gut, gut microbiota, and atherosclerotic cardiovascular diseases. Lipids Health Dis. 17:17. doi: 10.1186/s12944-017-0653-9
Chieffi, S., Carotenuto, M., Monda, V., Valenzano, A., Villano, I., Precenzano, F., et al. (2017). Orexin system: the key for a healthy life. Front. Physiol. 8:357. doi: 10.3389/fphys.2017.00357
Cholesterol Treatment Trialists’ [CTT] Collaborators (2012). The effects of lowering LDL cholesterol with statin therapy in people at low risk of vascular disease: meta-analysis of individual data from 27 randomised trials. Lancet 380, 581–590. doi: 10.1016/S0140-6736(12)60367-5
Clarke, S. F., Murphy, E. F., O’Sullivan, O., Lucey, A. J., Humphreys, M., Hogan, A., et al. (2014). Exercise and associated dietary extremes impact on gut microbial diversity. Gut 63, 1913–1920. doi: 10.1136/gutjnl-2013-306541
Clemente, J. C., Manasson, J., and Scher, J. U. (2018). The role of the gut microbiome in systemic inflammatory disease. BMJ 360:j5145. doi: 10.1136/BMJ.J5145
Clemente, J. C., Ursell, L. K., Parfrey, L. W., and Knight, R. (2012). The impact of the gut microbiota on human health: an integrative view. Cell 148, 1258–1270. doi: 10.1016/j.cell.2012.01.035
Conti, P., and Shaik-Dasthagirisaeb, Y. (2015). Atherosclerosis: a chronic inflammatory disease mediated by mast cells. Cent. J. Immunol. 40, 380–386. doi: 10.5114/ceji.2015.54603
Cook, C., Cole, G., Asaria, P., Jabbour, R., and Francis, D. P. (2014). The annual global economic burden of heart failure. Int. J. Cardiol. 171, 368–376. doi: 10.1016/j.ijcard.2013.12.028
Davenport, P., and Tipping, P. G. (2003). The role of interleukin-4 and interleukin-12 in the progression of atherosclerosis in apolipoprotein E-deficient mice. Am. J. Pathol. 163, 1117–1125. doi: 10.1016/S0002-9440(10)63471-2
Diaz, K. M., Booth, J. N., Seals, S. R., Hooker, S. P., Sims, M., Dubbert, P. M., et al. (2016). Sedentary behavior and subclinical atherosclerosis in African Americans: cross-sectional analysis of the Jackson heart study. Int. J. Behav. Nutr. Phys. Act. 13:31. doi: 10.1186/s12966-016-0349-y
DuBroff, R., and de Lorgeril, M. (2015). Cholesterol confusion and statin controversy. World J. Cardiol. 7, 404–409. doi: 10.4330/wjc.v7.i7.404
Eefting, D., Schepers, A., De Vries, M. R., Pires, N. M. M., Grimbergen, J. M., Lagerweij, T., et al. (2007). The effect of interleukin-10 knock-out and overexpression on neointima formation in hypercholesterolemic APOE∗3-Leiden mice. Atherosclerosis 193, 335–342. doi: 10.1016/j.atherosclerosis.2006.09.032
Fenton, S. A. M., Veldhuijzen van Zanten, J. J. C. S., Kitas, G. D., Duda, J. L., Rouse, P. C., Yu, C.-A., et al. (2017). Sedentary behaviour is associated with increased long-term cardiovascular risk in patients with rheumatoid arthritis independently of moderate-to-vigorous physical activity. BMC Musculoskelet. Disord. 18:131. doi: 10.1186/s12891-017-1473-9
Fernández-Friera, L., Fuster, V., López-Melgar, B., Oliva, B., García-Ruiz, J. M., Mendiguren, J., et al. (2017). Normal LDL-cholesterol levels are associated with subclinical atherosclerosis in the absence of risk factors. J. Am. Coll. Cardiol. 70, 2979–2991. doi: 10.1016/j.jacc.2017.10.024
Florido, R., Kwak, L., Lazo, M., Nambi, V., Ahmed, H. M., Hegde, S. M., et al. (2018). Six-year changes in physical activity and the risk of incident heart failure: the atherosclerosis risk in communities (ARIC) study. Circulation 137, 2142–2151. doi: 10.1161/CIRCULATIONAHA.117.030226
