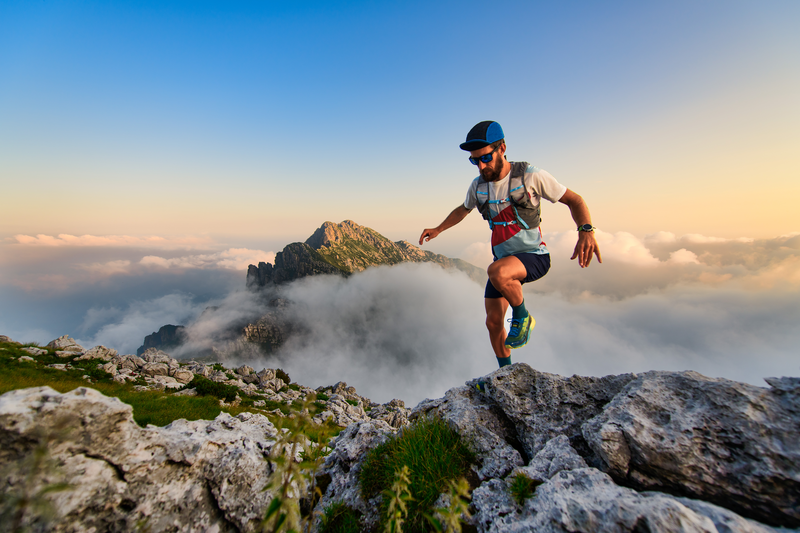
94% of researchers rate our articles as excellent or good
Learn more about the work of our research integrity team to safeguard the quality of each article we publish.
Find out more
SYSTEMATIC REVIEW article
Front. Physiol. , 13 February 2018
Sec. Exercise Physiology
Volume 9 - 2018 | https://doi.org/10.3389/fphys.2018.00073
Background: This systematic review and meta-analysis quantified the effect of acute exercise mode on arterial stiffness and wave reflection measures including carotid-femoral pulse wave velocity (cf-PWV), augmentation index (AIx), and heart rate corrected AIx (AIx75).
Methods: Using standardized terms, database searches from inception until 2017 identified 45 studies. Eligible studies included acute aerobic and/or resistance exercise in healthy adults, pre- and post-intervention measurements or change values, and described their study design. Data from included studies were analyzed and reported in accordance with the Cochrane Handbook for Systematic Reviews of Interventions and PRISMA guidelines. Meta-analytical data were reported via forest plots using absolute differences with 95% confidence intervals with the random effects model accounting for between-study heterogeneity. Reporting bias was assessed via funnel plots and, individual studies were evaluated for bias using the Cochrane Collaboration's tool for assessing risk of bias. A modified PEDro Scale was applied to appraise methodological concerns inherent to included studies.
Results: Acute aerobic exercise failed to change cf-PWV (mean difference: 0.00 ms−1 [95% confidence interval: −0.11, 0.11], p = 0.96), significantly reduced AIx (−4.54% [−7.05, −2.04], p = 0.0004) and significantly increased AIx75 (3.58% [0.56, 6.61], p = 0.02). Contrastingly, acute resistance exercise significantly increased cf-PWV (0.42 ms−1 [0.17, 0.66], p = 0.0008), did not change AIx (1.63% [−3.83, 7.09], p = 0.56), and significantly increased AIx75 (15.02% [8.71, 21.33], p < 0.00001). Significant heterogeneity was evident within all comparisons except cf-PWV following resistance exercise, and several methodological concerns including low applicability of exercise protocols and lack of control intervention were identified.
Conclusions: Distinct arterial stiffness and wave reflection responses were identified following acute exercise with overall increases in both cf-PWV and AIx75 following resistance exercise potentially arising fromcardiovascular and non-cardiovascular factors that likely differ from those following aerobic exercise. Future studies should address identified methodological limitations to enhance interpretation and applicability of arterial stiffness and wave reflection indices to exercise and health.
Cardiovascular disease (CVD) is projected to remain the leading cause of death worldwide until 2030 with measures for early detection and progress monitoring essential to manage the condition and its associated health costs (Pereira et al., 2015). Arterial stiffness, typically reported from assessments of carotid-femoral pulse wave velocity (cf-PWV), has been validated as an independent predictor of future incidence of coronary heart disease, stroke, and mortality (Mattace-Raso et al., 2006) with greater arterial stiffness considered one of the first signs of pathological arterial wall modifications leading to CVD (Pereira et al., 2015). While arterial stiffness increases naturally with aging (Shirwany and Zou, 2010), augmented arterial stiffness has been associated with long-term, poor lifestyle choices such as inadequate diet, lack of physical activity, and smoking (Shirwany and Zou, 2010). Altered arterial stiffness has been implicated as a primary haemodynamic factor influencing augmentation index (AIx) (Kelly et al., 2001), a measure of wave reflection and left ventricular afterload, and diagnostically useful indicator of future CVD risk, particularly in younger individuals (McEniery et al., 2005). Arterial stiffening contributes to augmented cf-PWV and AIx, which increases cardiac workload and reduces coronary perfusion, thereby advancing the future risk of left ventricular hypertrophy and myocardial ischemia (Cavalcante et al., 2011). Subsequently, actions to minimize increases in arterial stiffness may be vital to manage CVD risk.
A recent systematic review and meta-analysis of 42 randomized, controlled trials reported that chronic aerobic training resulted in reduced cf-PWV and AIx, whereas chronic resistance and combined (aerobic + resistance) training had no effect on these measures (Ashor et al., 2014). A consequent review of 17 randomized, controlled trials also reported reductions in cf-PWV following aerobic but not resistance training (Li et al., 2015). Collectively, these reviews indicated exercise mode as an important modulator of chronic arterial stiffness and wave reflection responses.
While the effects of chronic exercise have been collated (Ashor et al., 2014; Li et al., 2015), no reviews to date have systematically examined the overall acute effects of exercise mode (i.e., aerobic vs. resistance exercise) on arterial stiffness and wave reflection. Numerous cross-group studies investigating the effects of acute exercise on these measures have produced inconclusive findings with some studies reporting decreases (Kingwell et al., 1997; Sugawara et al., 2015; Kobayashi et al., 2017), no change (Campbell et al., 2011; Ranadive et al., 2012; Ribeiro et al., 2014), or increases (Fahs et al., 2009; Yoon et al., 2010; Kingsley et al., 2016) in cf-PWV and/or AIx. Factors such as selection of outcome measures, timing of measurement, and, particularly, exercise mode, may explain the divergent findings. Findings from a review that will identify the acute responses to exercise modes will clarify common limitations of research designs, and provide recommendations to improve the experimental approach for future studies. Furthermore, such findings may identify the potential beneficial or adverse effects of acute exercise modes on arterial stiffness and wave reflection that may assist in understanding the impact of exercise on cardiovascular function for improved health. This systematic review and meta-analysis aimed to investigate and quantify the effect of exercise mode, during a single bout, on post-exercise cf-PWV, AIx, and AIx normalized to a heart rate of 75 beats per minute (AIx75). Since aerobic and resistance exercise modes are currently endorsed by major health organizations for comprehensive health programmes (Williams et al., 2007; Garber et al., 2011), findings from this review and meta-analysis may determine the potential exercise mode effects on arterial stiffness and wave reflection for cardiovascular health.
This systematic review was conducted in accordance with the Cochrane Handbook for Systematic Reviews of Interventions (Higgins and Green, 2011) and reported in line with PRISMA guidelines (Liberati et al., 2009).
Studies were deemed eligible and included into the review if they examined all of the following criteria: (1) included apparently healthy young human adults ≤45 years; (2) investigated a single bout of aerobic and/or resistance exercise only or included a comparative intervention (e.g., no exercise); (3) included an exercise alone condition in studies with additional exposures (e.g., vascular occlusion); (4) reported pre- and post-intervention measurements or change values of cf-PWV, AIx, and/or AIx75 (aortic AIx only); and (5) described their study design. Exclusion criteria included studies that involved: (1) athletes or highly trained participants (e.g., ≥10 years of training history); (2) anaerobic exercise (e.g., Wingate) or isometric resistance exercise interventions; and (3) no pre- or post-intervention measurements. All studies were screened to ensure that participants had no pre-existing medical conditions that could affect arterial stiffness and wave reflection responses to exercise. To control for the potential influence of age, particularly older age, on these responses (Thiebaud et al., 2016), only studies with participants aged 18–45 years were included in this review. Studies with clinical populations were eligible for inclusion in this review if they incorporated a control group of healthy participants; only measurements for the healthy control group were reported and/or included in the analyses. Similarly, if the exercise intervention involved additional stressors (e.g., heat exposure), only the exercise alone condition was considered for analyses. Furthermore, studies had to report one or more of the following outcome measures, which are considered diagnostically superior predictors of future CVD risk (Davies and Struthers, 2003; Franklin, 2008): cf-PWV, AIx, or AIx75. Finally, only studies that focused on exercise modalities recommended for the maintenance and improvement of cardiovascular and general health (Garber et al., 2011) were considered for inclusion.
