- 1Center of Excellence for Poultry Science, University of Arkansas, Fayetteville, AR, United States
- 2Department of Animal and Avian Sciences, University of Maryland, College Park, MD, United States
- 3USDA, Agricultural Research Service, Fayetteville, AR, United States
Heat stress (HS) has been reported to alter fat deposition in broilers, however the underlying molecular mechanisms are not well-defined. The objectives of the current study were, therefore: (1) to determine the effects of acute (2 h) and chronic (3 weeks) HS on the expression of key molecular signatures involved in hepatic lipogenic and lipolytic programs, and (2) to assess if diet supplementation with dried Noni medicinal plant (0.2% of the diet) modulates these effects. Broilers (480 males, 1 d) were randomly assigned to 12 environmental chambers, subjected to two environmental conditions (heat stress, HS, 35°C vs. thermoneutral condition, TN, 24°C) and fed two diets (control vs. Noni) in a 2 × 2 factorial design. Feed intake and body weights were recorded, and blood and liver samples were collected at 2 h and 3 weeks post-heat exposure. HS depressed feed intake, reduced body weight, and up regulated the hepatic expression of heat shock protein HSP60, HSP70, HSP90 as well as key lipogenic proteins (fatty acid synthase, FASN; acetyl co-A carboxylase alpha, ACCα and ATP citrate lyase, ACLY). HS down regulated the hepatic expression of lipoprotein lipase (LPL) and hepatic triacylglycerol lipase (LIPC), but up-regulated ATGL. Although it did not affect growth performance, Noni supplementation regulated the hepatic expression of lipogenic proteins in a time- and gene-specific manner. Prior to HS, Noni increased ACLY and FASN in the acute and chronic experimental conditions, respectively. During acute HS, Noni increased ACCα, but reduced FASN and ACLY expression. Under chronic HS, Noni up regulated ACCα and FASN but it down regulated ACLY. In vitro studies, using chicken hepatocyte cell lines, showed that HS down-regulated the expression of ACCα, FASN, and ACLY. Treatment with quercetin, one bioactive ingredient in Noni, up-regulated the expression of ACCα, FASN, and ACLY under TN conditions, but it appeared to down-regulate ACCα and increase ACLY levels under HS exposure. In conclusion, our findings indicate that HS induces hepatic lipogenesis in chickens and this effect is probably mediated via HSPs. The modulation of hepatic HSP expression suggest also that Noni might be involved in modulating the stress response in chicken liver.
Introduction
Driven by economic demands and consumer preference for low fat and high protein sources, commercial broiler chickens have been intensively selected for high growth rate and high breast yield (Griffin and Goddard, 1994). Poultry meat and egg production has seen the largest increase during past decades and supports the livelihoods and food security of billions of people worldwide. However, this spectacular progress is also accompanied by several undesirable changes including hyperphagia (Denbow, 1994), metabolic disorders (Julian, 1998; Velleman, 2015), and hypersensitivity to high environmental temperature due to high metabolic activity and lack of sweat glands (Settar et al., 1999; Huang et al., 2015; Velleman, 2015).
Heat stress (HS) is detrimental to poultry production from its strong adverse effects on feed intake, growth, meat yield, welfare, and mortality (Dale and Fuller, 1980; Cahaner and Leenstra, 1992; Deeb and Cahaner, 2002; Deeb et al., 2002). HS resulted in an estimated total annual economic loss to the U.S. poultry industry of more than one-hundred million dollars and, therefore, represents a serious financial burden (St-Pierre et al., 2003). At the animal level, its adverse effects can range from discomfort (mild stress) to multiple organ damage and, under extreme conditions (sever stress), leads to mortality following spiraling hyperthermia. At the cellular level, HS induces many alterations such as, protein misfolding and aggregation (Ruan et al., 2017), cell cycle arrest (Trotter et al., 2001), redox state (Keyse and Emslie, 1992), and transcription modulation (Sanchez et al., 1994). Depending on the type, severity, and duration of the stress, cells can develop highly efficient stress response and protein quality control systems to ensure their survival or activate stress signaling cascades that proceed into cell-death pathways. These responses are controlled by a complex molecular network that is still not completely defined particularly in avian species. At the molecular level, a general rapid response to HS is increased synthesis of heat shock proteins (HSPs). Based on their monomeric molecular size, these ubiquitously expressed chaperones are classified into about six families, with HSP70 and HSP90 being the most extensively studied. Besides their classical roles as molecular chaperones and housekeepers (folding/unfolding, assembly/disassembly), HSPs are now understood to play a pivotal role in many cellular processes including transport and trafficking, protein degradation, and cell signaling (Drew et al., 2014). In turn, these cellular alterations induced by HS can lead to various neuroendocrine, physiological, and immunological adaptations including modulation of lipid and glucose metabolism (Sanz Fernandez et al., 2015; Victoria Sanz Fernandez et al., 2015).
The liver is a highly metabolic tissue that plays a vital role in digestion (bile production), metabolism, immunity, and storage of nutrients as well as detoxification. It has been shown that the liver is responsive and susceptible to HS (Lin et al., 2006; Tang et al., 2015) and that HS alters the hepatic lipid metabolism (Faylon et al., 2015; Dalvi et al., 2017; Jastrebski et al., 2017). Drinking Morinda citrifolia (Noni) juice has been shown to enhance hepatic antioxidant capacity, improve lipid homeostasis and protect the liver from environmental and chemical stressors (Wang et al., 2008a,b; Chang et al., 2013; Lin et al., 2017). The botanical name of this Rubiaceae plant is originally derived from the two Latin words “morus” ascribing to mulberry, and “indicus” imputing to Indian. It is known as Noni in Hawaii, mulberry in India, mengkudu in Malaysia, nhaut in Southeast Asia, painkiller bush or cheese fruit in Caribbean, Canary Wood in Australia, and many other names in different countries (for review see Potterat and Hamburger, 2007). This medicinal plant has been widely used in human nutrition and health (Issell et al., 2009). Due to the recent concerns in the sub-therapeutic use of antibiotics in food animal industries, Noni fruits and leaves have also gained considerable popularity in animal nutrition (Brooks et al., 2009; Sunder and Kundu, 2015; Sunder et al., 2015). However, the effect of Noni on chicken liver metabolism under control and HS conditions is not known.
As chicken liver is the main site for de novo fatty acid synthesis (lipogenesis) and also a site for fat storage, and as there is a subtle balance between hepatic lipogenesis and lipolysis, we aimed to determine in the present study the effects of Noni-enriched diet on growth performance, circulating metabolite and hormone levels as well as on the expression of hepatic lipogenesis- and lipolysis-related genes in broiler chickens exposed to acute and chronic HS.
Materials and Methods
Animals
One-day-old Cobb 500 by-product male chicks (480 total) were randomly assigned into 12 environmentally controlled chambers for rearing. Each chamber was composed of 2 pens (20 bird/pen) with their own feeder and water line. Each pen was given one of two diets: starter corn-soy based diet (Control, 3.9 Mcal ME Kg−1 and 21% CP), or control diet supplemented with 0.2% dried Noni plant (Noni, 3.9 Mcal ME Kg−1, 19.8% CP), a commonly incorporated levels by poultry producers in tropical regions (Sunder and Kundu, 2016). Chicks were given ad libitum access to feed and clean water. The ambient temperature was reduced gradually from 32°C to 24°C with relative humidity at 55 ± 5% for 21 days. On d 22, birds were allocated into two environmental conditions (thermoneutral TN, 24°C vs. heat stress HS, 35°C for 2 h or 3 weeks) and received two finisher diets (control, 3.9 Mcal ME kg−1 and 16.4% CP vs. Noni, 3.9 Mcal ME kg−1 and 16.8% CP) in a 2 × 2 factorial design. The day before the experiment, the chickens were equipped with a Thermochron temperature logger (iButton, DS1922L, Maxim, CA) for continuous monitoring of core body temperature. The environmental temperature and relative humidity (RH) were also continuously recorded in each chamber. Birds were humanely euthanized by cervical dislocation after acute (2 h) and chronic (3 week) HS. Blood samples were collected aseptically from wing veins using vacutainers with PST gel and lithium heparin (BD, NJ) at each time point and plasma was separated after centrifugation (1,500 g, 10 min, 4°C) and stored at −20°C for later analyses of circulating metabolites. Liver samples (n = 8/group per time point) were quickly isolated, snap frozen in liquid nitrogen, and stored at −80°C for subsequent gene and protein expression analyses. All experimental procedures involving animals used in this study were conducted in accordance with the recommendations in the guide for the care and use of laboratory animals of the National Institutes of Health and the protocol was reviewed and approved by the University of Arkansas Animal Care and Use Committee.
Cell Culture
Spontaneous immortalized chicken embryo liver cells (sim-CEL, Piekarski et al., 2014) were grown in Waymouth's media (Life Technology, Waltham, MA) supplemented with 10% fetal bovine serum (Life Technologies, Waltham, MA), and 1% penicillin/streptomycin (Biobasic, Amherst, NY) at 37°C under a humidified atmosphere of 5% CO2 and 95% air. At 80–90% confluency, cells were exposed to two environmental conditions (45°C vs. 37°C) and two treatments (50 μM quercetin, QCT vs. control) for 4 h. The dose of QCT was selected based on pilot and previous studies (Dok-Go et al., 2003; Martirosyan et al., 2016; Wang et al., 2017)
RNA Isolation, Reverse Transcription (RT), and Quantitative Real-Time PCR
Total RNA isolation from liver samples and sim-CEL cells was performed using Trizol® reagent (ThermoFisher Scientific, Rockford, IL) according to manufacturer's recommendations. RNA integrity and quality was assessed using 1% agarose gel electrophoresis and RNA concentrations and purity were determined for each sample by Take 3 Micro-Volume Plate using Synergy HT multi-mode micro plate reader (BioTek,Winooski, VT). The RNA samples were DNase treated and 1 μg RNA was reverse transcribed using qScript cDNA Synthesis Kit (Quanta Biosciences, Gaithersburg, MD) as we previously described (Rajaei-Sharifabadi H. et al., 2017). Real-time quantitative PCR (Applied Biosystems 7500 Real-Time PCR system) was performed as described previously (Rajaei-Sharifabadi H. et al., 2017) using 5 μL of 10X diluted cDNA, 0.5 μM of each forward and reverse specific primer, and SYBR Green Master Mix (ThermoFisher Scientific, Rockford, IL) in a total 20 μL reaction. Oligonucleotide primers used for chicken ATP citrate lyase (ACLY), acetyl-CoA carboxylase alpha (ACCα), fatty acid synthase (FASN), stearoyl-CoA desaturase (SCD-1), malic enzyme (ME), sterol regulatory element-binding protein 1 and 2 (SREBP-1/2), SREBP Cleavage-Activating Protein (SCAP), insulin induced gene 2 (INSIG2), lipoprotein lipase (LPL), hepatic triglyceride lipase (LIPC), adipose triglyceride lipase (ATGL), fatty acid translocase (CD36), peroxisome proliferator-activated receptor alpha and gamma (PPARα/γ), and ribosomal 18S as housekeeping gene were summarized in Table 1. The qPCR cycling conditions were the same as described in Lassiter et al. (2015). Relative expression of target genes was determined by the 2−ΔΔCt method (Schmittgen and Livak, 2008) and the group with control diet under thermoneutral condition was used as calibrator.
