- 1Maxillo-Oral Disorders, Tohoku University Hospital, Sendai, Japan
- 2Department of Orthodontics, School of Dental Medicine, Tsurumi University, Yokohama, Japan
Periodontitis, an inflammatory disease that affects the tissues surrounding the teeth, is a common disease worldwide. It is caused by a dysregulation of the host inflammatory response to bacterial infection, which leads to soft and hard tissue destruction. In particular, it is the excessive inflammation in response to bacterial plaque that leads to the release of reactive oxygen species (ROS) from neutrophils, which, then play a critical role in the destruction of periodontal tissue. Generally, ROS produced from immune cells exhibit an anti-bacterial effect and play a role in host defense and immune regulation. Excessive ROS, however, can exert cytotoxic effects, cause oxidative damage to proteins, and DNA, can interfere with cell growth and cell cycle progression, and induce apoptosis of gingival fibroblasts. Collectively, these effects enable ROS to directly induce periodontal tissue damage. Some ROS also act as intracellular signaling molecules during osteoclastogenesis, and can thus also play an indirect role in bone destruction. Cells have several protective mechanisms to manage such oxidative stress, most of which involve production of cytoprotective enzymes that scavenge ROS. These enzymes are transcriptionally regulated via NRF2, Sirtuin, and FOXO. Some reports indicate an association between periodontitis and these cytoprotective enzymes' regulatory axes, with superoxide dismutase (SOD) the most extensively investigated. In this review article, we discuss the role of oxidative stress in the tissue destruction manifest in periodontitis, and the mechanisms that protect against this oxidative stress.
Introduction
Periodontitis is a common disease worldwide of the tissues surrounding the teeth, and is caused by bacterial infection. It is characterized by a dysregulation of the host inflammatory response, which eventually results in soft and hard tissue destruction (Mercado et al., 2003; Bartold et al., 2005). Tissue destruction in periodontitis is considered to result from an excessive inflammatory response to bacterial plaque. This leads to release of reactive oxygen species (ROS), such as hydrogen peroxide and superoxide, from neutrophils (Waddington et al., 2000; Canakci et al., 2005; Dahiya et al., 2013; Miricescu et al., 2014; Callaway and Jiang, 2015; White et al., 2016), which results in oxidative stress—an imbalance between the production of ROS and antioxidant defenses.
Generally, ROS produced from immune cells exhibit an anti-bacterial effect and play a role in host defense and immune regulation (Baehner et al., 1975; Canakci et al., 2005). Excessive ROS however, can exert cytotoxic effects (Esterbauer et al., 1991), cause oxidative damage to proteins and DNA (Wells et al., 2009), can interfere with cell growth and cell cycle progression (Chang et al., 2013), and induce apoptosis (Yu et al., 2012) of gingival fibroblasts. This collectively enables ROS to directly induce periodontal tissue damage. Some ROS are also indirectly involved in periodontal tissue destruction through their role as intracellular signaling molecules in the osteoclastogenic pathway (Ha et al., 2004), and indeed, excessive activation of osteoclasts is a typical pathology in severe periodontitis.
Cells normally have several protective mechanisms against these oxidative stressors (Furukawa-Hibi et al., 2005; Kensler et al., 2007; Hsu et al., 2008), most of which involve the induction of cytoprotective enzymes that scavenge ROS (Mates and Sanchez-Jimenez, 1999). One protective mechanism involves nuclear factor E2-related factor 2 (NRF2), a master regulatory transcription factor for the synthesis of cytoprotective enzymes. NRF2 has been reported to be a negative regulator of osteoclastogenesis (Kanzaki et al., 2013, 2014, 2015).
The relationship between periodontitis and ROS is illustrated in Figure 1. In this review article, we will discuss; (1) the role of oxidative stress in tissue destruction in periodontitis, and (2) mechanisms that protect against oxidative stress.
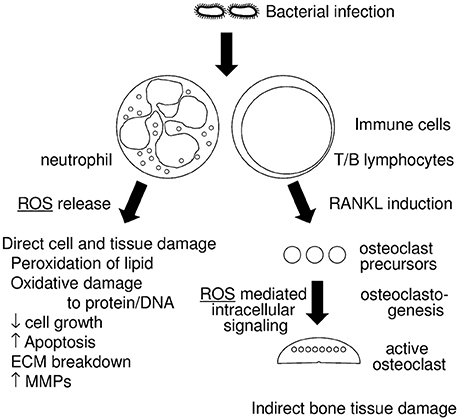
Figure 1. Diagram of the relationship between periodontitis and reactive oxygen species (ROS). Infections with periodontopathogenic bacteria induce host immune responses, which includes neutrophil and T/B lymphocyte activation. Activated neutrophils generate ROS that have an anti-bacterial effect and are thus a form of host defense. Excessive ROS, however, result in direct cell and tissue damage, which includes (1) peroxidation of lipids, (2) oxidative damage to protein and DNA, (3) decrease of cell growth, (4) increased apoptosis, (5) breakdown of the extracellular matrix (ECM), (6) induction of matrix metalloproteases (MMPs). Activated T/B lymphocytes induce RANKL in periodontal tissue, which results in the augmentation of osteoclastogenesis. Some ROS work as intracellular signaling molecules in RANKL stimulation.