Using standardized terms, a search of the PubMed, Ovid MEDLINE, Cochrane Library, and SPORTDiscus databases from database inception until the search date (10-16/05/2017) was conducted. Searches were limited to studies involving “Humans” and reported in English. Keywords used in the searches were: “arterial stiffness,” “vascular stiffness,” “acute exercise,” “pulse wave velocity,” “pulse wave analysis,” “augmentation index,” “PWV,” “PWA,” “AIx,” “AIx75.” The PubMed search strategy is detailed in the Supplementary Material (Supplementary Figure 1) and search strategies were adapted for each database. Additionally, reference lists of relevant articles and reviews identified in the searches were searched for eligible studies. Database alerts for recently published studies (May 2017–September 2017) were also continuously monitored for potentially eligible studies.
One reviewer (DP) conducted the initial searches and screening of all identified titles, abstracts, and full original articles in line with the eligibility criteria (Figure 1). To assess the clarity of the inclusion/exclusion criteria, 212 articles were also independently screened by two other reviewers (ASL and KD) for inclusion into this review with initial inter-rater reliability >0.86 (McHugh, 2012). Where discrepancies between reviewers were present, decisions to include studies were discussed until consensus was reached. A total of 45 articles were identified for inclusion into this review (Figure 1). As no common post-intervention time point was assessed in all studies included in the review, data from the last time point within the immediate 60-min, post-intervention period were utilized for the meta-analysis. Three studies were excluded from the meta-analysis (Barnes et al., 2010; Lin et al., 2016; Perdomo et al., 2016) as they did not include data within the 60-min post-intervention period, while a fourth one (Collier et al., 2010) was excluded due to lack of pre-intervention data that could not be obtained from the corresponding author upon follow-up, leaving 41 studies to be included in the meta-analysis.
Figure 1. Selection process detailing the implemented search procedure in assessing eligibility for inclusion in this systematic review and meta-analysis.
A customized form was used to extract information about study design, participant details (e.g., health status, number, age, sex, stage of menstrual cycle in females, smoking status, activity level, recruitment strategy), study aim and hypothesis, methodology (e.g., intervention details, outcome measures, data collection time points, instruments/systems used), main findings, limitations, and conclusions. Results for each study's outcome measures were then entered into Microsoft Excel with pre- and post-intervention values for each outcome measure and time point entered as mean ± standard deviation (SD). For cross-over trials, mean ± SD were reported separately for each condition.
Methodological aspects were appraised using a modified PEDro scale. As blinding of participants or researchers to an exercise intervention was difficult, if not impossible, items 5–7 (i.e., blinding) of the original PEDro scale (Elkins et al., 2010) were replaced with items describing a priori power analysis, adequacy of intervention details, and inclusion of a control group. Two further items assessing bias (i.e., reporting of dropout/participation rates and sources of other potential bias) were added, resulting in a maximum quality score of 13. Additionally, the Cochrane Collaboration's tool for assessing risk of bias (i.e., selection, performance, detection, attrition, reporting and other bias) using a domain-based evaluation (Higgins and Green, 2011) was applied to all studies, as traditional scales and checklists generally contain items that are not directly related to internal validity (Higgins and Green, 2011).
Corresponding authors were contacted where data were not clear or unavailable, and data were updated for inclusion into this review. Several studies investigating the acute effects of aerobic exercise were included in a previous review of acute aerobic exercise (Mutter et al., 2016), and data extracted were cross-referenced with the previously reported data for quality assurance, where available.
Reporting bias, including publication bias, was minimized by use of eligibility criteria, consensus of multiple reviewers, and inspection of funnel plots. Funnel plots are simple scatter plots with the studies' mean differences plotted on the x-axis and the standard error on the y-axis (Sterne and Egger, 2001). In the absence of publication bias, the plots should resemble an inverted funnel (Sterne and Egger, 2001). Participant selection bias was inherent within the incorporated studies, as only studies investigating healthy, young adult participants were included. However, this was intentional, as the aim was to review normal arterial stiffness and wave reflection responses after different modes of acute exercise in this healthy population group.
Forest and funnel plots were generated using Review Manager Software (Review Manager [RevMan]. Version 5.3. Copenhagen: The Nordic Cochrane Centre, The Cochrane Collaboration, 2014), and data are presented as mean ± SD, unless otherwise stated. Data originally reported as mean ± standard error or mean ± confidence interval were converted to mean ± SD for consistency following Cochrane guidelines (Higgins and Green, 2011). Where only baseline and change values (post-pre) were reported in a study, the post-intervention measurement was computed by adding the means and calculating the pooled SD (Higgins and Green, 2011). Data not reported in tables or the main text were extracted manually from figures as described previously (Kadic et al., 2016). The outcome of each individual meta-analysis represents the pre-post intervention difference in outcome variable (i.e., cf-PWV, AIx, or AIx75) for each intervention. The meta-analytical data were reported via forest plots using absolute differences with 95% confidence interval, given that each outcome measure (i.e., cf-PWV, AIx, and AIx75) was clustered separately (Higgins and Green, 2011). The random effects model was used to account for possible between-study heterogeneity regarding study design, participant characteristics, and methodology used to assess arterial stiffness and wave reflection (Higgins and Green, 2011). The percentage of variation across studies indicative of heterogeneity was reported using the I2 statistic and the Chi-squared test. Interpretation of the I2 statistic was in accordance with Cochrane guidelines as following: <40% might not be important; 30–60% may represent moderate heterogeneity; 50–90% may represent substantial heterogeneity; and >75% considerable heterogeneity (Higgins and Green, 2011). Furthermore, a statistically significant effect based on the Chi-squared test suggested evidence of heterogeneity (Higgins and Green, 2011). Pearson product-moment correlation coefficients were computed to assess the relationship between timing of post-intervention measurement and reported change (%) in cf-PWV, AIx, and AIx75. Statistical significance was determined with an alpha level of ≤0.05.
A total of 45 and 41 studies met the eligibility criteria and were included into the qualitative review and meta-analysis, respectively. Figure 1 summarizes the screening and selection process. Primary reasons for exclusion of articles during screening of the full-text records were assessment of arterial stiffness and wave reflection following chronic exercise training as opposed to acute exercise (92 studies) and reporting of outcome measures not aligned with our selection criteria (34 studies, Figure 1).
The total number of participants across all studies was 1,211 (889 men, 297 women, 25 not specified) with a mean sample size of 22.4 ± 17.2 (range 9–122) and participant age ranging from 18 to 45 years. Twenty-six studies included men only, no study included women only, 18 included both men and women, and sex distribution was not reported for one study (Table 1). Participants' baseline physical activity status, although not reported in all studies, ranged from sedentary to highly physically active. Nine studies (Sharman et al., 2008; Fahs et al., 2009; Collier et al., 2010; Gkaliagkousi et al., 2014; Hanssen et al., 2015; Kingsley et al., 2016; Perdomo et al., 2016; Thiebaud et al., 2016; Tai et al., 2018) reported an a priori sample size calculation.
The study designs were variable and included 17 cross-over studies (Kingwell et al., 1997; Heffernan et al., 2007c,d; Barnes et al., 2010; Collier et al., 2010; Yoon et al., 2010; Figueroa and Vicil, 2011; Ranadive et al., 2012; Ribeiro et al., 2014; Hanssen et al., 2015; Lefferts et al., 2015; Siasos et al., 2016a,b; Thiebaud et al., 2016; Kobayashi et al., 2017; Yan et al., 2017; Tai et al., 2018), 24 pre-post interventions (Heffernan et al., 2006, 2007a,b; Lydakis et al., 2008; Munir et al., 2008; Peres et al., 2010; Boutcher et al., 2011; Doonan et al., 2011, 2013; Hull et al., 2011; Hu et al., 2013; Lane et al., 2013; Gkaliagkousi et al., 2014; Yan et al., 2014; Burr et al., 2015; Chandrakumar et al., 2015; Mak and Lai, 2015; Milatz et al., 2015; Sugawara et al., 2015; Sun et al., 2015; Kingsley et al., 2016; Melo et al., 2016; Moore et al., 2016; Perdomo et al., 2016) and four randomized, controlled trials utilizing a pharmaceutical/placebo design (Sharman et al., 2008; Fahs et al., 2009; Campbell et al., 2011; Lin et al., 2016). Thirty-four studies assessed the effects of aerobic exercise (Table 2) and 13 examined the effects of resistance exercise (Table 3); two of these studies (Heffernan et al., 2007d; Collier et al., 2010) compared the effects of aerobic vs. resistance exercise. Eight of the 45 studies (six resistance, two aerobic) included a control group that completed no exercise (Table 4). Aerobic exercise was either performed on a cycle ergometer (21 studies) or a treadmill (13 studies) with varying intensities and durations (Table 2).
Table 2. Summary of studies that examined carotid-femoral pulse wave velocity and/or augmentation indices post aerobic exercise intervention.