Protein Isolation and Western Blot Analysis
Liver tissues and sim-CEL cells were homogenized in lysis buffer as previously described (Lassiter et al., 2015). Total protein concentrations were determined using Synergy HT multi-mode microplate reader (BioTek, Winooski, VT) and a Bradford assay kit (Bio-Rad, Hercules, CA) with bovine serum albumin as a standard. Protein samples (30 μg for cells, 80 μg for tissues) were run on NuPAGE 4–12% Bis-Tris Gels (Life Technologies, Waltham, MA). The transferred membranes were blocked for 1 h at room temperature, and incubated with primary antibodies (diluted 1:1000) overnight at 4°C. The following polyclonal antibodies were used: rabbit anti-FASN (Novus Biologicals, Littleton, CO), rabbit anti-ACC, rabbit anti phospho-ACC (Cell Signaling Technologies, Danvers, MA), rabbit anti- ACLY (LSBio, Seattle, WA), mouse anti-HSP70 (ThermoFisher Scientific, Waltham, MA), rabbit anti-HSP90 (Thermo Fisher Scientific, Waltham, MA), goat polyclonal anti-HSP60 (Santa Cruz Biotechnology, Dallas, TX), and rabbit anti-GAPDH as housekeeping protein (Santa Cruz Biotechnology, Dallas, TX). The secondary HRP conjugated antibody, at a dilution of 1:5000, was used for 1 h at room temperature. Pre-stained molecular weight marker (Precision Plus Protein Dual Color) was used as a standard (BioRad, Hercules, CA). The signal was visualized by enhanced chemiluminescence (ECL plus; GE Healthcare Bio-Sciences, Buckinghamshire, UK) and captured by FluorChem M MultiFluor System (Proteinsimple, Santa Clara, CA). Image Acquisition and Analysis were performed by AlphaView software (Version 3.4.0, 1993–2011, Proteinsimple, Santa Clara, CA).
Immunofluorescence Staining
Immunofluorescence staining was performed as previously described (Dridi et al., 2012). Briefly, cells were grown in chamber slides and fixed with methanol for 20 min at −20°C. Cells were blocked with serum-free protein block (Dako, Carpinteria, CA) for 1 h at room temperature, and then incubated with primary antibodies (1:200, in Antibody Diluent, Dako, Carpinteria, CA) overnight at 4°C. The signal was visualized with DyLight 488- or 590-conjugated secondary antibody (Thermo Fisher Scientific, Grand Island, NY). Slides were cover slipped with a Vectashield with DAPI (Vector Laboratories, Burlingame, CA), and images were obtained and analyzed using Zeiss Imager M2 and AxioVision software (Carl Zeiss Microscopy).
Plasma Metabolite and Hormone Measurement
Plasma glucose, triglyceride, cholesterol, lactate dehydrogenase (LDH), and uric acid levels were measured using an automated spectrophotometer as we previously described (Nguyen et al., 2015). Plasma levels of total 3, 5, 3′-triiodothyronine (T3) and thyroxine (T4) were determined using coated tube radioimmunoassay kits (MP Biomedicals, Solon, OH) as we previously described (Rajaei-Sharifabadi H. et al., 2017).
Statistical Analysis
Growth performance (feed intake and body weight) data were analyzed by two-way repeated measures ANOVA. The rest of the data from both in vivo and in vitro experiments were analyzed by two-way ANOVA with diet (Control vs. Noni or QCT) and ambient temperature (TN vs. HS) as the main effects. If ANOVA revealed significant effects the means were compared by Tukey's multiple comparison test using GraphPad Prism version 6.00 for Windows, GraphPad Software (La Jolla, CA). Differences were considered significant at P < 0.05.
Results
Growth Performance
As depicted in Figure 1, there was no difference between all groups in daily individual or cumulative feed intake (Figures 1A,B) as well as in weekly BW and BW gain during the first 3 weeks of the experiment (Figures 1C,D). However, shortly after heat stress exposure (2 h), core body temperature was significantly increased by ~1°C in control group and Noni supplementation not only delayed this increase but also reduced it (Rajaei-Sharifabadi L. E. H. et al., 2017). During the acute HS exposure, feed intake was significantly reduced by 10% in control diet and 13% in Noni-fed group compared to their counterparts maintained under thermoneutral (TN) conditions (Rajaei-Sharifabadi L. E. H. et al., 2017). This reduction in feed intake was increased over the extended period of HS to reach 28 and 29% after 3 weeks of HS in control diet- and Noni-fed groups, respectively, compared to their counterparts maintained under TN conditions (Figures 1A,B). This, in turn, resulted in a significant reduction in BW by 38% in control diet and 40% in Noni-fed heat stressed groups compared to their TN counterparts (Figures 1C,D).
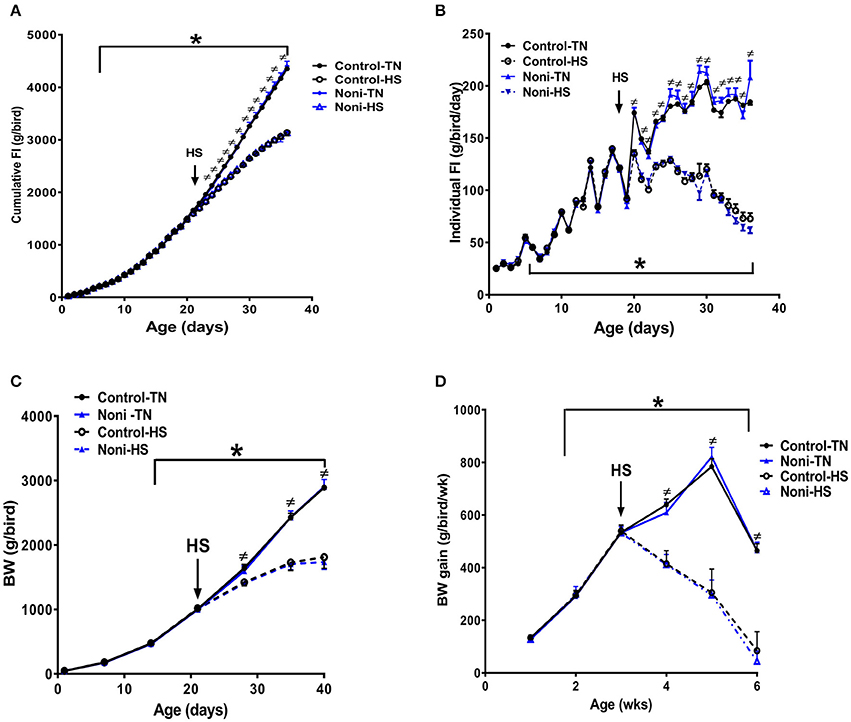
Figure 1. Effects of HS and Noni-enriched diet on feed intake and growth in broilers. HS depressed cumulative (A) and individual (B) feed intake which in turn results in a reduction of body weight (BW, C), and BW gain (D). Data are presented as mean ±SEM (n = 160). ≠ denote significant difference between TN and HS conditions at P < 0.05. *Indicate significant effect of time at P < 0.05.
Metabolites and Thyroid Hormone
Acute HS did not affect the plasma levels of all measured parameters (Rajaei-Sharifabadi L. E. H. et al., 2017), however chronic HS significantly increased plasma glucose and uric acid levels (Table 2), and significantly decreased that of T3 and T4 (Table 2). Plasma triglyceride, cholesterol, and LDH levels were not affected by HS (Table 2). Noni supplementation did not elicit any changes to these parameters (Table 2).
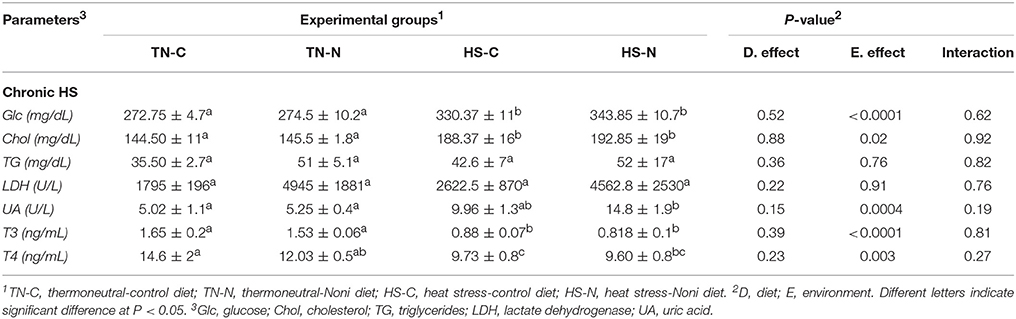
Table 2. Effects of Noni supplementation and HS on circulating metabolite and hormone levels in broiler chickens.
Heat Stress and Noni Modulate Hepatic HSP Expressions in a Time-Specific Manner
As shown in Figure 2, the hepatic expression of HSP60, HSP70, and HSP90 was significantly up-regulated after acute, but not after chronic heat stress (Figures 2A–H). During the acute experimental conditions and in comparison with the control diet, Noni supplementation did not affect the expression of all tested HSPs in the liver of TN group (Figures 2A–D), however it did down-regulate that of HSP90 in the HS group (Figure 2D). Under the chronic experimental conditions, Noni supplementation increased the hepatic expression of HSP60 in both TN and HS groups (Figures 2E,F) and that of HSP90 in the TN group only (Figure 2H). The hepatic expression of HSP70 remained unchanged between the control and Noni groups under both environmental (TN and HS) conditions (Figures 2E,G).
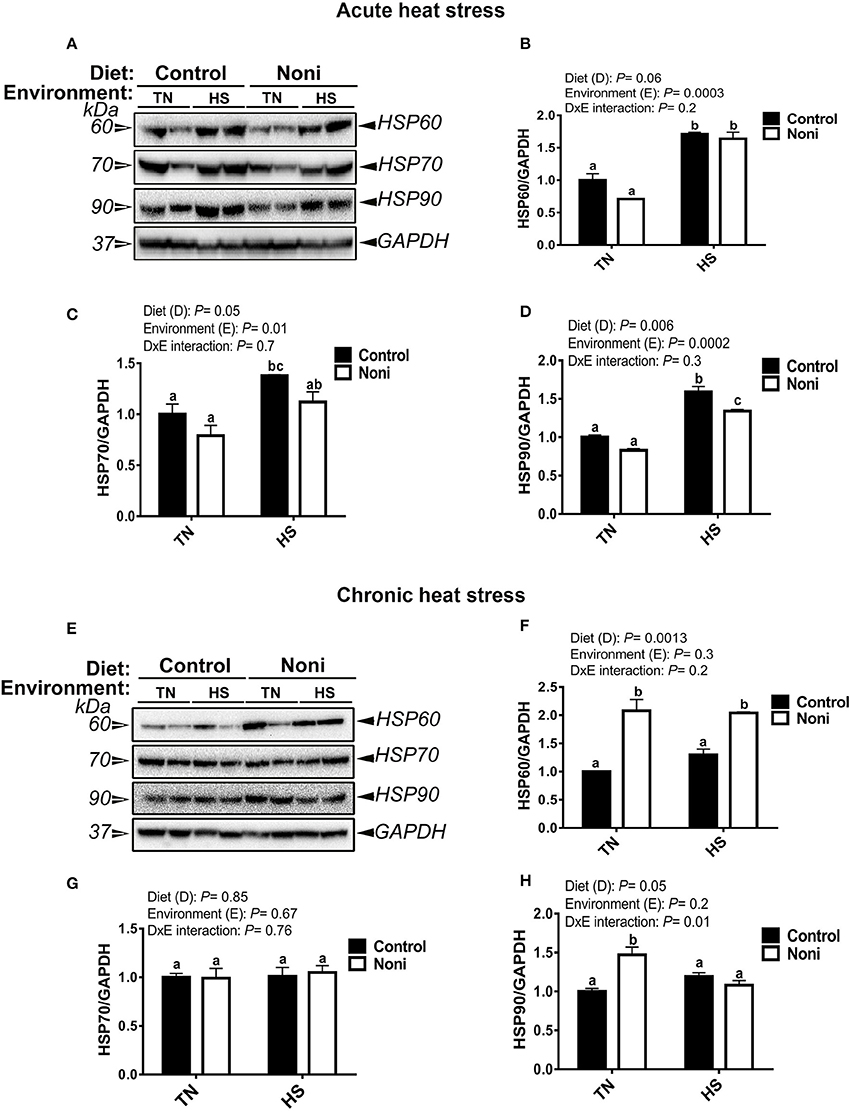
Figure 2. Effect of Noni supplementation on hepatic HSP expression in heat-stressed broilers. (A–D) acute HS and (E–H) chronic HS. HSP levels were determined by Western blot and their relative expression was presented as a normalized ratio to housekeeping GAPDH protein. Data are presented as mean ± SEM (n = 8/group). Different letters indicate significant difference at P < 0.05.
