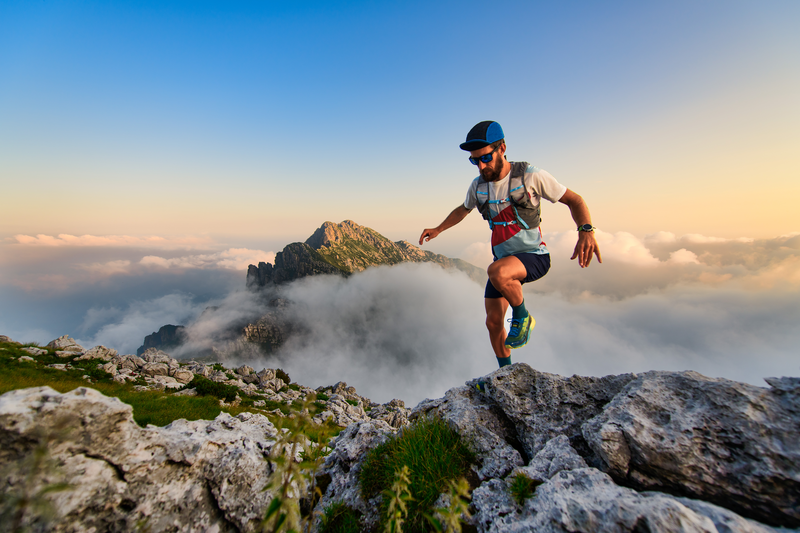
94% of researchers rate our articles as excellent or good
Learn more about the work of our research integrity team to safeguard the quality of each article we publish.
Find out more
REVIEW article
Front. Physiol. , 17 August 2017
Sec. Invertebrate Physiology
Volume 8 - 2017 | https://doi.org/10.3389/fphys.2017.00598
This article is part of the Research Topic The Digestive Tract of Cephalopods: at the Interface Between Physiology and Ecology View all 19 articles
The diversity of cephalopod species and the differences in morphology and the habitats in which they live, illustrates the ability of this class of molluscs to adapt to all marine environments, demonstrating a wide spectrum of patterns to search, detect, select, capture, handle, and kill prey. Photo-, mechano-, and chemoreceptors provide tools for the acquisition of information about their potential preys. The use of vision to detect prey and high attack speed seem to be a predominant pattern in cephalopod species distributed in the photic zone, whereas in the deep-sea, the development of mechanoreceptor structures and the presence of long and filamentous arms are more abundant. Ambushing, luring, stalking and pursuit, speculative hunting and hunting in disguise, among others are known modes of hunting in cephalopods. Cannibalism and scavenger behavior is also known for some species and the development of current culture techniques offer evidence of their ability to feed on inert and artificial foods. Feeding requirements and prey choice change throughout development and in some species, strong ontogenetic changes in body form seem associated with changes in their diet and feeding strategies, although this is poorly understood in planktonic and larval stages. Feeding behavior is altered during senescence and particularly in brooding octopus females. Cephalopods are able to feed from a variety of food sources, from detritus to birds. Their particular requirements of lipids and copper may help to explain why marine crustaceans, rich in these components, are common prey in all cephalopod diets. The expected variation in climate change and ocean acidification and their effects on chemoreception and prey detection capacities in cephalopods are unknown and needs future research.
The physiology, behavior, and sensory world of cephalopods have been succesfully adapted from the luminous shallow waters to the dark and cold deep-sea, where they look for the diverse prey that meet their energy requirements. Thus, a variety of feeding behaviors have been recorded both in the wild and laboratory, in association with diverse feeding strategies (see between others, the reviews of Nixon, 1987; Hanlon and Messenger, 1996; Rodhouse and Nigmatullin, 1996). Despite being limited in number, with 845 cephalopod species described to date (Hoving et al., 2014) when compared with the very populous phylum Mollusca to which they belong, nautiluses and coleoid cephalopods (cuttlefish, squid, octopus) are an astonishing example of diversity of form and function well equipped to deal with the various marine habitats they occupy (Clarke, 1988). This is an example of how evolution can drive potential limitations in design, based to their molluscan clade, to extreme complexities (e.g., Young, 1977; Budelmann, 1995; Godfrey-Smith, 2013; Albertin et al., 2015; Allcock et al., 2015; Shigeno, 2017). Cephalopod coastal species have received more research attention because of their ease of accessibility in the field and their ability to be maintained under laboratory conditions. Most shallow water species are active visual predators with vigorous metabolic activity and sophisticated behaviors (see between others Hanlon et al., 2008; Ebisawa et al., 2011; Benoit-Bird and Gilly, 2012; Vidal et al., 2014). On the other hand, mesopelagic and deep-sea cephalopod species have been less well-studied and their feeding strategies and behaviors are not well known. Cephalopods show a significant negative relationship between metabolism and minimum habitat depth (Seibel et al., 1997; Seibel and Childress, 2000) however, in addition to buoyancy and body mass, phylogenetic position also has an influence on the metabolic rates of each individual species (Seibel and Carlini, 2001). As showed by Seibel et al. (1997), cephalopods of the family Cranchiidae as Cranchia and Liocranchia have low metabolic rates. These cephalopods live both in epipelagic waters (as subadults) and deep-sea (when adults) and do not follow the negative relationship between minimum depth and metabolic rate showed for most cephalopod species studied. The example illustrate that phylogeny is also an important factor when considering metabolic rates of individual species (Seibel and Carlini, 2001).
The following text seeks to briefly review recent advances on cephalopod predation and identify the main gaps in knowledge on this aspect of cephalopod biology and behavior. Here, we aim to briefly account for the wide spectrum of morphological, behavioral, and physiological features that cephalopods use to meet their energetic needs through predation and food intake. Along this journey we will identify possible gaps in knowledge, thus providing a short guide for future studies.
The physiology and sensory processing capabilities of cephalopods are adapted to all marine environments. Animals looking for diverse prey needed to meet energetic requirements; metabolic energetic needs that change dramatically according to the ontogenetic state, the habitat they live in and life cycle stage. A variety of feeding behaviors have been recorded in association with diverse feeding strategies (for review see Hanlon and Messenger, 1996; but see also Rodhouse and Nigmatullin, 1996), and such richness is accompanied by a sophisticated set of sensory systems (review in: Budelmann, 1994; Wells, 1994; Budelmann et al., 1997; Table 1). This developed sensory system allows them to achieve sophisticated behaviors to detect food, avoid predators and communicate between congeners in a way comparable to vertebrates. Photo-, mechano-, and chemoreceptors provide support for the collection of information about their potential prey.
Table 1. Biological and behavioral adaptations utilized by cephalopods for the sake of their predatory behavior.
Probably one of the most striking features of cephalopods is their developed eye, superficially resembling that of teleost fish. It has a single nearly spherical lens with a graded refractive index, the ability to accommodate the len and a similar capacity for eye movement, showing an example of convergent evolution (Packard, 1972). The use of an adjustable pupil to control the amount of light entering the eye distinguishes the cephalopods' eye from their fish counterpart and the light-evoked pupillary constriction in cephalopods is among the fastest in the animal kingdom (Douglas et al., 2005). Among the few exceptions is the deep-sea cirrate octopod Cirrothauma murrayi, whose eye lacks lenses and the optic lobes are simply organized (Aldred et al., 1983), however, it is probably able to detect bioluminescence (Warrant and Locket, 2004). Most cephalopods studied have a single type of rhodopsin as a visual pigment, suggesting they are blind to color (Messenger et al., 1973; Marshall and Messenger, 1996; Mäthger et al., 2006). They can achieve spectral and color discrimination by exploiting chromatic aberration and pupil shape (Stubbs and Stubbs, 2016), but this system could work for only a narrow range of visual tasks (Gagnon et al., 2016). The giant (Architeuthis) and the colossal (Mesonychoteuthis) squids have the largest eyes in the animal kingdom, however their characteristics suggest they are mainly used for detecting and identifying bioluminescent waves generated by sperm whales during their dive into the deep, thus protecting them from potential predation, rather than detecting prey at long distances (Nilsson et al., 2012). The importance of the visual system to locate prey is also reflected in the ability for aerial capture, such as, when Sepia officinalis is able to attack and capture prey shown above the water surface by an experimenter (Boletzky, 1972). The complexity of the visual system of cephalopods is also achieved through extra-ocular light perception capabilities, providing an intricate network of sensory devices on their skin (see also Kingston et al., 2015; Ramirez and Oakley, 2015; Kelley and Davies, 2016). In addition, cephalopods are sensitive to polarized light and polarization vision serves to enhance the detection and recognition of prey. Squid hatchlings attack planktonic prey under polarized illumination at a 70% greater distance than under depolarized illumination (Shashar et al., 1998) and the polarization vision helps cuttlefish to see further into turbid water and to better detect prey (Cartron et al., 2013).
Sensory capabilities are not limited to vision. Cephalopods have sensory receptors that form the lateral line system, which detects gentle water currents and vibrations. Ciliated primary sensory hair cells, sensitive to local water movements, are arranged in epidermal lines located on the arms, head, anterior part of dorsal mantle and funnel (e.g., Sundermann, 1983; Budelmann and Bleckmann, 1988; Budelmann, 1994; Lenz et al., 1995) and are known to provide sensory capabilities in detecting prey (Komak et al., 2005). In fact, cuttlefish are able to catch small shrimp in the darkness and behavioral experiments showed they use the epidermal lines to detect prey (Budelmann et al., 1991).
Distant chemoreceptor organs such as, olfactory organs and rhinophores, further provide additional sensory capabilities. Olfactory organs are paired, oval shaped organs situated on either side of the head, ventrally behind the eye and near the mantle edge. Their possible role in prey detection is poorly understood. Water containing food odor (shrimp) is detected by S. officinalis (Boal and Golden, 1999) and embryos exposed to the odors of prey later influences prey choice in the same species (Guibé et al., 2010). Increased ventilation rates in response to prey chemicals was described for Eledone cirrhosa (Boyle, 1986); and positive chemotaxis for Octopus maya during Y-maze experiments, with amino acids (alanine, proline), nucleotids (ATP), and crab extract functioned as excitants, while betaine and taurine functioned as arrestants (Lee, 1992). The rhinophores of Nautilus are paired organs located below each eye and open to the exterior by a narrow pore. They are similar to the olfactory organs but are significantly larger (Basil et al., 2005).
