- 1College of Plant Science, Jilin University, Changchun, China
- 2Department of Entomology, China Agricultural University, Beijing, China
Uridine diphosphate (UDP)-glycosyltransferases (UGTs) are major phase II enzymes that conjugate a variety of small lipophilic molecules with UDP sugars and alter them into more water-soluble metabolites. Therefore, glucosidation plays a major role in the inactivation and excretion of a great variety of both endogenous and exogenous compounds. In this study, two inhibitors of UGT enzymes, sulfinpyrazone and 5-nitrouracil, significantly increased the toxicity of thiamethoxam against the resistant strain of Aphis gossypii, which indicates that UGTs are involved in thiamethoxam resistance in the cotton aphid. Based on transcriptome data, 31 A. gossypii UGTs belonging to 11 families (UGT329, UGT330, UGT341, UGT342, UGT343, UGT344, UGT345, UGT348, UGT349, UGT350, and UGT351) were identified. Compared with the thiamethoxam-susceptible strain, the transcripts of 23 UGTs were elevated, and the transcripts of 13 UGTs (UGT344J2, UGT348A2, UGT344D4, UGT341A4, UGT343B2, UGT342B2, UGT350C3, UGT344N2, UGT344A14, UGT344B4, UGT351A4, UGT344A11, and UGT349A2) were increased by approximately 2.0-fold in the resistant cotton aphid. The suppression of selected UGTs significantly increased the insensitivity of resistant aphids to thiamethoxam, suggesting that the up-regulated UGTs might be associated with thiamethoxam tolerance. This study provides an overall view of the possible metabolic factor UGTs that are relevant to the development of insecticide resistance. The results might facilitate further work to validate the roles of these UGTs in thiamethoxam resistance.
Introduction
Uridine diphosphate (UDP)-glycosyltransferases (UGTs, EC 2.4.1.17) catalyze the conjugation of a range of small lipophilic compounds with sugars to produce glycosides, which are soluble in water and can be efficiently excreted (Mackenzie et al., 1997). The protein structure of UGTs is divided into two main parts: the aglycone substrate-binding domain at the N-terminus and the UDP sugar donor-binding domain at the C-terminus (Magdalou et al., 2010). Glycoside conjugation is one of the most important metabolic pathways for the biotransformation of a number of lipophilic endogenous and exogenous compounds of xenobiotics and endobiotics (Bock, 2003, 2016; Bowles et al., 2005). Therefore, the glycosylation of toxins by UGTs is a particularly important detoxification mechanism (Heckel, 2014; Heidel-Fischer and Vogel, 2015). Long-term evolution has led to the development of sophisticated detoxification systems that allow organisms to resist various harmful substances occurring in the external environment. Insects use UDP-glucose as an activated sugar donor that is then transferred to UGTs, which are anchored in the endoplasmic reticulum (ER) (Ahn et al., 2012). Previous studies have indicated the involvement of insect UGTs in the detoxification of plant secondary xenobiotics in Manduca sexta (Ahmad and Hopkins, 1992), Helicoverpa assulta (Ahn et al., 2011a,b), Spodoptera littoralis (Wouters et al., 2014), Helicoverpa armigera, and Heliothis virescens (Krempl et al., 2016). In addition to plant secondary xenobiotic tolerance, insect UGTs might be involved in insecticide detoxification. Recent studies have demonstrated that the overexpression of UGT2B17 (renamed UGT33AA4) is associated with chlorantraniliprole resistance in Plutella xylostella (Li et al., 2017a,b), and many studies have reported on cytochrome P450 monooxygenase-mediated insecticides resistance (Scott, 2008; Feyereisen, 2015). However, few studies have described the involvement of UGTs in the detoxification of insecticide resistance.
The cotton aphid, Aphis gossypii Glover (Hemiptera: Aphididae), is one of the most economically important insect pests in agriculture and has developed different levels of resistance to broad-spectrum insecticides, including organophosphates, pyrethroids, carbamates, and neonicotinoids (Denholm and Rowland, 1992; Shang et al., 2012; Chen et al., 2017). Thiamethoxam, a second-generation neonicotinoid insecticide that irreversibly binds to the nicotinic acetylcholine receptors (nAChR) of cells in the nervous system and interferes with the transmission of nerve impulses in insects (Casida and Durkin, 2013), and is effective for controlling resistant A. gossypii (Elbert et al., 2008). Research studies have indicated that enhanced detoxification caused by P450 gene overexpression accounts for neonicotinoids in Bemisia tabaci, Myzus persicae, and Nilaparvata lugens (Karunker et al., 2008, 2009; Puinean et al., 2010; Bao et al., 2016; Zhang et al., 2016). Consistent with these reports, our previous synergism analysis demonstrated that P450s are also involved in thiamethoxam resistance in A. gossypii (Wei et al., 2017). Whether UGTs are involved in insecticide resistance as well as P450-mediated resistance in A. gossypii has not been determined. The results of a synergism study illustrate that UGTs might be involved in the resistance present in thiamethoxam-resistant A. gossypii.
In this study, to clarify the potent roles of UGTs in thiamethoxam resistance in cotton aphids, (1) the UGT genes in the A. gossypii transcriptome were identified, and the phylogenetic relationships between these genes and their homologs in two other insects were analyzed; (2) the expression profiles of these UGTs in thiamethoxam-susceptible and thiamethoxam-resistant strains were analyzed by quantitative real-time polymerase chain reaction (qRT-PCR); and (3) the involvement of overexpressed UGTs in resistance was functionally tested by RNA interference (RNAi). Our data provide preliminary insights into the dynamic changes in the gene expression of UGTs and their involvement in thiamethoxam resistance. The results might facilitate further study of the functions in UGTs in the insecticide resistance of A. gossypii.