Foks, A. C., Lichtman, A. H., and Kuiper, J. (2015). Treating atherosclerosis with regulatory T cells. Arterioscler. Thromb. Vasc. Biol. 35, 280–287. doi: 10.1161/ATVBAHA.114.303568
Francavilla, C. V., Sessa, F., Salerno, M., Albano, G. D., Villano, I., Messina, G., et al. (2018). Influence of football on physiological cardiac indexes in professional and young athletes. Front. Physiol. 9:153. doi: 10.3389/fphys.2018.00153
Gabriel, S. E. (2008). Cardiovascular morbidity and mortality in rheumatoid arthritis. Am. J. Med. 121, S9–S14. doi: 10.1016/j.amjmed.2008.06.011
Galetta, F., Franzoni, F., Tocchini, L., Camici, M., Milanesi, D., Belatti, F., et al. (2013). Effect of physical activity on heart rate variability and carotid intima-media thickness in older people. Intern. Emerg. Med. 8, 27–29. doi: 10.1007/s11739-013-0919-9
Gimbrone, M. A., and García-Cardeña, G. (2016). Endothelial cell dysfunction and the pathobiology of atherosclerosis. Circ. Res. 118, 620–636. doi: 10.1161/CIRCRESAHA.115.306301
Gisterå, A., and Hansson, G. K. (2017). The immunology of atherosclerosis. Nat. Rev. Nephrol. 13, 368–380. doi: 10.1038/nrneph.2017.51
Goff, D. C., Lloyd-Jones, D. M., Bennett, G., Coady, S., D’Agostino, R. B., Gibbons, R., et al. (2014). 2013 ACC/AHA guideline on the assessment of cardiovascular risk: a report of the American College of Cardiology/American Heart Association Task Force on Practice Guidelines. Circulation 129, S49–S73. doi: 10.1161/01.cir.0000437741.48606.98
Green, D. J., and Smith, K. J. (2017). Effects of exercise on vascular function, structure, and health in humans. Cold Spring Harb. Perspect. Med. 8:a029819. doi: 10.1101/cshperspect.a029819
Grundy, S. M. (2016). Primary prevention of cardiovascular disease with statins: assessing the evidence base behind clinical guidance. Clin. Pharm. 8. doi: 10.1211/CP.2016.20200568
Gupta, S., Pablo, A. M., Jiang, X. C., Wang, N., Tall, A. R., and Schindler, C. (1997). IFN-gamma potentiates atherosclerosis in ApoE knock-out mice. J. Clin. Invest. 99, 2752–2761. doi: 10.1172/JCI119465
Halfvarson, J., Brislawn, C. J., Lamendella, R., Vázquez-Baeza, Y., Walters, W. A., Bramer, L. M., et al. (2017). Dynamics of the human gut microbiome in inflammatory bowel disease. Nat. Microbiol. 2:17004. doi: 10.1038/nmicrobiol.2017.4
Henrot, P., Foret, J., Barnetche, T., Lazaro, E., Duffau, P., Seneschal, J., et al. (2018). Assessment of subclinical atherosclerosis in systemic lupus erythematosus: a systematic review and meta-analysis. Joint Bone Spine 85, 155–163. doi: 10.1016/j.jbspin.2017.12.009
Hotta, K., Chen, B., Behnke, B. J., Ghosh, P., Stabley, J. N., Bramy, J. A., et al. (2017). Exercise training reverses age-induced diastolic dysfunction and restores coronary microvascular function. J. Physiol. 595, 3703–3719. doi: 10.1113/JP274172
Huber, S. A., Sakkinen, P., Conze, D., Hardin, N., and Tracy, R. (1999). Interleukin-6 exacerbates early atherosclerosis in mice. Arterioscler. Thromb. Vasc. Biol. 19, 2364–2367. doi: 10.1161/01.ATV.19.10.2364
Jackaman, C., Tomay, F., Duong, L., Abdol Razak, N. B., Pixley, F. J., Metharom, P., et al. (2017). Aging and cancer: the role of macrophages and neutrophils. Ageing Res. Rev. 36, 105–116. doi: 10.1016/j.arr.2017.03.008
Jian, D., Wang, W., Zhou, X., Jia, Z., Wang, J., Yang, M., et al. (2018). Interferon-induced protein 35 inhibits endothelial cell proliferation, migration and re-endothelialization of injured arteries by inhibiting the nuclear factor-kappa B pathway. Acta Physiol. doi: 10.1111/apha.13037 [Epub ahead of print].