Heat Stress and Noni Supplementation Differentially Regulates the Hepatic Expression of Lipogenic Genes and Proteins
Under TN conditions and prior to HS, Noni supplementation significantly increased the protein levels of hepatic ACLY, but not that of ACCα and FASN compared to the control diet-fed group (Figures 3A–D). However, the mRNA abundances of these genes remained unchanged (Figures 3E–I). During acute HS, Noni supplementation significantly increased the protein levels of hepatic ACCα, but reduced that of FASN, and ACLY as well as the gene expression of hepatic ME and SCD-1 compared to the control diet-fed group (Figures 3A–I). Interestingly, when all groups were pooled together, acute HS significantly up-regulated the hepatic expression of ACCα, ACLY, and FASN, but it down-regulated the hepatic expression of all tested genes compared to TN group (Figures 3E–I). The induction of FASN and ACLY protein was more noticeable in the heat-stressed birds fed with control diet resulting in a significant interaction (P = 0.02 for FASN, P = 0.003 for ACLY) between environment and diet (Figures 3B–D).
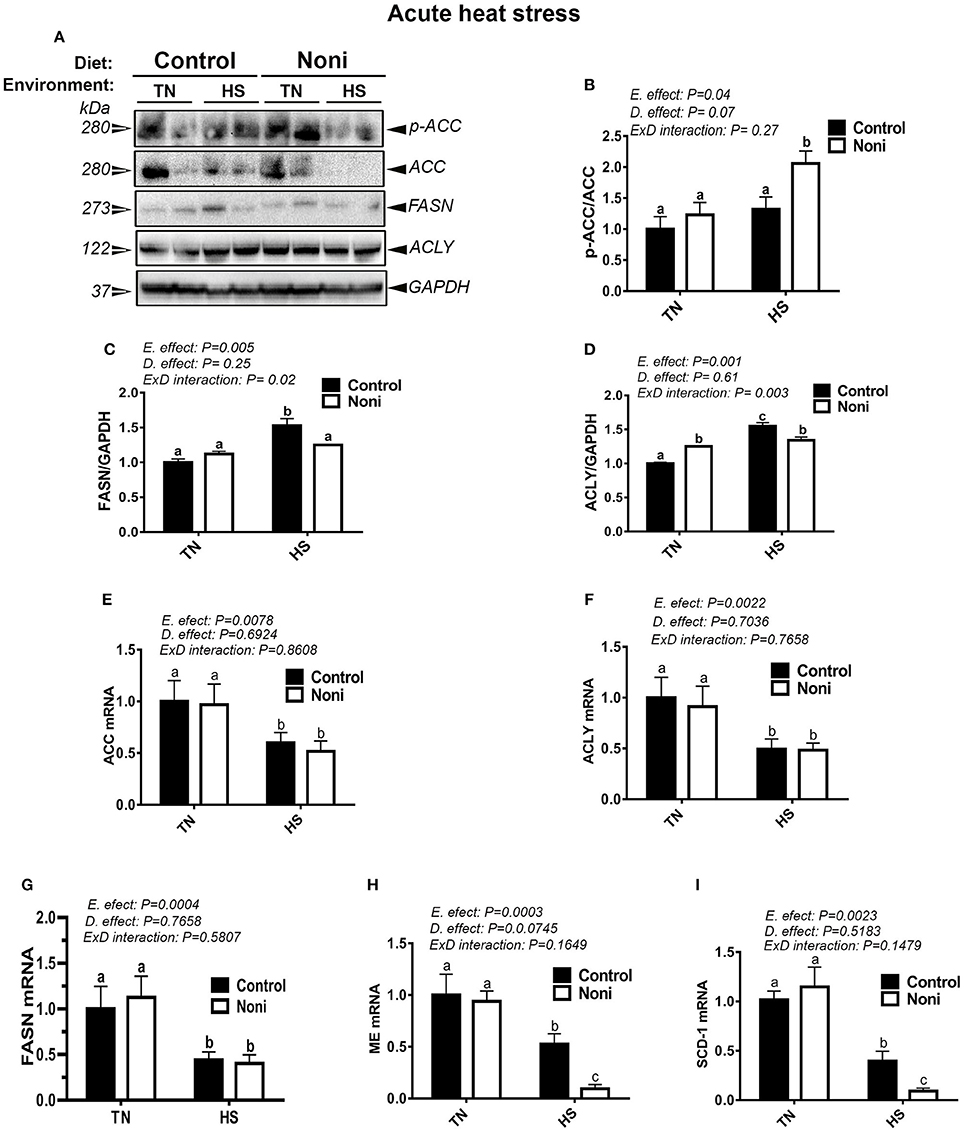
Figure 3. Effect of Noni supplementation on hepatic expression of lipogenic markers in acute heat-stressed broilers. Phosphorylated and pan levels of ACCα as well as FASN and ACLY were determined using Western blot (A) and their relative expression was presented as normalized ratio of phosphorylated/total target protein (B–D). Relative abundance of ACCα (E), ACLY (F), FASN (G), ME (H), and SCD-1 (I) mRNA was measured by real-time RT-PCR. Data are presented as mean ± SEM (n = 8/group). Different letters indicate significant difference at P < 0.05.
Similarly, during the chronic experimental conditions and prior to HS, Noni supplementation did not affect the mRNA abundance of all tested genes (Figures 4E–I) as well as that of hepatic ACCα, and ACLY protein (Figures 4A,B,D). However, the expression of FASN protein was significantly up-regulated in Noni compared to control group (Figure 4C). During chronic HS, Noni supplementation significantly increased the protein levels of hepatic ACCα and FASN, but decreased that of ACLY compared to control diet-fed group (Figures 4B–D). The mRNA levels of all tested genes did not differ between Noni- and control diet-fed groups reared under chronic HS (Figures 4E–I). Interestingly, when all groups were pooled together, chronic HS significantly down-regulated the hepatic expression of all tested genes (Figures 4A–I).
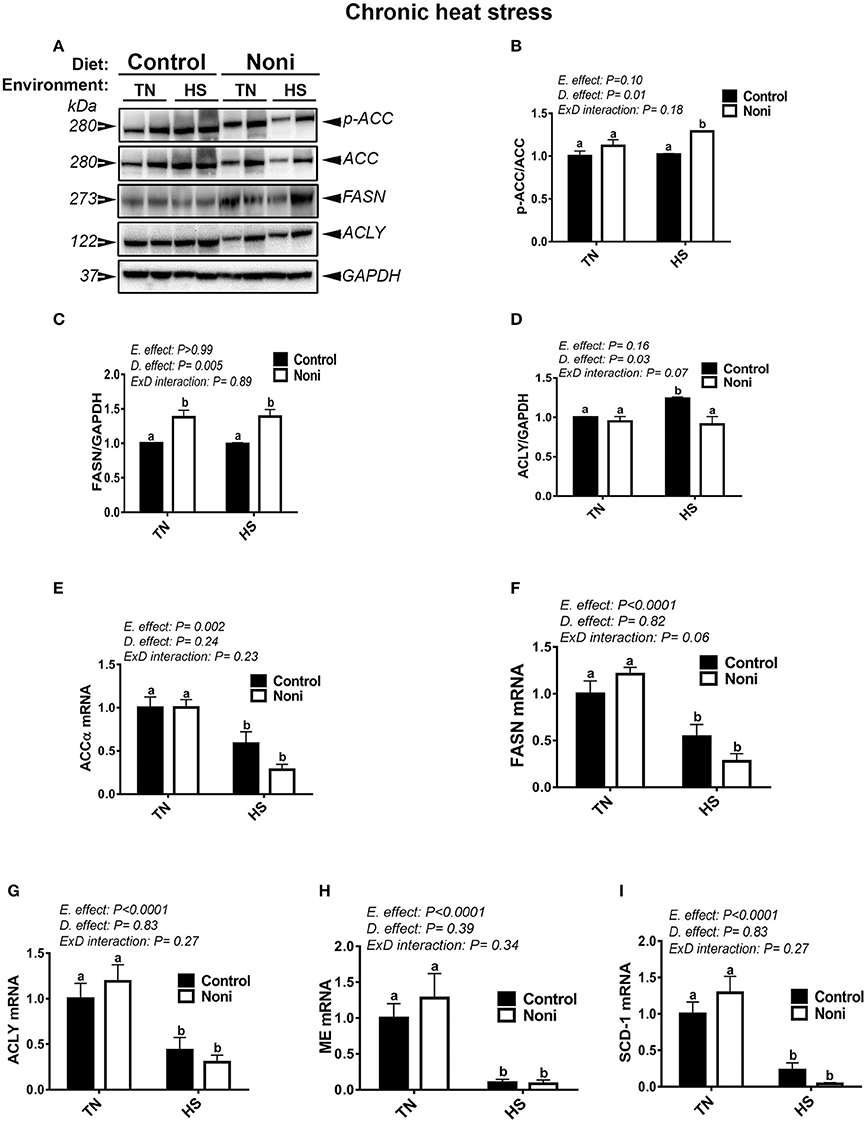
Figure 4. Effect of Noni supplementation on hepatic expression of lipogenic markers in chronic heat-stressed broilers. Phosphorylated and pan levels of ACCα as well as FASN and ACLY were determined using Western blot (A) and their relative expression was presented as normalized ratio of phosphorylated/total target protein (B–D). Relative abundance of ACCα (E), ACLY (F), FASN (G), ME (H), and SCD-1 (I) mRNA was measured by real-time RT-PCR. Data are presented as mean ± SEM (n = 8/group). Different letters indicate significant difference at P < 0.05.
Heat Stress Modulates the Hepatic Expression of Lipogenesis-Related Transcription Factors
As shown in Figure 5, both acute and chronic HS significantly down-regulated the hepatic expression of SREBP-1 and SCAP gene (Figures 5A,C,D,F), however it did not affect that of SREBP-2 (Figures 5B,E), INSIG-2, PPARα, or PPARγ (Table 3). Noni supplementation did not elicit any change to the expression of these transcription factors under both environmental (TN and HS) conditions (Figures 5A–F).