The Role of Oxidative Stress in the Tissue Destruction in Periodontitis
Increased ROS are a hallmark of inflammation induced by neutrophils against invading bacteria, and are involved in tissue destruction (Sheikhi et al., 2000, 2001; Chapple et al., 2007; Matthews et al., 2007a). Periodontal tissue destruction is caused, in part, by neutrophils exhibiting an excessive inflammatory response to bacterial plaque (Canakci et al., 2005; Miricescu et al., 2014), with a high volume of ROS also generated by neutrophils in periodontal tissues with chronic periodontitis (Matthews et al., 2007b). ROS production in neutrophils is driven, in part, by nicotinamide adenine dinucleotide phosphate oxidase (NOX) and the purine degradation pathway, which is significantly accelerated in inflamed periodontal tissue (Giannopoulou et al., 2008; Barnes et al., 2009).
The release of ROS plays a critical role in periodontitis-associated tissue destruction, where ROS exert both direct and indirect effects on bone destruction. Directly, it can induce cytotoxic effects and oxidative damage to proteins and DNA. It can also interfere with cell growth and cell cycle progression (Chang et al., 2013), induce apoptosis (Yu et al., 2012) of gingival fibroblasts, and cause matrix degradation via the induction of matrix proteinases. Indirectly, ROS acts as an intracellular signaling molecule during osteoclastogenesis; an important process in hard tissue degeneration.
ROS exert cytotoxicity, such as peroxidation of lipids and phospholipids, against cells as well as the extracellular matrix (ECM). Additionally, protein aggregation through ROS-mediated oxidation (Squier and Bigelow, 2000; Squier, 2001; Friguet, 2002) leads to the breakdown of cell/tissue homeostasis (Hohn et al., 2014). ROS can also stimulate ECM degradation by inducing the breakdown of glycosaminoglycan (Fuchs and Schiller, 2014) and matrix proteinases (Dasgupta et al., 2009, 2010; Kar et al., 2010).
In addition to having a direct cytotoxic effect, ROS can indirectly promote hard tissue degeneration through their role in osteoclastogenesis. Intracellular signaling molecules that mediate osteoclastogenesis, a signaling cascade central to the destruction of alveolar bone, include ROS (Bax et al., 1992; Ha et al., 2004). In hard tissue destruction, alveolar bone resorption is driven by osteoclasts, the tissue-specific macrophage polykaryon created by the differentiation of monocyte/macrophage precursors cells on the bone surface (Teitelbaum, 2000). Generation of osteoclasts requires physical contact between osteoclast precursor cells and specific mesenchymal cells, such as marrow stromal cells or osteoblasts (Udagawa et al., 1990). The key osteoclastogenic cytokine receptor activator of nuclear factor-kB ligand (RANKL) is a membrane-bound protein on osteoblasts and their precursors, which is recognized by the receptor, RANK, on marrow macrophages, thus prompting them to differentiate into osteoclasts (Lacey et al., 1998; Yasuda et al., 1998). In normal physiological circumstances, RANKL is principally expressed by mesenchymal cells of the osteoblast lineage, but in states of skeletal inflammation (Kong et al., 1999), as well as in periodontitis (Kawai et al., 2006), RANKL is produced in abundance by lymphocytes. Other key factors involved in osteoclastogenesis include TRAF6, RAC1, and NOX (Abo et al., 1991; Wong et al., 1998; Wang et al., 2008; Sasaki et al., 2009a). The NOX homologs, NOX1, and NOX2, complementarily generate ROS during osteoclastogenesis (Sasaki et al., 2009b).
Several reports have suggested that ROS signaling can lead to activation of mitogen-activated protein kinase (MAPK), phosphoinositide-3 kinase (PI3K), and nuclear factor kappa-light-chain-enhancer of activated B cells (NF-kB) (Thannickal and Fanburg, 2000; Droge, 2002). NF-kB, which mediates IκBα phosphorylation and degradation, was the first transcription factor shown to respond to ROS (Schreck et al., 1991). Pretreatment of osteoclasts with antioxidants, to inhibit oxidation, was shown to reduce RANKL-induced AKT, NF-kB, and extracellular signal-regulated kinase (ERK) activation (Ha et al., 2004). Coincidentally, NF-kB also plays a pivotal role in cytokine-induced periodontal tissue damage (Chapple, 1997). Application of an antioxidant inhibited the responses of osteoclast precursors to RANKL; including activation of c-Jun N-terminal kinase, p38 MAPK and ERK, and inhibited osteoclast differentiation (Lee et al., 2005). RANKL-mediated ROS induces long lasting Ca2+ oscillations that activate the transcription factor, nuclear factor of activated T-cells, cytoplasmic 1 (NFATC1) (Kim et al., 2010). Taken together, ROS cause hard tissue destruction via osteoclastogenesis, as well as soft tissue destruction.
Mechanisms that Protect Against Oxidative Stress
Cells normally possess several regulatory pathways that protect against oxidative stress (Furukawa-Hibi et al., 2005; Kensler et al., 2007; Hsu et al., 2008), by producing cytoprotective enzymes that scavenge ROS (Mates and Sanchez-Jimenez, 1999). NRF2 (Thimmulappa et al., 2002), Sirtuin (Chen et al., 2011), and FOXO (Liu et al., 2005) are major regulatory pathways for cytoprotective enzymes (Kanzaki et al., 2016a). Figure 2 summarizes the linking the periodontitis, the regulatory pathways of ROS scavenging enzymes, and the defense mechanism against tissue destruction.