Materials and Methods
Insects
Two cotton aphid (A. gossypii) strains were used in this study. One strain was resistant to thiamethoxam (ThR), and the other was susceptible to thiamethoxam (SS) (Pan et al., 2015). The SS strain was collected in 2008 from Jilin Province of China, where limited insecticides have been applied. The aphid species has been maintained without any insecticide treatment since its collection. The ThR strain was established from the SS population via consecutive selection with increased concentrations of thiamethoxam (LC30) via the leaf-dipping method. Both the resistant and susceptible strains were reared on cotton plants [Gossypium hirsutum (L.)] in the laboratory at 20–23°C with a photoperiod of 16:8 h (light:dark).
Chemicals
Sulfinpyrazone (Sul) and 5-nitrouracil (5-Nul) were obtained from Sigma-Aldrich (St. Louis, MO, USA). Thiamethoxam (25% WDG) was purchased from Syngenta (Switzerland). The PrimeScriptTM First-Strand cDNA Synthesis kit, SYBR® Premix Ex Taq™ II (Tli RNaseH Plus), oligo(dT)18, Ex Taq DNA polymerase, RNase-free DNase I, RNase Inhibitor, DNA Marker DL2000, and agarose were purchased from TaKaRa (Dalian, China). The pGEM-T vector and the T7 RiboMAX™ Express RNAi System were purchased from Promega (USA). All the reagents were of the highest purity available.
Bioassays
The synergistic effects of two UGT inhibitors, 5-nitrouracil (5-Nul) and sulfinpyrazone (Sul), on the toxicity of thiamethoxam to the SS and ThR strains were tested using a leaf dipping method, as described by Peng et al. (2016a) and Wei et al. (2017) with some modifications. The maximum sublethal doses of 5-Nul and Sul for the SS strain were determined using the bioassay method described by Wei et al. (2017). 5-Nul and Sul were used to prepare a series of concentrations (six or seven concentrations) with distilled water containing 0.05% (v/v) Triton X-100. The leaves were dipped for 15 s in the required concentration of insecticide or into 0.05% (v/v) Triton X-100 water (as the control treatment) and then placed in the shade and allowed to air dry. Bioassays were conducted by transferring at least 30 apterous adult aphids onto the treated cotton leaves obtained from each whole seedling. The bioassay samples were maintained in the laboratory at 20–23°C with a photoperiod of 16:8 h (light:dark). Three replicates were performed for each concentration, and the mortality was assessed after 3 days. The maximum dose that led to zero mortality in the SS strain was adopted as the maximum sublethal concentration in our study, and the maximum sublethal concentrations of 5-Nul and Sul were 400 mg/L. For synergism bioassays, apterous adult aphids were exposed to cotton leaves that were treated with the mixture of thiamethoxam with 5-Nul or Sul (final concentration of 400 mg/L). Three replicates were performed for each concentration, and the mortality was assessed after 3 days and used to estimate the synergistic effects of 5-Nul or Sul with thiamethoxam in both strains. The synergistic ratio was calculated using the conventional approach, which divides the LC50 without the synergist by the LC50 with the synergist. A probit analysis was conducted using POLO software (LeOra Software Inc., Berkeley, CA, USA).
Nomenclature and Phylogenetic Analysis
The UGT sequences were derived from the transcriptome (the clean reads have been submitted to and are available from the National Center for Biotechnology Information (NCBI)/SRA database with SRA experiment accession number SRX683625) (Pan et al., 2015). These UGT sequences were named by the UGT Nomenclature Committee guidelines (Dr. Michael H. Court, Department of Veterinary Clinical Sciences, Washington State University, USA) using the following criteria: the gene symbol UGT, a family number, a subfamily letter, and an individual gene number. UGT families are defined at 40% amino acid sequence identity, and subfamilies are defined at 60% amino acid identity or greater (Mackenzie et al., 1997, 2005). Names were assigned to the A. gossypii sequences on this basis. The UGT predicted protein sequences from H. armigera and Bombyx mori were extracted from the UGT Nomenclature Committee (http://prime.vetmed.wsu.edu/resources/udp-glucuronsyltransferase-homepage)and analyzed with the A. gossypii UGTs via ClustalW alignment using MEGA 7 software (http://www.megasoftware.net/). The alignment results were used to build a consensus phylogenetic tree using the neighbor-joining method. Pairwise and multiple alignments were performed with a gap opening penalty of 10 and a gap extension penalty left of 0.2. A total of 1,000 bootstrap replications were performed, and branches with bootstrap values above 50% are indicated.
Protein Structure Prediction
Multiple alignments of 10 overexpressed representative protein sequences from the A. gossypii UGTs were obtained via ClustalW, and the structural domains, such as the UGT signature motif, were detected by comparison with other sequences for which primary structures have been characterized. The signal peptides were predicted by SignalP 4.1 on the CBS Prediction Servers (http://www.cbs.dtu.dk/services/SignalP/). The C-terminal transmembrane domain was searched using TMHMM2.0 (http://www.cbs.dtu.dk/services/TMHMM).
Total RNA Isolation and cDNA Synthesis
The total RNA from apterous adult aphids was extracted using TRIzol (Invitrogen, USA) according to the manufacturer's instructions and then treated with RNase-free DNase I (TaKaRa, Japan). The RNA samples were quantified by measuring the absorbance at 260 nm, and the quality was assessed via agarose gel electrophoresis. First-strand cDNA was synthesized from the total RNA using a PrimeScriptTM First-Strand cDNA Synthesis kit (TaKaRa, Japan) with oligo (dT)18 as a primer.