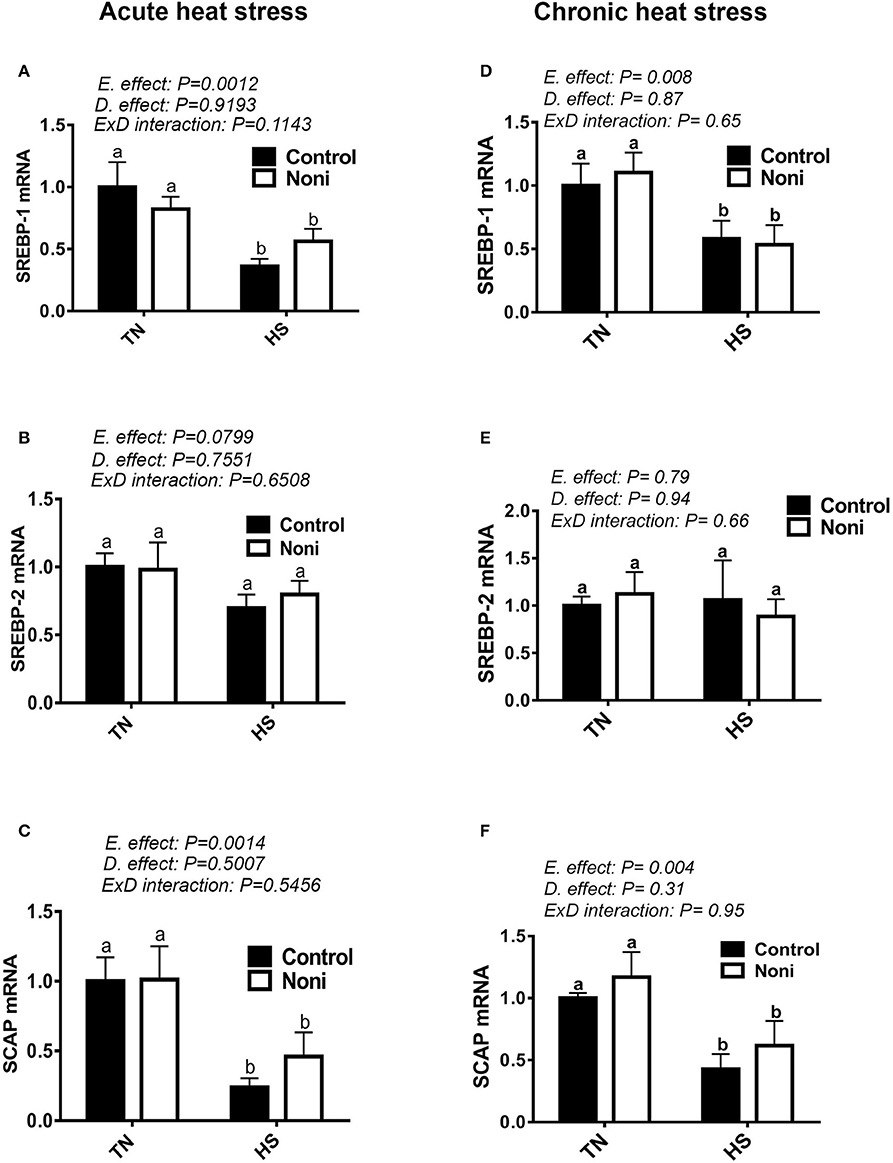
Figure 5. Effect of Noni on hepatic expression of lipogenesis-related transcription factors in heat stressed broilers. (A–C) acute HS and (D–F) chronic HS. Relative expression of SREBP-1 (A,D), SREBP-2 (B,E), and SCAP (C,F) was measured by real-time RT-PCR. Data are presented as mean ± SEM. D, diet; E, environment; TN, thermoneutral; HS, heat stress. Different letters indicate significant difference at p < 0.05.
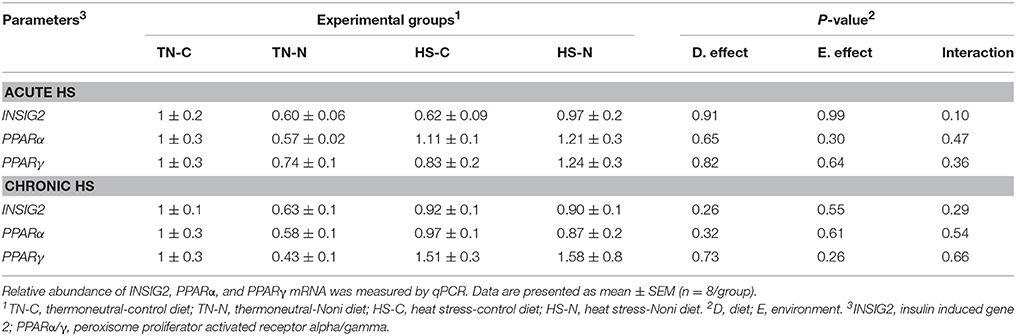
Table 3. Effects of Noni supplementation on hepatic expression of INSIG2, PPARα and PPARγ genes in heat stressed broiler chickens.
Heat Stress Modulates the Hepatic Expression of Lipolytic Genes
In comparison with the control diet, Noni supplementation did not affect the hepatic expression of LPL, ATGL, LIPC, and CD36 genes under the TN environment for both acute and chronic experimental conditions (Figures 6A–H). Under HS exposure, however, Noni significantly decreased the mRNA levels of hepatic ATGL in both acute and chronic experimental conditions (Figures 6B,F). When all groups were pooled together, both acute and chronic HS down-regulated the hepatic expression of LIPC and CD36 gene compared to TN conditions (Figures 6C,D,G,H). However, the hepatic LPL mRNA levels were significantly decreased only after chronic but not acute HS (Figures 6A,E). Interestingly, both acute and chronic HS up-regulated the hepatic expression of ATGL gene compared to TN condition (Figures 6B,F) and this increase was more pronounced in the control diet-fed group.
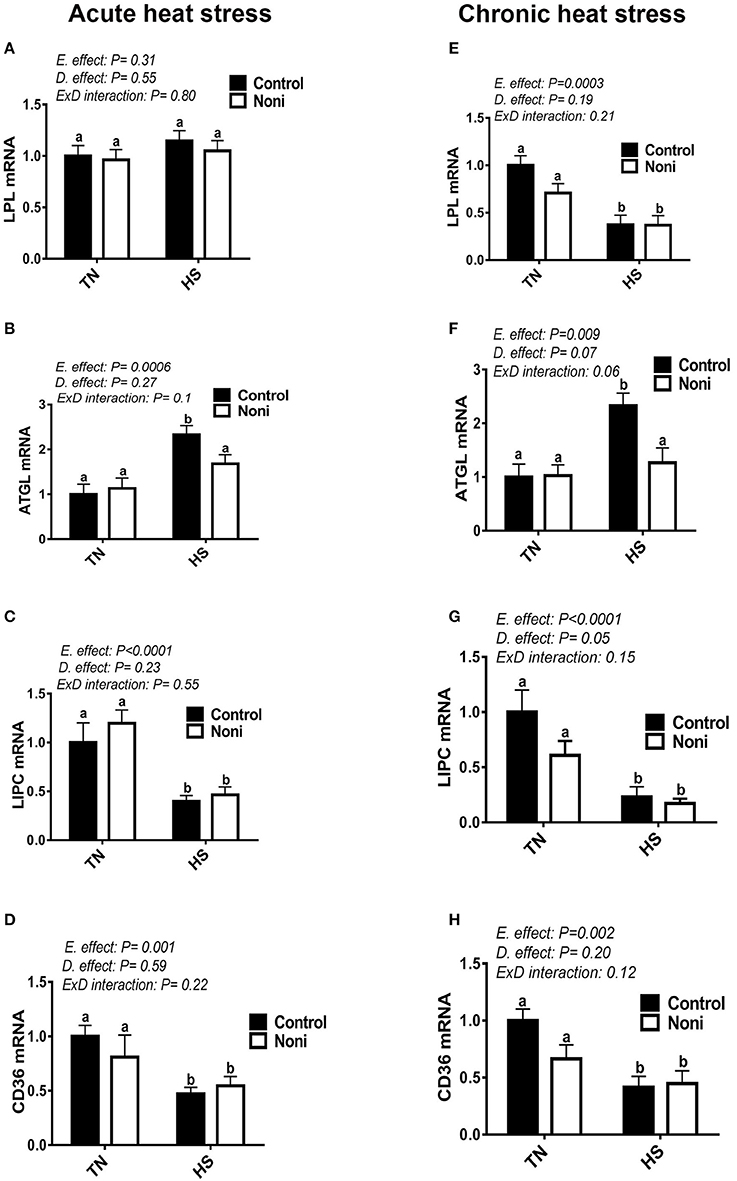
Figure 6. Effect of Noni supplementation on hepatic expression of lipolytic pathway in heat-stressed broilers. (A–D) acute HS, (E–H) chronic HS. Relative expression of LPL (A,E), ATGL (B,F), LIPC (C,G), and CD36 gene (D,H) was measured by real-time RT-PCR. Data are presented as mean ± SEM (n = 8/group). Different letters indicate significant difference at P < 0.05.
QCT, the Active Ingredient of Noni, Modulates Lipogenic Gene Expression in Sim-CEL Cells
HS significantly down-regulated the expression of lipogenic genes at mRNA and protein levels (Figures 7A–E, Table 4) as well as their related transcription factors SREBP-1, SREBP-2, and SCAP (Figure 7F, Table 4). However, HS did not alter the expression of LPL, ATGL, and LIPC (Table 4). The up-regulation of both HSP60 and HSP70, demonstrated by immunofluorescence staining (Figure 7E), indicated that sim-CEL cells were undergoing stress induced by heat load. QCT treatment significantly increased ACCα, FASN, and ACLY protein levels in control (37°C) environment only, however it did significantly reduced the phosphorylated levels of ACCα at Serine 79 site and increased that of ACLY protein levels under HS conditions (Figures 7B,D).