Table 3. Summary of studies that examined carotid-femoral pulse wave velocity and/or augmentation indices post resistance exercise intervention.
Table 4. Summary of studies that examined carotid-femoral pulse wave velocity and/or augmentation indices post control (seated rest) intervention.
Exercise intensity was expressed in relative (i.e., a percentage of maximum heart rate) or absolute (i.e., Watt or km h−1) terms, and duration was either limited by time (10–60 min) or participant fatigue. Resistance exercise was executed using lower body only (four studies), upper body only (two studies), or whole-body exercise (seven studies) with varying repetitions (5 to fatigue), sets (1–15), intensities (10–110% of one repetition maximum) and rest periods (70 s to 4 min).
Outcome measures were assessed as follows: cf-PWV only in 23 studies (18 aerobic, 7 resistance, 2 assessed both exercise modes), AIx only in 4 studies (2 aerobic, 2 resistance), AIx75 only in 2 studies (2 aerobic), cf-PWV, and AIx/AIx75 in 9 studies (8 aerobic, 1 resistance), AIx and AIx75 in 3 studies (2 aerobic, 1 resistance), and cf-PWV, AIx, and AIx75 in 4 studies (2 aerobic, 2 resistance). In summary, 29 studies (64.4%) reported only one, 12 studies (26.7%) reported two and 4 studies (8.9%) reported three outcome measures.
The timing of outcome measures varied amongst studies from 0 min to 72 h; however, most (93.3%) examined variables at 0–30 min (91.2% of aerobic, 84.6% of resistance, 75% of the control group) of the post-intervention period. Time points for studies included in the meta-analysis varied from 0 to 60 min for aerobic studies and from 0 to 40 min for resistance studies. There was a weak, but significant, negative correlation (r = −0.368, n = 37, p = 0.02) between timing of post-intervention measurement and pre-post change (%) for cf-PWV following aerobic exercise only, indicating that early post-intervention measurements were more likely to demonstrate increased cf-PWV compared to a later measurement. No significant correlations were found for other outcome measures and following resistance exercise.
Mean score for methodological quality was 8.6 ± 1.4 (range 5–12) out of a possible maximum score of 13. The main reasons for poor scoring were: (a) lack of randomization of participants to interventions (26 studies, 57.8%) largely due to study design (i.e., pre-post intervention studies with only one intervention; (b) lack of control group (37 studies, 82.2%); and (c) no reporting of dropout/participation rates (24 studies, 53.3%). All but five studies (Kingwell et al., 1997; Munir et al., 2008; Figueroa and Vicil, 2011; Sun et al., 2015; Siasos et al., 2016a) discussed limitations and sources of potential bias. Risk of bias assessment via the Cochrane Collaboration's tool indicated that most information was from studies exhibiting overall low or unclear risk of bias (Supplementary Table 1).
No significant change in cf-PWV was identified following aerobic exercise, and we found significant heterogeneity within this comparison (Figure 2). In contrast, resistance exercise was associated with significantly greater cf-PWV and non-significant heterogeneity. The pooled data for cf-PWV following aerobic and resistance exercise demonstrated an overall non-significant effect with significant heterogeneity among the studies overall (Figure 2). Heterogeneity between the two sub-groups (aerobic and resistance) was also significant (Figure 2). For the control group, no significant change in cf-PWV was observed, and heterogeneity between the studies was non-significant (Figure 3). Visual inspection of the funnel plot (Supplementary Figure 2A) did not suggest publication bias for resistance exercise but showed asymmetry on the right for aerobic exercise, suggesting the absence of studies with positive/increased cf-PWV, either because of publication bias or because of a true nonexistence of these studies (i.e., absence of publication bias).
Figure 2. Forest plots showing the effect of acute aerobic and resistance exercise on cf-PWV. HW, healthy weight; 30-minEX, 30-min exercise duration; NRT, non-resistance trained; 45-minEX, 45-min exercise duration; AA, African American; CAM, Caucasian men; M, men; CA, Caucasian; CAE, continuous aerobic exercise; 15-minEX, 15-min exercise duration; NHS, no heat stress; HIAE, high-intensity aerobic exercise; Leg, leg ergometry; OW, overweight; CAW, Caucasian women; W, women; WH, white; NS, non-smokers; CH, Chinese; AAW, African American women; Arm, arm ergometry; RT, resistance trained; AAM, African-American men; NVM, no Valsalva maneuvre; YG, young group.
Figure 3. Forest plots showing the effect of seated rest on cf-PWV, AIx, and AIx75. YG, young group.
A significant reduction in AIx was identified following aerobic exercise, and we found significant heterogeneity within this comparison (Figure 4). In contrast, resistance exercise was not associated with a significant change in AIx, but significant heterogeneity among the studies existed (Figure 4). The pooled data for AIx following aerobic and resistance exercise demonstrated an overall significant reduction with significant heterogeneity among the studies overall (Figure 4). Heterogeneity between the two sub-groups (aerobic and resistance) was significant (Figure 4). For the control group, no significant change in AIx was observed, and heterogeneity between the studies was non-significant (Figure 3). Visual inspection of the funnel plot (Supplementary Figure 2B) did not suggest publication bias for aerobic exercise but showed asymmetry on the left for resistance exercise, suggesting the absence of studies with negative/decreased AIx, either because of publication bias or because of a true nonexistence of these studies (i.e., absence of publication bias). Since decreased AIx would be a desirable outcome following resistance exercise, it is unlikely that studies finding such reduction would remain unpublished; the asymmetry may therefore, reflect a true nonexistence.
Figure 4. Forest plots showing the effect of acute aerobic and resistance exercise on AIx. W, women; CAW, Caucasian women; AAW, African American women; M, men; AAM, African American men; CAM, Caucasian men; NRT, non-resistance trained; HIIT, high-intensity interval training; RT- resistance trained; NFHT, no family history of hypertension; FHT, family history of hypertension; MCT, moderate continuous training; OW, overweight; HW, healthy weight; NVO, no vascular occlusion.
A significant increase in AIx75 was identified following both aerobic and resistance exercise, and we found significant heterogeneity within each comparison (Figure 5). The pooled data for AIx75 following aerobic and resistance exercise demonstrated an overall significant increase with significant heterogeneity among the studies overall (Figure 5). Heterogeneity between the subgroups (aerobic and resistance) was significant (Figure 5). For the control group, no significant change in AIx75 was observed, and heterogeneity between the studies was non-significant (Figure 3). Visual inspection of the funnel plot (Supplementary Figure 2C) showed asymmetry on the right for aerobic exercise, suggesting the absence of studies with positive/increased AIx75, either because of publication bias or because of a true non-existence of these studies (i.e., absence of publication bias). For resistance exercise, the funnel plot showed asymmetry on the left for resistance studies, suggesting the absence of studies with negative/decreased AIx75, either because of publication bias or because of a true nonexistence of these studies (i.e., absence of publication bias). Since decreased AIx75 would be a desirable outcome following resistance exercise, it is unlikely that studies finding such a reduction would remain unpublished; the asymmetry may therefore, reflect a true nonexistence.
Figure 5. Forest plots showing the effect of acute aerobic and resistance exercise on AIx75. CAE, continuous aerobic exercise; CAW, Caucasian women; AAW, African American women; HIAE, high-intensity aerobic exercise; AAM, African American men; MCT, moderate continuous training; NRT, non-resistance trained; CAM, Caucasian men; HIIT, high-intensity interval training; RT, resistance trained; W, women; M, men; NS, non-smokers; YG, young group.
The current systematic review and meta-analysis demonstrated that, overall, distinct arterial stiffness recovery responses existed following a single bout of aerobic and resistance exercise with the differences possibly originating from unique cardiovascular (i.e., blood pressure, heart rate) and non-cardiovascular (i.e., inflammatory products) processes. Additionally, the results from the present review and meta-analysis demonstrate the limitations of current research designs that can assist in improving the experimental approach of future studies.
Unlike the mean difference following resistance exercise, mean difference for cf-PWV following aerobic exercise in the present meta-analysis was not significant overall, which suggested an inability for aerobic exercise to significantly alter arterial stiffness responses. Although the exact mechanisms regulating modal differences in cf-PWV have not been identified, several mechanisms have been implicated in previous studies (Yoon et al., 2010) and likely involve distinct blood pressure responses to exercise (Izzo, 2004).