Quantitative Real-Time PCR and Data Analysis
Quantitative real-time PCR was performed on an ABI 7500 system (Applied Biosystems) using SYBR® Premix Ex Taq™ II (Tli RNaseH Plus; TaKaRa, Japan) (Wei et al., 2017). Gene-specific primers for real-time PCR (Supplementary Table 1) were designed based on the UGT sequences (Supplementary Data 1) and synthesized by Sangon Biotech Co., Ltd. (Shanghai, China). The thermal cycling protocol included an initial denaturation at 95°C for 30 s followed by 40 cycles of 95°C for 5 s and 60°C for 34 s. The fluorescence signal was measured at the end of each extension step at 60°C. After the amplification, a dissociation step consisting of 95°C for 15 s, 60°C for 1 min and 95°C for 15 s was performed to confirm that only specific products were amplified. The glyceraldehyde-3-phosphate dehydrogenase (GAPDH) and elongation factor 1-alpha (EF1a) were used as internal reference genes for A. gossypii (Peng et al., 2016a,b). Relative gene expression was calculated using the 2−ΔΔCT method (Pfaffl, 2001). The experiment was independently performed three times for each strain. Significant differences were analyzed using GraphPad InStat3 statistical software (GraphPad Software, 2000, http://www.apponic.com/publisher/graphpad-software-18307/top-downloads/).
Rearing on Artificial Diet and dsRNA Feeding
The dsRNA design and synthesis methods were previously described by Peng et al. (2016a). Based on the UGT sequences (Supplementary Data 1) and the possible interference sites predicted with online prediction software (http://www.dkfz.de/signaling/e-rnai3/), we designed specific primers using DNAMAN 6.0 software (http://www.lynnon.com/dnaman.html). The gene fragments were amplified from cDNA and cloned into pGEM-T (Promega, USA). The purified plasmids served as templates for RNA synthesis using the T7 RiboMAX™ Express RNAi System (Promega, USA). ECFP dsRNA was used as the control and synthesized under the same conditions as the primers (Supplementary Table 1). The artificial diet and the rearing method used in this study were previously reported by Peng et al. (2016a,b). The diet was prepared in DEPC-treated water to ensure the absence of RNase activity. For the dsRNA feeding experiments, dsRNA was added to the artificial diet at a concentration of 100 ng/μL. An artificial diet containing dsRNA-ECFP was used as a control. Sixty adult apterous thiamethoxam-resistant A. gossypii were transferred onto the artificial diet for rearing. To analyze the efficiency of dsRNA knockdown on UGT expression, the aphids were fed an artificial diet containing dsRNA (100 ng/μL) for 48 h and then subjected to RT-qPCR. To assess the sensitivity of the cotton aphids to thiamethoxam after RNAi of UGT, 80 resistant adult aphids were transferred to the artificial diet containing thiamethoxam (1.0 mg/L) mixed with dsRNA-UGT (100 ng/μL), and dsRNA-ECFP was used as the control. The mortality of the cotton aphids was recorded after 48 h. Each treatment included three replicates (80 aphids were used in each replication).
Results
Thiamethoxam Toxicity and Synergism Bioassays
The probit analyses of thiamethoxam toxicity and synergism bioassays of A. gossypii are summarized in Table 1. 5-Nul and Sul increased the thiamethoxam toxicity in the ThR strain by 9.38- and 10.31-fold, respectively. These results indicate that UDP-glucuronosyltransferases are involved in thiamethoxam resistance in the ThR strain at the observed resistance state.
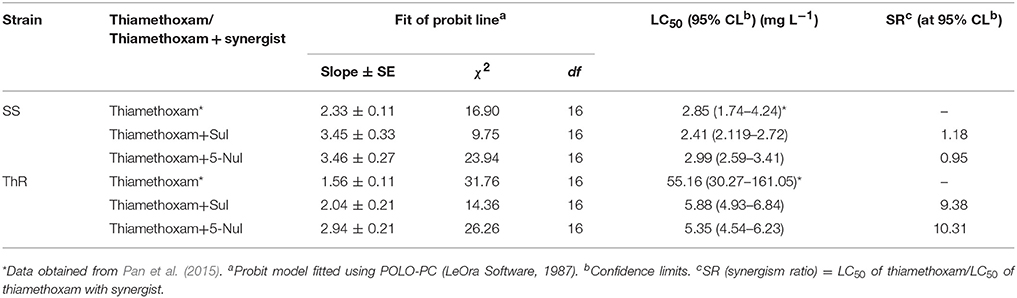
Table 1. Synergistic effects of Sul and 5-Nul on the toxicity of thiamethoxam in the SS and ThR strains.
Identification and Phylogenetic Analysis of the A. gossypii UGTs
Based on the transcriptome data from A. gossypii, the full lengths of 31 UGT genes were identified, and these UGT genes were named by the UGT Nomenclature Committee (Dr. Michael H. Court, Department of Veterinary Clinical Sciences, Washington State University, USA). The GenBank accession numbers are listed in Supplementary Data 1. To construct a phylogenetic tree (Figure 1), a total of 83 UGT gene sequences of H. armigera and B. mori from the UGT Nomenclature Committee (Supplementary Data 1) and 31 UGT gene sequences from our cotton aphid transcriptome were used in the phylogenetic tree. ClustalW alignments performed using MEGA 7 software (http://www.megasoftware.net/) were used to align the amino acid sequences, and the neighbor-joining method with 1,000 bootstrap replicates was used to construct the phylogenetic trees.