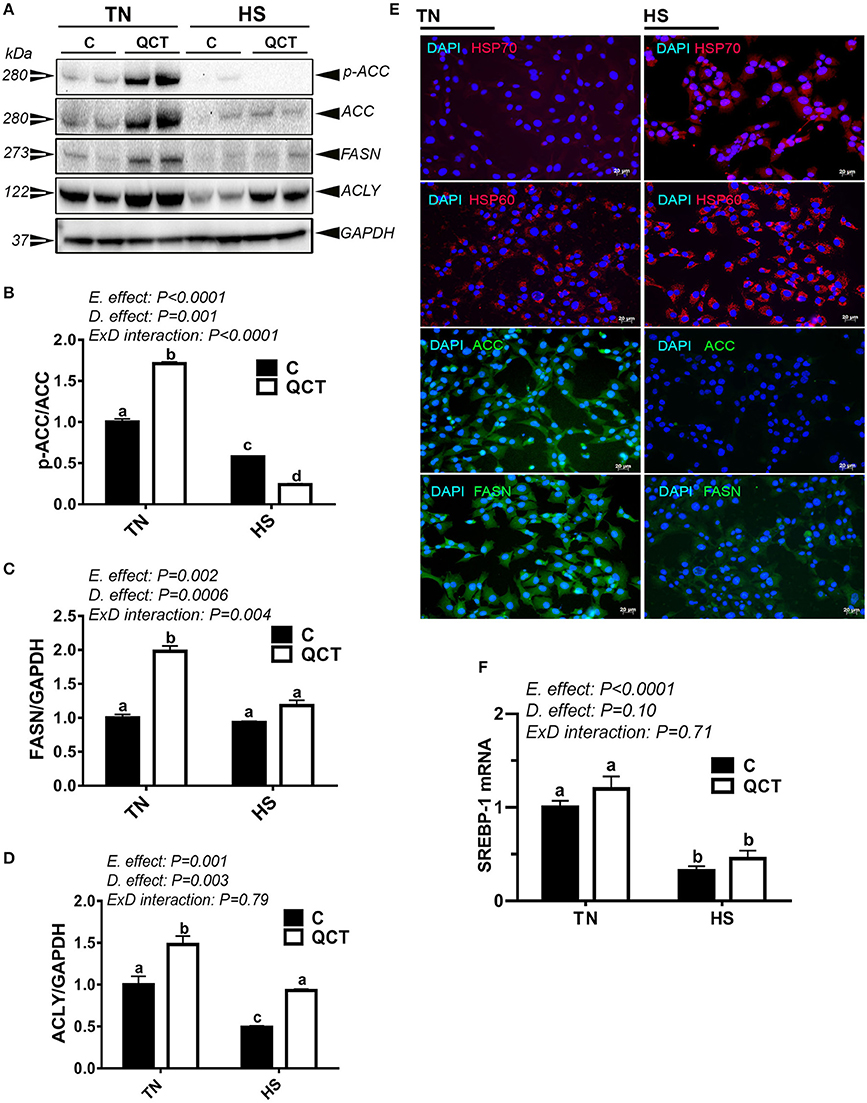
Figure 7. Effect of QCT on lipogenesis-related signatures and SREBP-1 gene expression in heat stressed sim-CEL cells. Phosphorylated and pan levels of ACCα as well as FASN and ACLY were determined using Western blot and their relative expression was presented as normalized ratio of phosphorylated/total target protein (A–D). Immunofluorescence staining (E) shows that HS induce HSP 60 and 70, and reduces ACCα and FASN protein levels in sim-CEL cells. SREBP-1 mRNA abundance was measured by real-time RT-PCR (F). Data are presented as mean ±SEM (n = 6/groups). Different letters indicate significant differences at P < 0.05. Western blotting figure is representative of 3 replicates.
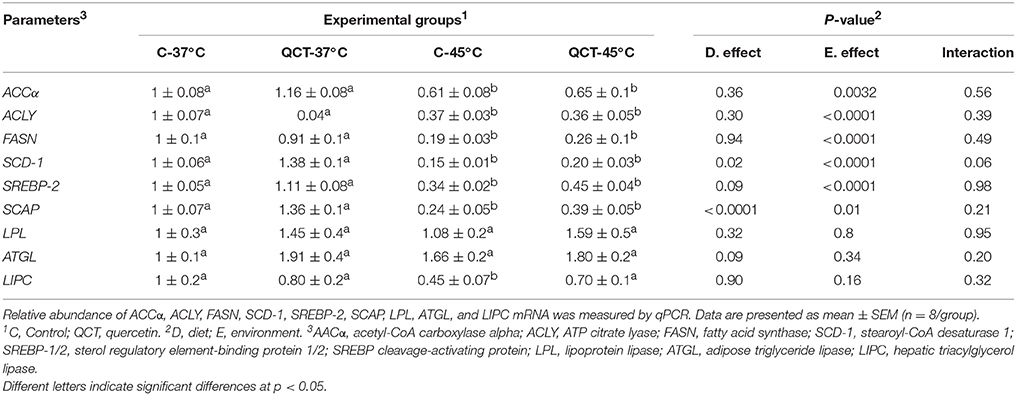
Table 4. Effects of quercetin (QCT) supplementation on hepatic expression of lipogenic-lipolytic genes in heat stressed Celi cells.
Discussion
HS adversely affects poultry production through depressed feed intake and growth as shown in many previous reports (Dale and Fuller, 1980; Cahaner and Leenstra, 1992; Leenstra and Cahaner, 1992; Deeb et al., 2002) and confirmed by the present study. These effects are mediated by numerous molecular, neuroendocrine, and physiological processes including nutrient portioning and lipid metabolism that are not completely defined, at least, in avian species.
As commercial broilers are fed lipid-poor diets (< 10%), the majority of the accumulated fat is derived from the liver which is the main site for lipogenesis (Goodridge and Ball, 1967; Leveille et al., 1968; Yeh and Leveille, 1971). Indeed, the avian liver is responsible for more than 90% of de novo fatty acid synthesis and is also a site of lipid storage in birds (Goodridge and Ball, 1967; Leveille et al., 1968; Yeh and Leveille, 1971). The increased levels of hepatic FASN and ACLY proteins, rate limiting enzymes in de novo fatty acid synthesis, indicates that HS induce hepatic lipogenesis in chickens which corroborates previous study reported by Geraert et al. (1996) and Jastrebski et al. (2017). However, the upregulation of p-ACCαSer79 by HS, which indicates that the enzyme is less active, is intriguing. Although it is not clear at this time-point why ACCα is down-regulated, it is possible that a compensatory increase in another ACC isoform such as, ACCβ (ACC2) is involved. Furthermore, as its regulation is complex, it is possible that the down regulation of ACCα might be due to a negative feedback loop. For instance, the ACCα decrease could result from an increase in glucagon and/or catecholamine levels which has been reported to be induced by stressful stimuli including HS and feed deprivation (Bloom et al., 1973; Iguchi et al., 2012). Although we didn't determine the plasma glucagon levels in the present study, the significant increase in circulating glucose concentration (23%) by HS, despite the significant reduction in feed intake, supports the above mentioned hypothesis. In fact, during HS along with reduced energy intake, glucagon plays a key role in maintaining glucose homeostasis via stimulating hepatic glycogenolysis and gluconeogenesis (Exton and Park, 1968; Garrison and Haynes, 1973; Watford, 1985) which is evidenced in our study by the upregulation of hepatic phosphoenolpyruvate carboxykinase (PEPCK), glycogene phosphorylase (GLGP), and fructose 2,6-biphosphatase 4 (PFKFB4) (data not shown). An additional possibility is that HS activates the energy sensor AMPKα1/2 (data not shown) and AMPK has been shown to phosphorylate ACCαSer79 (Park et al., 2002). We should note that we measured here only one ACC phosphorylation site (Ser79), however there are several other sites including Ser1200 and Ser1215 (Brownsey et al., 2006) that might be differently regulated and phosphorylated by HS.
HS down regulated the hepatic expression of all tested lipogenic genes as well as their related transcription factors which is different from previous integrated transcriptomic studies reported in rodents and chickens (Bhusari et al., 2007; Jastrebski et al., 2017). This discrepancy might due to the technique used and the choice of the normalization (housekeeping) control (real-time qPCR in our study vs. RNAseq or microarray in previous studies) and/or experimental conditions (species, age, duration, intensity, and/or severity of HS, diet composition, etc.). The absence of correlation between gene and protein expression in our study indicates that the transcription and translation of these target markers are differentially regulated by HS which is not surprising. It is plausible that under our experimental stress conditions, the pool of already synthesized lipogenic mRNA might be efficiently translated with a quick turnover or degradation, however its protein product accumulation increases and remain in the cellular pool due to its high half-life (Schwanhausser et al., 2011). The protein stability may also increase by post-translational modification like acetylation or glycosylation. An additional possibility is that mRNA translation can be upregulated by RNA binding proteins and/or down-regulation of micro (mi) RNA targeting that mRNA.
Interestingly, our study showed that the lipolytic pathway is also expressed in the chicken liver which is a considerable site for lipid storage and secretion (Hermier, 1997). HS seems to regulate the expression of hepatic lipolysis-related genes in a time-specific manner. For instance, LPL mRNA abundance was not affected by acute HS, but it was decreased by chronic HS. Both acute and chronic HS up regulate hepatic ATGL, but decreased LIPC expression which may explain the unchanged levels of plasma triglycerides. As LPL has other functions beside its hydrolytic activity, it is conceivable that HS might affect the maturation of plasma HDL (Strauss et al., 2001), uptake of lipoprotein-associated lipids and vitamins (Rinninger et al., 1998, 2001; Goti et al., 2002), and/or degradation of lipoprotein (Heeren et al., 2002) which warrant further investigations. Of particular interest, a novel function of LPL in the regulation of appetite and energy balance regulation has recently been postulated (Wang et al., 2011). Thus, it is possible that the reduced feed intake and body weight induced by HS in this study might be mediated via down regulation of LPL expression and this merit further in depth studies.
As expected and in agreement with previous studies (Xie et al., 2014; Wang et al., 2015), HSPs (HSP60, HSP70, and HSP90) were all upregulated by acute HS, but remained unchanged after longer HS exposure compared to TN conditions. This later observation is not surprising and indicates that the birds become acclimated to chronic HS which in turn results in no further increase in cellular HSPs (Givisiez et al., 1999). Noni supplementation reduced the expression of HSP90, but not that of HSP60 and HSP70-induced by acute HS suggesting that the regulation of HSP by Noni is family (molecular weight)-dependent. However, contrary to our expectation, Noni-enriched diet upregulated the hepatic expression of HSP60 and HSP90 in broilers maintained under TN conditions, as well as that of HSP60 in chronic heat-stressed broilers without affecting the expression of HSP70. The down regulation of hepatic HSP90 during acute HS supports the notion that Noni might alleviate stress induced by heat load and this has been substantiated by the reduction of core body temperature-induced by HS (data not shown). However, the upregulation of both HSP60 and HSP90 during the chronic but not acute TN conditions is intriguing. It has been reported that Noni juice induced stress and reduced body weight in mice (Muto et al., 2010). This is an unlikely scenario in our experimental conditions because birds fed control or Noni diet showed similar feed intake, body weight, and body weight gain without any sign of discomfort or stress (physical activity and behavior). In addition, necropsy analysis of all major organs in both groups revealed no pathology. Although the physiological meaning and biological determinants are not known at this time point, it is foreseeable that age (3 vs. 6 weeks in acute vs. chronic conditions, respectively) might impact the effect of Noni on the hepatic HSP60 expression. Age-dependent differences in liver gene expression has been recently reported (Uno et al., 2017) and HSP60 levels have been found to progressively decline with increasing age in humans (Rea et al., 2001). Besides their role in stress response, HSPs act also as molecular chaperones under normal physiological conditions where they carry out such essential functions as protein translocation, folding, and assembly (Hendrick and Hartl, 1993). It is, therefore, possible that HSP might mediate the effect of Noni in regulating these cellular processes, however owing to the gap in avian HSP biology, experimental evidence using mechanistic and functional studies supporting these possibilities needs to be provided.
The lipogenic proteins followed a similar hepatic expression pattern as HSPs across our experimental conditions. For instance, HSPs (HSP70 and HSP90), FASN, and ACLY showed similar expression patterns in the liver of acute heat-stressed broilers. Similarly, the hepatic expression of HSP60 and FASN follow the same trend under chronic HS conditions. As HSPs are now understood to be involved in many cellular processes from transcription regulation to cell death signaling (Rutherford and Zuker, 1994), our data suggest that HS might modulate the expression of lipogenic protein through HSPs. Tang and co-workers (Tang et al., 2016) have shown that HSP60 silencing alter directly the expression of FASN and ACCα in glioblastoma cells. FASN has also been recently identified as a bona fide HSP90 client (Fierro-Monti et al., 2013). Zhang et al. (2012) reported that HSP90 binds to AMPK and regulates ACCα expression.