Jie, Z., Xia, H., Zhong, S.-L., Feng, Q., Li, S., Liang, S., et al. (2017). The gut microbiome in atherosclerotic cardiovascular disease. Nat. Commun. 8:845. doi: 10.1038/s41467-017-00900-1
Karlsson, F. H., Fåk, F., Nookaew, I., Tremaroli, V., Fagerberg, B., Petranovic, D., et al. (2012). Symptomatic atherosclerosis is associated with an altered gut metagenome. Nat. Commun. 3:1245. doi: 10.1038/ncomms2266
Kelly, T. N., Bazzano, L. A., Ajami, N. J., He, H., Zhao, J., Petrosino, J. F., et al. (2016). Gut microbiome associates with lifetime cardiovascular disease risk profile among Bogalusa heart study participants. Circ. Res. 119, 956–964. doi: 10.1161/CIRCRESAHA.116.309219
King, V. L., Cassis, L. A., and Daugherty, A. (2007). Interleukin-4 does not influence development of hypercholesterolemia or angiotensin II-induced atherosclerotic lesions in mice. Am. J. Pathol. 171, 2040–2047. doi: 10.2353/ajpath.2007.060857
Kirii, H., Niwa, T., Yamada, Y., Wada, H., Saito, K., Iwakura, Y., et al. (2003). Lack of Interleukin-1beta decreases the severity of atherosclerosis in ApoE-deficient mice. Arterioscler. Thromb. Vasc. Biol. 23, 656–660. doi: 10.1161/01.ATV.0000064374.15232.C3
Koelwyn, G. J., Wennerberg, E., Demaria, S., and Jones, L. W. (2015). Exercise in regulation of inflammation-immune axis function in cancer initiation and progression. Oncology 29, 908–920. doi: 10.1016/j.semcancer.2015.04.010.Targeting
Koenen, R. R., and Weber, C. (2010). Therapeutic targeting of chemokine interactions in atherosclerosis. Nat. Rev. Drug Discov. 9, 141–153. doi: 10.1038/nrd3048
Koenen, R. R., and Weber, C. (2011). Chemokines: established and novel targets in atherosclerosis. EMBO Mol. Med. 3, 713–725. doi: 10.1002/emmm.201100183
Koeth, R. A., Wang, Z., Levison, B. S., Buffa, J. A., Org, E., Sheehy, B. T., et al. (2013). Intestinal microbiota metabolism of L-carnitine, a nutrient in red meat, promotes atherosclerosis. Nat. Med. 19, 576–585. doi: 10.1038/nm.3145
Kokkinos, P. (2012). Physical activity, health benefits, and mortality risk. ISRN Cardiol. 2012:718789. doi: 10.5402/2012/718789
Kreznar, J. H., Keller, M. P., Traeger, L. L., Rabaglia, M. E., Schueler, K. L., Stapleton, D. S., et al. (2017). Host genotype and gut microbiome modulate insulin secretion and diet-induced metabolic phenotypes. Cell Rep. 18, 1739–1750. doi: 10.1016/j.celrep.2017.01.062
Lin, J., Kakkar, V., and Lu, X. (2014). Impact of MCP-1 in atherosclerosis. Curr. Pharm. Des. 20, 4580–4588. doi: 10.2174/1381612820666140522115801
Lin, X., Zhang, X., Guo, J., Roberts, C. K., McKenzie, S., Wu, W.-C., et al. (2015). Effects of exercise training on cardiorespiratory fitness and biomarkers of cardiometabolic health: a systematic review and meta-analysis of randomized controlled trials. J. Am. Heart Assoc 4, 1–28. doi: 10.1161/JAHA.115.002014
Llewellyn, S. R., Britton, G. J., Contijoch, E. J., Vennaro, O. H., Mortha, A., Colombel, J.-F., et al. (2017). Interactions between diet and the intestinal microbiota alter intestinal permeability and colitis severity in mice. Gastroenterology 154, 1037.e2–1046.e2. doi: 10.1053/j.gastro.2017.11.030