In addition, cephalopods have contact receptors in the tentacles, sucker rims, and lips; known to allow sensing of a broad spectrum of chemical and mechanical signals. Sucker receptors are more elaborated in octopus. There are about 10,000 chemoreceptor cells in a single sucker of an octopod, but only about 100 are present in the sucker of a cuttlefish (Budelmann, 1996). The food searching habit of benthic octopods (see below Speculative pounce), that make extensive use of the arms and suckers exploring rocks and crevices, may justify this marked difference. In contrast, cuttlefish use their arms mostly for manipulating their prey (Chichery and Chichery, 1988). Contact receptors located in lips of octopus and cuttlefish are more advanced in structure and organization than those of squid. As cuttlefish and octopus are more sedentary and benthic than pelagic squid, they may rely more on tactile and chemical stimuli (Emery, 1975). Chemical receptors in cephalopods help them to locate prey and also to avoid unwanted prey. Cuttlefish were able to learn that a prey is not acceptable food, to recognize and to avoid it and, as a result, to choose a usually non-preferred prey when necessary (Darmaillacq et al., 2004).
Hatchling cephalopods are of relatively large size, ranging from 0.6 (Argonauta hians) to 28 (Graneledone boreopacifica) mm mantle length (Villanueva et al., 2016), allowing the animal to start an active mode of food searching marked by the coexistence of two nutritive systems: (a) an embryonic energy in the form of yolk, and (b) a post-hatching energy provided by captured food (Boletzky, 2003). Preference for prey at hatchling when previously exposed during the latest embryonic stages (Darmaillacq et al., 2006) and visual imprinting during a short sensitive period during the first day of life (Darmaillacq et al., 2008) showed some of the available tools employed by the young cuttlefish, S. officinalis, to successfully capture prey and survive during the first days of life as a predator. In this species, the development of learning and predatory behavior is observed during late embryonic and early juvenile development. This occurs simultaneously with the maturation of the vertical–subvertical lobe tracts of the brain, allowing the animals to maintain a prey in the frontal field during predatory pursuit (Dickel et al., 1997). Then, during the first 3 months of life, feeding hierarchy has been reported for the same species (Mather, 1986; Warnke, 1994). A comprehensive review on this behavioral development is provided by O'Brien et al. (2016). On the other hand, in the juvenile holobenthic octopuses O. maya, preference to attack a prey is not obtained through previous life experience. Juvenile octopuses selected crabs as prey when individuals had previously been fed shrimp earlier in life. This could be the result of innate biological processes (Portela et al., 2014).
In squids, brain developmental differences can be found when observing the relatively large Loliginid Sepioteuthis lessoniana hatchlings, with a subvertical lobe of especially complicated domain structure, which may reflect an active predatory behavior (Shigeno and Yamamoto, 2005). In comparison, the minor development of higher motor centers of the small ommastrephid Todarodes pacificus hatchlings, suggests these animals are not active predators at this time but perhaps suspension feeders after hatching (Shigeno et al., 2001a,b). The first food and feeding strategy of the ommastrephid paralarvae before they start to actively feed on zooplankton is an unresolved question that merits further research (O'Dor et al., 1985; Vidal and Haimovici, 1998).
Diet of planktonic cephalopods in the wild is poorly understood (Passarella and Hopkins, 1991; Roura et al., 2012, 2016; Olmos-Pérez et al., 2017). Roura et al. (2016) found that Octopus vulgaris hatchlings targeted low abundance prey like decapod crustacean larvae independently of the zooplankton community they inhabit, thus showing a selective behavior in these patchy environments. Stable isotope ratios allowed discrimination of specific feeding strategies during ontogenesis and accumulations of metals as cadmium and mercury also reflected the ontogenetic stage in five species of cephalopods (Chouvelon et al., 2011). Externally, strong morphological changes during early life are recognized in some cephalopod groups, particularly in oegopsid squids and merobenthic octopods, associated with different habitats and feeding modes during early life. Ontogeny of prey capture develops progressively, from a simple type after hatching to an adult-like capture behavior involving structures such as, tentacles and hooks, which are absent or poorly developed in larval forms (Sweeney et al., 1992). In young ommastrephid squids, the fused tentacles forms the proboscis and its functionality, supposedly related to food capture, remain an open question that again needs future research (Uchikawa et al., 2009).
In loliginid squids, ontogeny of prey capture develops progressively, from a simple type after hatching to an adult-like capture behavior involving tentacles after 1 month of age in Doryteuthis opalescens raised with copepods (Chen et al., 1996). In merobenthic octopods, a positive allometric arm growth takes place during planktonic life, probably helping the animal to capture benthic prey after settlement. At the same time animals lose the oral denticles of the beaks, of which the trophic function remains unclear (Villanueva and Norman, 2008). However, observations on the external digestion and initial ingestion process in the pymy squid Idiosepius paradoxus, suggest that oral denticles may be used to detach the semidigested flesh from the exoskeleton of the crustacean prey (Kasugai et al., 2004). The early development of the muscular, protein-rich arm crown in merobenthic octopods is related to the decrease in lipid content of the animal, due to the relative decrease of the visceral mass, where lipids are abundant. During planktonic life, the octopus feeding behavior is that of a visual predator. The presence of prey increases the turning rate and reduces the swimming speed in O. vulgaris paralarvae, possibly improving the exploitation of patchy food environments in the wild (Villanueva et al., 1997).
At the other end of early life is senescence, a period coincident with the end of the single reproductive period characteristic of this group of semelparous molluscs. Chichery and Chichery (1992) found in aging S. officinalis signs of degeneration of the anterior basal lobe, a structure that plays an important role in the control of the predatory behavior, as indicated by previous studies by the same authors (Chichery and Chichery, 1987). In addition, they suggested that visual capacities were also affected during the aging process by reducing the attention mechanisms and also the maintenance of the predator's visual tracking behavior, concluding that the low interest in the prey shown by senescent cuttlefish may be related to the deterioration of the basal lobe and the decreasing visual input. The progressive loss of appetite in both senescent male and female octopuses is fairly well documented (see review by Anderson et al., 2002). In the brooding O. vulgaris, female food intake decreases about 90% and the method of predation and handling over the scarce prey changes and becomes irregular (Wodinsky, 1978). Interestingly, in the brooding female Octopus filosus, Wodinsky (1977) found that removal of optic glands made them cease brooding, start feeding again, and live longer than normal. This surprising behavior after removal of these glands has not been studied in other cephalopod species.
Crustaceans are present in nearly all the cephalopod diets studied to date. Teleost fish and molluscs complement their energetic needs in different proportions, depending on the species, habitat, and ontogenetic stage (see reviews of Nixon, 1987; Rodhouse and Nigmatullin, 1996). Why crustaceans seem to be an indispensable prey in the diet to sustain suitable growth for cephalopods under culture conditions, and particularly for their young stages, is a subject of current debate (Iglesias et al., 2014). Large protein and amino acid content in the diet are required to maintain positive growth, at least in shallow water cephalopod species characterized by vigorous protein metabolism and showing a relatively low quantity of lipids in their body composition. However, phospholipids, cholesterol, and long-chain polyunsaturated fatty acids (PUFA), all of them abundant in marine crustaceans, seem to play an important role. Particularly, the n-3 PUFA, due to their high demand for cell membrane synthesis where they are incorporated, due to the inability of cephalopods to synthesize them (Monroig et al., 2013; Reis et al., 2014), These findings suggest that PUFA, play an essential role in cephalopod nutrition, at least for shallow water, fast growing cephalopod species (Navarro et al., 2014). In addition, the elemental composition of natural food strongly suggests that cephalopod paralarvae and juveniles must require a food rich in copper (Villanueva and Bustamante, 2006). This fact is probably related to the haemocyanin requirements for oxygen transport, as copper is the dioxygen carrier of haemocyanin typical of crustaceans and molluscs. Again, marine crustaceans seem to play a pivotal role in the diet of cephalopods, also considering that diet of a species can change from different locations depending on the prey availability and abundance (Leite et al., 2016). The general tendency of cephalopods to prey mainly on crustaceans, fish, molluscs, and other invertebrates, such as, polychaetes, echinoderms, hydroids (Olmos-Pérez et al., 2017), and also on gelatinous fauna (Hoving and Haddock, 2017) is not followed by the deep-sea species, Vampyroteuthis infernalis. This species is able to fuel its low metabolism mainly on detritus (Hoving and Robison, 2012). On the other hand, as an extreme comparative example, the Giant Pacific octopus (Enteroctopus dofleini) and Octopus cf insularis occasionally feeds on large marine birds (Anderson and Shimek, 2014), and attacks and bite damage to the skipjack tuna (Katsuwonus pelamis) and yellowfin tuna (Thunnus albacares) inside purse seine nets have also been described for the jumbo squid Dosidicus gigas (Olson et al., 2006). These species are extreme examples showing the adaptive capacity of cephalopod species to obtain energy from the different marine habitats in which they live. In addition, when resources are scarce or when the density of congeners is high, cephalopods can choose cannibalism as a feeding behavior. Cannibalistic behavior has been reported from video recordings in the wild for both squids (Hoving and Robison, 2016) and octopods (Hernández-Urcera et al., 2014) independently of fishing operations, which may induce unnatural feeding behaviors. Cannibalism is common in most cephalopod species whose diet has been studied, an uncommon characteristic in the animal kingdom which may be related to their high metabolic demands. Factors influencing this unusual feeding behavior are environmental variations, population density, food availability, body size, and sexual dimorphism (Ibáñez and Keyl, 2010). In addition to visual stomach content analysis, recent tools are being used as trophic indicators and tracers in food chain pathways including stable isotope (Lorrain et al., 2011; Ohkouchi et al., 2013; Guerreiro et al., 2015), heavy metal (Bustamante et al., 1998), and fatty acid signature analysis (Pethybridge et al., 2013; Rosa et al., 2013), as well as molecular techniques (Deagle et al., 2005; Braley et al., 2010; Roura et al., 2012; Olmos-Pérez et al., 2017) and food web models (Hunsicker et al., 2010; Coll et al., 2013).