To gain a better understanding of HS responses, we next evaluated the effect of HS alone or in combination with QCT on the same parameters by using chicken hepatocyte cell culture. We chose QCT because it is a highly bioactive ingredient of Noni with an average concentration of 7.4 mg/g (CP Foods, personal communication, Kampkotter et al., 2007). Although the optimal temperature of cell culture (37°C) and chicken body temperature (~41°C) are quite different, HS induced the expression of HSP60 and HSP70 in sim-CEL cells comparably to chicken liver tissues. However, it down-regulated the expression of lipogenic (ACCα, FASN, and ACLY) proteins in vitro which is opposite to that in animals. This indicates, firstly, that HS may directly alter the expression of hepatic lipogenic genes independently of the reduction of feed intake. Secondly, the differential expression profile of hepatic lipogenic proteins between the in vivo and in vitro HS studies might be due to the involvement of other organs such as, the brain (central neural pathways for thermoregulation) (Morrison and Nakamura, 2011) and the hormonal milieu. In fact, we have previously shown that HS altered the hepatic expression of leptin (Dridi et al., 2008) which is a key regulator of lipogenic genes (Dridi et al., 2005). Additionally, HS has been shown to dysregulate several other appetite-related hormones including ghrelin, thyroid hormone, and adipocytokines (i.e., adiponectin) and these hormones orchestrate the regulation of hepatic lipogenic genes (Yin et al., 2005; Tao et al., 2006; Buyse et al., 2009; Morera et al., 2012). QCT treatment induces the expression of lipogenic protein in sim-CEL cells maintained under both control and short (4 h) HS exposure, indicating that a short-term control mechanism of lipogenesis by QCT is effective. Moreover, the prompt responsiveness of cultured hepatocytes to QCT suggest that the QCT effect may be direct rather than secondary. The disaccord of QCT effects between chicken and mammalian hepatocytes is striking. It has been reported that QCT administration inhibits FASN expression in human HepG2 (Li et al., 2013; Zhao et al., 2014), rat H4llE (Zhou et al., 2014), and rat BRL-3A cells (Wang et al., 2013). This discrepancy might be due to dose and/or species-specific regulation of lipogenic genes by QCT (Dridi et al., 2006). In fact, QCT has been shown to have a dose-dependent biphasic effect on mammalian cell proliferation (van der Woude et al., 2003) and on CYP1A2 expression (Kang et al., 2004).
In conclusion (Figure 8), HS induces the hepatic expression of HSPs and stimulates de novo fatty acid synthesis in broilers which may explain the increased fat deposition observed during hot season (Geraert et al., 1996). In this study, Noni supplementation did not ameliorate feed intake and growth depression under HS conditions, but it did modulate the hepatic expression of HSPs in a time-specific manner suggesting a potential role in stress response that merit further investigations. Possibly, the lack of effect of Noni in the present study could be attributed to clean litter and low relative humidity (20%) in the experimental environmental chambers. A less pristine environment such as, that found in commercial poultry houses may be required to demonstrate potential benefits of Noni.
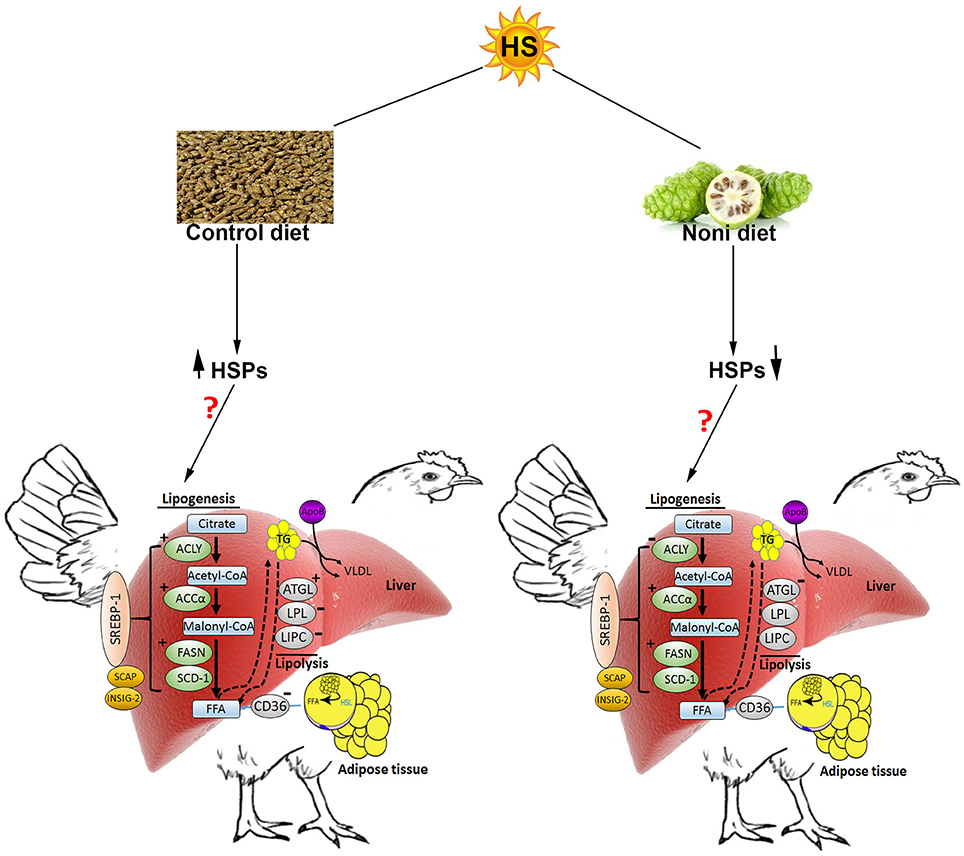
Figure 8. Schematic representation of the effect of heat stress and Noni supplementation on hepatic lipid metabolism in broilers (from protein expression analysis). Heat stress up-regulated the hepatic expression of heat shock proteins (HSP60, HSP70, and HSP90) as well as key lipogenic proteins (FASN, ACCα, and ACLY). HS down-regulated the hepatic expression of LPL and LIPC, but up-regulated ATGL. Noni supplementation down-regulated the hepatic expression of HSPs, ACCα, and FASN but it down regulated ACLY. ↑ and “+” denote induction, “−” indicates inhibition. HSL, hormone sensitive lipase; FFA, free fatty acids; VLDL, very low density lipoprotein.
Author Contributions
JF, HR-S, and EG performed the experiments. LB and BH generate the immunofluorescence data. LE and TP measured the thyroid hormone levels. SD, AD, and WB purchased the reagents. SD designed the experiment and wrote the manuscript.
Funding
This study was supported by grant from Charoen Pokphand Foods Indonesia (CPFI) (to WB and SD) and grant from Arkansas Bioscience Institute (ABI) to SD. CPFI and ABI have no role in conducting the research, generating the data, interpreting the results, or writing the manuscript.
Conflict of Interest Statement
The authors declare that the research was conducted in the absence of any commercial or financial relationships that could be construed as a potential conflict of interest.
Acknowledgments
Authors would like to thank Hsiu-Chuan ST (ARS, USDA) for technical assistance in this trial.
References
Bhusari, S., Liu, Z., Hearne, L. B., Spiers, D. E., Lamberson, W. R., and Antoniou, E. (2007). Expression profiling of heat stress effects on mice fed ergot alkaloids. Toxicol. Sci. 95, 89–97. doi: 10.1093/toxsci/kfl142
Bloom, S. R., Daniel, P. M., Johnston, D. I., Ogawa, O., and Pratt, O. E. (1973). Release of glucagon, induced by stress. Q. J. Exp. Physiol. Cogn. Med. Sci. 58, 99–108. doi: 10.1113/expphysiol.1973.sp002195
Brooks, V. J., Schäfer, M., Sharp, P., Xu, J., Cai, J., Keuler, N. S., et al. (2009). Effects of Morindacitrifolia (Noni) on CD4+ and CD8+ T-cell activation in neonatal calves. Prof. Anim. Sci. 20, 202–206. doi: 10.15232/S1080-7446(15)30716-6
Brownsey, R. W., Boone, A. N., Elliott, J. E., Kulpa, J. E., and Lee, W. M. (2006). Regulation of acetyl-CoA carboxylase. Biochem. Soc. Trans. 34, 223–227. doi: 10.1042/BST0340223
Buyse, J., Janssen, S., Geelissen, S., Swennen, Q., Kaiya, H., Darras, V. M., et al. (2009). Ghrelin modulates fatty acid synthase and related transcription factor mRNA levels in a tissue-specific manner in neonatal broiler chicks. Peptides 30, 1342–1347. doi: 10.1016/j.peptides.2009.04.015
Cahaner, A., and Leenstra, F. (1992). Effects of high temperature on growth and efficiency of male and female broilers from lines selected for high weight gain, favorable feed conversion, and high or low fat content. Poult. Sci. 71, 1237–1250. doi: 10.3382/ps.0711237
Chang, Y. Y., Lin, Y. L., Yang, D. J., Liu, C. W., Hsu, C. L., Tzang, B. S., et al. (2013). Hepatoprotection of noni juice against chronic alcohol consumption: lipid homeostasis, antioxidation, alcohol clearance, and anti-inflammation. J. Agric. Food Chem. 61, 11016–11024. doi: 10.1021/jf4038419
Dale, N. M., and Fuller, H. L. (1980). Effect of diet composition on feed intake and growth of chicks under heat stress. II. Constant vs. cycling temperatures. Poult. Sci. 59, 1434–1441. doi: 10.3382/ps.0591434
Dalvi, R. S., Das, T., Debnath, D., Yengkokpam, S., Baruah, K., Tiwari, L. R., et al. (2017). Metabolic and cellular stress responses of catfish, Horabagrus brachysoma (Gunther) acclimated to increasing temperatures. J. Therm. Biol. 65, 32–40. doi: 10.1016/j.jtherbio.2017.02.003
Deeb, N., and Cahaner, A. (2002). Genotype-by-environment interaction with broiler genotypes differing in growth rate. 3. Growth rate and water consumption of broiler progeny from weight-selected versus nonselected parents under normal and high ambient temperatures. Poult. Sci. 81, 293–301. doi: 10.1093/ps/81.3.293
Deeb, N., Shlosberg, A., and Cahaner, A. (2002). Genotype-by-environment interaction with broiler genotypes differing in growth rate. 4. Association between responses to heat stress and to cold-induced ascites. Poult. Sci. 81, 1454–1462. doi: 10.1093/ps/81.10.1454
Dok-Go, H., Lee, K. H., Kim, H. J., Lee, E. H., Lee, J., Song, Y. S., et al. (2003). Neuroprotective effects of antioxidative flavonoids, quercetin, (+)-dihydroquercetin and quercetin 3-methyl ether, isolated from Opuntia ficus-indica var. saboten. Brain Res. 965, 130–136. doi: 10.1016/S0006-8993(02)04150-1
Drew, B. G., Ribas, V., Le, J. A., Henstridge, D. C., Phun, J., Zhou, Z., et al. (2014). HSP72 is a mitochondrial stress sensor critical for Parkin action, oxidative metabolism, and insulin sensitivity in skeletal muscle. Diabetes 63, 1488–1505. doi: 10.2337/db13-0665
Dridi, S., Buyse, J., Decuypere, E., and Taouis, M. (2005). Potential role of leptin in increase of fatty acid synthase gene expression in chicken liver. Domest. Anim. Endocrinol. 29, 646–660. doi: 10.1016/j.domaniend.2005.05.002
Dridi, S., Hirano, Y., Tarallo, V., Kim, Y., Fowler, B. J., Ambati, B. K., et al. (2012). ERK1/2 activation is a therapeutic target in age-related macular degeneration. Proc. Natl. Acad. Sci. U.S.A. 109, 13781–13786. doi: 10.1073/pnas.1206494109
Dridi, S., Temim, S., Derouet, M., Tesseraud, S., and Taouis, M. (2008). Acute cold- and chronic heat-exposure upregulate hepatic leptin and muscle uncoupling protein (UCP) gene expression in broiler chickens. J. Exp. Zool. A Ecol. Genet. Physiol. 309, 381–388. doi: 10.1002/jez.461
Dridi, S., Ververken, C., Hillgartner, F. B., Arckens, L. E., Van der Gucht Cnops, L., et al. (2006). FAS inhibitor cerulenin reduces food intake and melanocortin receptor gene expression without modulating the other (an)orexigenic neuropeptides in chickens. Am. J. Physiol. Regul. Integr. Comp. Physiol. 291, R138–R147. doi: 10.1152/ajpregu.00899.2005
Exton, J. H., and Park, C. R. (1968). Control of gluconeogenesis in liver. II. Effects of glucagon, catecholamines, and adenosine 3',5'-monophosphate on gluconeogenesis in the perfused rat liver. J. Biol. Chem. 243, 4189–4196.