While blood pressure increases during both acute aerobic and resistance exercise, the magnitude and nature of this increase differs between modes (MacDougall et al., 1985). Increases in blood pressure during aerobic exercise can be moderate and more sustained due to the use of large muscles in a rhythmic manner whereas intense resistance exercise can result in brief, intermittent increases in blood pressure, reaching up to fourfold resting values, due to mechanical compression of blood vessels, a strong exercise pressor reflex, and execution of the Valsalva maneuver (MacDougall et al., 1985). Subsequently, this extreme, intermittent distending pressure may cause a transient switch in load bearing from elastin to collagen fibers of arteries and thus, increased cf-PWV (MacDougall et al., 1985), which is not seen with the moderate and stable pressure during aerobic exercise. However, the significant increase in cf-PWV was not observed in all resistance exercise studies. Studies that employed upper and whole-body resistance exercise reported increased cf-PWV (Heffernan et al., 2007d; Fahs et al., 2009; Kingsley et al., 2016), whereas studies investigating lower body resistance exercise reported no change (Heffernan et al., 2006, 2007c). These findings were possibly facilitated by the greater blood pressure changes during upper body compared to lower body resistance exercise (Stenberg et al., 1967), highlighting the influence of muscle groups exercised rather than intensity (Heffernan et al., 2007c) as a key contributor to changes in cf-PWV. The addition of the Valsalva maneuver, almost obligatory during intense (>80–85% of one repetition maximum) resistance exercise (McCartney, 1999), may also contribute independently to the increase in cf-PWV through promotion of large increases in intra-thoracic and intra-abdominal pressures, which are transmitted to the aorta (MacDougall et al., 1985; Heffernan et al., 2007c).
Reduced left ventricular ejection time, as seen with tachycardia during and following acute exercise, has also been implicated as an independent predictor of cf-PWV with Salvi et al. (2013) reporting a significant and inverse association between cf-PWV and left ventricular ejection time for all age groups. Previous studies indicated that heart rate values during intense aerobic exercise remained below these reached during resistance exercise with heart rates of 147 beats per minute reported during high-intensity cycling (80% of maximum heart rate) (Sharman et al., 2005) compared to 160–170 beats per minute reported during each set of a lift (McCartney, 1999). One suggested mechanism for the left ventricular ejection time and cf-PWV association was the greater left ventricular, ejection force during a reduced left ventricular ejection time with this increased force translating to augmented blood pressure and increased cf-PWV as a result of changes in arterial wall viscoelastic properties as described above. Additionally, reduced arterial recoil time for predominantly elastic arteries (e.g., aorta) resulting from tachycardia may also contribute to vascular stiffening (Mangoni et al., 1996). Together, changes in blood pressure, heart rate, and left ventricular ejection time may be crucial cardiovascular modulators of cf-PWV following different modes of acute exercise; however, non-cardiovascular factors may also modify cf-PWV post-exercise.
Compared to more concentrically-biased aerobic activities (e.g., cycling), substantially elevated inflammation (Barnes et al., 2010) was reported following the greater eccentric component of acute resistance exercise that may also be partially responsible for increased cf-PWV following resistance exercise. Specifically, elevated levels of acute inflammatory markers such as c-reactive protein, interleukin-6, and matrix metalloproteinase-9 were associated with significantly increased cf-PWV (Yasmin et al., 2004; Vlachopoulos et al., 2005; Shirwany and Zou, 2010; Jae et al., 2013). Potentially, eccentric muscle contractions during resistance exercise and eccentrically-biased aerobic exercise protocols such as downhill treadmill running (Burr et al., 2015) may induce greater muscle damage and inflammatory products that negatively influence nitric oxide bioavailability (Vlachopoulos et al., 2005) and endothelium-dependent dilatation (Hingorani et al., 2000), thereby enhancing arterial stiffness (Kano et al., 2005). Further, resistance exercise has been reported to promote the release of angiotensin II (Kraemer et al., 1999). Besides its vasoconstrictive effect, angiotensin II may cause a shift from elastin to collagen synthesis, vascular hypertrophy, a heightened inflammatory response, and depression of nitric oxide dependent signaling (Zieman et al., 2005). This alteration in endothelial cell signaling and vascular smooth muscle tone has been demonstrated to affect vascular stiffening and, particularly, cf-PWV (Rehman et al., 2002). In addition to these reported cardiovascular and non-cardiovascular mechanisms modulating cf-PWV, methodological factors such as timing of post-exercise measurement, and duration and intensity of exercise may also explain the absence of significant changes in cf-PWV following aerobic exercise.
Previously, increased cf-PWV was reported for both healthy young men and women at 2-min following a treadmill protocol to volitional exhaustion (Doonan et al., 2013), whereas other studies (Gkaliagkousi et al., 2014; Melo et al., 2016) reported no change in cf-PWV at 10, 30, and 60 min following aerobic exercise. Exercise protocols and study participants were nearly identical amongst these studies with the divergent findings possibly resulting from the timing of measurement. Our finding of a weak, but significant, negative correlation between timing of post-intervention measurement and cf-PWV outcomes following aerobic exercise indicates that early post-intervention measurements were more likely to demonstrate increased cf-PWV compared to later measurements. A recent systematic review of acute aerobic exercise studies also reported that timing of post-exercise measurement possibly influenced arterial stiffness outcomes, since changes may be only short-lived (Mutter et al., 2016). Therefore, measurement of arterial stiffness indices early during post-intervention may allow the detection of significant changes that may have abated later during post-intervention (e.g., 10-min). No significant correlation between timing of post-intervention measurement and cf-PWV following resistance exercise was evident in this review, indicating a more consistent effect of acute resistance exercise on cf-PWV, most likely due to the severity of cardiovascular disturbance during exercise (MacDougall et al., 1985; McCartney, 1999; Sharman et al., 2005). Therefore, the characteristics of the exercise bout were likely to be crucial to the post-exercise response. In the current review, exercise durations varied between studies (10–60 min) with one aerobic exercise study (Kobayashi et al., 2017) reporting a prolonged reduction in cf-PWV following a 60-min protocol compared to a 15- and 30-min protocol. However, many studies failed to report exercise duration which limited conclusions about the effect of exercise duration on post-exercise change in cf-PWV. Additionally, exercise intensity may be a significant factor that contributes to changes in post-exercise cf-PWV. Similar to duration, intensity of exercise bouts varied between studies with the nature of some aerobic interventions (i.e., graded exercise protocols) and the diversity of exercise intensity measures used limiting a meaningful comparison within and between exercise modes in the current review. Future studies, including systematic reviews and meta-analyses, are encouraged to report and consider both duration and intensity of the exercise bout when examining post-exercise cf-PWV.
While attention to the exercise bout itself should be considered, factors such as diversity of measuring techniques and participants may also be crucial to the current cf-PWV findings. Different assessment techniques, including automated devices, applanation tonometry/calculation, and direct/subtraction methods, have been utilized with variable findings after aerobic exercise. Further, several studies (Lydakis et al., 2008; Munir et al., 2008; Peres et al., 2010; Hull et al., 2011; Ranadive et al., 2012; Hu et al., 2013; Gkaliagkousi et al., 2014; Burr et al., 2015; Sun et al., 2015; Melo et al., 2016; Kingsley et al., 2017; Yan et al., 2017; Tai et al., 2018) failed to report separate cf-PWV values for female and male participants with the known inherent sex differences in cf-PWV at rest and following exercise (Doonan et al., 2013) potentially impeding valid comparisons between studies.
In summary, acute resistance exercise induces adverse effects on cf-PWV due to cardiovascular and/or non-cardiovascular mechanisms. In contrast, acute aerobic exercise effects were minimal due to these cardiovascular and/or non-cardiovascular mechanisms with findings also potentially influenced by exercise protocols (e.g., muscle groups exercised), timing of measurement, duration and intensity of exercise, measuring techniques, and/or participants that remain to be elucidated.
Overall, the mean difference for AIx was large and negative following aerobic exercise, representing a substantial reduction in wave reflection, whereas it was small following resistance exercise, indicating no acute change. Results from studies investigating acute aerobic exercise were largely homogenous with most studies reporting decreased AIx. Previously, aerobic exercise was reported to promote nitric oxide-induced vasodilation via increased blood flow and shear stress, resulting in reduced wave reflection (Munir et al., 2008). The current meta-analysis provides further support of this beneficial effect of aerobic exercise on wave reflection. In contrast, AIx results from resistance exercise studies were inconsistent, with results possibly a result of distinct muscle group differences. Whereas upper and whole body resistance exercise were associated with increased wave reflection (Fahs et al., 2009; Tai et al., 2018), lower body resistance exercise was associated with reduced wave reflection (Figueroa and Vicil, 2011), implying that muscle group activation and/or changes in cardiovascular and non-cardiovascular function resolved the effect of acute resistance exercise on indices of wave reflection. Previous measures of wave reflection were determined by the velocity of the incident wave (i.e., cf-PWV), left ventricular ejection time, and peripheral vasomotor tone (Kelly et al., 2001; London and Pannier, 2010). Although the greater systolic and, consequently, shorter diastolic duration associated with increased left ventricular ejection time also increases the probability of an early wave return (London and Pannier, 2010), cf-PWV may be the predominant influence on wave reflection timing (Kelly et al., 2001). Additionally, as magnitude of wave reflection is affected by a mismatch between central and peripheral vasomotor tone, the greater peripheral vessel constriction seen with resistance exercise may result in greater wave reflection (Kelly et al., 2001). Greater activation of the sympathetic nervous system during resistance exercise, and specifically during upper body resistance exercise, has been identified as a key mechanism for the vasoconstrictive effect (Okamoto et al., 2009). Therefore, the greater wave reflection and increased AIx reported with upper and whole-body resistance exercise may result from increased cf-PWV and/or greater peripheral vasomotor tone.