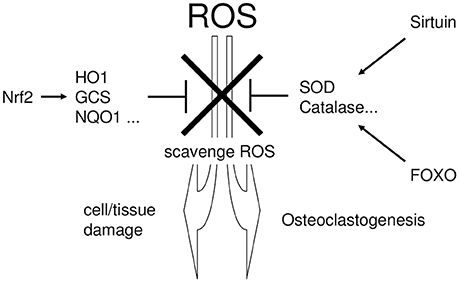
Figure 2. The linking the periodontitis, the regulatory pathways of ROS scavenging enzymes, and the defense mechanism against tissue destruction. Excessive ROS exhibit cytotoxicity and induce tissue destruction in periodontal tissue. To protect such ROS-mediated cytotoxicity, cells possess several regulatory pathways which regulate the production of cytoprotective enzymes that scavenge ROS. NRF2, Sirtuin, and FOXO are situated in the major regulatory pathways for cytoprotective enzyme production.
NRF2 transcriptionally induces cytoprotective enzymes, such as heme oxygenase-1 (HO-1) (Alam et al., 1999), NAD(P)H: quinone reductase (NQO1) (Favreau and Pickett, 1991), gamma-glutamylcysteine synthetase (GCS) (Wild et al., 1998), and the auxiliary cellular NADPH regenerating enzyme, glucose 6-phosphate dehydrogenase (G6PD) (Thimmulappa et al., 2002). In addition, Sirtuin- and FOXO-induced superoxide dismutase (SOD) (Kops et al., 2002; Nemoto and Finkel, 2002) convert superoxide to hydrogen peroxide (Baehner et al., 1975), which is subsequently detoxified by catalase (CAT) (Essers et al., 2004). These cytoprotective enzymes play a critical role in the scavenging and detoxification of ROS.
Maintaining a balance between ROS and antioxidants is essential for periodontal health. In patients with severe chronic periodontitis, down-regulation of the NRF2 pathway was observed in polymorphonuclear leucocytes (Sima et al., 2016). In addition, neutrophils from patients with severe chronic periodontitis exhibited hyper-reactivity to bacterial stimuli (Dias et al., 2013). Conversely, activation of NRF2 prevented alveolar bone loss in an experimental animal study (Bhattarai et al., 2016). In this context, downregulation of NRF2 pathway might lead to the increase in ROS, which causes tissue destruction.
Cytoprotective enzymes are also thought to interfere with osteoclastogenesis, where ROS functions as an intracellular signaling molecule (Ha et al., 2004). Indeed, RANKL stimulation decreases expression of NRF2-dependent cytoprotective enzymes, thereby facilitating intracellular ROS signaling (Kanzaki et al., 2013, 2016b). Induction of cytoprotective enzymes by NRF2 activation subsequently inhibits osteoclastogenesis (Kanzaki et al., 2013, 2014, 2015; Sakai et al., 2013; Gambari et al., 2014; Lee et al., 2014; Lu et al., 2015; Bhattarai et al., 2016). Inversely, augmented osteoclastogenesis and bone destruction was observed with NRF2 deficiency (Rana et al., 2012; Hyeon et al., 2013; Ibanez et al., 2014; Lippross et al., 2014; Sun et al., 2015). Taken together, this shows that NRF2-dependent cytoprotective enzymes play a critical role in the regulation of bone destruction.
Enlargement of periapical lesions of the teeth in experimental animals has been associated with decreased expression of Sirtuin (SIRT6), causing increased apoptosis of osteoblasts (Kok et al., 2015). Activation of Sirtuin, by resveratrol (Tamaki et al., 2014) or overexpression of SIRT6 in osteoblasts by lentiviral gene transfer (Kok et al., 2015; Hou et al., 2016), inhibited bone destruction. Therefore, not only NRF2- but also Sirtuin-mediated cytoprotective mechanisms control periodontal tissue homeostasis.
Activated FOXOs enhance antioxidant defense by augmenting cytoprotective enzymes, which successfully prevent inflammation and bone destruction (Chung et al., 2011; Kousteni, 2011). FOXO activation directly inhibits osteoclastogenesis (Bartell et al., 2014; Tan et al., 2015). However, FOXO binds to β-catenin, which exhibits inhibitory effects on osteoblastic differentiation. Thus, continuous stimulation of the FOXO/β-catenin pathway may reduce bone formation (Galli et al., 2011). In addition, porphyromonas gingivalis-induced ROS activate FOXO transcription factors through JNK signaling, which resulted in the FOXO1-controlled oxidative stress responses, such as inflammatory cytokine production and cell survival (Wang et al., 2015).
A relationship between cytoprotective enzymes themselves and periodontitis has also been reported. SOD is one of the most extensively investigated enzymes, and is closely associated with periodontitis. A positive relationship was demonstrated between the progression of periodontitis and serum SOD concentrations in experimental animals (Sobaniec and Sobaniec-Lotowska, 2000). This has been confirmed in human gingival crevicular fluid (GCF) (Akalin et al., 2005; Wei et al., 2010), serum (Wei et al., 2010), and saliva SOD (Canakci et al., 2009; Guentsch et al., 2012; Karim et al., 2012). Clinical studies in humans indicate that periodontal therapy returns elevated SOD levels to normal (Novakovic et al., 2013, 2014; Sukhtankar et al., 2013; Singh et al., 2014). Induction of SOD inhibited experimental periodontitis in animals (Petelin et al., 2000) and augmentation of anti-oxidant capacity by nutrient supplementation positively affected periodontal therapy in clinical trials (Biju et al., 2014; Daiya et al., 2014; Muniz et al., 2015). Furthermore, genetic mutation of SOD is considered to be a risk factor for periodontitis (Kazemi et al., 2015). These data support a role for SOD as a potential diagnostic marker for periodontitis. Therapeutically targeting SOD for the treatment of periodontitis may however produce substantive side effects, due to its beneficial effect on periodontopathogenic bacteria. SOD exhibited protective effects on the periodontopathogenic anaerobes Porphyromonas gingivalis (Lynch and Kuramitsu, 1999) and Aggregatibacter actinomycetemcomitans (Balashova et al., 2007).