Faylon, M. P., Baumgard, L. H., Rhoads, R. P., and Spurlock, D. M. (2015). Effects of acute heat stress on lipid metabolism of bovine primary adipocytes. J. Dairy Sci. 98, 8732–8740. doi: 10.3168/jds.2015-9692
Fierro-Monti, I., Echeverria, P., Racle, J., Hernandez, C., Picard, D., and Quadroni, M. (2013). Dynamic impacts of the inhibition of the molecular chaperone Hsp90 on the T-cell proteome have implications for anti-cancer therapy. PLoS ONE 8:e80425. doi: 10.1371/journal.pone.0080425
Garrison, J. C., and Haynes, R. C. Jr. (1973). Hormonal control of glycogenolysis and gluconeogenesis in isolated rat liver cells. J. Biol. Chem. 248, 5333–5343.
Geraert, P. A., Padilha, J. C., and Guillaumin, S. (1996). Metabolic and endocrine changes induced by chronic heat exposure in broiler chickens: biological and endocrinological variables. Br. J. Nutr. 75, 205–216. doi: 10.1079/BJN19960125
Givisiez, P. E., Ferro, J. A., Ferro, M. I., Kronka, S. N., Decuypere, E., and Macari, M. (1999). Hepatic concentration of heat shock protein 70 kD (Hsp70) in broilers subjected to different thermal treatments. Br. Poult. Sci. 40, 292–296. doi: 10.1080/00071669987728
Goodridge, A. G., and Ball, E. G. (1967). Lipogenesis in the pigeon: in vivo studies. Am. J. Physiol. 213, 245–249.
Goti, D., Balazs, Z., Panzenboeck, U., Hrzenjak, A., Reicher, H., Wagner, E., et al. (2002). Effects of lipoprotein lipase on uptake and transcytosis of low density lipoprotein (LDL) and LDL-associated alpha-tocopherol in a porcine in vitro blood-brain barrier model. J. Biol. Chem. 277, 28537–28544. doi: 10.1074/jbc.M203989200
Griffin, H. D., and Goddard, C. (1994). Rapidly growing broiler (meat-type) chickens: their origin and use for comparative studies of the regulation of growth. Int. J. Biochem. 26, 19–28. doi: 10.1016/0020-711X(94)90190-2
Heeren, J., Niemeier, A., Merkel, M., and Beisiegel, U. (2002). Endothelial-derived lipoprotein lipase is bound to postprandial triglyceride-rich lipoproteins and mediates their hepatic clearance in vivo. J. Mol. Med. 80, 576–584. doi: 10.1007/s00109-002-0351-5
Hendrick, J. P., and Hartl, F. U. (1993). Molecular chaperone functions of heat-shock proteins. Annu. Rev. Biochem. 62, 349–384. doi: 10.1146/annurev.bi.62.070193.002025
Huang, C., Jiao, H., Song, Z., Zhao, J., Wang, X., and Lin, H. (2015). Heat stress impairs mitochondria functions and induces oxidative injury in broiler chickens. J. Anim. Sci. 93, 2144–2153. doi: 10.2527/jas.2014-8739
Iguchi, M., Littmann, A. E., Chang, S. H., Wester, L. A., Knipper, J. S., and Shields, R. K. (2012). Heat stress and cardiovascular, hormonal, and heat shock proteins in humans. J. Athl. Train. 47, 184–190. doi: 10.4085/1062-6050-47.2.184
Issell, B. F., Gotay, C. C., Pagano, I., and Franke, A. A. (2009). Using quality of life measures in a Phase I clinical trial of noni in patients with advanced cancer to select a Phase II dose. J. Diet. Suppl. 6, 347–359. doi: 10.3109/19390210903280272
Jastrebski, S. F., Lamont, S. J., and Schmidt, C. J. (2017). Chicken hepatic response to chronic heat stress using integrated transcriptome and metabolome analysis. PLoS ONE 12:e0181900. doi: 10.1371/journal.pone.0181900
Julian, R. J. (1998). Rapid growth problems: ascites and skeletal deformities in broilers. Poult. Sci. 77, 1773–1780. doi: 10.1093/ps/77.12.1773
Kampkotter, A., Nkwonkam, C. G., Zurawski, R. F., Timpel, C., Chovolou, Y., Watjen, W., et al. (2007). Investigations of protective effects of the flavonoids quercetin and rutin on stress resistance in the model organism Caenorhabditis elegans. Toxicology 234 113–123. doi: 10.1016/j.tox.2007.02.006
Kang, I. H., Kim, H. J., Oh, H., Park, Y. I., and Dong, M. S. (2004). Biphasic effects of the flavonoids quercetin and naringenin on the metabolic activation of 2-amino-3,5-dimethylimidazo[4,5-f]quinoline by Salmonella typhimurium TA1538 co-expressing human cytochrome P450 1A2, NADPH-cytochrome P450 reductase, and cytochrome b5. Mutat. Res. 545, 37–47. doi: 10.1016/j.mrfmmm.2003.08.002
Keyse, S. M., and Emslie, E. A. (1992). Oxidative stress and heat shock induce a human gene encoding a protein-tyrosine phosphatase. Nature 359 644–647. doi: 10.1038/359644a0
Lassiter, K., Greene, E., Piekarski, A., Faulkner, O. B., Hargis, B. M., Bottje, W., et al. (2015). Orexin system is expressed in avian muscle cells and regulates mitochondrial dynamics. Ame. J. Physiol. Regul. Integr. Comp. Physiol. 308, R173–R187. doi: 10.1152/ajpregu.00394.2014
Leenstra, F., and Cahaner, A. (1992). Effects of low, normal, and high temperatures on slaughter yield of broilers from lines selected for high weight gain, favorable feed conversion, and high or low fat content. Poult. Sci. 71, 1994–2006. doi: 10.3382/ps.0711994
Leveille, G. A., O'Hea, E. K., and Chakbabarty, K. (1968). In vivo lipogenesis in the domestic chicken. Proceedings of the Society for Experimental Biology and Medicine. Soc. Exp. Biol. Med. 128, 398–401. doi: 10.3181/00379727-128-33022
Li, X., Wang, R., Zhou, N., Wang, X., Liu, Q., Bai, Y., et al. (2013). Quercetin improves insulin resistance and hepatic lipid accumulation in vitro in a NAFLD cell model. Biomed. Rep. 1, 71–76. doi: 10.3892/br.2012.27
Lin, H., Decuypere, E., and Buyse, J. (2006). Acute heat stress induces oxidative stress in broiler chickens. Comp. Biochem. Physiol. A Mol. Integr. Physiol. 144, 11–17. doi: 10.1016/j.cbpa.2006.01.032
Lin, Y. L., Lin, H. W., Chen, Y. C., Yang, D. J., Li, C. C., and Chang, Y. Y. (2017). Hepatoprotective effects of naturally fermented noni juice against thioacetamide-induced liver fibrosis in rats. J. Chinese Med. Assoc. 80, 212–221. doi: 10.1016/j.jcma.2016.10.014
Martirosyan, A., Grintzalis, K., Polet, M., Laloux, L., and Schneider, Y. J. (2016). Tuning the inflammatory response to silver nanoparticles via quercetin in Caco-2 (co-)cultures as model of the human intestinal mucosa. Toxicol. Lett. 253, 36–45. doi: 10.1016/j.toxlet.2016.04.018
Morera, P., Basirico, L., Hosoda, K., and Bernabucci, U. (2012). Chronic heat stress up-regulates leptin and adiponectin secretion and expression and improves leptin, adiponectin and insulin sensitivity in mice. J. Mol. Endocrinol. 48, 129–138. doi: 10.1530/JME-11-0054
Morrison, S. F., and Nakamura, K. (2011). Central neural pathways for thermoregulation. Front. Biosci. 16, 74–104. doi: 10.2741/3677
Muto, J., Hosung, L., Uwaya, A., Isami, F., Ohno, M., and Mikami, T. (2010). Morinda citrifolia fruit reduces stress-induced impairment of cognitive function accompanied by vasculature improvement in mice. Physiol. Behav. 101, 211–217. doi: 10.1016/j.physbeh.2010.04.014
Nguyen, P., Greene, E., Ishola, P., Huff, G., Donoghue, A., Bottje, W., et al. (2015). Chronic mild cold conditioning modulates the expression of hypothalamic neuropeptide and intermediary metabolic-related genes and improves growth performances in young chicks. PLoS ONE 10:e0142319. doi: 10.1371/journal.pone.0142319
Park, S. H., Gammon, S. R., Knippers, J. D., Paulsen, S. R., Rubink, D. S., and Winder, W. W. (2002). Phosphorylation-activity relationships of AMPK and acetyl-CoA carboxylase in muscle. J. Appl. Physiol. 92, 2475–2482. doi: 10.1152/japplphysiol.00071.2002
Piekarski, A. L., Kong, B. W., Lassiter, K., Hargis, B. M., and Bottje, W. G. (2014). Cell bioenergetics in Leghorn male hepatoma cells and immortalized chicken liver cells in response to 4-hydroxy 2-nonenal-induced oxidative stress. Poult. Sci. 93, 2870–2877. doi: 10.3382/ps.2014-04113
Potterat, O., and Hamburger, M. (2007). Morinda citrifolia (Noni) fruit–phytochemistry, pharmacology, safety. Planta Med. 73 191–199. doi: 10.1055/s-2007-967115
Rajaei-Sharifabadi, H., Greene, E., Piekarski, A., Falcon, D., Ellestad, L., Donoghue, A., et al. (2017). Surface wetting strategy prevents acute heat exposure-induced alterations of hypothalamic stress- and metabolic-related genes in broiler chickens. J. Anim. Sci. 95, 1132–1143. doi: 10.2527/jas2016.1290
Rajaei-Sharifabadi, L. E. H., Porter, T., Donodhue, A., Bottje, W., and Dridi, S. (2017). Noni (Morinda citrifolia) modulates the hypothalamic expression of stress- and metabolic-related genes in broilers exposed to acute heat stress. Front. Genet. doi: 10.3389/fgene.2017.00204
Rea, I. M., McNerlan, S., and Pockley, A. G. (2001). Serum heat shock protein and anti-heat shock protein antibody levels in aging. Exp. Gerontol. 36, 341–352. doi: 10.1016/S0531-5565(00)00215-1
Rinninger, F., Brundert, M., Brosch, I., Donarski, N., Budzinski, R. M., and Greten, H. (2001). Lipoprotein lipase mediates an increase in selective uptake of HDL-associated cholesteryl esters by cells in culture independent of scavenger receptor BI. J. Lipid Res. 42, 1740–1751.