Until food satiation is obtained, cephalopods explore their environment looking for food. Known modes of hunting in cephalopods include ambushing, luring, stalking and pursuit, speculative hunting and hunting in disguise, among others (Table 2), described in detail by Hanlon and Messenger (1996). Behavioral observations on foraging cephalopods in their natural habitat usually come from shallow-water environments, mostly on cuttlefishes and octopuses using scuba diving. A variety of behaviors have been recorded and mimicry has been observed during octopus foraging (Forsythe and Hanlon, 1997; Hanlon et al., 2008; Krajewski et al., 2009; Caldwell et al., 2015). The sequences of foraging behavior in shallow water octopuses usually showed characteristics of a tactile saltatory searching predator, as well as a visual opportunist (Leite et al., 2009). Using acoustic techniques, coordinated school behavior during foraging was recorded at night in shallow water for jumbo squid D. gigas. They were observed using ascending, spiral-like swimming paths to emerge from extremely dense aggregations (Benoit-Bird and Gilly, 2012).
Table 2. Comparison between different hunting strategies adopted by some species of cephalopods and vertebrates (not an exhaustive list).
Behavioral studies of predation in the laboratory are more detailed and abundant. The predatory strategy is part of a series of body and locomotory patterns. The visual attack is executed with great accuracy leading to a final strike, a sequence described in cuttlefishes (Messenger, 1968) and identified in different species (Lolliguncula brevis, Jastrebsky et al., 2017) revealing similar behavioral performances. During the attack, raised arms and dynamic skin patterns are part of these sophisticated behavioral sequences utilized presumably to deceive the potential prey and facilitate capture. Raised arms are expressed during predation when the cuttlefish has located its prey and is approaching it to reach a position suitable for attack. Arms I appear extended vertically upwards (Messenger, 1968, p. 345) and often separated in a V, each forming an S-shaped curve (review in Borrelli et al., 2006). In some cases, arms II may also be similarly raised. Raised arms are generally dark and may sway to and fro. Messenger (1968) suggests that this peculiar posture and swaying movement of the arms may act as lures, directing the prey's attention away from the tentacles. Chromatic pulses and rhythmic passing waves as been described as dynamic skin chromatic patterns of cephalopods during hunting displays. Chromatic pulses are known in cuttlefishes and also in squids and octopuses and consist of a single band of color contrast sweeping across part of the predator in a particular direction. Rhythmic passing waves are known in cuttlefishes and octopuses, involving the movement of rhythmic bands across the predator in a constant direction (see How et al., 2017 for review).
The most accurate description of full attack response of octopuses (e.g., O. vulgaris) is provided by Andrew Packard: “In full attack…an octopus launches itself directly toward the crab…swimming by the propulsion of water from its funnel (siphon) and without touching the bottom” (Packard, 1963, p. 39). The chromatic, postural, and locomotor components (i.e., body patterning), making up the behavior, include: (i) head and eyes raised, with the latter “wide open”; (ii) the body is or darkens to “a deep reddish-brown hue” (Packard, 1963, p. 39); (iii) the arms are outstretched or loose “with the suckers facing downwards” (Packard, 1963, p. 39); (iv) the octopus orients the siphon posteriorly, away from its target. In O. vulgaris, as in squid and cuttlefish, the full attack response is elicited by the initial visual recognition of an edible “object” followed by the final outcome of the attack (i.e., obtainment of food; for review see also Borrelli et al., 2006). The full attack is only one example of the variety of predatory behaviors. A full gradient of locomotor patterns appear to be exhibited. As reviewed by Borrelli et al. (2006) during crawling an octopus moves relatively slowly in contact with the ground, and may also be aided by brief swimming sequences. The animal moves along the substrate aided by the suckers of the central half of the arm, while the arms push or pull, depending on their position, to facilitate the direction of movement; this crawling may imply several arms (Finn et al., 2009) or just the posterior pair as in bipedal locomotion, also referred as walking or tiptoeing (see also Huffard et al., 2005; Borrelli et al., 2006). Crawling is adopted by octopuses to explore their surroundings and approach sites that they eventually explore for prey capture.
On the other hand, speculative hunting (or speculative pounce) is characteristic of several octopus species (see for e.g., Borrelli et al., 2006; Leite et al., 2009). While searching for prey, “the octopus moves across the bottom in a combination of swimming and crawling actions. Every 1–2 m it makes a speculative pounce, covering a rock, a clump of algae, or a small area of the bottom with its web. Pausing for a few seconds to feel under the web the octopus continues its trip” (Yarnall, 1969, p. 749).
In addition, cephalopods use different tools to enhance prey capture. For example, disguise strategies using ink during predation, has been reported recently by Sato et al. (2016) for I. paradoxus. These pygmy squid use ink during prey attacks in two modes: releasing ink between themselves and the prey and then attack through the ink cloud, and also releasing ink away from the prey and attacking the prey from another position. Another tool used in the darkness is the dinoflagelate bioluminescence, employed by Euprymna scolopes and S. officinalis to locate non-luminous crustaceans and fish prey (Fleisher and Case, 1995). During foraging under culture conditions, it is remarkable that cuttlefish (Sepia pharaonis) are able to identify the amount of prey available, discriminate prey numbers, and the following prey selection, all depending on their satiation state (Yang and Chiao, 2016). When cuttlefish detect a prey, they perform a well-known three-stage visual attack sequence of attention, positioning, and seizure (Hanlon and Messenger, 1996). Observing conspecifics during prey capture, these events do not seem to improve their predation techniques (Boal et al., 2000).
Venom is used by cuttlefishes and octopods to kill the prey and for muscle relaxation. Octopuses bored holes in the carapace, the eye or the arthrodial membrane of crustaceans (Grisley et al., 1996; Pech-Puch et al., 2016). The selection of the preferred area to inject the cephalotoxin in the crab seems to be a combination of factors related to prey and octopus size. For example, large octopuses use eye puncture less frequently than small individuals (Grisley et al., 1999). Prey handling in octopus eating bivalves showed different combinations of pulling and drilling feeding behaviors. The injection of the cephalotoxin into the bivalve and gastropod prey is associated with drilling. Drilling occurs by the combined action of radula and salivary papilla (Nixon, 1980). A combination of drilling and pulling behaviors has been reported for preying on bivalve and gastropod prey (Runham et al., 1997; Fiorito and Gherardi, 1999; Steer and Semmens, 2003; Ebisawa et al., 2011). Octopuses hold the prey within the proximal part of the arms so, they cannot use vision during most prey handling period, probably choosing the most energetic, cost effective feeding behavior based on previous experience (Anderson and Mather, 2007). In the field, other factors may influence the bivalve selection and feeding mode. McQuaid (1994) showed that mussel size selected by small octopuses (<500 g) was related to octopus weight, with small octopuses eating on small mussels because they are unable to remove large mussels attached with the byssus threads from the rocks. In addition to pulling and drilling, shell crushing has been reported as a feeding behavior for the deep-sea octopod Graneledone preying on gastropods, a behavior that may be favored due to their relatively larger beaks in comparison with those of shallow water octopods (Voight, 2000). It is remarkable that the elevated diversity of cephalopod hunting behaviors, almost matches the strategies adopted by vertebrate predators (Table 2). Both taxa are so diverse and remote in their phylogenetic traits, but clearly there are cases of functional (and behavioral) convergence during evolution.
In neritic and epipelagic cephalopods, vision is probably the main sense utilized for prey detection and capture. As light intensity decreases in deep-sea environments, low temperature reduces the metabolic demands and predator-prey distance changes (Seibel et al., 2000). In this environment, the mechanoreceptor structures in the arms, tentacles, and filaments increase in number and complexity. These metabolic and morphological changes considered to be closely related with the prey selected by deep-sea cephalopods result in feeding strategies that are more diverse in the deep-sea that previously believed. The cirrate octopods are characterized by the possession of paired filamentous cirri along the arms, of diverse length according to families, which are interspersed between a single row of suckers, and are thought to have a sensory function involved in prey detection and capture. Cirrates feed mainly on small-sized organisms with low swimming speeds including amphipods and polychaetes (Collins and Villanueva, 2006). In the cirrate Stauroteuthis syrtensis, blue-green bioluminescence is emitted by modified suckers without adhesive function; this has been suggested to act as a light lure to attract prey and/or mates (Johnsen et al., 1999). For S. syrtensis of 60 g fresh weight, a daily ration of only 1–30 calanoid copepods day−1 has been estimated (Jacoby et al., 2009), showing the low metabolic rate of this group of deep-sea cephalopods. In a similar way, the colossal squid (Mesonychoteuthis hamiltoni), the world largest invertebrate, reaching 500 kg of total weight, seems to be an ambush or sit-and-float predator that uses the hooks on its arms and tentacles to capture prey and reach a projected daily energy consumption of 45 kcal day−1, equivalent to only 30 g of fish day−1 (Rosa and Seibel, 2010). The knowledge of the diet of deep-sea squids needs further research. A comprehensive review of the main prey found in stomachs of deep-sea squids has been provided by Hoving et al. (2014).
As suggested by Young et al. (1998), the great variation in squid tentacle morphologies may reflect variation in target prey and the handling of captured food. The deep-sea squid Grimalditeuthis bonplandi is an extreme example: its tentacles have a very thin and fragile elastic stalk, whereas the clubs bear no suckers, hooks, or photophores. It is unknown how these tentacles are used to capture and handle their prey, as they consist on cephalopods and crustaceans (Hoving et al., 2013). Very long dorsolateral arms with photophores are present in Lycoteuthis lorigera males (Villanueva and Sánchez, 1993) and extremely large and filamentous arms and tentacles approximately equal in thickness and length are key characters of the genus Magnapinna: these reach 15–20 times the mantle length of the animal, reaching to 7 m in total length (Vecchione et al., 2001; Guerra et al., 2002). V. infernalis uses its two thin and retractile filaments, which may be up to nine times the body length for food capture, i.e., remains of gelatinous zooplankton, discarded larvacean houses, crustacean remains, diatoms, and fecal pellets (Hoving and Robison, 2012), thus indicating that the use of luring as a mode of hunting is probably common in deep-sea cephalopods. Also the mesopelagic Spirula spirula feeds mainly on detritus and zooplankton (Ohkouchi et al., 2013).