HO-1 is another cytoprotective enzyme involved in the pathology of periodontitis. HO-1 inhibited RANKL upregulation in human cultured periodontal ligament cells (Lee et al., 2010) and lipopolysaccharide-induced production of proinflammatory mediators in cultured macrophages (Choi et al., 2014). Immunohistochemistry demonstrated that HO-1 was broadly expressed in periodontal tissue with chronic periodontitis (Gayathri et al., 2014). G6PD expression was also increased in the gingiva of patients with gingivitis (Di Paola et al., 2005; Yu et al., 2015). These data support the concept that the protective mechanisms provided by cytoprotective enzymes against ROS also play an inhibitory role in the progression of tissue destruction.
Clinically, it has been reported that markers of oxidative stress in saliva could serve as diagnostic markers for periodontitis (Sawamoto et al., 2005; Almerich-Silla et al., 2015; Banasova et al., 2015; Tothova et al., 2015) and therapeutic intervention against experimental periodontitis in rats improved total serum antioxidant levels (Saglam et al., 2015). A better understanding of the mechanisms that protect against oxidative stress will be useful for gaining a precise understanding of periodontitis pathology, which in turn, will contribute to the development of therapies for periodontitis treatment.
Summary and Perspective
In this review, we have summarized recent evidence of the relationship between oxidative stress and periodontitis, a disease in which oxidative stress is both directly and indirectly involved with tissue destruction. Many reports describe a relationship between oxidative stress and periodontitis, with the balance between oxidative stress and defense mechanisms characterizing the pathological condition of the periodontitis. As such, some enzymes that are cytoprotective against oxidative stress could serve as diagnostic markers for periodontitis. Furthermore, a strong potential therapeutic approach for periodontitis would be to augment enzymes that protect against oxidative stress.
Although oxidative stress in periodontitis has been extensively investigated, there remains little information about the relationship between tissue damage and the immunological reactions exerted via ROS production in immune cells. Further investigation in this area is urgently needed for a comprehensive understanding of periodontitis.
Author Contributions
Conception and design: HK and YN. Search references: SW, TN, YY, YN, YK, KI, SF, and YM. Drafted manuscript: HK, SW, YY, and YN. Critically revised the manuscript: HK, SW, YY, YK, KI, SF, YM, and YN.
Conflict of Interest Statement
The authors declare that the research was conducted in the absence of any commercial or financial relationships that could be construed as a potential conflict of interest.
Acknowledgments
This research was supported by Grants-in-Aid for Scientific Research from the Japan Society for the Promotion of Science (23689081, 25670841, 15K11376, and 16H05552), the Nestle Nutrition Council, Japan, the Translational Research Network Program from the Japanese Ministry of Education, Culture, Sports, Science, and Technology (A-03), the Adaptable and Seamless Technology Transfer Program through Target-driven R&D from the Japan Science and Technology Agency (AS231Z01205G), and the Astellas Foundation for Research on Metabolic Disorders. Finally, the author (HK) gives heartfelt appreciation to the experimental reagent companies and instrument companies for their support in the rehabilitation from the damage caused by the Tohoku earthquake on March 11, 2011.