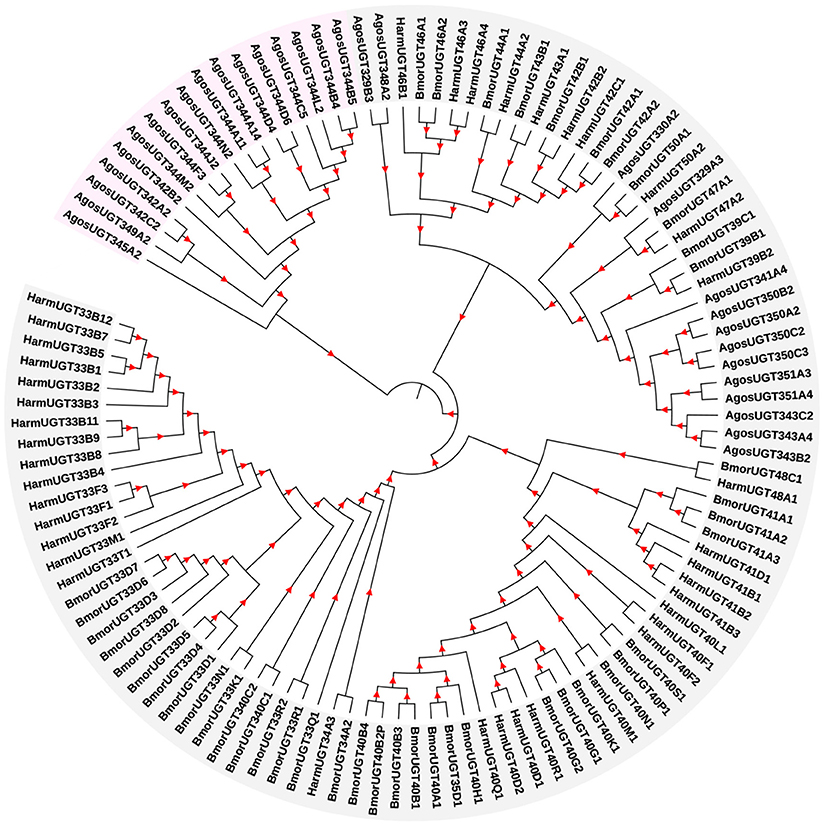
Figure 1. Phylogenetic analysis of A. gossypii UGT genes with other orthologs. The phylogram was generated using the maximum likelihood method in MEGA 7, and bootstrap values were calculated based on 1,000 replicates with a cutoff of <50%. The sequences used for constructing the tree and their corresponding GenBank accession numbers are listed in Supplementary Data 1.
The 31 A. gossypii UGTs were distributed into 11 families, namely, the UGT344 (12 UGTs), UGT350 (4), UGT342 (3), UGT343 (3), UGT329 (2), UGT351 (2), UGT330 (1), UGT341 (1), UGT345 (1), UGT348 (1), and UGT349 (1) families. The number of UGT genes in A. gossypii (31 UGTs) was less than that in H. armigera and B. mori, and this finding is related to the lack of a complete genome sequence for A. gossypii.
Structural Motifs of the A. gossypii UGT Proteins
Multiple alignments of eight representative A. gossypii UGT amino acid sequences revealed two major domains: the highly variable N-terminal substrate-binding domain and the conserved C-terminal sugar donor-binding domain (Figure 2) (Ahn et al., 2012). All A. gossypii UGTs consisted of a different length amino acid signal peptide found at the N-terminal end, which is presumably cleaved after integration into the ER compartment. The two predicted sugar donor-binding regions (DBR1 and DBR2), important residues interacting with the sugar donor, and catalytic residues were conserved. The UGT motif signature sequences [also called the plant secondary product glycosyltransferase (PSPG) motif in plants], (FVA)-(LIVMF)-(TS)-(HQ)-(SGAC)-G-X(2)-(STG)-X(2)-(DE)-X(6)-P-(LIVMFA)-(LIVMFA)-X(2)-P-(LMVFIQ)-X(2)-(DE)-Q (where X is any amino acid), was found in the middle of the C-terminal domain, which shows higher conservation (Mackenzie et al., 1997; Ahn et al., 2012). The alignment data suggested that most A. gossypii UGTs were active proteins.
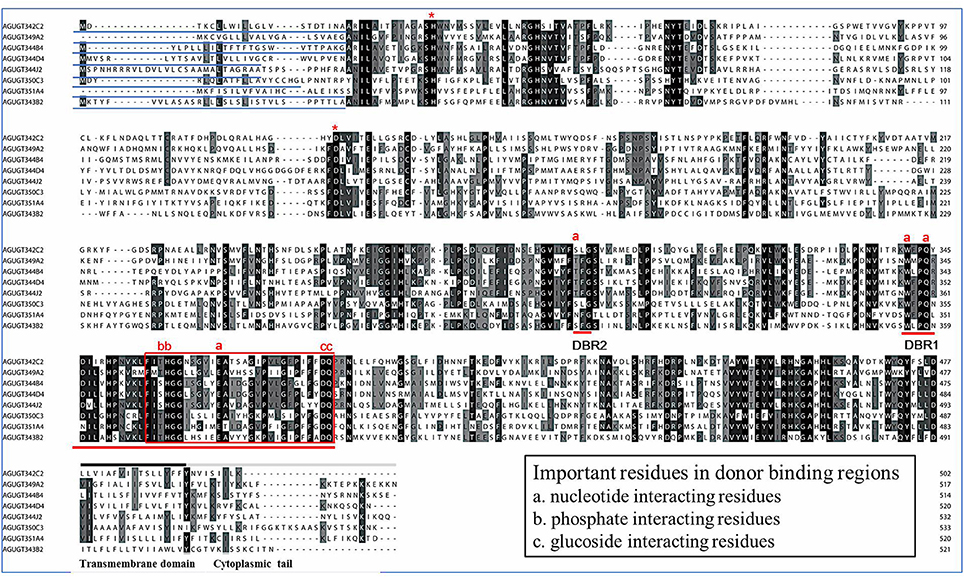
Figure 2. Alignment of the amino acid sequences of eight UGT genes from A. gossypii. The predicted signal peptides are underlined, and the UGT signature motif is boxed. The transmembrane domains and cytoplasmic tails in the C-terminus are underlined by black and gray lines above the alignment, respectively. The conserved catalytic residues, H and D, are indicated by * above the alignment. DBR refers to the donor-binding region, and several important residues interacting with the sugar donor are indicated (a, b, or c) above the alignment.