The current review extensively evaluated the acute influence of exercise mode on indices of arterial stiffness and wave reflection, including AIx75, which has rarely been examined in terms of exercise mode. Based upon the meta-analysis, AIx75 was overall significantly increased following both acute aerobic and resistance exercise with the mean difference following resistance exercise nearly five times that following aerobic exercise (15.02 vs. 3.54%). This considerably greater and non-heart rate mediated (i.e., cf-PWV, left ventricular ejection time, and peripheral vasomotor tone) response following resistance exercise further exemplified the modal differences in arterial stiffness and wave reflection responses. Additionally, these results indicated that AIx75 may be a more useful measure of left ventricular afterload, compared to AIx, when comparing exercise responses in future studies.
The meta-analysis reported here combined data from many studies to estimate acute intervention effects on arterial stiffness and wave reflection with more precision than possible in a single study. The main limitation of this approach was the heterogeneity amongst studies in terms of participants, exercise protocols (i.e., exercise mode, duration and intensity), and outcome assessment (e.g., timing of measurement). The modified PEDro scale and risk of bias assessment using the Cochrane tool identified several possible sources for risk of bias within the studies. For example, lack of random sequence generation in 57.8% of all studies (item 2 on PEDro scale), largely due to study design (i.e., pre-post intervention design without control intervention) and lack of allocation concealment (item 3), may have caused an unclear risk of selection bias. Similarly, the nature of the interventions, that is aerobic, resistance or no exercise, made blinding of participants and outcome assessors impossible, which may have led to performance or detection bias. However, participants were likely unaware of the expected outcome associated with their individual intervention or the mechanisms affecting these outcomes; therefore, attempts at manipulating outcomes were unlikely. Assessors may have been aware of expected outcomes with each intervention, but automated measurement techniques of cf-PWV and wave reflection likely minimized the possibility of detection bias. A lack of reporting of dropout/participation rates (item 12) in more than 50% of studies raised the issue of attrition bias. However, no trend between any components of bias and mean difference was identified.
During this review, several common, methodological concerns were also noted. Firstly, very few studies employed a specific exercise protocol recommended for improving health of the general population (Garber et al., 2011). This selection of exercise protocols may greatly restrict the applicability and generalizability of findings from prior studies with future studies encouraged to examine exercise modalities and intensities/durations prescribed for general and cardiovascular health. Secondly, more than 50% of studies were designed as non-randomized, pre-post interventions without a non-exercise control group, thereby weakening internal validity (Harris et al., 2006). A mere 17.7% (8/45) of studies included a control group to account for normal variation in arterial stiffness. A randomized, cross-over design including a control group may be a more appropriate study design to avoid potential threats for establishing causality in future studies (Harris et al., 2006). Thirdly, measurements of post-exercise arterial stiffness in most studies were conducted at isolated time points rather than over a time course. As changes in post-exercise arterial stiffness and wave reflection indices are generally transient in nature (Mutter et al., 2016), prior studies may have “missed” these real changes and reported erroneous conclusions. Future studies could avoid this shortcoming by adopting a schedule that incorporates measurements at regular intervals over a time course (e.g., every 10-min). Fourth, measurement of only one index was conducted in most studies and may have not provided a comprehensive picture of changes in cardiovascular loading, particularly as AIx has been implicated as a more sensitive marker in younger adults, while cf-PWV is considered a more meaningful marker in older adults (Mitchell et al., 2004). Finally, several studies did not report results separately for female and male participants. Despite some efforts to control for menstrual cycle phase (Madhura and Sandhya, 2014), the apparent difference in resting cf-PWV and cf-PWV responses to exercise between males and females (Doonan et al., 2013) may have impacted on arterial stiffness and wave reflection results. More importantly though, most of the included studies did not look at exercise modal differences in the same population within the same study. Therefore, the observed exercise modal differences may be due to confounding factors such as different participant characteristics.
Like most reviews, several limitations need to be acknowledged. The current systematic review and meta-analysis was based upon studies reported in English. The use of English search terms may have led to omission of studies reported in other languages. To minimize the risk of English-language bias, several databases, including The Cochrane Controlled Trials Register that has been reported as the best single source of trials for inclusion in systematic reviews and meta-analyses (Egger et al., 1997), were searched. Additionally, reference lists were searched manually to identify potential further trials, which has been described as potentially more important in finding further trials than the choice of electronic database (Egger et al., 1997). Furthermore, due to great heterogeneity in post-exercise measurement time points, our meta-analysis was based on data within a post-intervention period rather than at one time point. Of the 45 included studies, 41 reported at least one measurement time point within a 60-min post-intervention period with no consistent time point identified for most studies. Consequently, the last reported measurement within the 60-min period was used in the calculation of pre-post mean differences for consistency of comparisons in post-exercise assessment of arterial stiffness indices.
Increased central arterial stiffness (i.e., cf-PWV) and wave reflection measures (i.e., AIx/AIx75) following acute resistance exercise were observed for healthy adults in the present meta-analysis. This transient increase may not be cause for concern in a young, healthy population group with low baseline levels of central arterial stiffness, as the observed, average 0.46 ms−1 increase in cf-PWV was well below the 1 ms−1 increase associated with a 15% increase in CVD risk (Vlachopoulos et al., 2010). Nevertheless, these arterial stiffness and wave reflection alterations may contribute transiently to an increased risk of cardiovascular events seen with acute, strenuous exercise in older, high-risk populations (Willich et al., 1993; Hallqvist et al., 2000). Despite the well-known benefits of resistance exercise on muscular strength and endurance, functional capacity and quality of life (Garber et al., 2011), the safety of resistance exercise on arterial stiffness indices and subsequent cardiovascular risk for individuals with unstable medical conditions has yet to be clearly established. Similar to aerobic exercise, cardiovascular risks associated with resistance exercise are likely determined by an individual's physical fitness and activity level, age, exercise intensity, and existing cardiovascular conditions (Williams et al., 2007). Specifically, resistance exercise is contraindicated in individuals with uncontrolled or high-risk, pre-existing cardiovascular conditions, highlighting the importance of vigilant patient screening and monitoring of strenuous exercise in at-risk populations prior to and during exercise (Williams et al., 2007). Future studies will elucidate the effect of acute resistance exercise on arterial stiffness and wave reflections in individuals with cardiovascular risk factors and CVD for improved cardiovascular health.
In conclusion, distinct arterial stiffness recovery responses were identified following a single acute bout of aerobic and resistance exercise. Overall, acute aerobic exercise did not change cf-PWV but resulted in reduced AIx and increased AIx75. In contrast, acute resistance was likely to induce an adversarial effect on arterial stiffness with overall increases in both cf-PWV and AIx75, potentially arising from cardiovascular and non-cardiovascular factors. Common limitations of current research designs, including great diversity in exercise protocols, selective timing of measurements, and lack of control group should be addressed in future studies to facilitate interpretation and improve generalizability of arterial stiffness findings to cardiovascular health.
All authors contributed ideas to the design of this study and developed the search strategy. DP conducted the literature search and the study quality assessment. All authors decided on the final selection of studies to be included in this review and meta-analysis. DP extracted study information and outcome data. KD performed the statistical analyses. DP developed the first paper draft, and all authors revised the manuscript for important intellectual content and approved the final version of the article.
The authors declare that the research was conducted in the absence of any commercial or financial relationships that could be construed as a potential conflict of interest.
The Supplementary Material for this article can be found online at: https://www.frontiersin.org/articles/10.3389/fphys.2018.00073/full#supplementary-material
Supplementary Figure 1. The PubMed search strategy employed in the current review.
Supplementary Figure 2. Funnel plot analyses for studies investigating cf-PWV (A). AIx (B) and AIx75 (C).
Supplementary Table 1. Risk of Bias summary.
AIx, Augmentation index; AIx75, Augmentation index corrected for heart rate of 75 beats per minute; cf-PWV, Carotid-femoral pulse wave velocity; CVD, Cardiovascular disease; SD, Standard deviation.