Mallat, Z., Gojova, A., Brun, V., Esposito, B., Fournier, N., Cottrez, F., et al. (2003). Induction of a regulatory T cell type 1 response reduces the development of atherosclerosis in apolipoprotein E-knockout mice. Circulation 108, 1232–1237. doi: 10.1161/01.CIR.0000089083.61317.A1
Mehta, N. N., Teague, H. L., Swindell, W. R., Baumer, Y., Ward, N. L., Xing, X., et al. (2017). IFN-γ and TNF-α synergism may provide a link between psoriasis and inflammatory atherogenesis. Sci. Rep. 7:13831. doi: 10.1038/s41598-017-14365-1
Messina, G., Di Bernardo, G., Viggiano, A., De Luca, V., Monda, V., Messina, A., et al. (2016). Exercise increases the level of plasma orexin A in humans. J. Basic Clin. Physiol. Pharmacol. 27, 611–616. doi: 10.1515/jbcpp-2015-0133
Messina, G., Valenzano, A., Moscatelli, F., Salerno, M., Lonigro, A., Esposito, T., et al. (2017). Role of autonomic nervous system and orexinergic system on adipose tissue. Front. Physiol. 8:137. doi: 10.3389/fphys.2017.00137
Michelson, A. D., Barnard, M. R., Krueger, L. A., Valeri, C. R., and Furman, M. I. (2001). Circulating monocyte-platelet aggregates are a more sensitive marker of in vivo platelet activation than platelet surface P-selectin: studies in baboons, human coronary intervention, and human acute myocardial infarction. Circulation 104, 1533–1537. doi: 10.1161/hc3801.095588
Moholdt, T., Lavie, C. J., and Nauman, J. (2018). Sustained physical activity, not weight loss, associated with improved survival in coronary heart disease. J. Am. Coll. Cardiol. 71, 1094–1101. doi: 10.1016/j.jacc.2018.01.011
Moldoveanu, A. I., Shephard, R. J., and Shek, P. N. (2000). Exercise elevates plasma levels but not gene expression of IL-1β, IL-6, and TNF-α in blood mononuclear cells. J. Appl. Physiol. 89, 1499–1504. doi: 10.1152/jappl.2000.89.4.1499
Moore, K. J., Sheedy, F. J., and Fisher, E. A. (2013). Macrophages in atherosclerosis: a dynamic balance. Nat. Rev. Immunol. 13, 709–721. doi: 10.1038/nri3520
Moss, J. W., and Ramji, D. P. (2016). Cytokines: roles in atherosclerosis disease progression and potential therapeutic targets. Future Med. Chem. 8, 1317–1330. doi: 10.4155/fmc-2016-0072
Murtagh, E. M., Nichols, L., Mohammed, M. A., Holder, R., Nevill, A. M., and Murphy, M. H. (2015). The effect of walking on risk factors for cardiovascular disease: an updated systematic review and meta-analysis of randomised control trials. Prev. Med. 72, 34–43. doi: 10.1016/j.ypmed.2014.12.041
Myers, J. (2003). Cardiology patient pages. Exercise and cardiovascular health. Circulation 107, e2–e5. doi: 10.1161/01.CIR.0000048890.59383.8D
Na, Y. M., Kim, M. Y., Kim, Y. K., Ha, Y. R., and Yoon, D. S. (2000). Exercise therapy effect on natural killer cell cytotoxic activity in stomach cancer patients after curative surgery. Arch. Phys. Med. Rehabil. 81, 777–779. doi: 10.1053/apmr.2000.3871
Olson, B. M., Sullivan, J. A., and Burlingham, W. J. (2013). Interleukin 35: a key mediator of suppression and the propagation of infectious tolerance. Front. Immunol. 4:315. doi: 10.3389/fimmu.2013.00315
Ou, H.-X., Guo, B.-B., Liu, Q., Li, Y.-K., Yang, Z., Feng, W.-J., et al. (2018). Regulatory T cells as a new therapeutic target for atherosclerosis. Acta Pharmacol. Sin. doi: 10.1038/aps.2017.140 [Epub ahead of print].