Expression Profiling of A. gossypii UGT Genes in the Resistant and Susceptible Strains
The quantitative real-time PCR results indicated that the transcripts of 23 UGT genes of the 27 determinate genes were elevated, and the transcripts of 13 UGT genes were increased by approximately 2.0-fold or greater in the thiamethoxam-resistant cotton aphid compared with the susceptible aphids. Specifically, the mRNA levels of UGT344J2, UGT348A2, UGT344D4, UGT341A4, UGT343B2, UGT342B2, UGT350C3, UGT344N2, UGT344A14, UGT344B4, UGT351A4, UGT344A11, and UGT349A2 were increased to 4.96, 3.95, 3.64, 2.98, 2.54, 2.22, 2.14, 2.14, 2.18, 2.05, 2.00, 1.96, and 1.94-fold, respectively. In contrast, the transcripts of UGT344D6, UGT329A3, UGT344B5, and UGT351A3 were down-regulated in the ThR strain compared with the SS strain, and the UGT351A3 level was decreased by 0.29-fold (Figure 3).
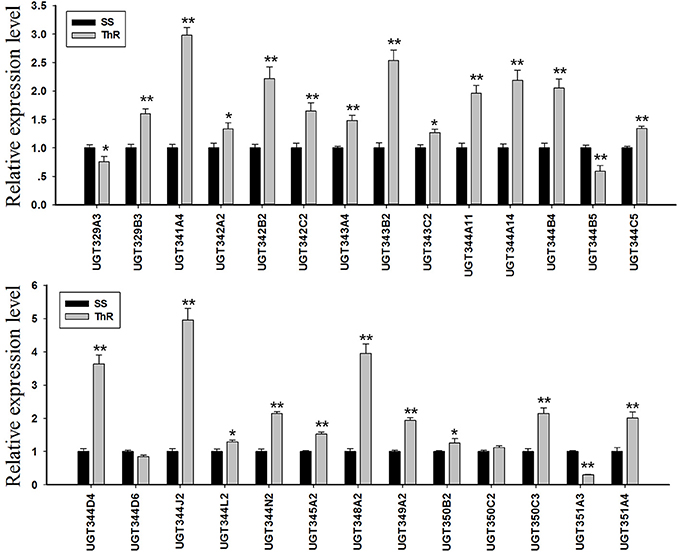
Figure 3. Transcription levels of UGTs in the SS and SR strains determined by real-time PCR. GAPDH and EF1a were used as internal reference genes. The error bars indicate 95% confidence intervals (n = 3). *P < 0.05 difference, as determined by Student's t-test. **P < 0.01 difference, as determined by Student's t-test.
Suppression of UGT Transcripts Increases Thiamethoxam Toxicity
An orally delivered dsRNA method for RNAi was performed to elucidate the relationship between the overexpression of massive UGT genes and thiamethoxam resistance. Because many UGT genes were up-regulated in the ThR strain, we chose five UGT genes for the RNAi experiment. Under the RNAi treatments, the expression levels of UGT342C2, UGT344B4, UGT344J2, UGT348A2, and UGT349A2 were reduced to 0.65-, 0.72-, 0.67-, 0.65-, 0.69-, and 0.86-fold in the corresponding dsRNA-UGT-treated (100 ng/μL) aphids after 48 h of treatment compared with the control expression levels (Figure 4). The mortality increased from 50.46% in the control to 55.03, 60.74, 57.66, 61.79, 52.38, and 63.27% in the aphids fed dsRNA-UGT342C2, dsRNA-UGT344B4, dsRNA-UGT344J2, dsRNA-UGT348A2, dsRNA-UGT349A2, and dsRNA-Mix (the ratio of six dsRNA-UGT is 1:1:1:1:1:1:1) under the 1.0 mg/L thiamethoxam treatments, respectively (Figure 5).

Figure 4. dsRNA-mediated suppression of UGT transcripts in A. gossypii. dsRNA-mediated suppression of UGT342C2, UGT344B4, UGT344J2, UGT348A2, and UGT349A2 transcripts in resistant aphids fed an artificial diet with the corresponding dsRNA (100 ng/μL). The error bars indicate 95% confidence intervals (n = 3). *P < 0.05 difference, as determined by Student's t-test. **P < 0.01 difference, as determined by Student's t-test.
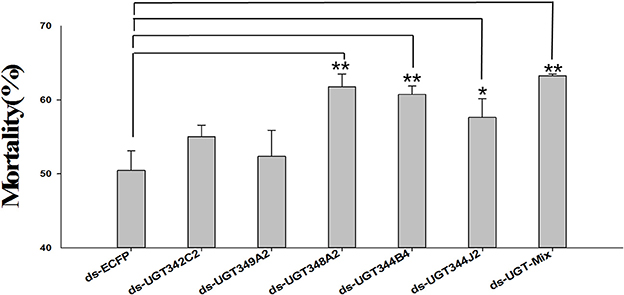
Figure 5. Effects of the knockdown UGT on thiamethoxam toxicity in A. gossypii. Mean mortality ± SE (n = 3) of resistant cotton aphids that were fed thiamethoxam (1.0 mg/L) and a dsRNA-UGT (100 ng/μL) for 48 h. Each treatment included three replicates, and 80 adults of the resistant aphid strain were used in each replication. The error bars indicate 95% confidence intervals (n = 3). *P < 0.05 difference, as determined by Student's t-test. **P < 0.01 difference, as determined by Student's t-test.