Ashor, A. W., Lara, J., Siervo, M., Celis-Morales, C., and Mathers, J. C. (2014). Effects of exercise modalities on arterial stiffness and wave reflection: a systematic review and meta-analysis of randomized controlled trials. PLoS ONE 9:e11034. doi: 10.1371/journal.pone.0110034
Barnes, J. N., Trombold, J. R., Dhindsa, M., Lin, H.-F., and Tanaka, H. (2010). Arterial stiffening following eccentric exercise-induced muscle damage. J. Appl. Physiol. 109, 1102–1108. doi: 10.1152/japplphysiol.00548.2010
Boutcher, Y. N., Hopp, J. P., and Boutcher, S. H. (2011). Acute effect of a single bout of aerobic exercise on vascular and baroreflex function of young males with a family history of hypertension. J. Hum. Hypertens. 25, 311–319. doi: 10.1038/jhh.2010.62
Burr, J. F., Boulter, M., and Beck, K. (2015). Arterial stiffness results from eccentrically biased downhill running exercise. J. Sci. Med. Sport 18, 230–235. doi: 10.1016/j.jsams.2014.03.003
Campbell, R., Fisher, J. P., Sharman, J. E., McDonnell, B. J., and Frenneaux, M. P. (2011). Contribution of nitric oxide to the blood pressure and arterial responses to exercise in humans. J. Hum. Hypertens. 25, 262–270. doi: 10.1038/jhh.2010.53
Cavalcante, J. L., Lima, J. A., Redheuil, A., and Al-Mallah, M. H. (2011). Aortic stiffness: current understanding and future directions. J. Am. Coll. Cardiol. 57, 1511–1522. doi: 10.1016/j.jacc.2010.12.017
Chandrakumar, D., Boutcher, S. H., and Boutcher, Y. N. (2015). Acute exercise effects on vascular and autonomic function in overweight men. J. Sports Med. Phys. Fitness 55, 91–102.
Collier, S. R., Diggle, M. D., Heffernan, K. S., Kelly, E. E., Tobin, M. M., and Fernhall, B. (2010). Changes in arterial distensibility and flow-mediated dilation after acute resistance vs. aerobic exercise. J. Strength Cond. Res. 24, 2846–2852. doi: 10.1519/JSC.0b013e3181e840e0
Davies, J. I., and Struthers, A. D. (2003). Pulse wave analysis and pulse wave velocity: a critical review of their strengths and weaknesses. J. Hypertens. 21, 463–472. doi: 10.1097/00004872-200303000-00004
Doonan, R. J., Mutter, A., Egiziano, G., Gomez, Y.-H., and Daskalopoulou, S. S. (2013). Differences in arterial stiffness at rest and after acute exercise between young men and women. Hypertens. Res. 36, 226–231. doi: 10.1038/hr.2012.158
Doonan, R. J., Scheffler, P., Yu, A., Egiziano, G., Mutter, A., Bacon, S., et al. (2011). Altered arterial stiffness and subendocardial viability ratio in young healthy light smokers after acute exercise. PLoS ONE 6:e26151. doi: 10.1371/journal.pone.0026151
Egger, M., Zellweger-Zähner, T., Schneider, M., Junker, C., Lengeler, C., and Antes, G. (1997). Language bias in randomised controlled trials published in English and German. Lancet 350, 326–329. doi: 10.1016/S0140-6736(97)02419-7
Elkins, M. R., Herbert, R. D., Moseley, A. M., Sherrington, C., and Maher, C. (2010). Rating the quality of trials in systematic reviews of physical therapy interventions. Cardiopulm. Phys. Ther. J. 21, 20–26.
Fahs, C. A., Heffernan, K. S., and Fernhall, B. (2009). Hemodynamic and vascular response to resistance exercise with L-arginine. Med. Sci. Sports Exerc. 41, 773–779. doi: 10.1249/MSS.0b013e3181909d9d
Figueroa, A., and Vicil, F. (2011). Post-exercise aortic hemodynamic responses to low-intensity resistance exercise with and without vascular occlusion. Scand. J. Med. Sci. Sports 21, 431–436. doi: 10.1111/j.1600-0838.2009.01061.x
Franklin, S. S. (2008). Beyond blood pressure: arterial stiffness as a new biomarker of cardiovascular disease. J. Am. Soc. Hypertens. 2, 140–151. doi: 10.1016/j.jash.2007.09.002
Garber, C. E., Blissmer, B., Deschenes, M. R., Franklin, B. A., Lamonte, M. J., Lee, I. M., et al. (2011). Quantity and quality of exercise for developing and maintaining cardiorespiratory, musculoskeletal, and neuromotor fitness in apparently healthy adults: guidance for prescribing exercise. Med. Sci. Sports Exerc. 43, 1334–1359. doi: 10.1249/MSS.0b013e318213fefb
Gkaliagkousi, E., Gavriilaki, E., Nikolaidou, B., Triantafyllou, G., and Douma, S. (2014). Exercise-induced pulse wave velocity changes in untreated patients with essential hypertension: the effect of an angiotensin receptor antagonist. J. Clin. Hypertens. 16, 482–487. doi: 10.1111/jch.12340
Hallqvist, J., Möller, J., Ahlbom, A., Diderichsen, F., Reuterwall, C., de Faire, U., et al. (2000). Does heavy physical exertion trigger myocardial infarction? A case-crossover analysis nested in a population-based case-referent study. Am. J. Epidemiol. 151, 459–467. doi: 10.1093/oxfordjournals.aje.a010231
Hanssen, H., Nussbaumer, M., Moor, C., Cordes, M., Schindler, C., and Schmidt-Trucksäss, A. (2015). Acute effects of interval versus continuous endurance training on pulse wave reflection in healthy young men. Atherosclerosis 238, 399–406. doi: 10.1016/j.atherosclerosis.2014.12.038
Harris, A. D., McGregor, J. C., Perencevich, E. N., Furuno, J. P., Zhu, J., Peterson, D. E., et al. (2006). The use and interpretation of quasi-experimental studies in medical informatics. J. Am. Med. Inform. Assoc. 13, 16–23. doi: 10.1197/jamia.M1749
Heffernan, K. S., Jae, S. Y., and Fernhall, B. (2007a). Racial differences in arterial stiffness after exercise in young men. Am. J. Hypertens. 20, 840–845. doi: 10.1016/j.amjhyper.2007.03.015
Heffernan, K. S., Jae, S. Y., Echols, G. H., Lepine, N. R., and Fernhall, B. (2007b). Arterial stiffness and wave reflection following exercise in resistance-trained men. Med. Sci. Sports Exerc. 39, 842–848. doi: 10.1249/mss.0b013e318031b03c
Heffernan, K. S., Jae, S. Y., Edwards, D. G., Kelly, E. E., and Fernhall, B. (2007c). Arterial stiffness following repeated Valsalva maneuvers and resistance exercise in young men. Appl. Physiol. Nutr. Metab. 32, 257–264. doi: 10.1139/h06-107
Heffernan, K. S., Rossow, L., Jae, S. Y., Shokunbi, H. G., Gibson, E. M., and Fernhall, B. (2006). Effect of single-leg resistance exercise on regional arterial stiffness. Eur. J. Appl. Physiol. 98, 185–190. doi: 10.1007/s00421-006-0259-9
Heffernan, K., Collier, S., Kelly, E., Jae, S., and Fernhall, B. (2007d). Arterial stiffness and baroreflex sensitivity following bouts of aerobic and resistance exercise. Int. J. Sports Med. 28, 197–203. doi: 10.1055/s-2006-924290
Higgins, J., and Green, S. (eds.). (2011). Cochrane Handbook for Systematic Reviews of Interventions Version 5.1.0. Available online at: http://handbook-5-1.cochrane.org/ (updated March, 2011).