As mentioned above, cephalopods do not necessarily predate exclusively on live prey. Some cephalopod species are collected in large numbers from the wild using baited traps such as, Nautilus (Dunstan et al., 2011) and O. vulgaris (Guerra, 1997) showing that scavenger behavior exists in nature. Recent development of the cephalopod culture techniques (review in Iglesias et al., 2014) allowed the use of frozen prey and/or artificial food in supporting growth during part of the life cycle in a number of species including Nautilus, cuttlefish (S. officinalis, S. pharaonis, Sepiella inermis, Sepiella japonica), squid (Loligo vulgaris, S. lessoniana), and octopus (Amphioctopus aegina, O. maya, Octopus mimus, Octopus minor, O. vulgaris). The first feeding period usually requires live crustacean prey, particularly for the delicate planktonic stages, although planktonic octopuses are able to detect, capture and ingest inert particles from the water surface (Marliave, 1981) or descending in the water column (Villanueva et al., 2002; Iglesias et al., 2007). A successful semi-humid squid paste-bound gelatine has been developed to feed O. maya benthic hatchlings from first feeding, showing that this species can live and reach normal growth with artificial food during the whole life cycle under laboratory conditions (Rosas et al., 2014). In other species, after a variable acclimation period, inert food is readily accepted by advanced juvenile, subadult, and/or adult stages of cephalopods under culture conditions (Vidal et al., 2014).
The training phase from feeding on live prey to inert food shows the behavioral adaptions and learning capacities of these animals under laboratory conditions. As an example noted by Nabhitabhata and Ikeda (2014), S. lessoniana aged 20 days can be fed sliced fish meat of two or three times the mantle length of the squid, that seize the food in the water column: when squid feed on live prey, the prey is seized by the tentacles, when the squid are fed dead feed, they change their prey capture behavior, using only their arms to seize the food and do not perform the positioning phase typical of the squid attack (Messenger, 1968). The same behavioral adaptions and prey capture modes are observed in S. pharaonis (Nabhitabhata, 2014a) and S. inermis (Nabhitabhata, 2014b) when changing from live to inert prey.
In this review, we surfed through a number of important topics that require further research and possibly a dedicated effort. Research on cephalopod predatory strategies is needed in a variety of fields, from behavior to ecology. Studies of feeding behavior, nutrition, and feeding requirements are critical in order to develop the nascent cephalopod aquaculture of key species, particularly from early young stages. Studies on nutritional requirements are only at the beginning. The role of lipids on the early growth and survival of shallow water species seems more important than previously supposed and research is also needed in that field (Navarro et al., 2014). Hatchlings of 13% of the cephalopod species described to date has been obtained under laboratory conditions, most of them belonging to shallow water octopods (Villanueva et al., 2016). As this number increases in the future, new larval and juvenile predatory behavioral strategies will mostly likely be described. Similarly, the future study of deep-sea and oceanic cephalopod forms will provide further instances of novel, undescribed receptors, organs, behaviors, and modes of prey detection and capture in cephalopods (Hoving et al., 2014). In addition, whether our knowledge on diet richness of a given cephalopod species in the wild is affected or not by research effort remains to be explored; data we presented above may represent only a starting-point. The variability of conformation of cephalopod beaks and their functional relation with possible prey-items is another possible challenging avenue of research (Franco-Santos and Vidal, 2014; Franco-Santos et al., 2014). The use of modern techniques as genomics (Olmos-Pérez et al., 2017) and proteomics technologies (Varó et al., 2017), microbiota associated with different diets (Roura et al., 2017), or venom structure (Whitelaw et al., 2016) may further extend our knowledge on cephalopod diets. Some aspects, such as, the hormonal control over feeding in cephalopods are practically unknown (Wodinsky, 1977). Interactions with other species such as, intraguild predation (when species compete simultaneously for resources and interact as prey and predator), is another aspect that may need further attention in cephalopod science. The interaction between shallow water octopus and juvenile lobster is potentially an example of intraguild predation involving interference competition for refuge (Butler and Lear, 2009) but cannibalism (see above) may also be seen under this framework.
Interaction with other species, as well as competition for spatial and feeding resources will probably be modified with global change. A representative example is the case of the jumbo squid D. gigas. During the daytime, jumbo squids dive to the depth, suppressing metabolism in the oxygen minimum zone, an energy saving strategy in hours of prey limitation in shallow waters (Rosa and Seibel, 2008). The expected climate change expansion of deep-water hypoxia and the warming and acidification of surface waters will concentrate both prey and predators, with unknown effects on D. gigas predatory dynamics (Seibel, 2015). The expected variation in climate change and ocean acidification has been shown to induce complex changes in chemoreception and prey detection, including altered cue detection behaviors in some marine organisms. Changes in CO2 may have effects on predator handling time, satiation, and search time in coastal molluscs (Kroeker et al., 2014) as shown by increased activity in the pigmy squid Idiosepius pygmaeus (Spady et al., 2014). Nonetheless, the possible effects of elevated CO2 on chemoreception in cephalopods are unknown. The ability of carnivorous fish and sharks to detect chemical cues produced by their prey appears reduced and, as a consequence, the activity levels spent on food searching increases upon exposure to elevated CO2 (Cripps et al., 2011). This leads to a considerable reduction in the growth rates of sharks (Pistevos et al., 2015). Being carnivorous predators, similar effects may be expected in cephalopods, a research subject that merits future exploration.
RV and GF are responsible for the conceptual design. RV, GF, and VP contributed to the writing of the manuscript.
The authors declare that the research was conducted in the absence of any commercial or financial relationships that could be construed as a potential conflict of interest.
This work has been supported by the Spanish Ministry of Education and Culture (Grant no. PRX15/00100) and by the research project CALOCEAN-2 (AGL2012-39077) from the Ministry of Economy and Competitiveness of Spain to RV. GF has been supported by RITMARE Flagship Project (Italian Ministry of Education, University and Research–MIUR, and Stazione Zoologica Anton Dohrn-SZN) and by the Stazione Zoologica Anton Dohrn. This study benefited from networking activities carried out under the COST ACTION FA1301, and is considered a contribution to the COST (European Cooperation on Science and Technology) Action FA1301 “A network for improvement of cephalopod welfare and husbandry in research, aquaculture and fisheries.”
Albertin, C. B., Simakov, O., Mitros, T., Wang, Z. Y., Pungor, J. R., Edsinger-Gonzales, E., et al. (2015). The octopus genome and the evolution of cephalopod neural and morphological novelties. Nature 524, 220–224. doi: 10.1038/nature14668
Aldred, R. G., Nixon, M., and Young, J. Z. (1983). Cirrothauma murrayi Chun, a finned octopod. Philos. Trans. R. Soc. Lond. 301, 1–54. doi: 10.1098/rstb.1983.0021
Allcock, A. L., Lindgren, A., and Strugnell, J. M. (2015). The contribution of molecular data to our understanding of cephalopod evolution and systematics: a review. J. Nat. Hist. 49, 1373–1421. doi: 10.1080/00222933.2013.825342
Anderson, R. C., and Mather, J. A. (2007). The packaging problem: bivalve prey selection and prey entry techniques of the octopus Enteroctopus dofleini. J. Comp. Psychol. 121, 300–305. doi: 10.1037/0735-7036.121.3.300
Anderson, R. C., and Shimek, R. L. (2014). Octopuses have a fowl diet. Am. Malacol. Bull. 32, 220–222. doi: 10.4003/006.032.0213
Anderson, R. C., Wood, J. B., and Byrne, R. A. (2002). Octopus senescence: the beginning of the end. J. Appl. Anim. Welfare Sci. 5, 275–283. doi: 10.1207/S15327604JAWS0504_02
Angilletta, M. (1994). Sedentary behavior by green mambas Dendroaspis angusticeps. Herpetol. Nat. Hist. 2, 105–111.
Basil, J. A., Bahctinova, I., Kuroiwa, K., Lee, N., Mims, D., Preis, M., et al. (2005). The function of the rhinophore and the tentacles of Nautilus pompilius L. (Cephalopoda, Nautiloidea) in orientation to odor. Mar. Freshw. Behav. Physiol. 38, 209–221. doi: 10.1080/10236240500310096
Benoit-Bird, K. J., and Gilly, W. F. (2012). Coordinated nocturnal behavior of foraging jumbo squid Dosidicus gigas. Mar. Ecol. Prog. Ser. 455, 211–228. doi: 10.3354/meps09664
Boal, J. G., and Golden, D. K. (1999). Distance chemoreception in the common cuttlefish, Sepia officinalis (Mollusca, Cephalopoda). J. Exp. Mar. Biol. Ecol. 235, 307–317. doi: 10.1016/S0022-0981(98)00187-7
Boal, J. G., Wittenberg, K. M., and Hanlon, R. T. (2000). Observational learning does not explain improvement in predation tactics by cuttlefish (Mollusca: Cephalopoda). Behav. Process. 52, 141–153. doi: 10.1016/S0376-6357(00)00137-6
Boletzky, S. V. (1972). A note on aerial prey-capture by Sepia officinalis (Mollusca, cephalopoda). Vie Milieu 23, 133–140.
Boletzky, S. V. (2003). Biology of early life stages in cephalopod molluscs. Adv. Mar. Biol. 44, 143–203. doi: 10.1016/S0065-2881(03)44003-0
Borrelli, L., and Fiorito, G. (2008). “Behavioral analysis of learning and memory in cephalopods,” in Learning and Memory: a Comprehensive Reference, ed J. J. Byrne. (Oxford: Academic Press), 605–627.
Borrelli, L., Gherardi, F., and Fiorito, G. (2006). A Catalogue of Body Patterning in Cephalopoda. Napoli: Stazione Zoologica A. Dohrn; Firenze University Press.
Boyle, P. R. (1986). Responses to water-borne chemicals by the octopus Eledone cirrhosa (Lamarck, 1798). J. Exp. Mar. Biol. Ecol. 104, 23–30. doi: 10.1016/0022-0981(86)90095-X
Braley, M., Goldsworthy, S. D., Page, B., Steer, M., and Austin, J. J. (2010). Assessing morphological and DNA-based diet analysis techniques in a generalist predator, the arrow squid Nototodarus gouldi. Mol. Ecol. Resour. 10, 466–474. doi: 10.1111/j.1755-0998.2009.02767.x
Budelmann, B., Schipp, R., and Boletzky, S. V. (1997). “Cephalopoda,” in Microscopic Anatomy of Invertebrates, Vol. 6A, eds F. W. Harrison and A. J. Kohn (New York, NY: Wiley-Liss, Inc.,), 119–414.