Discussion
Due to the extensive use of the neonicotinoid insecticide imidacloprid for controlling cotton aphids in the field, the resistance to imidacloprid ranged from 1.48 to >1,200-fold among different A. gossypii populations collected from various Bt cotton planting areas in China in 2014, and the LC50-value of imidacloprid was >5,000 mg/L in the population from Yuncheng of Shanxi Province (Chen et al., 2017). Thiamethoxam has been used as an alternative neonicotinoid insecticide for the control of cotton aphids. Our previous synergism assay showed that phase I enzyme P450s (acting directly on the toxin molecule) are involved in thiamethoxam resistance (Wei et al., 2017). In addition to P450-mediated detoxification resistance, the roles of the phase II enzyme UGTs (which conjugate endogenous molecules to the toxins) in thiamethoxam resistance remains unknown. To clarify the roles of UGTs in thiamethoxam tolerance, the UGT inhibitors 5-nitrouracil and sulfinpyrazone were used in a synergism assay, and the results illustrated that these two UGT inhibitors significantly increased thiamethoxam toxicity in the ThR strain (Table 1), suggesting that UGTs, in addition to P450, are involved in thiamethoxam resistance. Therefore, glycosylation by UGTs might play an important role in the detoxification of xenobiotics in the cotton aphid. Notably, information regarding UGTs in the cotton aphid has not been available until now.
In this study, 31 UGT genes were identified from the transcriptome data of A. gossypii (Pan et al., 2015). The A. gossypii UGTs were distributed into 11 families: UGT329, UGT330, UGT341, UGT342, UGT343, UGT344, UGT345, UGT348, UGT349, UGT350, and UGT351 (Figure 1). The fewer number of UGT genes identified in A. gossypii (31 UGTs) compared with those found in H. armigera and B. mori might be due to the lack of a complete genome sequence for A. gossypii. The UGT protein structure is divided into two main parts: the aglycone substrate-binding domain in the N-terminus and the UDP sugar donor-binding domain in the C-terminus (Meech and Mackenzie, 1997; Meech et al., 2012). Alignments of the A. gossypii UGT amino acid sequences showed conserved domains, including the sugar donor-binding region (DBR1 and DBR2), important residues interacting with the sugar donor and catalytic residues, and the UGT motif signature sequences [(FVA)-(LIVMF)-(TS)-(HQ)-(SGAC)-G-X(2)-(STG)-X(2)-(DE)-X(6)-P-(LIVMFA)-(LIVMFA)-X(2)-P-(LMVFIQ)-X(2)-(DE)-Q] (Figure 2) (Mackenzie et al., 1997; Ahn et al., 2012). These findings suggested that A. gossypii UGTs were likely active proteins, which is similar to observations in mammals. Insect UGTs are capable of detoxifying plant secondary compounds. For example, the stereoselective reglucosylation of benzoxazinoid by UGT represents a detoxification strategy in S. littoralis (Wouters et al., 2014). UGTs are capable of glycosylating gossypol primarily to the diglycosylated gossypol isomer 5, which is a crucial step in gossypol detoxification in H. armigera (Krempl et al., 2016). Insect UGTs mediate plant xenobiotic tolerance and have also been reported to be involved in insecticide resistance. The ingestion of dsRNA, which successfully silences overexpressed UGTs, significantly increases the susceptibility of resistant Leptinotarsa decemlineata to imidacloprid (Kaplanoglu et al., 2017). In P. xylostella, the overexpression of UGTs is associated with chlorantraniliprole resistance (Li et al., 2017a,b). In this study, a screening of the expression profile of UGTs revealed that the expression of 13 UGT genes was increased by nearly 2-fold or more in the ThR strain compared with the SS strain (Figure 3), suggesting that multiple up-regulated UGTs might be associated with thiamethoxam resistance. To verify the influence of UGT gene overexpression on the susceptibility to thiamethoxam in A. gossypii, we performed RNAi via a dsRNA oral feeding method (Peng et al., 2016a,b) and found that the RNAi of the overexpressed UGT genes could have resulted in increased thiamethoxam susceptibility in the resistant cotton aphids (Figure 5). This result further confirmed that enzymes encoded by these overexpressed UGTs might contribute to the detoxification of thiamethoxam by glycosylation in A. gossypii. In conclusion, this study provides insights into the potential roles of UGTs in thiamethoxam resistance. These results should be useful for understanding thiamethoxam resistance mechanisms.
Author Contributions
All the authors listed have made a substantial, direct and intellectual contribution to the work and have approved its publication. YP and FT: designed and performed most of the experiments. XW and YW: performed the RNAi assays. XG and JX: revised the manuscript. QS: designed the experiments and wrote the manuscript.
Conflict of Interest Statement
The authors declare that the research was conducted in the absence of any commercial or financial relationships that could be construed as a potential conflict of interest.
Acknowledgments
This work was sponsored by the National Natural Science Foundation of China (31772188, 31301728). The authors thank Prof. Michael H. Court of Washington State University for his kind help in renaming the UGTs identified in this study on behalf of the UGT nomenclature committee.
Supplementary Material
The Supplementary Material for this article can be found online at: https://www.frontiersin.org/articles/10.3389/fphys.2018.00322/full#supplementary-material
Supplementary Table 1. Primers used in the experiments. dsRNA, double-stranded RNA; EF1a, elongation factor 1-alpha; F, forward; GAPDH, glyceraldehyde-3-phosphate dehydrogenase; ORF, open reading frame; R, reverse. The lowercase letters indicate the T7 RNA polymerase promoter.
Supplementary Data 1. Gene sequences of A. gossypii UGTs and other insect orthologs.