References
Abo, A., Pick, E., Hall, A., Totty, N., Teahan, C. G., and Segal, A. W. (1991). Activation of the NADPH oxidase involves the small GTP-binding protein p21rac1. Nature 353, 668–670. doi: 10.1038/353668a0
Akalin, F. A., Toklu, E., and Renda, N. (2005). Analysis of superoxide dismutase activity levels in gingiva and gingival crevicular fluid in patients with chronic periodontitis and periodontally healthy controls. J. Clin. Periodontol. 32, 238–243. doi: 10.1111/j.1600-051X.2005.00669.x
Alam, J., Stewart, D., Touchard, C., Boinapally, S., Choi, A. M., and Cook, J. L. (1999). Nrf2, a Cap'n'Collar transcription factor, regulates induction of the heme oxygenase-1 gene. J. Biol. Chem. 274, 26071–26078. doi: 10.1074/jbc.274.37.26071
Almerich-Silla, J. M., Montiel-Company, J. M., Pastor, S., Serrano, F., Puig-Silla, M., and Dasi, F. (2015). Oxidative stress parameters in saliva and its association with periodontal disease and types of bacteria. Dis. Markers 2015:653537. doi: 10.1155/2015/653537
Baehner, R. L., Murrmann, S. K., Davis, J., and Johnston, R. B. Jr. (1975). The role of superoxide anion and hydrogen peroxide in phagocytosis-associated oxidative metabolic reactions. J. Clin. Invest. 56, 571–576. doi: 10.1172/JCI108126
Balashova, N. V., Park, D. H., Patel, J. K., Figurski, D. H., and Kachlany, S. C. (2007). Interaction between leukotoxin and Cu,Zn superoxide dismutase in Aggregatibacter actinomycetemcomitans. Infect. Immun. 75, 4490–4497. doi: 10.1128/IAI.00288-07
Banasova, L., Kamodyova, N., Jansakova, K., Tothova, L., Stanko, P., Turna, J., et al. (2015). Salivary DNA and markers of oxidative stress in patients with chronic periodontitis. Clin. Oral Investig. 19, 201–207. doi: 10.1007/s00784-014-1236-z
Barnes, V. M., Teles, R., Trivedi, H. M., Devizio, W., Xu, T., Mitchell, M. W., et al. (2009). Acceleration of purine degradation by periodontal diseases. J. Dent. Res. 88, 851–855. doi: 10.1177/0022034509341967
Bartell, S. M., Kim, H. N., Ambrogini, E., Han, L., Iyer, S., Serra Ucer, S., et al. (2014). FoxO proteins restrain osteoclastogenesis and bone resorption by attenuating H2O2 accumulation. Nat. Commun. 5:3773. doi: 10.1038/ncomms4773
Bartold, P. M., Marshall, R. I., and Haynes, D. R. (2005). Periodontitis and rheumatoid arthritis: a review. J. Periodontol. 76, 2066–2074. doi: 10.1902/jop.2005.76.11-S.2066
Bax, B. E., Alam, A. S., Banerji, B., Bax, C. M., Bevis, P. J., Stevens, C. R., et al. (1992). Stimulation of osteoclastic bone resorption by hydrogen peroxide. Biochem. Biophys. Res. Commun. 183, 1153–1158. doi: 10.1016/S0006-291X(05)80311-0
Bhattarai, G., Poudel, S. B., Kook, S. H., and Lee, J. C. (2016). Resveratrol prevents alveolar bone loss in an experimental rat model of periodontitis. Acta Biomater. 29, 398–408. doi: 10.1016/j.actbio.2015.10.031
Biju, T., Shabeer, M. M., Amitha, R., Rajendra, B. P., and Suchetha, K. (2014). Comparative evaluation of serum superoxide dismutase and glutathione levels in periodontally diseased patients: an interventional study. Indian J. Dent. Res. 25, 613–616. doi: 10.4103/0970-9290.147105
Callaway, D. A., and Jiang, J. X. (2015). Reactive oxygen species and oxidative stress in osteoclastogenesis, skeletal aging and bone diseases. J. Bone Miner. Metab. 33, 359–370. doi: 10.1007/s00774-015-0656-4
Canakci, C. F., Cicek, Y., and Canakci, V. (2005). Reactive oxygen species and human inflammatory periodontal diseases. Biochemistry (Mosc). 70, 619–628. doi: 10.1007/s10541-005-0161-9
Canakci, C. F., Cicek, Y., Yildirim, A., Sezer, U., and Canakci, V. (2009). Increased levels of 8-hydroxydeoxyguanosine and malondialdehyde and its relationship with antioxidant enzymes in saliva of periodontitis patients. Eur. J. Dent. 3, 100–106.
Chang, M. C., Tsai, Y. L., Chen, Y. W., Chan, C. P., Huang, C. F., Lan, W. C., et al. (2013). Butyrate induces reactive oxygen species production and affects cell cycle progression in human gingival fibroblasts. J. Periodont. Res. 48, 66–73. doi: 10.1111/j.1600-0765.2012.01504.x
Chapple, L. (1997). Reactive oxygen species and antioxidants in inflammatory diseases. J. Clin. Periodontol. 24, 287–296. doi: 10.1111/j.1600-051X.1997.tb00760.x
Chapple, L., Brock, G. R., Milward, M. R., Ling, N., and Matthews, J. B. (2007). Compromised GCF total antioxidant capacity in periodontitis: cause or effect? J. Clin. Periodontol. 34, 103–110. doi: 10.1111/j.1600-051X.2006.01029.x
Chen, Y., Zhang, J., Lin, Y., Lei, Q., Guan, K. L., Zhao, S., et al. (2011). Tumour suppressor SIRT3 deacetylates and activates manganese superoxide dismutase to scavenge ROS. EMBO Rep. 12, 534–541. doi: 10.1038/embor.2011.65