Palomo, I., Herrera, J., Cancino, V., Alarcón, M., Mujica, V., Leiva, E., et al. (2010). Physical activity reduces circulating TNF-alpha but not pro-thrombotic factors levels in patients with metabolic syndrome. Diabetes Metab. Syndr. Clin. Res. Rev. 4, 234–238. doi: 10.1016/J.DSX.2010.05.004
Paramsothy, S., Kamm, M. A., Kaakoush, N. O., Walsh, A. J., van den Bogaerde, J., Samuel, D., et al. (2017). Multidonor intensive faecal microbiota transplantation for active ulcerative colitis: a randomised placebo-controlled trial. Lancet 389, 1218–1228. doi: 10.1016/S0140-6736(17)30182-4
Parsons, T. J., Sartini, C., Welsh, P., Sattar, N., Ash, S., Lennon, L. T., et al. (2017). Physical activity, sedentary behavior, and inflammatory and hemostatic markers in men. Med. Sci. Sports Exerc. 49, 459–465. doi: 10.1249/MSS.0000000000001113
Payette, C., Blackburn, P., Lamarche, B., Tremblay, A., Bergeron, J., Lemieux, I., et al. (2009). Sex differences in postprandial plasma tumor necrosis factor-α, interleukin-6, and C-reactive protein concentrations. Metabolism 58, 1593–1601. doi: 10.1016/j.metabol.2009.05.011
Pedersen, B. K. (2006). The anti-inflammatory effect of exercise: its role in diabetes and cardiovascular disease control. Essays Biochem. 42, 105–117. doi: 10.1042/bse0420105
Pedersen, B. K. (2017). Anti-inflammatory effects of exercise: role in diabetes and cardiovascular disease. Eur. J. Clin. Invest. 47, 600–611. doi: 10.1111/eci.12781
Pedersen, B. K., Steensberg, A., and Schjerling, P. (2001). Muscle-derived interleukin-6: possible biological effects. J. Physiol. 536, 329–337. doi: 10.1111/J.1469-7793.2001.0329C.XD
Pedersen, B. K., and Ullum, H. (1994). NK cell response to physical activity: possible mechanisms of action. Med. Sci. Sports Exerc. 26, 140–146. doi: 10.1249/00005768-199402000-00003
Pedersen, L., Idorn, M., Olofsson, G. H., Lauenborg, B., Nookaew, I., Hansen, R. H., et al. (2016). Voluntary running suppresses tumor growth through epinephrine- and IL-6-dependent NK cell mobilization and redistribution. Cell Metab. 23, 554–562. doi: 10.1016/j.cmet.2016.01.011
Phillips, C. M., Dillon, C. B., and Perry, I. J. (2017). Does replacing sedentary behaviour with light or moderate to vigorous physical activity modulate inflammatory status in adults? Int. J. Behav. Nutr. Phys. Act. 14:138. doi: 10.1186/s12966-017-0594-8
Piepoli, M. F., Hoes, A. W., Agewall, S., Albus, C., Brotons, C., Catapano, A. L., et al. (2016). 2016 European Guidelines on cardiovascular disease prevention in clinical practice. Eur. Heart J. 37, 2315–2381. doi: 10.1093/eurheartj/ehw106
Rajamani, K., and Fisher, M. (2017). “An overview of atherosclerosis,” in Primer on Cerebrovascular Diseases, 2nd Edn, eds L. R. Caplan, J. Biller, M. Leary, E. Lo, A. Thomas, M. Yenari., et al. (Cambridge, MA: Academic Press), 105–108. doi: 10.1016/B978-0-12-803058-5.00020-5
Ramji, D. P., and Davies, T. S. (2015). Cytokines in atherosclerosis: key players in all stages of disease and promising therapeutic targets. Cytokine Growth Factor Rev. 26, 673–685. doi: 10.1016/j.cytogfr.2015.04.003
Reiss, A. B., Siegart, N. M., and De Leon, J. (2017). Interleukin-6 in atherosclerosis: atherogenic or atheroprotective? Clin. Lipidol. 12, 14–23. doi: 10.1080/17584299.2017.1319787
Ridaura, V. K., Faith, J. J., Rey, F. E., Cheng, J., Duncan, A. E., Kau, A. L., et al. (2013). Gut microbiota from twins discordant for obesity modulate metabolism in mice. Science 341:1241214. doi: 10.1126/science.1241214