Budelmann, B. U. (1994). Cephalopod sense organs, nerves and the brain: adaptations for high performance and life style. Mar. Freshw. Behav. Physiol. 25, 13–33. doi: 10.1080/10236249409378905
Budelmann, B. U. (1995). “The cephalopod nervous system: what evolution has made of the molluscan design,” in The Nervous Systems of Invertebrates: An Evolutionary and Comparative Approach, eds O. Breidbach and W. Kutsch (Basel,: Birkhäuser Verlag), 115–138.
Budelmann, B. U. (1996). Active marine predators: the sensory world of cephalopods. Mar. Freshw. Behav. Physiol. 27, 59–75. doi: 10.1080/10236249609378955
Budelmann, B. U., and Bleckmann, H. (1988). A lateral line analogue in cephalopods: water waves generate microphonic potentials in the epidermal head lines of Sepia and Lolliguncula. J. Comp. Physiol. A 164, 1–5. doi: 10.1007/BF00612711
Budelmann, B. U., Riese, U., and Belckmann, H. (1991). “Structure, function, biological significance of the cuttlefish “lateral lines,” in The Cuttlefish, ed E. Boucaud-Camou (Caen: Centre de publications de l'Université de Caen), 201–209.
Bustamante, P., Caurant, F., Fowler, S. W., and Miramand, P. (1998). Cephalopods as a vector for the transfer of cadmium to top marine predators in the north-east Atlantic Ocean. Sci. Total Environ. 220, 71–80. doi: 10.1016/S0048-9697(98)00250-2
Butler, M. J., and Lear, J. A. (2009). Habitat-based intraguild predation by Caribbean reef octopus Octopus briareus on juvenile Caribbean spiny lobster Panulirus argus. Mar. Ecol. Prog. Ser. 386, 115–122. doi: 10.3354/meps08071
Caldwell, R. L., Ross, R., Rodaniche, A., and Huffard, C. L. (2015). Behavior and body patterns of the larger pacific striped octopus. PLoS ONE 10:e0134152. doi: 10.1371/journal.pone.0134152
Cartron, L., Josef, N., Lerner, A., McCusker, S. D., Darmaillacq, A.-S., Dickel, L., et al. (2013). Polarization vision can improve object detection in turbid waters by cuttlefish. J. Exp. Mar. Biol. Ecol. 447, 80–85. doi: 10.1016/j.jembe.2013.02.013
Chen, D. S., Dykhuizen, G., Hodge, J., and Gilly, W. F. (1996). Ontogeny of copepod predation in juvenile squid (Loligo opalescens). Biol. Bull. 190, 69–81. doi: 10.2307/1542676
Chichery, M., and Chichery, R. (1988). Manipulative motor activity of the cuttlefish Sepia officinalis during prey-capture. Behav. Process. 17, 45–56. doi: 10.1016/0376-6357(88)90049-6
Chichery, M. P., and Chichery, R. (1987). The anterior basal lobe and control of prey-capture in the cuttlefish (Sepia officinalis). Physiol. Behav. 40, 329–336. doi: 10.1016/0031-9384(87)90055-2
Chichery, M.-P., and Chichery, R. (1992). Behavioural and neurohistological changes in aging Sepia. Brain Res. 574, 77–84. doi: 10.1016/0006-8993(92)90802-G
Chouvelon, T., Spitz, J., Cherel, Y., Caurant, F., Sirmel, R., Mendez-Fernández, P., et al. (2011). Inter-specific and ontogenic differences in δ13 C and δ15 N values and Hg and Cd concentrations in cephalopods. Mar. Ecol. Prog. Series 433, 107–120. doi: 10.3354/meps09159
Clarke, M. R. (1988). “Evolution of buoyancy and locomotion in recent cephalopods,” in The Mollusca. Paleontology and Neontology of Cephalopods, Vol. 12, eds M. R. Clarke and E. R. Trueman (New York, NY; San Diego, CA: Academic Press), 203–213.
Cole, P. D., and Adamo, S. A. (2005). Cuttlefish (Sepia officinalis: Cephalopoda) hunting behavior and associative learning. Anim. Cogn. 8, 27–30. doi: 10.1007/s10071-004-0228-9
Coll, M., Navarro, J., Olson, R. J., and Christensen, V. (2013). Assessing the trophic position and ecological role of squids in marine ecosystems by means of food-web models. Deep Sea Res. II Top. Stud. Oceanogr. 95, 21–36. doi: 10.1016/j.dsr2.2012.08.020
Collins, M. A., and Villanueva, R. (2006). Taxonomy, ecology and behaviour of the cirrate octopods. Oceanogr. Mar. Biol. Annu. Rev. 44, 277–322. doi: 10.1201/9781420006391.ch6
Cripps, I. L., Munday, P. L., and McCormick, M. I. (2011). Ocean acidification affects prey detection by a predatory reef fish. PLoS ONE 6:e22736. doi: 10.1371/journal.pone.0022736
Darmaillacq, A. S., Chichery, R., Shashar, N., and Dickel, L. (2006). Early familiarization overrides innate prey preference in newly hatched Sepia officinalis cuttlefish. Anim. Behav. 71, 511–514. doi: 10.1016/j.anbehav.2005.04.019
Darmaillacq, A. S., Dickel, L., Chichery, M. P., Agin, V., and Chichery, R. (2004). Rapid taste aversion learning in adult cuttlefish, Sepia officinalis. Anim. Behav. 68, 1291–1298. doi: 10.1016/j.anbehav.2004.01.015
Darmaillacq, A.-S., Lesimple, C., and Dickel, L. (2008). Embryonic visual learning in the cuttlefish, Sepia officinalis. Anim. Behav. 76, 131–134. doi: 10.1016/j.anbehav.2008.02.006
Deagle, B. E., Jarman, S. N., Pemberton, D., and Gales, N. J. (2005). Genetic screening for prey in the gut contents from a giant squid (Architeuthis sp.). J. Hered. 96, 417–423. doi: 10.1093/jhered/esi036
Dickel, L., Chichery, M. P., and Chichery, R. (1997). Postembryonic maturation of the vertical lobe complex and early development of predatory behavior in the cuttlefish (Sepia officinalis). Neurobiol. Learn. Mem. 67, 150–160. doi: 10.1006/nlme.1996.3754
Douglas, R. H., Williamson, R., and Wagner, H. G. (2005). The pupillary response of cephalopods. J. Exp. Biol. 208, 261–265. doi: 10.1242/jeb.01395
Dunstan, A. J., Ward, P. D., and Marshall, N. J. (2011). Nautilus pompilius life history and demographics at the Osprey reef seamount, Coral Sea, Australia. PLoS ONE 6:e16312. doi: 10.1371/journal.pone.0016312
Ebisawa, S. I., Tsuchiya, K., and Segawa, S. (2011). Feeding behavior and oxygen consumption of Octopus ocellatus preying on the short-neck clam Ruditapes philippinarum. J. Exp. Mar. Biol. Ecol. 403, 1–8. doi: 10.1016/j.jembe.2011.03.014
Emery, D. G. (1975). Ciliated sensory cells and associated neurons in the lip of Octopus joubini Robson. Cell Tissue Res. 157, 331–340. doi: 10.1007/BF00225524
Ferguson, S. H., Higdon, J. W., and Westdal, K. H. (2012). Prey items and predation behavior of killer whales (Orcinus orca) in Nunavut, Canada based on Inuit hunter interviews. Aquat. Biosyst. 8:3. doi: 10.1186/2046-9063-8-3
Finn, J. K., Tregenza, T., and Norman, M. D. (2009). Defensive tool use in a coconut-carrying octopus. Curr. Biol. 19, R1069–R1070. doi: 10.1016/j.cub.2009.10.052
Fiorito, G., and Gherardi, F. (1999). Prey-handling behaviour of Octopus vulgaris (Mollusca, Cephalopoda) on bivalve preys. Behav. Process. 46, 75–88. doi: 10.1016/S0376-6357(99)00020-0
Fleisher, K. J., and Case, J. F. (1995). Cephalopod predation facilitated by dinoflagellate luminescence. Biol. Bull. 189, 263–271. doi: 10.2307/1542143
Forsythe, J. W., and Hanlon, R. T. (1997). Foraging and associated behavior by Octopus cyanea Gray, 1849 on a coral atoll, French Polynesia. J. Exp. Mar. Biol. Ecol. 209, 15–31. doi: 10.1016/S0022-0981(96)00057-3
Franco-Santos, R. M., Iglesias, J., Domingues, P. M., and Vidal, E. A. G. (2014). Early beak development in Argonauta nodosa and Octopus vulgaris (Cephalopoda: Incirrata) paralarvae suggests adaptation to different feeding mechanisms. Hydrobiologia 725, 69–83. doi: 10.1007/s10750-013-1721-4
Franco-Santos, R. M., and Vidal, E. A. G. (2014). Beak development of early squid paralarvae (Cephalopoda: Teuthoidea) may reflect an adaptation to a specialized feeding mode. Hydrobiologia 725, 85–103. doi: 10.1007/s10750-013-1715-2
Gagnon, Y. L., Osorio, D. C., Wardill, T. J., Marshall, N. J., Chung, W.-S., and Temple, S. E. (2016). Can chromatic aberration enable color vision in natural environments? Proc. Natl. Acad. Sci. U.S.A. 113, E6908–E6909. doi: 10.1073/pnas.1612239113
Godfrey-Smith, P. (2013). Cephalopods and the evolution of the mind. Pac. Conserv. Biol. 19, 4–9. doi: 10.1071/PC130004
Grisley, M. S., Boyle, P. R., and Key, L. N. (1996). Eye puncture as a route of entry for saliva during predation on crabs by the octopus Eledone cirrhosa (Lamark). J. Exp. Mar. Biol. Ecol. 202, 225–237. doi: 10.1016/0022-0981(96)00035-4
Grisley, M. S., Boyle, P. R., Pierce, G. J., and Key, L. N. (1999). Factors affecting prey handling in lesser octopus (Eledone cirrhosa) feeding on crabs (Carcinus maenas). J. Mar. Biol. Assoc. U.K. 79, 1085–1090. doi: 10.1017/S0025315499001332
Guerra, A. (1997). “Octopus vulgaris: review of the world fishery,” in Proccedings of the Workshop on the Fishery and Market Potential of Octopus in California, eds M. A. Lang and F. G. Hochberg (Washington, DC: Smithsonian Institution), 91–97.