References
Ahmad, S. A., and Hopkins, T. L. (1992). Phenol b-glucosyltransferase and b-glucosidase activities in the tobacco hornworm larva Manduca sexta (L.): properties and tissue localization. Arch. Insect Biochem. Physiol. 21, 207–224. doi: 10.1002/arch.940210305
Ahn, S. J., Badenes-Pérez, F. R., and Heckel, D. G. (2011a). A host-plant specialist, Helicoverpa assulta, is more tolerant to capsaicin from Capsicum annuum than other noctuid species. J. Insect Physiol. 57, 1212–1219. doi: 10.1016/j.jinsphys.2011.05.015
Ahn, S. J., Badenes-Pérez, F. R., Reichelt, M., Svatoš, A., Schneider, B., Gershenzon, J., et al. (2011b). Metabolic detoxification of capsaicin by UDP glycosyltransferase in three Helicoverpa species. Arch. Insect Biochem. Physiol. 78, 104–118. doi: 10.1002/arch.20444
Ahn, S. J., Vogel, H., and Heckel, D. G. (2012). Comparative analysis of the UDP-glycosyltransferase multigene family in insects. Insect biochem. Mol. Biol. 42, 133–147. doi: 10.1016/j.ibmb.2011.11.006
Bao, H., Gao, H., Zhang, Y., Fan, D., Fang, J., and Liu, Z. (2016). The roles of CYP6AY1 and CYP6ER1 in imidacloprid resistance in the brown planthopper: expression levels and detoxification efficiency. Pestic. Biochem. Physiol. 129, 70–74. doi: 10.1016/j.pestbp.2015.10.020
Bock, K. W. (2003). Vertebrate UDP-glucuronosyltransferases: functional and evolutionary aspects. Biochem. Pharmacol. 66, 691–696. doi: 10.1016/S0006-2952(03)00296-X
Bock, K. W. (2016). The UDP-glycosyltransferase (UGT) superfamily expressed in humans, insects and plants: animal-plant arms-race and co-evolution. Biochem. Pharmacol. 99, 11–17. doi: 10.1016/j.bcp.2015.10.001
Bowles, D., Isayenkova, J., Lim, E. K., and Poppenberger, B. (2005). Glycosyltransferases: managers of small molecules. Curr. Opin. Plant Biol. 8, 254–263. doi: 10.1016/j.pbi.2005.03.007
Casida, J. E., and Durkin, K. A. (2013). Neuroactive insecticides: targets, selectivity, resistance, and secondary effects. Annu. Rev. Entomol. 58, 99–117. doi: 10.1146/annurev-ento-120811-153645
Chen, X., Li, F., Chen, A., Ma, K., Liang, P., Liu, Y., et al. (2017). Both point mutations and low expression levels of the nicotinic acetylcholine receptor β1 subunit are associated with imidacloprid resistance in an Aphis gossypii (Glover) population from a Bt cotton field in China. Pestic. Biochem. Physiol. 141, 1–8. doi: 10.1016/j.pestbp.2016.11.004
Denholm, I., and Rowland, M. W. (1992). Tactics for managing pesticide resistance in arthropods: theory and practice. Annu. Rev. Entomol. 37, 91–112. doi: 10.1146/annurev.en.37.010192.000515
Elbert, A., Haas, M., Springer, B., Thielert, W., and Nauen, R. (2008). Applied aspects of neonicotinoid uses in crop protection. Pest Manag. Sci. 64, 1099–1105. doi: 10.1002/ps.1616
Feyereisen, R. (2015). Insect P450 inhibitors and insecticides: challenges and opportunities. Pest Manag. Sci. 71, 793–800. doi: 10.1002/ps.3895
Heckel, D. G. (2014). Insect detoxification and sequestration strategies. Ann. Plant Rev. 47, 77–114. doi: 10.1002/9781118829783.ch3
Heidel-Fischer, H. M., and Vogel, H. (2015). Molecular mechanisms of insect adaptation to plant secondary compounds. Curr. Opin. Insect Sci. 8, 8–14. doi: 10.1016/j.cois.2015.02.004
Kaplanoglu, E., Chapman, P., Scott, I. M., and Donly, C. (2017). Overexpression of a cytochrome P450 and a UDP-glycosyltransferase is associated with imidacloprid resistance in the Colorado potato beetle, Leptinotarsa decemlineata. Sci. Rep. 7:1762. doi: 10.1038/s41598-017-01961-4
Karunker, I., Benting, J., Lueke, B., Ponge, T., Nauen, R., Roditakis, E., et al. (2008). Over-expression of cytochrome P450 CYP6CM1 is associated with high resistance to imidacloprid in the B and Q biotypes of Bemisia tabaci (Hemiptera: Aleyrodidae). Insect Biochem. Mol. Biol. 38, 634–644. doi: 10.1016/j.ibmb.2008.03.008
Karunker, I., Morou, E., Nikou, D., Nauen, R., Sertchook, R., Stevenson, B. J., et al. (2009). Structural model and functional characterization of the Bemisia tabaci CYP6CM1vQ, a cytochrome P450 associated with high levels of imidacloprid resistance. Insect Biochem. Mol. Biol. 39, 697–706. doi: 10.1016/j.ibmb.2009.08.006
Krempl, C., Sporer, T., Reichelt, M., Ahn, S. J., Heidel-Fischer, H., Vogel, H., et al. (2016). Potential detoxification of gossypol by UDP-glycosyltransferases in the two Heliothine moth species Helicoverpa armigera and Heliothis virescens. Insect Biochem. Mol. Biol. 71, 49–57. doi: 10.1016/j.ibmb.2016.02.005
Li, X., Shi, H., Gao, X., and Liang, P. (2017a). Characterization of UDP-glucuronosyltransferase genes and their possible roles in multi-insecticide resistance in Plutella xylostella (L.). Pest Manag. Sci. 74, 695–704. doi: 10.1002/ps.4765