Ridker, P. M., Everett, B. M., Thuren, T., MacFadyen, J. G., Chang, W. H., Ballantyne, C., et al. (2017). Antiinflammatory therapy with canakinumab for atherosclerotic disease. N. Engl. J. Med. 377, 1119–1131. doi: 10.1056/NEJMoa1707914
Ridker, P. M., Howard, C. P., Walter, V., Everett, B., Libby, P., Hensen, J., et al. (2012). Effects of interleukin-1β inhibition with canakinumab on hemoglobin A1c, lipids, C-reactive protein, interleukin-6, and fibrinogen: a phase IIb randomized, placebo-controlled trial. Circulation 126, 2739–2748. doi: 10.1161/CIRCULATIONAHA.112.122556
Ridker, P. M., MacFadyen, J. G., Everett, B. M., Libby, P., Thuren, T., Glynn, R. J., et al. (2018). Relationship of C-reactive protein reduction to cardiovascular event reduction following treatment with canakinumab: a secondary analysis from the CANTOS randomised controlled trial. Lancet 391, 319–328. doi: 10.1016/S0140-6736(17)32814-3
Robertson, A.-K. L., Rudling, M., Zhou, X., Gorelik, L., Flavell, R. A., and Hansson, G. K. (2003). Disruption of TGF-beta signaling in T cells accelerates atherosclerosis. J. Clin. Invest. 112, 1342–1350. doi: 10.1172/JCI18607
Romano, K. A., Vivas, E. I., Amador-Noguez, D., and Rey, F. E. (2015). Intestinal microbiota composition modulates choline bioavailability from diet and accumulation of the proatherogenic metabolite trimethylamine-N-oxide. MBio 6:e02481. doi: 10.1128/mBio.02481-14
Ross, R. (1999). Atherosclerosis — An inflammatory disease. N. Engl. J. Med. 340, 115–126. doi: 10.1056/NEJM199901143400207
Sakaue, A., Adachi, H., Enomoto, M., Fukami, A., Kumagai, E., Nakamura, S., et al. (2017). Association between physical activity, sitting time and mortality in a general population: the 15-year prospective survey in the Tanushimaru study. Atherosclerosis 263, e186–e187. doi: 10.1016/J.ATHEROSCLEROSIS.2017.06.598
Scheller, J., Chalaris, A., Schmidt-Arras, D., and Rose-John, S. (2011). The pro- and anti-inflammatory properties of the cytokine interleukin-6. Biochim. Biophys. Acta 1813, 878–888. doi: 10.1016/J.BBAMCR.2011.01.034
Schieffer, B., Selle, T., Hilfiker, A., Hilfiker-Kleiner, D., Grote, K., Tietge, U. J. F., et al. (2004). Impact of interleukin-6 on plaque development and morphology in experimental atherosclerosis. Circulation 110, 3493–3500. doi: 10.1161/01.CIR.0000148135.08582.97
Schild, M., Eichner, G., Beiter, T., Zügel, M., Krumholz-Wagner, I., Hudemann, J., et al. (2016). Effects of acute endurance exercise on plasma protein profiles of endurance-trained and untrained individuals over time. Mediators Inflamm. 2016:4851935. doi: 10.1155/2016/4851935
Senthong, V., Wang, Z., Fan, Y., Wu, Y., Hazen, S. L., and Tang, W. H. W. (2016). Trimethylamine N-oxide and mortality risk in patients with peripheral artery disease. J. Am. Heart Assoc. 5:e004237. doi: 10.1161/JAHA.116.004237
Sessa, F., Anna, V., Messina, G., Cibelli, G., Monda, V., Marsala, G., et al. (2018). Heart rate variability as predictive factor for sudden cardiac death. Aging 10, 166–177. doi: 10.18632/aging.101386
Shah, S. R., Abbasi, Z., Fatima, M., Ochani, R. K., Shahnawaz, W., Asim Khan, M., et al. (2018). Canakinumab and cardiovascular outcomes: results of the CANTOS trial. J. Community Hosp. Intern. Med. Perspect. 8, 21–22. doi: 10.1080/20009666.2018.1428023
Sharif, K., Watad, A., Bragazzi, N. L., Lichtbroun, M., Amital, H., and Shoenfeld, Y. (2018). Physical activity and autoimmune diseases: get moving and manage the disease. Autoimmun. Rev. 17, 53–72. doi: 10.1016/j.autrev.2017.11.010
Sheikine, Y. A., and Hansson, G. K. (2006). Chemokines as potential therapeutic targets in atherosclerosis. Curr. Drug Targets 7, 13–27. doi: 10.2174/138945006775270240