Guerra, A., González, A. F., Rocha, F., Segonzac, M., and Gracia, J. (2002). Observations from submersibles of rare long-arm bathypelagic squids. Sarsia 87, 189–192. doi: 10.1080/003648202320205274
Guerreiro, M., Phillips, R., Cherel, Y., Ceia, F. R., Alvito, P., Rosa, R., et al. (2015). Habitat and trophic ecology of Southern Ocean cephalopods from stable isotope analyses. Mar. Ecol. Prog. Ser. 530, 119–134. doi: 10.3354/meps11266
Guggisberg, C. A. W. (1972). Crocodiles: their Natural History, Folklore and Conservation. Cape Town; Johannesburg: Purnell.
Guibé, M., Boal, J. G., and Dickel, L. (2010). Early exposure to odors changes later visual prey preferences in cuttlefish. Dev. Psychobiol. 52, 833–837. doi: 10.1002/dev.20470
Hanlon, R. T. (1988). Behavioral and body patterning characters useful in taxonomy and field identification of cephalopods. Malacologia 29, 247–264.
Hanlon, R. T., Conroy, L. A., and Forsythe, J. W. (2008). Mimicry and foraging behaviour of two tropical sand-flat octopus species off North Sulawesi, Indonesia. Biol. J. Linn. Soc. 93, 23–38. doi: 10.1111/j.1095-8312.2007.00948.x
Hanlon, R. T., and Messenger, J. B. (1996). Cephalopod Behaviour. Cambridge: Cambridge University Press.
Hayward, M., Henschel, P., O'brien, J., Hofmeyr, M., Balme, G., and Kerley, G. (2006). Prey preferences of the leopard (Panthera pardus). J. Zool. 270, 298–313. doi: 10.1111/j.1469-7998.2006.00139.x
Hernández-Urcera, J., Garci, M. E., Roura, A., González, A. F., Cabanellas-Reboredo, M., Morales-Nin, B., et al. (2014). Cannibalistic behavior of octopus (Octopus vulgaris) in the wild. J. Comp. Psychol. 128, 427–430. doi: 10.1037/a0036883
Hoving, H. J. T., and Haddock, S. H. D. (2017). The giant deep-sea octopus Haliphron atlanticus forages on gelatinous fauna. Sci. Rep. 7:44952. doi: 10.1038/srep44952
Hoving, H.-J. T., Perez, J. A., Bolstad, K. S. R., Braid, H. E., Evans, A. B., Xavier, J. C. C., et al. (2014). The study of deep-sea cephalopods. Adv. Mar. Biol. 67, 235–359. doi: 10.1016/B978-0-12-800287-2.00003-2
Hoving, H. J. T., and Robison, B. H. (2012). Vampire squid: detritivores in the oxygen minimum zone. Proc. R. Soc. B Biol. Sci. 279, 4559–4567. doi: 10.1098/rspb.2012.1357
Hoving, H. J. T., and Robison, B. H. (2016). Deep-sea in situ observations of gonatid squid and their prey reveal high occurrence of cannibalism. Deep Sea Res. Part I Oceanogr. Res. Pap. 116, 94–98. doi: 10.1016/j.dsr.2016.08.001
Hoving, H. J. T., Zeidberg, L. D., Benfield, M. C., Bush, S. L., Robison, B. H., and Vecchione, M. (2013). First in situ observations of the deep-sea squid Grimalditeuthis bonplandi reveal unique use of tentacles. Proc. R. Soc. B Biol. Sci. 280:20131463. doi: 10.1098/rspb.2013.1463
How, M. J., Norman, M. D., Finn, J., Chung, W.-S., and Marshall, N. J. (2017). Dynamic skin patterns in cephalopods. Front. Physiol. 8:393. doi: 10.3389/fphys.2017.00393
Huffard, C. L., Boneka, F., and Full, R. J. (2005). Underwater bipedal locomotion by octopuses in disguise. Science 307, 1927. doi: 10.1126/science.1109616
Hunsicker, M. E., Essington, T. E., Watson, R., and Sumaila, U. R. (2010). The contribution of cephalopods to global marine fisheries: can we have our squid and eat them too? Fish Fish. 11, 421–438. doi: 10.1111/j.1467-2979.2010.00369.x
Ibáñez, C. M., and Keyl, F. (2010). Cannibalism in cephalopods. Rev. Fish Biol. Fish 20, 123–136. doi: 10.1007/s11160-009-9129-y
Iglesias, J., Fuentes, L., and Villanueva, R. (Eds.) (2014). Cephalopod Culture. New York, NY; Heidelberg; Dordrecht: Springer, 494.
Iglesias, J., Sánchez, F. J., Bersano, J. G. F., Carrasco, J. F., Dhont, J., Fuentes, L., et al. (2007). Rearing of Octopus vulgaris paralarvae: present status, bottlenecks and trends. Aquaculture 266, 1–15. doi: 10.1016/j.aquaculture.2007.02.019
Jacoby, C. A., Youngbluth, M. J., Frost, J. R., Flood, P. R., Uiblein, F., Båmstedt, U., et al. (2009). Vertical distribution, behavior, chemical composition and metabolism of Stauroteuthis syrtensis (Octopoda: Cirrata) in the northwest Atlantic. Aquat. Biol. 5, 13–22. doi: 10.3354/ab00117
Jastrebsky, R. A., Bartol, I. K., and Krueger, P. S. (2017). Turning performance of brief squid Lolliguncula brevis during attacks on shrimp and fish. J. Exp. Biol. 220, 908–919. doi: 10.1242/jeb.144261
Johnsen, S., Balser, E. J., Fisher, E. C., and Widder, E. A. (1999). Bioluminescence in the deep-sea cirrate octopod Stauroteuthis syrtensis Verrill (Mollusca: Cephalopoda). Biol. Bull. 197, 26–39. doi: 10.2307/1542994
Kasugai, T., Shigeno, S., and Ikeda, Y. (2004). Feeding and external digestion in the Japanese pigmy squid Idiosepius paradoxus (Cephalopoda: Idiosepiidae). J. Molluscan Stud. 70, 231–236. doi: 10.1093/mollus/70.3.231
Kelley, J. L., and Davies, W. I. L. (2016). The biological mechanisms and behavioral functions of opsin-based light detection by the skin. Front. Ecol. Evol. 4:106. doi: 10.3389/fevo.2016.00106
Kingston, A. C. N., Kuzirian, A. M., Hanlon, R. T., and Cronin, T. W. (2015). Visual phototransduction components in cephalopod chromatophores suggest dermal photoreception. J. Exp. Biol. 218, 1596–1602. doi: 10.1242/jeb.117945
Komak, S., Boal, J. G., Dickel, L., and Budelmann, B. U. (2005). Behavioural responses of juvenile cuttlefish (Sepia officinalis) to local water movements. Mar. Fresw. Behav. Physiol. 38, 117–125. doi: 10.1080/10236240500139206
Krajewski, J. P., Bonaldo, R. M., Sazima, C., and Sazima, I. (2009). Octopus mimicking its follower reef fish. J. Nat. Hist. 43, 185–190. doi: 10.1080/00222930802450965
Kroeker, K. J., Sanford, E., Jellison, B. M., and Gaylord, B. (2014). Predicting the effects of ocean acidification on predator-prey interactions: a conceptual framework based on coastal molluscs. Biol. Bull. 226, 211–222. doi: 10.1086/BBLv226n3p211
Kubodera, T., and Mori, K. (2005). First-ever observations of a live giant squid in the wild. Proc. Royal Soc. Lond. B Biol. Sci. 272, 2583–2586. doi: 10.1098/rspb.2005.3158
Lee, P. G. (1992). Chemotaxis by Octopus maya Voss et Solís in a Y-maze. J. Exp. Mar. Biol. Ecol. 156, 53–67. doi: 10.1016/0022-0981(92)90016-4
Leite, T. S., Batista, A. T., Lima, F. D., Barbosa, J. C., and Mather, J. (2016). Geographic variability of Octopus insularis diet: from oceanic island to continental populations. Aquat. Biol. 25, 17–27. doi: 10.3354/ab00655
Leite, T. S., Haimovici, M., and Mather, J. (2009). Octopus insularis (Octopodidae), evidences of a specialized predator and a time-minimizing hunter. Mar. Biol. 156, 2355–2367. doi: 10.1007/s00227-009-1264-4
Lenz, S., Sundermann, G., and Fioroni, P. (1995). The epidermal lines of Octopus vulgaris Lamarck, 1798, and Sepiola affinis Naef, 1912 (Mollusca: Cephalopoda) at hatching state. Zool. Anz. 234, 145–157.
Lorrain, A., Arguelles, J., Alegre, A., Bertrand, A., Munaron, J. M., Richard, P., et al. (2011). Sequential isotopic signature along gladius highlights contrasted individual foraging strategies of Jumbo squid (Dosidicus gigas). PLoS ONE 6:e22194. doi: 10.1371/journal.pone.0022194
Marliave, J. B. (1981). Neustonic feeding in early larvae of Octopus dofleini (Wülker). Veliger 23, 350–351.