Choi, E. Y., Jin, J. Y., Choi, J. I., Choi, I. S., and Kim, S. J. (2014). DHA suppresses Prevotella intermedia lipopolysaccharide-induced production of proinflammatory mediators in murine macrophages. Br. J. Nutr. 111, 1221–1230. doi: 10.1017/S0007114513003681
Chung, H. Y., Lee, E. K., Choi, Y. J., Kim, J. M., Kim, D. H., Zou, Y., et al. (2011). Molecular inflammation as an underlying mechanism of the aging process and age-related diseases. J. Dent. Res. 90, 830–840. doi: 10.1177/0022034510387794
Dahiya, P., Kamal, R., Gupta, R., Bhardwaj, R., Chaudhary, K., and Kaur, S. (2013). Reactive oxygen species in periodontitis. J. Indian Soc. Periodontol. 17, 411–416. doi: 10.4103/0972-124X.118306
Daiya, S., Sharma, R. K., Tewari, S., Narula, S. C., and Kumar Sehgal, P. (2014). Micronutrients and superoxide dismutase in postmenopausal women with chronic periodontitis: a pilot interventional study. J. Periodontal Implant Sci. 44, 207–213. doi: 10.5051/jpis.2014.44.4.207
Dasgupta, J., Kar, S., Liu, R., Joseph, J., Kalyanaraman, B., Remington, S. J., et al. (2010). Reactive oxygen species control senescence-associated matrix metalloproteinase-1 through c-Jun-N-terminal kinase. J. Cell. Physiol. 225, 52–62. doi: 10.1002/jcp.22193
Dasgupta, J., Kar, S., Van Remmen, H., and Melendez, J. A. (2009). Age-dependent increases in interstitial collagenase and MAP Kinase levels are exacerbated by superoxide dismutase deficiencies. Exp. Gerontol. 44, 503–510. doi: 10.1016/j.exger.2009.04.005
Dias, H., Chapple, I. L., Milward, M., Grant, M. M., Hill, E., Brown, J., et al. (2013). Sulforaphane restores cellular glutathione levels and reduces chronic periodontitis neutrophil hyperactivity in vitro. PLoS ONE 8:e66407. doi: 10.1371/journal.pone.0066407
Di Paola, R., Mazzon, E., Rotondo, F., Dattola, F., Britti, D., De Majo, M., et al. (2005). Reduced development of experimental periodontitis by treatment with M40403, a superoxide dismutase mimetic. Eur. J. Pharmacol. 516, 151–157. doi: 10.1016/j.ejphar.2005.04.039
Droge, W. (2002). Free radicals in the physiological control of cell function. Physiol. Rev. 82, 47–95. doi: 10.1152/physrev.00018.2001
Essers, M. A., Weijzen, S., de Vries-Smits, A. M., Saarloos, I., de Ruiter, N. D., Bos, J. L., et al. (2004). FOXO transcription factor activation by oxidative stress mediated by the small GTPase Ral and JNK. EMBO J. 23, 4802–4812. doi: 10.1038/sj.emboj.7600476
Esterbauer, H., Schaur, R. J., and Zollner, H. (1991). Chemistry and biochemistry of 4-hydroxynonenal, malonaldehyde and related aldehydes. Free Radic. Biol. Med. 11, 81–128. doi: 10.1016/0891-5849(91)90192-6
Favreau, L. V., and Pickett, C. B. (1991). Transcriptional regulation of the rat NAD(P)H:quinone reductase gene. Identification of regulatory elements controlling basal level expression and inducible expression by planar aromatic compounds and phenolic antioxidants. J. Biol. Chem. 266, 4556–4561.
Friguet, B. (2002). Protein repair and degradation during aging. Sci. World J. 2, 248–254. doi: 10.1100/tsw.2002.98
Fuchs, B., and Schiller, J. (2014). Glycosaminoglycan degradation by selected reactive oxygen species. Antioxid. Redox Signal. 21, 1044–1062. doi: 10.1089/ars.2013.5634
Furukawa-Hibi, Y., Kobayashi, Y., Chen, C., and Motoyama, N. (2005). FOXO transcription factors in cell-cycle regulation and the response to oxidative stress. Antioxid. Redox Signal. 7, 752–760. doi: 10.1089/ars.2005.7.752
Galli, C., Passeri, G., and Macaluso, G. M. (2011). FoxOs, Wnts and oxidative stress-induced bone loss: new players in the periodontitis arena? J. Periodont. Res. 46, 397–406. doi: 10.1111/j.1600-0765.2011.01354.x
Gambari, L., Lisignoli, G., Cattini, L., Manferdini, C., Facchini, A., and Grassi, F. (2014). Sodium hydrosulfide inhibits the differentiation of osteoclast progenitor cells via NRF2-dependent mechanism. Pharmacol. Res. 87, 99–112. doi: 10.1016/j.phrs.2014.06.014
Gayathri, G., Muthukumar, S., Joseph, L. D., and Suresh, R. (2014). Immunolocalization of heme oxygenase-1 in periodontal diseases. Indian J. Dent. Res. 25, 567–571. doi: 10.4103/0970-9290.147080
Giannopoulou, C., Krause, K. H., and Muller, F. (2008). The NADPH oxidase NOX2 plays a role in periodontal pathologies. Semin. Immunopathol. 30, 273–278. doi: 10.1007/s00281-008-0128-1
Guentsch, A., Hiese, I., Puklo, M., Kiehntopf, M., Pfister, W., and Eick, S. (2012). Variables of host response in saliva of patients with periodontitis: a pilot study. Quintessence Int. 43, e104–e114.