Shephard, R. J., and Shek, P. N. (1994). Potential impact of physical activity and sport on the immune system–a brief review. Br. J. Sports Med. 28, 247–255. doi: 10.1136/BJSM.28.4.247
Skeoch, S., and Bruce, I. N. (2015). Atherosclerosis in rheumatoid arthritis: is it all about inflammation? Nat. Rev. Rheumatol. 11, 390–400. doi: 10.1038/nrrheum.2015.40
Slingerland, A. E., Schwabkey, Z., Wiesnoski, D. H., and Jenq, R. R. (2017). Clinical evidence for the microbiome in inflammatory diseases. Front. Immunol. 8:400. doi: 10.3389/fimmu.2017.00400
Slusher, A. L., Zúñiga, T. M., and Acevedo, E. O. (2017). Maximal exercise alters the inflammatory phenotype and response of mononuclear cells. Med. Sci. Sport Exerc. 1, doi: 10.1249/MSS.0000000000001480
Smith, J. K., Dykes, R., Douglas, J. E., Krishnaswamy, G., and Berk, S. (1999). Long-term exercise and atherogenic activity of blood mononuclear cells in persons at risk of developing ischemic heart disease. JAMA 281, 1722–1727. doi: 10.1001/jama.281.18.1722
Soares-Miranda, L., Siscovick, D. S., Psaty, B. M., Longstreth, W. T., Mozaffarian, D., and Mozaffarian, D. (2016). Physical activity and risk of coronary heart disease and stroke in older adults: the cardiovascular health study. Circulation 133, 147–155. doi: 10.1161/CIRCULATIONAHA.115.018323
Steppich, B., Dayyani, F., Gruber, R., Lorenz, R., Mack, M., and Ziegler-Heitbrock, H. W. L. (2000). Selective mobilization of CD14(+)CD16(+) monocytes by exercise. Am. J. Physiol. Physiol. 279, C578–C586. doi: 10.1152/ajpcell.2000.279.3.C578
Strömberg, A., Olsson, K., Dijksterhuis, J. P., Rullman, E., Schulte, G., and Gustafsson, T. (2016). CX3CL1–a macrophage chemoattractant induced by a single bout of exercise in human skeletal muscle. Am. J. Physiol. Integr. Comp. Physiol. 310, R297–R304. doi: 10.1152/ajpregu.00236.2015
Struyf, S., van Coillie, E., Paemen, L., Put, W., Lenaerts, J.-P., Proost, P., et al. (1998). Synergistic induction of MCP-1 and -2 by IL-1β and interferons in fibroblasts and epithelial cells. J. Leukoc. Biol. 63, 364–372. doi: 10.1002/jlb.63.3.364
Taylor, F., Ward, K., Moore, T. H., Burke, M., Davey Smith, G., Casas, J.-P., et al. (2011). Statins for the primary prevention of cardiovascular disease. Cochrane Database Syst. Rev. CD004816. doi: 10.1002/14651858.CD004816.pub4
Tegos, T. J., Kalodiki, E., Sabetai, M. M., and Nicolaides, A. N. (2001). The genesis of atherosclerosis and risk factors: a review. Angiology 52, 89–98.
Turner, M. D., Nedjai, B., Hurst, T., and Pennington, D. J. (2014). Cytokines and chemokines: at the crossroads of cell signalling and inflammatory disease. Biochim. Biophys. Acta 1843, 2563–2582. doi: 10.1016/J.BBAMCR.2014.05.014
Upadhya, S., Mooteri, S., Peckham, N., and Pai, R. G. (2004). Atherogenic effect of interleukin-2 and antiatherogenic effect of interleukin-2 antibody in apo-E-deficient mice. Angiology 55, 289–294. doi: 10.1177/000331970405500308
Valero-Elizondo, J., Salami, J. A., Osondu, C. U., Ogunmoroti, O., Arrieta, A., Spatz, E. S., et al. (2016). Economic impact of moderate-vigorous physical activity among those with and without established cardiovascular disease: 2012 medical expenditure panel survey. J. Am. Heart Assoc. 5:e003614. doi: 10.1161/JAHA.116.003614
van den Elsen, L. W., Poyntz, H. C., Weyrich, L. S., Young, W., and Forbes-Blom, E. E. (2017). Embracing the gut microbiota: the new frontier for inflammatory and infectious diseases. Clin. Transl. Immunol. 6:e125. doi: 10.1038/cti.2016.91
van Hall, G., Steensberg, A., Sacchetti, M., Fischer, C., Keller, C., Schjerling, P., et al. (2003). Interleukin-6 stimulates lipolysis and fat oxidation in humans. J. Clin. Endocrinol. Metab. 88, 3005–3010. doi: 10.1210/jc.2002-021687