Marshall, N., and Messenger, J. (1996). Colour-blind camouflage. Nature 382, 408. doi: 10.1038/382408b0
Martin, R. A., Hammerschlag, N., Collier, R. S., and Fallows, C. (2005). Predatory behaviour of white sharks (Carcharodon carcharias) at Seal Island, South Africa. J. Mar. Biol. Assoc. U.K. 85, 1121–1135. doi: 10.1017/S002531540501218X
Mather, J. A. (1986). A female-dominated feeding hierarchy in juvenile Sepia officinalis in the laboratory. Mar. Behav. Physiol. 12, 233–244. doi: 10.1080/10236248609378650
Mäthger, L. M., Barbosa, A., Miner, S., and Hanlon, R. T. (2006). Color blindness and contrast perception in cuttlefish (Sepia officinalis) determined by a visual sensorimotor assay. Vis. Res. 46, 1746–1753. doi: 10.1016/j.visres.2005.09.035
McQuaid, C. D. (1994). Feeding behavior and selection of bivalve prey by Octopus vulgaris Cuvier. J. Exp. Mar. Biol. Ecol. 177, 187–202. doi: 10.1016/0022-0981(94)90236-4
Messenger, J. B. (1968). The visual attack of the cuttlefish Sepia officinalis. Anim. Behav. 16, 342–357. doi: 10.1016/0003-3472(68)90020-1
Messenger, J., Wilson, A., and Hedge, A. (1973). Some evidence for colour-blindness in Octopus. J. Exp. Biol. 59, 77–94.
Monroig, Ó., Tocher, D., and Navarro, J. (2013). Biosynthesis of polyunsaturated fatty acids in marine invertebrates: recent advances in molecular mechanisms. Mar. Drugs 11, 3998–4018. doi: 10.3390/md11103998
Moynihan, M., and Rodaniche, A. F. (1982). The behavior and natural history of the caribbean reef squid Sepioteuthis sepioidea. With a consideration of social, signal and defensive patterns for difficult and dangerous environments. Adv. Ethol. 25, 1–150.
Nabhitabhata, J. (2014a). “Sepia pharaonis,” in Cephalopod Culture, eds J. Iglesias, L. Fuentes, and R. Villanueva (New York, NY: Springer Netherlands), 205–224.
Nabhitabhata, J. (2014b). “Sepiella inermis,” in Cephalopod Culture, eds J. Iglesias, L. Fuentes, and R. Villanueva (New York, NY: Springer Netherlands), 225–240.
Nabhitabhata, J., and Ikeda, I. (2014). “Sepioteuthis lessoniana,” in Cephalopod Culture, eds J. Iglesias, L. Fuentes, and R. Villanueva (New York, NY: Springer Netherlands), 315–347.
Navarro, J. C., Monroig, Ó., and Sykes, A. V. (2014). “Nutrition as a key factor for cephalopod aquaculture,” in Cephalopod Culture, eds J. Iglesias, L. Fuentes and R. Villanueva (New York, NY: Springer Netherlands), 77–95.
Nilsson, D. E., Warrant, E. J., Johnsen, S., Hanlon, R., and Shashar, N. (2012). A unique advantage for giant eyes in giant squid. Curr. Biol. 22, 683–688. doi: 10.1016/j.cub.2012.02.031
Nixon, M. (1980). Salivary papilla of Octopus as an accessory radula for drilling shells. J. Zool. 190, 53–57. doi: 10.1111/j.1469-7998.1980.tb01422.x
Nixon, M. (1987). “Cephalopod diets,” in Cephalopod Life Cycles. Comparative Reviews, Vol. 2, ed P. R. Boyle (London: Academic Press), 201–219.
O'Brien, C. E., Mezrai, N., Darmaillacq, A.-S., and Dickel, L. (2016). Behavioral development in embryonic and early juvenile cuttlefish (Sepia officinalis). Dev. Psychobiol. 59, 145–160. doi: 10.1002/dev.21476
O'Dor, R. K., Helm, P., and Balch, N. (1985). Can rhynchoteuthions suspension feed? (Mollusca: Cephalopoda). Vie et Milieu 35, 267–271.
Ohkouchi, N., Tsuda, R., Chikaraishi, Y., and Tanabe, K. (2013). A preliminary estimate of the trophic position of the deep-water ram's horn squid Spirula spirula based on the nitrogen isotopic composition of amino acids. Mar. Biol. 160, 773–779. doi: 10.1007/s00227-012-2132-1
Olmos-Pérez, L., Roura, Á., Pierce, G. J., Boyer, S., and González, Á. F. (2017). Diet composition and variability of wild Octopus vulgaris and Alloteuthis media (Cephalopoda) paralarvae through a metagenomic lens. Front. Physiol. 8:321. doi: 10.3389/fphys.2017.00321
Olson, R. J., Roman-Verdesoto, M. H., and Macias-Pita, G. L. (2006). Bycatch of jumbo squid Dosidicus gigas in the tuna purse-seine fishery of the eastern Pacific Ocean and predatory behaviour during capture. Fish. Res. 79, 48–55. doi: 10.1016/j.fishres.2006.02.012
Packard, A. (1963). The behaviour of Octopus vulgaris. Bull. l'Inst. Océanogr. (Monaco) Numéro Spécial 1D, 35–49.
Packard, A. (1972). Cephalopods and fish: the limits of convergence. Biol. Rev. 47, 241–307. doi: 10.1111/j.1469-185X.1972.tb00975.x
Passarella, K. C., and Hopkins, T. L. (1991). Species composition and food habits of the micronektonic cephalopod assemblage in the eastern Gulf of Mexico. Bull. Mar. Sci. 49, 638–659.
Pech-Puch, D., Cruz-López, H., Canche-Ek, C., Campos-Espinosa, G., García, E., Mascaro, M., et al. (2016). Chemical tools of Octopus maya during crab predation are also active on conspecifics. PLoS ONE 11:e0148922 doi: 10.1371/journal.pone.0148922
Pethybridge, H. R., Nichols, P. D., Virtue, P., and Jackson, G. D. (2013). The foraging ecology of an oceanic squid, Todarodes filippovae: the use of signature lipid profiling to monitor ecosystem change. Deep Sea Res. II Top. Stud. Oceanogr. 95, 119–128. doi: 10.1016/j.dsr2.2012.07.025
Pistevos, J. C. A., Nagelkerken, I., Rossi, T., Olmos, M., and Connell, S. D. (2015). Ocean acidification and global warming impair shark hunting behaviour and growth. Sci. Rep. 5:16293. doi: 10.1038/srep16293
Portela, E., Simoes, N., Rosas, C., and Mascaró, M. (2014). Can preference for crabs in juvenile Octopus maya be modified through early experience with alternative prey? Behaviour 151, 1597–1616. doi: 10.1163/1568539X-00003206
Ramirez, M. D., and Oakley, T. H. (2015). Eye-independent, light-activated chromatophore expansion (LACE) and expression of phototransduction genes in the skin of Octopus bimaculoides. J. Exp. Biol. 218, 1513–1520. doi: 10.1242/jeb.110908
Reis, D. B., Acosta, N. G., Almansa, E., Navarro, J. C., Tocher, D. R., Monroig, O., et al. (2014). In vivo metabolism of unsaturated fatty acids in Octopus vulgaris hatchlings determined by incubation with 14C-labelled fatty acids added directly to seawater as protein complexes. Aquaculture 431, 28–33. doi: 10.1016/j.aquaculture.2014.05.016
Robison, B. H., Reisenbichler, K. R., Hunt, J. C., and Haddock, S. H. (2003). Light production by the arm tips of the deep-sea cephalopod Vampyroteuthis infernalis. Biol. Bull. 205, 102–109. doi: 10.2307/1543231
Rodhouse, P. G., and Nigmatullin, C. M. (1996). Role as consumers. Philos. Trans. R. Soc. Lond. Ser. B Biol. Sci. 351, 1003–1022. doi: 10.1098/rstb.1996.0090
Rosa, R., Pimentel, M., Baptista, M., Trübenbach, K., Calado, R., Nunes, M., et al. (2013). Trophic and reproductive biochemistry of a deep-sea gelatinous octopus, Opisthoteuthis calypso. Mar. Biol. 160, 263–275. doi: 10.1007/s00227-012-2084-5
Rosa, R., and Seibel, B. A. (2008). Synergistic effects of climate-related variables suggest future physiological impairment in a top oceanic predator. Proc. Natl. Acad. Sci. U.S.A. 105, 20776–20780. doi: 10.1073/pnas.0806886105
Rosa, R., and Seibel, B. A. (2010). Slow pace of life of the Antarctic colossal squid. J. Mar. Biol. Assoc. U.K. 90, 1375–1378. doi: 10.1017/S0025315409991494
Rosas, C., Gallardo, P., Mascaro, M., Caamal-Monsreal, C., and Pascual, C. (2014). “Octopus maya,” in Cephalopod Culture, eds J. Iglesias, L. Fuentes, and R. Villanueva (New York, NY: Springer Netherlands), 383–396.
Roura, Á., Antón Álvarez-Salgado, X., González, Á. F., Gregori, M., Rosón, G., Otero, J., et al. (2016). Life strategies of cephalopod paralarvae in a coastal upwelling system (NW Iberian Peninsula): insights from zooplankton community and spatio-temporal analyses. Fish. Oceanogr. 25, 241–258. doi: 10.1111/fog.12151
Roura, Á., Doyle, S. R., Nande, M., and Strugnell, J. M. (2017). You are what you eat: a genomic analysis of the gut microbiome of captive and wild Octopus vulgaris paralarvae and their zooplankton prey. Front. Physiol. 8:362. doi: 10.3389/fphys.2017.00362
Roura, Á., González, Á., Redd, K., and Guerra, Á. (2012). Molecular prey identification in wild Octopus vulgaris paralarvae. Mar. Biol. 159, 1335–1345. doi: 10.1007/s00227-012-1914-9
Runham, N. W., Bailey, C. J., Carr, M., Evans, C. A., and Malham, S. (1997). Hole drilling in crab and gastropod shells by Eledone cirrhosa (Lamarck, 1978). Sci. Mar. 61, 67–76.