Li, X., Zhu, B., Gao, X., and Liang, P. (2017b). Over-expression of UDP-glycosyltransferase gene UGT2B17 is involved in chlorantraniliprole resistance in Plutella xylostella (L.). Pest Manag. Sci. 73, 1402–1409. doi: 10.1002/ps.4469
Mackenzie, P. I., Owens, I. S., Burchell, B., Bock, K. W., Bairoch, A., Bélanger, A., et al. (1997). The UDP glycosyltransferase gene superfamily: recommended nomenclature update based on evolutionary divergence. Pharmacogenetics 7, 255–269. doi: 10.1097/00008571-199708000-00001
Mackenzie, P. I., Walter Bock, K., Burchell, B., Guillemette, C., Ikushiro, S., Iyanagi, T., et al. (2005). Nomenclature update for the mammalian UDP glycosyltransferase (UGT) gene superfamily. Pharmacogenet. Genomics 15, 677–685. doi: 10.1097/01.fpc.0000173483.13689.56
Magdalou, J., Fournel-Gigleux, S., and Ouzzine, M. (2010). Insights on membrane topology and structure/function of UDP-glucuronosyltransferases. Drug Metab. Rev. 42, 159–166. doi: 10.3109/03602530903209270
Meech, R., and Mackenzie, P. I. (1997). UDP-glucuronosyltransferase, the role of the amino terminus in dimerization. J. Biol. Chem. 272, 26913–26917. doi: 10.1074/jbc.272.43.26913
Meech, R., Miners, J. O., Lewis, B. C., and Mackenzie, P. I. (2012). The glycosidation of xenobiotics and endogenous compounds: versatility and redundancy in the UDP glycosyltransferase superfamily. Pharmacol. Ther. 134, 200–218. doi: 10.1016/j.pharmthera.2012.01.009
Pan, Y., Peng, T., Gao, X., Zhang, L., Yang, C., Xi, J., et al. (2015). Transcriptomic comparison of thiamethoxam-resistance adaptation in resistant and susceptible strains of Aphis gossypii Glover. Comp. Biochem. Physiol. D Genomics Proteomics 13, 10–15. doi: 10.1016/j.cbd.2014.11.001
Peng, T., Pan, Y., Gao, X., Xi, J., Zhang, L., Yang, C., et al. (2016b). Cytochrome P450 CYP6DA2 regulated by cap ‘n’ collar isoform C (CncC) is associated with gossypol tolerance in Aphis gossypii Glover. Insect Mol. Biol. 25, 450–459. doi: 10.1111/imb.12230
Peng, T., Pan, Y., Yang, C., Gao, X., Xi, J., Wu, Y., et al. (2016a). Over-expression of CYP6A2 is associated with spirotetramat resistance and cross-resistance in the resistant strain of Aphis gossypii Glover. Pestic. Biochem. Physiol. 126, 64–69. doi: 10.1016/j.pestbp.2015.07.008
Pfaffl, M. W. (2001). A new mathematical model for relative quantification in real-time RT-PCR. Nucleic Acids Res. 29:e45. doi: 10.1093/nar/29.9.e45
Puinean, A. M., Foster, S. P., Oliphant, L., Denholm, I., Field, L. M., Millar, N. S., et al. (2010). Amplification of a cytochrome P450 gene is associated with resistance to neonicotinoid insecticides in the aphid Myzus persicae. PLoS Genet. 6:e1000999. doi: 10.1371/journal.pgen.1000999
Scott, J. G. (2008). “Insect cytochrome P450s: thinking beyond detoxification, in recent advances,” in Insect Physiology, Toxicology and Molecular Biology, ed N. Liu (Research Signpost), 117–124.
Shang, Q., Pan, Y., Fang, K., Xi, J., and Brennan, J. A. (2012). Biochemical characterization of acetylcholinesterase, cytochrome P450 and cross-resistance in an omethoate-resistant strain of Aphis gossypii Glover. Crop Prot. 31, 15–20. doi: 10.1016/j.cropro.2011.09.014
Wei, X., Pan, Y., Xin, X., Zheng, C., Gao, X., Xi, J., et al. (2017). Cross-resistance pattern and basis of resistance in a thiamethoxam-resistant strain of Aphis gossypii Glover. Pestic. Biochem. Physiol. 138, 91–96. doi: 10.1016/j.pestbp.2017.03.007
Wouters, F. C., Reichelt, M., Glauser, G., Bauer, E., Erb, M., Gershenzon, J., et al. (2014). Reglucosylation of the benzoxazinoid DIMBOA with inversion of stereochemical configuration is a detoxification strategy in lepidopteran herbivores. Angew. Chem. 53, 11320–11324. doi: 10.1002/anie.201406643
Keywords: UDP-glucuronosyltransferase, insecticide resistance, Aphis gossypii, RNAi, thiamethoxam
Citation: Pan Y, Tian F, Wei X, Wu Y, Gao X, Xi J and Shang Q (2018) Thiamethoxam Resistance in Aphis gossypii Glover Relies on Multiple UDP-Glucuronosyltransferases. Front. Physiol. 9:322. doi: 10.3389/fphys.2018.00322
Received: 04 February 2018; Accepted: 15 March 2018;
Published: 03 April 2018.
Edited by:
Bin Tang, Hangzhou Normal University, ChinaReviewed by:
Guorui Yuan, Southwest University, ChinaMuthugounder S. Shivakumar, Periyar University, India
Copyright © 2018 Pan, Tian, Wei, Wu, Gao, Xi and Shang. This is an open-access article distributed under the terms of the Creative Commons Attribution License (CC BY). The use, distribution or reproduction in other forums is permitted, provided the original author(s) and the copyright owner are credited and that the original publication in this journal is cited, in accordance with accepted academic practice. No use, distribution or reproduction is permitted which does not comply with these terms.
*Correspondence: Qingli Shang, shangqingli@163.com
†Co-fist authors.