Veillard, N. R., Kwak, B., Pelli, G., Mulhaupt, F., James, R. W., Proudfoot, A. E. I., et al. (2004). Antagonism of RANTES receptors reduces atherosclerotic plaque formation in mice. Circ. Res. 94, 253–261. doi: 10.1161/01.RES.0000109793.17591.4E
Vella, C. A., Allison, M. A., Cushman, M., Jenny, N. S., Miles, M. P., Larsen, B., et al. (2017). Physical activity and adiposity-related inflammation: the MESA. Med. Sci. Sports Exerc. 49, 915–921. doi: 10.1249/MSS.0000000000001179
Voloshyna, I., Littlefield, M. J., and Reiss, A. B. (2014). Atherosclerosis and interferon-γ: new insights and therapeutic targets. Trends Cardiovasc. Med. 24, 45–51. doi: 10.1016/j.tcm.2013.06.003
Wainstein, M. V., Mossmann, M., Araujo, G. N., Gonçalves, S. C., Gravina, G. L., Sangalli, M., et al. (2017). Elevated serum interleukin-6 is predictive of coronary artery disease in intermediate risk overweight patients referred for coronary angiography. Diabetol. Metab. Syndr. 9:67. doi: 10.1186/s13098-017-0266-5
Waters, D. D., and Hsue, P. Y. (2017). PCSK9 inhibition to reduce cardiovascular risk: tempering expectations. Circ. Res. 120, 1537–1539. doi: 10.1161/CIRCRESAHA.117.311015
Weinhold, M., Shimabukuro-Vornhagen, A., Franke, A., Theurich, S., Wahl, P., Hallek, M., et al. (2016). Physical exercise modulates the homeostasis of human regulatory T cells. J. Allergy Clin. Immunol 137, 1607.e8–1610.e8. doi: 10.1016/j.jaci.2015.10.035
Whelton, P. K., Carey, R. M., Aronow, W. S., Casey, D. E., Collins, K. J., Dennison Himmelfarb, C., et al. (2018). 2017 ACC/AHA/AAPA/ABC/ACPM/AGS/APhA/ASH/ASPC/NMA/PCNA guideline for the prevention, detection, evaluation, and management of high blood pressure in adults: executive summary: a report of the American College of Cardiology/American Heart Association Task Force on Clinical Practice Guidelines. 71, 1269–1324. doi: 10.1016/j.jacc.2017.11.006
Yakeu, G., Butcher, L., Isa, S., Webb, R., Roberts, A. W., Thomas, A. W., et al. (2010). Low-intensity exercise enhances expression of markers of alternative activation in circulating leukocytes: roles of PPARγ and Th2 cytokines. Atherosclerosis 212, 668–673. doi: 10.1016/j.atherosclerosis.2010.07.002
Zamani, A., Salehi, I., and Alahgholi-Hajibehzad, M. (2017). Moderate exercise enhances the production of interferon-γ and interleukin-12 in peripheral blood mononuclear cells. Immune Netw. 17, 186–191. doi: 10.4110/in.2017.17.3.186
Keywords: atherosclerosis, inflammation, microbiome, cardiovascular disease, physical activity
Citation: Fernandez DM, Clemente JC and Giannarelli C (2018) Physical Activity, Immune System, and the Microbiome in Cardiovascular Disease. Front. Physiol. 9:763. doi: 10.3389/fphys.2018.00763
Received: 22 March 2018; Accepted: 30 May 2018;
Published: 29 June 2018.
Edited by:
Vincent Pialoux, Claude Bernard University Lyon 1, FranceReviewed by:
Giovanni Messina, University of Foggia, ItalyBeat Knechtle, University Hospital Zürich, Switzerland
Copyright © 2018 Fernandez, Clemente and Giannarelli. This is an open-access article distributed under the terms of the Creative Commons Attribution License (CC BY). The use, distribution or reproduction in other forums is permitted, provided the original author(s) and the copyright owner(s) are credited and that the original publication in this journal is cited, in accordance with accepted academic practice. No use, distribution or reproduction is permitted which does not comply with these terms.
*Correspondence: Chiara Giannarelli, Chiara.Giannarelli@mssm.edu