Hingorani, A. D., Cross, J., Kharbanda, R. K., Mullen, M. J., Bhagat, K., Taylor, M., et al. (2000). Acute systemic inflammation impairs endothelium-dependent dilatation in humans. Circulation 102, 994–999. doi: 10.1161/01.CIR.102.9.994
Hu, M., Yan, H., Ranadive, S. M., Agiovlasitis, S., Fahs, C. A., Atiq, M., et al. (2013). Arterial stiffness response to exercise in persons with and without Down syndrome. Res. Dev. Disabil. 34, 3139–3147. doi: 10.1016/j.ridd.2013.06.041
Hull, J. H., Ansley, L., Bolton, C. E., Sharman, J. E., Knight, R. K., Cockcroft, J. R., et al. (2011). The effect of exercise on large artery haemodynamics in cystic fibrosis. J. Cystic Fibros. 10, 121–127. doi: 10.1016/j.jcf.2010.12.001
Izzo, J. J. L. (2004). Arterial stiffness and the systolic hypertension syndrome. Curr. Opin. Cardiol. 19, 341–352. doi: 10.1097/01.hco.0000126581.89648.10
Jae, S. Y., Yoon, E. S., Jung, S. J., Jung, S. G., Park, S. H., Kim, B. S., et al. (2013). Effect of cardiorespiratory fitness on acute inflammation induced increases in arterial stiffness in older adults. Eur. J. Appl. Physiol. 113, 2159–2166. doi: 10.1007/s00421-013-2648-1
Kadic, A. J., Vucic, K., Dosenovic, S., Sapunar, D., and Puljak, L. (2016). Extracting data from figures with software was faster, with higher interrater reliability than manual extraction. J. Clin. Epidemiol. 74, 119–123. doi: 10.1016/j.jclinepi.2016.01.002
Kano, Y., Padilla, D. J., Behnke, B. J., Hageman, K. S., Musch, T. I., and Poole, D. C. (2005). Effects of eccentric exercise on microcirculation and microvascular oxygen pressures in rat spinotrapezius muscle. J. Appl. Physiol. 99, 1516–1522. doi: 10.1152/japplphysiol.00069.2005
Kelly, R. P., Millasseau, S. C., Ritter, J. M., and Chowienczyk, P. J. (2001). Vasoactive drugs influence aortic augmentation index independently of pulse-wave velocity in healthy men. Hypertension 37, 1429–1433. doi: 10.1161/01.HYP.37.6.1429
Kingsley, J. D. P. D., Mayo, X. M. S., Tai, Y. L. M. S., and Fennell, C. M. S. (2016). Arterial stiffness and autonomic modulation following free-weight resistance exercises in resistance trained individuals. J. Strength Cond. Res. 30, 3373–3380. doi: 10.1519/JSC.0000000000001461
Kingsley, J. D., Tai, Y. L., Vaughan, J. A., and Mayo, X. (2017). High-intensity interval cycling exercise on wave reflection and pulse wave velocity. J. Strength Cond. Res. 31, 1313–1320. doi: 10.1519/JSC.0000000000001598
Kingwell, B. A., Berry, K. L., Cameron, J. D., Jennings, G. L., and Dart, A. M. (1997). Arterial compliance increases after moderate-intensity cycling. Am. J. Physiol. 273(5 Pt 2), H2186–H2191. doi: 10.1152/ajpheart.1997.273.5.H2186
Kobayashi, R., Hatakeyama, H., Hashimoto, Y., and Okamoto, T. (2017). Acute effects of different aerobic exercise duration on pulse wave velocity in healthy young men. J. Sports Med. Phys. Fitness 57, 1695–1701. doi: 10.23736/S0022-4707.16.06894-8
Kraemer, W. J., Fleck, S. J., Maresh, C. M., Ratamess, N. A., Gordon, S. E., Goetz, K. L., et al. (1999). Acute hormonal responses to a single bout of heavy resistance exercise in trained power lifters and untrained men. Can. J. Appl. Physiol. 24, 524–537. doi: 10.1139/h99-034
Lane, A., Ranadive, S., Yan, H., Kappus, R., Cook, M., Sun, P., et al. (2013). Effect of sex on wasted left ventricular effort following maximal exercise. Int. J. Sports Med. 34, 770–776. doi: 10.1055/s-0032-1329990
Lefferts, W. K., Heffernan, K. S., Hultquist, E. M., Fehling, P. C., and Smith, D. L. (2015). Vascular and central hemodynamic changes following exercise-induced heat stress. Vasc. Med. 20, 222–229. doi: 10.1177/1358863X14566430
Li, Y., Hanssen, H., Cordes, M., Rossmeissl, A., Endes, S., and Schmidt-Trucksäss, A. (2015). Aerobic, resistance and combined exercise training on arterial stiffness in normotensive and hypertensive adults: a review. Eur. J. Sport Sci. 15, 443–457. doi: 10.1080/17461391.2014.955129
Liberati, A., Altman, D. G., Tetzlaff, J., Mulrow, C., Gøtzsche, P. C., Ioannidis, J. P. A., et al. (2009). The PRISMA statement for reporting systematic reviews and meta-analyses of studies that evaluate healthcare interventions: explanation and elaboration. BMJ 339:b2700. doi: 10.1136/bmj.b2700
Lin, H. F., Tung, K., Chou, C. C., Lin, C. C., Lin, J. G., and Tanaka, H. (2016). Panax ginseng and salvia miltiorrhiza supplementation abolishes eccentric exercise-induced vascular stiffening: a double-blind randomized control trial. BMC Complement. Altern. Med. 16:168. doi: 10.1186/s12906-016-1139-4
London, G. M., and Pannier, B. (2010). Arterial functions: how to interpret the complex physiology. Nephrol. Dial. Transplant. 25, 3815–3823. doi: 10.1093/ndt/gfq614
Lydakis, C., Momen, A., Blaha, C., Gugoff, S., Gray, K., Herr, M., et al. (2008). Changes of central haemodynamic parameters during mental stress and acute bouts of static and dynamic exercise. J. Hum. Hypertens. 22, 320–328. doi: 10.1038/jhh.2008.4
MacDougall, J. D., Tuxen, D., Sale, D. G., Moroz, J. R., and Sutton, J. R. (1985). Arterial blood pressure response to heavy resistance exercise. J. Appl. Physiol. 58, 785–790. doi: 10.1152/jappl.1985.58.3.785
Madhura, M., and Sandhya, T. (2014). Effect of different phases of menstrual cycle on reflection index, stiffness index and pulse wave velocity in healthy subjects. J. Clin. Diagn. Res. 8, BC01–BC04. doi: 10.7860/JCDR/2014/7385.4778
Mak, W. Y. V., and Lai, W. K. C. (2015). Acute effect on arterial stiffness after performing resistance exercise by using the Valsalva Manoeuvre during exertion. Biomed. Res. Int. 2015, 1–5. doi: 10.1155/2015/343916
Mangoni, A. A., Mircoli, L., Giannattasio, C., Ferrari, A. U., and Mancia, G. (1996). Heart rate-dependence of arterial distensibility in vivo. J. Hypertens. 14, 897–901. doi: 10.1097/00004872-199607000-00013
Mattace-Raso, F. U. S., Van Der Cammen, T. J. M., Hofman, A., Van Popele, N. M., Bos, M. L., Schalekamp, M. A. D. H., et al. (2006). Arterial stiffness and risk of coronary heart disease and stroke: the Rotterdam Study. Circulation 113, 657–663. doi: 10.1161/CIRCULATIONAHA.105.555235
McCartney, N. (1999). Acute responses to resistance training and safety. Med. Sci. Sports Exerc. 31, 31–37. doi: 10.1097/00005768-199901000-00007
McEniery, C. M., Yasmin Hall, I. R., Qasem, A., Wilkinson, I. B., Cockcroft, J. R., et al. (2005). Normal vascular aging: differential effects on wave reflection and aortic pulse wave velocity: the Anglo-Cardiff Collaborative Trial (ACCT). J. Am. Coll. Cardiol. 46, 1753–1760. doi: 10.1016/j.jacc.2005.07.037
McHugh, M. L. (2012). Interrater reliability: the Kappa statistic. Biochem. Med. 22, 276–282. doi: 10.11613/BM.2012.031
Melo, X., Fernhall, B., Santos, D. A., Pinto, R., Pimenta, N. M., Sardinha, L. B., et al. (2016). The acute effect of maximal exercise on central and peripheral arterial stiffness indices and hemodynamics in children and adults. Appl. Physiol. Nutr. Metab. 41, 266–276. doi: 10.1139/apnm-2015-0204
Milatz, F., Ketelhut, S., and Ketelhut, R. G. (2015). Favorable effect of aerobic exercise on arterial pressure and aortic pulse wave velocity during stress testing. Vasa 44, 271–276. doi: 10.1024/0301-1526/a000441
Mitchell, G. F., Parise, H., Benjamin, E. J., Larson, M. G., Keyes, M. J., Vita, J. A., et al. (2004). Changes in arterial stiffness and wave reflection with advancing age in healthy men and women: the Framingham Heart Study. Hypertension 43, 1239–1245. doi: 10.1161/01.HYP.0000128420.01881.aa
Moore, S. M., Berrones, A. J., Clasey, J. L., Abel, M. G., and Fleenor, B. S. (2016). Arterial hemodynamics are impaired at rest and following acute exercise in overweight young men. Vasc. Med. 21, 497–505. doi: 10.1177/1358863X16666692
Munir, S., Jiang, B., Guilcher, A., Brett, S., Redwood, S., Marber, M., et al. (2008). Exercise reduces arterial pressure augmentation through vasodilation of muscular arteries in humans. J. Physiol. Heart Circ. Physiol. 294, 1645–1650. doi: 10.1152/ajpheart.01171.2007
Mutter, A. F., Cooke, A. B., Saleh, O., Gomez, Y.-H., and Daskalopoulou, S. S. (2016). A systematic review on the effect of acute aerobic exercise on arterial stiffness reveals a differential response in the upper and lower arterial segments. Hypertens. Res. 16, 1–27. doi: 10.1038/hr.2016.111