Sato, N., Takeshita, F., Fujiwara, E., and Kasugai, T. (2016). Japanese pygmy squid (Idiosepius paradoxus) use ink for predation as well as for defence. Mar. Biol. 163, 1–5. doi: 10.1007/s00227-016-2833-y
Seibel, B. (2015). Environmental physiology of the jumbo squid, Dosidicus gigas (d'Orbigny, 1835) (Cephalopoda: Ommastrephidae): implications for changing climate. Am. Malacol. Bull. 33, 161–173. doi: 10.4003/006.033.0113
Seibel, B. A., and Carlini, D. B. (2001). Metabolism of pelagic cephalopods as a function of habitat depth: a reanalysis using phylogenetically independent contrasts. Biol. Bull. 201, 1–5. doi: 10.2307/1543519
Seibel, B. A., and Childress, J. J. (2000). Metabolism of benthic octopods (Cephalopoda) as a function of habitat depth and oxygen concentration. Deep Sea Res. I Oceanogr. Res. Pap. 47, 1247–1260. doi: 10.1016/S0967-0637(99)00103-X
Seibel, B. A., Thuesen, E. V., and Childress, J. J. (2000). Light-limitation on predator-prey interactions: consequences for metabolism and locomotion of deep-sea cephalopods. Biol. Bull. 198, 284–298. doi: 10.2307/1542531
Seibel, B. A., Thuesen, E. V., Childress, J. J., and Gorodezky, L. A. (1997). Decline in pelagic cephalopod metabolism with habitat depth reflects differences in locomotory efficiency. Biol. Bull. 192, 262–278. doi: 10.2307/1542720
Shashar, N., Hanlon, R. T., and Petz, A. deM. (1998). Polarization vision helps detect transparent prey. Nature 393, 222–223. doi: 10.1038/30380
Shigeno, S. (2017). “Brain evolution as an information flow designer: the ground architecture for biological and artificial general intelligence,” in Brain Evolution by Design: From Neural Origin to Cognitive Architecture, eds S. Shigeno, Y. Murakami, and T. Nomura (Tokyo: Springer Japan), 415–438.
Shigeno, S., Kidokoro, H., Goto, T., Tsuchiya, K., and Segawa, S. (2001a). Early ontogeny of the Japanese common squid Todarodes pacificus (Cephalopoda, Ommastrephidae) with special reference to its characteristic morphology and ecological significance. Zool. Sci. 18, 1011–1026. doi: 10.2108/zsj.18.1011
Shigeno, S., Kidokoro, H., Tsuchiya, K., Segawa, S., and Yamamoto, M. (2001b). Development of the brain in the oegopsid squid, Todarodes pacificus: an atlas from hatchling to juvenile. Zool. Sci. 18, 1081–1096. doi: 10.2108/zsj.18.1081
Shigeno, S., and Yamamoto, M. (2005). Embryonic brain development of Loliginids: axonal scaffold and neuropil formation related to early life styles. Phuket Mar. Biol. Center Res. Bull. 66, 155–165.
Spady, B., Watson, S.-A., Chase, T., and Munday, P. (2014). Projected near-future CO2 levels increase activity and alter defensive behaviours in the tropical squid Idiosepius pygmaeus. Biol. Open 3, 1063–1070. doi: 10.1242/bio.20149894
Steer, M. A., and Semmens, J. M. (2003). Pulling or drilling, does size or species matter? an experimental study of prey handling in Octopus dierythraeus (Norman, 1992). J. Exp. Mar. Biol. Ecol. 290, 165–178. doi: 10.1016/S0022-0981(03)00076-5
Stubbs, A. L., and Stubbs, C. W. (2016). Spectral discrimination in color blind animals via chromatic aberration and pupil shape. Proc. Natl. Acad. Sci. U.S.A. 113, 8206–8211. doi: 10.1073/pnas.1524578113
Sugimoto, C., and Ikeda, Y. (2013). Comparison of the ontogeny of hunting behavior in pharaoh cuttlefish (Sepia pharaonis) and oval squid (Sepioteuthis lessoniana). Biol. Bull. 225, 50–59. doi: 10.1086/BBLv225n1p50
Sundermann, G. (1983). The fine structure of epidermal lines on arms and head of postembryonic Sepia officinalis and Loligo vulgaris (Mollusca, Cephalopoda). Cell Tissue Res. 232, 669–677. doi: 10.1007/BF00216437
Sweeney, M. J., Roper, C. F. E., Mangold, K. M., Clarke, M. R., and Boletzky, S. V. (1992). “Larval” and Juvenile Cephalopods: A Manual for their Identification. Washington, DC: Smithsonian Institution Press.
Uchikawa, K., Sakai, M., Wakabayashi, T., and Ichii, T. (2009). The relationship between paralarval feeding and morphological changes in the proboscis and beaks of the neon flying squid Ommastrephes bartramii. Fish. Sci. 75, 317–323. doi: 10.1007/s12562-008-0036-2
Varó, I., Cardenete, G., Hontoria, F., Monroig, Ó., Iglesias, J., Otero, J. J., et al. (2017). Dietary effect on the proteome of the common octopus (Octopus vulgaris) paralarvae. Front. Physiol. 8:309. doi: 10.3389/fphys.2017.00309
Vecchione, M., Young, R. E., Guerra, A., Lindsay, D. J., Clague, D. A., Bernhard, J. M., et al. (2001). Worldwide observations of remarkable deep-sea squids. Science 294, 2505–2506. doi: 10.1126/science.294.5551.2505
Vidal, E. A. G., and Haimovici, M. (1998). Feeding and the possible role of the proboscis and mucus cover in the ingestion of microorganisms by rhynchoteuthion paralarvae (Cephalopoda:Ommastrephidae). Bull. Mar. Sci. 63, 305–316.
Vidal, E. A. G., Villanueva, R., Andrade, J. P., Gleadall, I. G., Iglesias, J., Koueta, N., et al. (2014). Cephalopod culture: current status of main biological models and research priorities. Adv. Mar. Biol. 67, 1–98. doi: 10.1016/B978-0-12-800287-2.00001-9
Villanueva, R., and Bustamante, P. (2006). Composition in essential and non-essential elements of early stages of cephalopods and dietary effects on the elemental profiles of Octopus vulgaris paralarvae. Aquaculture 261, 225–240. doi: 10.1016/j.aquaculture.2006.07.006
Villanueva, R., Koueta, N., Riba, J., and Boucaud-Camou, E. (2002). Growth and proteolytic activity of Octopus vulgaris paralarvae with different food rations during first-feeding, using Artemia nauplii and compound diets. Aquaculture 205, 269–286. doi: 10.1016/S0044-8486(01)00678-0
Villanueva, R., and Norman, M. D. (2008). Biology of the planktonic stages of benthic octopuses. Oceanogr. Mar. Biol. Annu. Rev. 46, 105–202. doi: 10.1201/9781420065756.ch4
Villanueva, R., Nozais, C., and Boletzky, S. V. (1997). Swimming behaviour and food searching in planktonic Octopus vulgaris Cuvier from hatching to settlement. J. Exp. Mar. Biol. Ecol. 208, 169–184. doi: 10.1016/S0022-0981(96)02670-6
Villanueva, R., and Sánchez, P. (1993). Cephalopods of the Benguela current off Namibia: new additions and considerations on the genus Lycoteuthis. J. Nat. Hist. 27, 15–46. doi: 10.1080/00222939300770021
Villanueva, R., Vidal, E. A. G., Fernández-Álvarez, F. Á., and Nabhitabhata, J. (2016). Early mode of life and hatchling size in cephalopod molluscs: influence on the species distributional ranges. PLoS ONE 11:e0165334. doi: 10.1371/journal.pone.0165334
Voight, J. R. (2000). A deep-sea octopus (Graneledone cf. boreopacifica) as a shell-crushing hydrothermal vent predator. J. Zool. 252, 335–341. doi: 10.1111/j.1469-7998.2000.tb00628.x
Warnke, K. (1994). Some aspects of social interaction during feeding in Sepia officinalis (Mollusca: Cephalopoda) hatched and reared in the laboratory. Vie et Milieu 44, 125–131.
Warrant, E. J., and Locket, N. A. (2004). Vision in the deep sea. Biol. Rev. 79, 671–712. doi: 10.1017/S1464793103006420
Wells, M. J. (1994). The evolution of a racing snail. Mar. Freshw. Behav. Physiol. 25, 1–12. doi: 10.1080/10236249409378904
Whitelaw, B. L., Strugnell, J. M., Faou, P., Da Fonseca, R. R., Hall, N. E., Norman, M., et al. (2016). Combined transcriptomic and proteomic analysis of the posterior salivary gland from the southern blue-ringed octopus and the southern sand octopus. J. Proteome Res. 15, 3284–3297. doi: 10.1021/acs.jproteome.6b00452
Wodinsky, J. (1977). Hormonal inhibition of feeding and death in octopus: control by optic gland secretion. Science 198, 948–951. doi: 10.1126/science.198.4320.948
Wodinsky, J. (1978). Feeding behaviour of broody female Octopus vulgaris. Anim. Behav. 26, 803–813. doi: 10.1016/0003-3472(78)90145-8
Yang, T.-I., and Chiao, C.-C. (2016). Number sense and state-dependent valuation in cuttlefish. Proc. R. Soc. B Biol. Sci. 283, 1837. doi: 10.1098/rspb.2016.1379
Yarnall, J. L. (1969). Aspects of the behaviour of Octopus cyanea Gray. Anim. Behav. 17, 747–754. doi: 10.1016/S0003-3472(69)80022-9
Young, J. Z. (1977). Brain, behaviour and evolution of cephalopods. Symp. Zool. Soc. Lond. 38, 377–434.
Keywords: predation, feeding behavior, prey capture
Citation: Villanueva R, Perricone V and Fiorito G (2017) Cephalopods as Predators: A Short Journey among Behavioral Flexibilities, Adaptions, and Feeding Habits. Front. Physiol. 8:598. doi: 10.3389/fphys.2017.00598
Received: 07 June 2017; Accepted: 03 August 2017;
Published: 17 August 2017.
Edited by:
Eduardo Almansa, Instituto Español de Oceanografía (IEO), SpainReviewed by:
Francisco Javier Rocha, University of Vigo, SpainCopyright © 2017 Villanueva, Perricone and Fiorito. This is an open-access article distributed under the terms of the Creative Commons Attribution License (CC BY). The use, distribution or reproduction in other forums is permitted, provided the original author(s) or licensor are credited and that the original publication in this journal is cited, in accordance with accepted academic practice. No use, distribution or reproduction is permitted which does not comply with these terms.
*Correspondence: Roger Villanueva, cm9nZXJAaWNtLmNzaWMuZXM=
Disclaimer: All claims expressed in this article are solely those of the authors and do not necessarily represent those of their affiliated organizations, or those of the publisher, the editors and the reviewers. Any product that may be evaluated in this article or claim that may be made by its manufacturer is not guaranteed or endorsed by the publisher.
Research integrity at Frontiers
Learn more about the work of our research integrity team to safeguard the quality of each article we publish.