Ha, H., Kwak, H. B., Lee, S. W., Jin, H. M., Kim, H. M., Kim, H. H., et al. (2004). Reactive oxygen species mediate RANK signaling in osteoclasts. Exp. Cell Res. 301, 119–127. doi: 10.1016/j.yexcr.2004.07.035
Hohn, T., Jung, T., and Grune, T. (2014). Pathophysiological importance of aggregated damaged proteins. Free Radic. Biol. Med. 71, 70–89. doi: 10.1016/j.freeradbiomed.2014.02.028
Hou, K. L., Lin, S. K., Chao, L. H., Hsiang-Hua Lai, E., Chang, C. C., Shun, C. T., et al. (2016). Sirtuin 6 suppresses hypoxia-induced inflammatory response in human osteoblasts via inhibition of reactive oxygen species production and glycolysis-A therapeutic implication in inflammatory bone resorption. Biofactors 43, 170–180. doi: 10.1002/biof.1320
Hsu, C. P., Odewale, I., Alcendor, R. R., and Sadoshima, J. (2008). Sirt1 protects the heart from aging and stress. Biol. Chem. 389, 221–231. doi: 10.1515/BC.2008.032
Hyeon, S., Lee, H., Yang, Y., and Jeong, W. (2013). Nrf2 deficiency induces oxidative stress and promotes RANKL-induced osteoclast differentiation. Free Radic. Biol. Med. 65, 789–799. doi: 10.1016/j.freeradbiomed.2013.08.005
Ibanez, L., Ferrandiz, M. L., Brines, R., Guede, D., Cuadrado, A., and Alcaraz, M. J. (2014). Effects of Nrf2 deficiency on bone microarchitecture in an experimental model of osteoporosis. Oxid. Med. Cell. Longev. 2014:726590. doi: 10.1155/2014/726590
Kanzaki, H., Shinohara, F., Itohiya-Kasuya, K., Ishikawa, M., and Nakamura, Y. (2015). Nrf2 activation attenuates both orthodontic tooth movement and relapse. J. Dent. Res. 94, 787–794. doi: 10.1177/0022034515577814
Kanzaki, H., Shinohara, F., Itohiya, K., Yamaguchi, Y., Katsumata, Y., Matsuzawa, M., et al. (2016b). RANKL induces Bach1 nuclear import and attenuates Nrf2-mediated antioxidant enzymes, thereby augmenting intracellular reactive oxygen species signaling and osteoclastogenesis in mice. FASEB J. 31, 781–792. doi: 10.1096/fj.201600826R
Kanzaki, H., Shinohara, F., Kajiya, M., Fukaya, S., Miyamoto, Y., and Nakamura, Y. (2014). Nuclear nrf2 induction by protein transduction attenuates osteoclastogenesis. Free Radic. Biol. Med. 77, 239–248. doi: 10.1016/j.freeradbiomed.2014.09.006
Kanzaki, H., Shinohara, F., Kajiya, M., and Kodama, T. (2013). The Keap1/Nrf2 protein axis plays a role in osteoclast differentiation by regulating intracellular reactive oxygen species signaling. J. Biol. Chem. 288, 23009–23020. doi: 10.1074/jbc.M113.478545
Kanzaki, H., Shinohara, F., Kanako, I., Yamaguchi, Y., Fukaya, S., Miyamoto, Y., et al. (2016a). Molecular regulatory mechanisms of osteoclastogenesis through cytoprotective enzymes. Redox Biol. 8, 186–191. doi: 10.1016/j.redox.2016.01.006
Kar, S., Subbaram, S., Carrico, P. M., and Melendez, J. A. (2010). Redox-control of matrix metalloproteinase-1: a critical link between free radicals, matrix remodeling and degenerative disease. Respir. Physiol. Neurobiol. 174, 299–306. doi: 10.1016/j.resp.2010.08.019
Karim, S., Pratibha, P. K., Kamath, S., Bhat, G. S., Kamath, U., Dutta, B., et al. (2012). Superoxide dismutase enzyme and thiol antioxidants in gingival crevicular fluid and saliva. Dent. Res. J. (Isfahan). 9, 266–272.
Kawai, T., Matsuyama, T., Hosokawa, Y., Makihira, S., Seki, M., Karimbux, N. Y., et al. (2006). B and T lymphocytes are the primary sources of RANKL in the bone resorptive lesion of periodontal disease. Am. J. Pathol. 169, 987–998. doi: 10.2353/ajpath.2006.060180
Kazemi, E., Moradi, M. T., Yari, K., Mousavi, S. A., and Kahrizi, D. (2015). Association between Manganese Superoxide Dismutase (MnSOD Val-9Ala) genotypes with the risk of generalized aggressive periodontitis disease. Cell. Mol. Biol. (Noisy-le-grand). 61, 49–52.