Okamoto, T., Masuhara, M., and Ikuta, K. (2009). Upper but not lower limb resistance training increases arterial stiffness in humans. Eur. J. Appl. Physiol. 107, 127–134. doi: 10.1007/s00421-009-1110-x
Perdomo, S. J., Moody, A. M., McCoy, S. M., Barinas-Mitchell, E., Jakicic, J. M., and Gibbs, B. B. (2016). Effects on carotid-femoral pulse wave velocity 24 h post exercise in young healthy adults. Hypertens. Res. 39, 435–439. doi: 10.1038/hr.2015.161
Pereira, T., Correia, C., and Cardoso, J. (2015). Novel methods for pulse wave velocity measurement. J. Med. Biol. Eng. 35, 555–565. doi: 10.1007/s40846-015-0086-8
Peres, P., Bernardelli, G. F., Mendes, C. C., Fischer, S. S., Servantes, D. M., Medeiros, W. M., et al. (2010). Immediate effects of submaximal effort on pulse wave velocity in patients with Marfan syndrome. Braz. J. Med. Biol. Res. 43, 397–402. doi: 10.1590/S0100-879X2010007500021
Ranadive, S. M., Fahs, C. A., Yan, H., Rossow, L. M., Agiovlasitis, S., and Fernhall, B. (2012). Comparison of the acute impact of maximal arm and leg aerobic exercise on arterial stiffness. Eur. J. Appl. Physiol. 112, 2631–2635. doi: 10.1007/s00421-011-2238-z
Rehman, A., Rahman, A., and Rasool, A. (2002). Effect of angiotensin II on pulse wave velocity in humans is mediated through angiotensin II type 1 (AT(1)) receptors. J. Hum. Hypertens. 16, 261–266. doi: 10.1038/sj.jhh.1001372
Ribeiro, F., Oliveira, N. L., Pires, J., Alves, A. J., and Oliveira, J. (2014). Treadmill walking with load carriage increases aortic pressure wave reflection. Rev. Port. Cardiol. 33, 425–430. doi: 10.1016/j.repc.2013.11.012
Salvi, P., Palombo, C., Salvi, G. M., Labat, C., Parati, G., and Benetos, A. (2013). Left ventricular ejection time, not heart rate, is an independent correlate of aortic pulse wave velocity. J. Appl. Physiol. 115, 1610–1617. doi: 10.1152/japplphysiol.00475.2013
Sharman, J. E., McEniery, C. M., Campbell, R. I., Coombes, J. S., Wilkinson, I. B., and Cockcroft, J. R. (2005). The effect of exercise on large artery haemodynamics in healthy young men. Eur. J. Clin. Invest. 35, 738–744. doi: 10.1111/j.1365-2362.2005.01578.x
Sharman, J. E., McEniery, C. M., Campbell, R., Pusalkar, P., Wilkinson, I. B., Coombes, J. S., et al. (2008). Nitric oxide does not significantly contribute to changes in pulse pressure amplification during light aerobic exercise. Hypertension 51, 856–861. doi: 10.1161/HYPERTENSIONAHA.107.102558
Shirwany, N. A., and Zou, M.-h. (2010). Arterial stiffness: a brief review. Acta Pharmacol. Sin. 31, 1267–1276. doi: 10.1038/aps.2010.123
Siasos, G., Athanasiou, D., Terzis, G., Stasinaki, A., Oikonomou, E., Tsitkanou, S., et al. (2016a). The acute impact of different types of aerobic exercise on arterial wave reflections and inflammation. Cardiology 135, 81–86. doi: 10.1159/000445993
Siasos, G., Athanasiou, D., Terzis, G., Stasinaki, A., Oikonomou, E., Tsitkanou, S., et al. (2016b). Acute effects of different types of aerobic exercise on endothelial function and arterial stiffness. Eur. J. Prev. Cardiol. 23, 1565–1572. doi: 10.1177/2047487316647185
Stenberg, J., Astrand, P., Ekblom, B., Royce, J., and Saltin, B. (1967). Hemodynamic response to work with different muscle groups, sitting and supine. J. Appl. Physiol. 22, 61–70. doi: 10.1152/jappl.1967.22.1.61
Sterne, J. A., and Egger, M. (2001). Funnel plots for detecting bias in meta-analysis: guidelines on choice of axis. J. Clin. Epidemiol. 54, 1046–1055. doi: 10.1016/S0895-4356(01)00377-8
Sugawara, J., Komine, H., Miyazawa, T., Imai, T., and Ogoh, S. (2015). Influence of single bout of aerobic exercise on aortic pulse pressure. Eur. J. Appl. Physiol. 115, 739–746. doi: 10.1007/s00421-014-3061-0
Sun, P., Yan, H., Ranadive, S. M., Lane, A. D., Kappus, R. M., Bunsawat, K., et al. (2015). Blood pressure changes following aerobic exercise in Caucasian and Chinese descendants. Int. J. Sports Med. 36, 189–196. doi: 10.1055/s-0034-1390493
Tai, Y. L., Gerhart, H., Mayo, X., and Kingsley, J. D. (2018). Acute resistance exercise using free weights on aortic wave reflection characteristics. Clin. Physiol. Funct. Imaging 38, 145–150. doi: 10.1111/cpf.12396
Thiebaud, R. S., Fahs, C. A., Rossow, L. M., Loenneke, J. P., Kim, D., Mouser, J. G., et al. (2016). Effects of age on arterial stiffness and central blood pressure after an acute bout of resistance exercise. Eur. J. Appl. Physiol. 116, 39–48. doi: 10.1007/s00421-015-3242-5
Vlachopoulos, C., Aznaouridis, K., and Stefanadis, C. (2010). Prediction of cardiovascular events and all-cause mortality with arterial stiffness: a systematic review and meta-analysis. J. Am. Coll. Cardiol. 55, 1318–1327. doi: 10.1016/j.jacc.2009.10.061
Vlachopoulos, C., Dima, I., Aznaouridis, K., Vasiliadou, C., Ioakeimidis, N., Aggeli, C., et al. (2005). Acute systemic inflammation increases arterial stiffness and decreases wave reflections in healthy individuals. Circulation 112, 2193–2200. doi: 10.1161/CIRCULATIONAHA.105.535435
Williams, M. A., Haskell, W. L., Ades, P. A., Amsterdam, E. A., Bittner, V., Franklin, B. A., et al. (2007). Resistance exercise in individuals with and without cardiovascular disease: 2007 update: a scientific statement from the American Heart Association Council on Clinical Cardiology and Council on Nutrition, Physical Activity, and Metabolism. Circulation 116, 572–584. doi: 10.1161/CIRCULATIONAHA.107.185214
Willich, S. N., Lewis, M., Lowel, H., Arntz, H.-R., Schubert, F., and Schroder, R. (1993). Physical exertion as a trigger of acute myocardial infarction. N. Engl. J. Med. 329, 1684–1690. doi: 10.1056/NEJM199312023292302
Yan, H., Ranadive, S. M., Heffernan, K. S., Lane, A. D., Kappus, R. M., Cook, M. D., et al. (2014). Hemodynamic and arterial stiffness differences between African-Americans and Caucasians after maximal exercise. Am. J. Physiol. Heart Circ. Physiol. 306, H60–68. doi: 10.1152/ajpheart.00710.2013
Yan, H., Ranadive, S. M., Lane-Cordova, A. D., Kappus, R. M., Behun, M. A., Cook, M. D., et al. (2017). The effect of acute aerobic exercise and histamine receptor blockade on arterial stiffness in African-Americans and Caucasians. J. Appl. Physiol. 122, 386–339. doi: 10.1152/japplphysiol.01115.2015
Yasmin McEniery, C. M., Wallace, S., Mackenzie, I. S., Cockcroft, J. R., and Wilkinson, I. B. (2004). C-reactive protein Is associated with arterial stiffness in apparently healthy individuals. Arterioscler. Thromb. Vasc. Biol. 24, 969–974. doi: 10.1161/01.ATV.zhq0504.0173
Yoon, E., Jung, S., Cheun, S., Oh, Y., Kim, S., and Jae, S. (2010). Effects of acute resistance exercise on arterial stiffness in young men. Korean Circ. J. 40, 16–22. doi: 10.4070/kcj.2010.40.1.16
Keywords: carotid-femoral pulse wave velocity, augmentation index, aerobic exercise, resistance exercise, cardiovascular health
Citation: Pierce DR, Doma K and Leicht AS (2018) Acute Effects of Exercise Mode on Arterial Stiffness and Wave Reflection in Healthy Young Adults: A Systematic Review and Meta-Analysis. Front. Physiol. 9:73. doi: 10.3389/fphys.2018.00073
Received: 09 November 2017; Accepted: 22 January 2018;
Published: 13 February 2018.
Edited by:
Jun Sugawara, National Institute of Advanced Industrial Science and Technology, JapanReviewed by:
Takanobu Okamoto, Nippon Sport Science University, JapanCopyright © 2018 Pierce, Doma and Leicht. This is an open-access article distributed under the terms of the Creative Commons Attribution License (CC BY). The use, distribution or reproduction in other forums is permitted, provided the original author(s) and the copyright owner are credited and that the original publication in this journal is cited, in accordance with accepted academic practice. No use, distribution or reproduction is permitted which does not comply with these terms.
*Correspondence: Anthony S. Leicht, YW50aG9ueS5sZWljaHRAamN1LmVkdS5hdQ==
Disclaimer: All claims expressed in this article are solely those of the authors and do not necessarily represent those of their affiliated organizations, or those of the publisher, the editors and the reviewers. Any product that may be evaluated in this article or claim that may be made by its manufacturer is not guaranteed or endorsed by the publisher.
Research integrity at Frontiers
Learn more about the work of our research integrity team to safeguard the quality of each article we publish.