Rinninger, F., Kaiser, T., Mann, W. A., Meyer, N., Greten, H., and Beisiegel, U. (1998). Lipoprotein lipase mediates an increase in the selective uptake of high density lipoprotein-associated cholesteryl esters by hepatic cells in culture. J. Lipid Res. 39, 1335–1348.
Ruan, L., Zhou, C., Jin, E., Kucharavy, A., Zhang, Y., Wen, Z., et al. (2017). Cytosolic proteostasis through importing of misfolded proteins into mitochondria. Nature 543, 443–446. doi: 10.1038/nature21695
Rutherford, S. L., and Zuker, C. S. (1994). Protein folding and the regulation of signaling pathways. Cell 79, 1129–1132. doi: 10.1016/0092-8674(94)90003-5
Sanchez, I., Hughes, R. T., Mayer, B. J., Yee, K., Woodgett, J. R., Avruch, J., et al. (1994). Role of SAPK/ERK kinase-1 in the stress-activated pathway regulating transcription factor c-Jun. Nature 372, 794–798. doi: 10.1038/372794a0
Sanz Fernandez, M. V., Stoakes, S. K., Abuajamieh, M., Seibert, J. T., Johnson, J. S., Horst, E. A., et al. (2015). Heat stress increases insulin sensitivity in pigs. Physiol. Rep. 3:e12478. doi: 10.14814/phy2.12478
Schmittgen, T. D., and Livak, K. J. (2008). Analyzing real-time PCR data by the comparative C(T) method. Nat. Protoc. 3, 1101–1108. doi: 10.1038/nprot.2008.73
Schwanhausser, B., Busse, D., Li, N., Dittmar, G., Schuchhardt, J., Wolf, J., et al. (2011). Global quantification of mammalian gene expression control. Nature 473, 337–342. doi: 10.1038/nature10098
Settar, P., Yalcin, S., Turkmut, L., Ozkan, S., and Cahanar, A. (1999). Season by genotype interaction related to broiler growth rate and heat tolerance. Poult. Sci. 78, 1353–1358. doi: 10.1093/ps/78.10.1353
Strauss, J. G., Frank, S., Kratky, D., Hammerle, G., Hrzenjak, A., Knipping, G., et al. (2001). Adenovirus-mediated rescue of lipoprotein lipase-deficient mice. Lipolysis of triglyceride-rich lipoproteins is essential for high density lipoprotein maturation in mice. J. Biol. Chem. 276, 36083–36090. doi: 10.1074/jbc.M104430200
Sunder, S. J. J., Sujatha, T., and Kundu, A. (2015). Grommune: Morinda citrifolia-based herbal tonic for growth and immunity for commercial broilers. J. Appl. Anim. Res. 43, 137–140. doi: 10.1080/09712119.2014.928628
Sunder, S. T. J., and Kundu, A. (2015). Efficacy of Feeding of Morinda citrifolia fruit juice and Lactobacillus acidophilus in broiler. Asian J. Anim. Vet. Adv. 10, 352–359. doi: 10.3923/ajava.2015.352.359
Sunder, T. S. J., and Kundu, A. (2016). Effect of Morinda citrifolia in growth, production and immunomodulatory properties in livestock and poultry: a review. J Exp. Biol. Agric. Sci. 4, 1–17. doi: 10.18006/2016.4(3S).249.265
St-Pierre, N. R., Cobanov, B., and Schnitkey, G. (2003). Economic losses from heat stress by US livestock industries. J. Dairy Sci. 86, E52–E77. doi: 10.3168/jds.S0022-0302(03)74040-5
Tang, H., Li, J., Liu, X., Wang, G., Luo, M., and Deng, H. (2016). Down-regulation of HSP60 suppresses the proliferation of glioblastoma cells via the ROS/AMPK/mTOR Pathway. Sci. Rep. 6:28388. doi: 10.1038/srep28388
Tang, X., Meng, Q., Gao, J., Zhang, S., Zhang, H., and Zhang, M. (2015). Label-free quantitative analysis of changes in broiler liver proteins under heat stress using SWATH-MS Technology. Sci. Rep. 5:15119. doi: 10.1038/srep15119
Tao, X., Zhang, Z. Y., Dong, H., Zhang, H., and Xin, H. (2006). Responses of thyroid hormones of market-size broilers to thermoneutral constant and warm cyclic temperatures. Poult. Sci. 85, 1520–1528.
Trotter, E. W., Berenfeld, L., Krause, S. A., Petsko, G. A., and Gray, J. V. (2001). Protein misfolding and temperature up-shift cause G1 arrest via a common mechanism dependent on heat shock factor in Saccharomycescerevisiae. Proc. Natl. Acad. Sci. U.S.A. 98, 7313–7318. doi: 10.1073/pnas.121172998
Uno, Y., Takata, R., Kito, G., Yamazaki, H., Nakagawa, K., Nakamura, Y., et al. (2017). Sex- and age-dependent gene expression in human liver: an implication for drug-metabolizing enzymes. Drug Metab. Pharmacokinet. 32, 100–107. doi: 10.1016/j.dmpk.2016.10.409
van der Woude, H., Gliszczynska-Swiglo, A., Struijs, K., Smeets, A., Alink, G. M., and Rietjens, I. M. (2003). Biphasic modulation of cell proliferation by quercetin at concentrations physiologically relevant in humans. Cancer Lett. 200, 41–47. doi: 10.1016/S0304-3835(03)00412-9
Velleman, S. G. (2015). Relationship of skeletal muscle development and growth to breast muscle myopathies: a review. Avian Dis. 59, 525–531. doi: 10.1637/11223-063015-Review.1
Victoria Sanz Fernandez, M., Johnson, J. S., Abuajamieh, M., Stoakes, S. K., Seibert, J. T., Cox, L., et al. (2015). Effects of heat stress on carbohydrate and lipid metabolism in growing pigs. Physiol. Rep. 3:e12315. doi: 10.14814/phy2.12315
Wang, H., Astarita, G., Taussig, M. D., Bharadwaj, K. G., DiPatrizio, N. V., Nave, K. A., et al. (2011). Deficiency of lipoprotein lipase in neurons modifies the regulation of energy balance and leads to obesity. Cell Metab. 13, 105–113. doi: 10.1016/j.cmet.2010.12.006
Wang, M. Y., Anderson, G., Nowicki, D., and Jensen, J. (2008a). Hepatic protection by noni fruit juice against CCl(4)-induced chronic liver damage in female SD rats. Plant Foods Hum. Nutr. 63, 141–145. doi: 10.1007/s11130-008-0083-y
Wang, M. Y., Nowicki, D., Anderson, G., Jensen, J., and West, B. (2008b). Liver protective effects of Morinda citrifolia (Noni). Plant Foods Hum. Nutr. 63, 59–63. doi: 10.1007/s11130-008-0070-3
Wang, S. H., Cheng, C. Y., Tang, P. C., Chen, C. F., Chen, H. H., Lee, Y. P., et al. (2015). Acute heat stress induces differential gene expressions in the testes of a broiler-type strain of Taiwan country chickens. PLoS ONE 10:e0125816. doi: 10.1371/journal.pone.0125816
Wang, W., Wang, C., Ding, X. Q., Pan, Y., Gu, T. T., Wang, M. X., et al. (2013). Quercetin and allopurinol reduce liver thioredoxin-interacting protein to alleviate inflammation and lipid accumulation in diabetic rats. Br. J. Pharmacol. 169, 1352–1371. doi: 10.1111/bph.12226
Wang, Y., Kim, H. J., and Sparrow, J. R. (2017). Quercetin and cyanidin-3-glucoside protect against photooxidation and photodegradation of A2E in retinal pigment epithelial cells. Exp. Eye Res. 160, 45–55. doi: 10.1016/j.exer.2017.04.010
Watford, M. (1985). Gluconeogenesis in the chicken: regulation of phosphoenolpyruvate carboxykinase gene expression. Fed. Proc. 44, 2469–2474.
Xie, J., Tang, L., Lu, L., Zhang, L., Xi, L., Liu, H. C., et al. (2014). Differential expression of heat shock transcription factors and heat shock proteins after acute and chronic heat stress in laying chickens (Gallus gallus). PLoS ONE 9:e102204. doi: 10.1371/journal.pone.0102204
Yeh, Y. Y., and Leveille, G. A. (1971). In vitro and in vivo restoration of hepatic lipogenesis in fasted chicks. J. Nutr. 101, 803–809.
Yin, L., Wang, Y., Dridi, S., Vinson, C., and Hillgartner, F. B. (2005). Role of CCAAT/enhancer-binding protein, histone acetylation, and coactivator recruitment in the regulation of malic enzyme transcription by thyroid hormone. Mol. Cell. Endocrinol. 245, 43–52. doi: 10.1016/j.mce.2005.10.002
Zhang, L., Yi, Y., Guo, Q., Sun, Y., Ma, S., Xiao, S., et al. (2012). Hsp90 interacts with AMPK and mediates acetyl-CoA carboxylase phosphorylation. Cell. Signal. 24, 859–865. doi: 10.1016/j.cellsig.2011.12.001
Zhao, P., Mao, J. M., Zhang, S. Y., Zhou, Z. Q., Tan, Y., and Zhang, Y. (2014). Quercetin induces HepG2 cell apoptosis by inhibiting fatty acid biosynthesis. Oncol. Lett. 8, 765–769. doi: 10.3892/ol.2014.2159
Keywords: heat stress, lipogenesis, lipolysis, noni, quercetin, chicken, liver
Citation: Flees J, Rajaei-Sharifabadi H, Greene E, Beer L, Hargis BM, Ellestad L, Porter T, Donoghue A, Bottje WG and Dridi S (2017) Effect of Morinda citrifolia (Noni)-Enriched Diet on Hepatic Heat Shock Protein and Lipid Metabolism-Related Genes in Heat Stressed Broiler Chickens. Front. Physiol. 8:919. doi: 10.3389/fphys.2017.00919
Received: 30 August 2017; Accepted: 30 October 2017;
Published: 27 November 2017.
Edited by:
Sandra G. Velleman, The Ohio State University Columbus, United StatesReviewed by:
Matt Koci, North Carolina State University, United StatesGisela - Kaplan, University of New England, Australia
Copyright © 2017 Flees, Rajaei-Sharifabadi, Greene, Beer, Hargis, Ellestad, Porter, Donoghue, Bottje and Dridi. This is an open-access article distributed under the terms of the Creative Commons Attribution License (CC BY). The use, distribution or reproduction in other forums is permitted, provided the original author(s) or licensor are credited and that the original publication in this journal is cited, in accordance with accepted academic practice. No use, distribution or reproduction is permitted which does not comply with these terms.
*Correspondence: Sami Dridi, dridi@uark.edu