Kensler, T. W., Wakabayashi, N., and Biswal, S. (2007). Cell survival responses to environmental stresses via the Keap1-Nrf2-ARE pathway. Annu. Rev. Pharmacol. Toxicol. 47, 89–116. doi: 10.1146/annurev.pharmtox.46.120604.141046
Kim, M. S., Yang, Y. M., Son, A., Tian, Y. S., Lee, S. I., Kang, S. W., et al. (2010). RANKL-mediated reactive oxygen species pathway that induces long lasting Ca2+ oscillations essential for osteoclastogenesis. J. Biol. Chem. 285, 6913–6921. doi: 10.1074/jbc.M109.051557
Kok, S. H., Hou, K. L., Hong, C. Y., Chao, L. H., Hsiang-Hua Lai, E., Wang, H. W., et al. (2015). Sirtuin 6 modulates hypoxia-induced apoptosis in osteoblasts via inhibition of glycolysis: implication for pathogenesis of periapical lesions. J. Endod. 41, 1631–1637. doi: 10.1016/j.joen.2015.05.008
Kong, Y. Y., Feige, U., Sarosi, I., Bolon, B., Tafuri, A., Morony, S., et al. (1999). Activated T cells regulate bone loss and joint destruction in adjuvant arthritis through osteoprotegerin ligand. Nature 402, 304–309. doi: 10.1038/46303
Kops, G. J., Dansen, T. B., Polderman, P. E., Saarloos, I., Wirtz, K. W., Coffer, P. J., et al. (2002). Forkhead transcription factor FOXO3a protects quiescent cells from oxidative stress. Nature 419, 316–321. doi: 10.1038/nature01036
Kousteni, S. (2011). FoxOs: unifying links between oxidative stress and skeletal homeostasis. Curr. Osteoporos. Rep. 9, 60–66. doi: 10.1007/s11914-011-0054-3
Lacey, D. L., Timms, E., Tan, H. L., Kelley, M. J., Dunstan, C. R., Burgess, T., et al. (1998). Osteoprotegerin ligand is a cytokine that regulates osteoclast differentiation and activation. Cell 93, 165–176. doi: 10.1016/S0092-8674(00)81569-X
Lee, H. J., Jeong, G. S., Pi, S. H., Lee, S. I., Bae, W. J., Kim, S. J., et al. (2010). Heme oxygenase-1 protects human periodontal ligament cells against substance P-induced RANKL expression. J. Periodont. Res. 45, 367–374. doi: 10.1111/j.1600-0765.2009.01247.x
Lee, N. K., Choi, Y. G., Baik, J. Y., Han, S. Y., Jeong, D. W., Bae, Y. S., et al. (2005). A crucial role for reactive oxygen species in RANKL-induced osteoclast differentiation. Blood 106, 852–859. doi: 10.1182/blood-2004-09-3662
Lee, S. H., Kim, J. K., and Jang, H. D. (2014). Genistein inhibits osteoclastic differentiation of RAW 264.7 cells via regulation of ROS production and scavenging. Int. J. Mol. Sci. 15, 10605–10621. doi: 10.3390/ijms150610605
Lippross, S., Beckmann, R., Streubesand, N., Ayub, F., Tohidnezhad, M., Campbell, G., et al. (2014). Nrf2 deficiency impairs fracture healing in mice. Calcif. Tissue Int. 95, 349–361. doi: 10.1007/s00223-014-9900-5
Liu, J. W., Chandra, D., Rudd, M. D., Butler, A. P., Pallotta, V., Brown, D., et al. (2005). Induction of prosurvival molecules by apoptotic stimuli: involvement of FOXO3a and ROS. Oncogene 24, 2020–2031. doi: 10.1038/sj.onc.1208385
Lu, S. H., Chen, T. H., and Chou, T. C. (2015). Magnolol Inhibits RANKL-induced osteoclast differentiation of raw 264.7 macrophages through heme oxygenase-1-dependent inhibition of NFATc1 expression. J. Nat. Prod. 78, 61–68. doi: 10.1021/np500663y
Lynch, M. C., and Kuramitsu, H. K. (1999). Role of superoxide dismutase activity in the physiology of Porphyromonas gingivalis. Infect. Immun. 67, 3367–3375.
Mates, J. M., and Sanchez-Jimenez, F. (1999). Antioxidant enzymes and their implications in pathophysiologic processes. Front. Biosci. 4:D339–D345. doi: 10.2741/A432
Matthews, J. B., Wright, H. J., Roberts, A., Cooper, P. R., and Chapple, I. L. (2007b). Hyperactivity and reactivity of peripheral blood neutrophils in chronic periodontitis. Clin. Exp. Immunol. 147, 255–264. doi: 10.1111/j.1365-2249.2006.03276.x
Matthews, J. B., Wright, H. J., Roberts, A., Ling-Mountford, N., Cooper, P. R., and Chapple, I. L. (2007a). Neutrophil hyper-responsiveness in periodontitis. J. Dent. Res. 86, 718–722. doi: 10.1177/154405910708600806
Mercado, F. B., Marshall, R. I., and Bartold, P. M. (2003). Inter-relationships between rheumatoid arthritis and periodontal disease. A review. J. Clin. Periodontol. 30, 761–772. doi: 10.1034/j.1600-051X.2003.00371.x
Miricescu, D., Totan, A., Calenic, B., Mocanu, B., Didilescu, A., Mohora, M., et al. (2014). Salivary biomarkers: relationship between oxidative stress and alveolar bone loss in chronic periodontitis. Acta Odontol. Scand. 72, 42–47. doi: 10.3109/00016357.2013.795659
Muniz, F. W., Nogueira, S. B., Mendes, F. L., Rosing, C. K., Moreira, M. M., de Andrade, G. M., et al. (2015). The impact of antioxidant agents complimentary to periodontal therapy on oxidative stress and periodontal outcomes: a systematic review. Arch. Oral Biol. 60, 1203–1214. doi: 10.1016/j.archoralbio.2015.05.007
Nemoto, S., and Finkel, T. (2002). Redox regulation of forkhead proteins through a p66shc-dependent signaling pathway. Science 295, 2450–2452. doi: 10.1126/science.1069004
Novakovic, N., Cakic, S., Todorovic, T., Raicevic, B. A., Dozic, I., Petrovic, V., et al. (2013). Antioxidative status of saliva before and after non-surgical periodontal treatment. Srp. Arh. Celok. Lek. 141, 163–168. doi: 10.2298/SARH1304163N
Novakovic, N., Todorovic, T., Rakic, M., Milinkovic, I., Dozic, I., Jankovic, S., et al. (2014). Salivary antioxidants as periodontal biomarkers in evaluation of tissue status and treatment outcome. J. Periodont. Res. 49, 129–136. doi: 10.1111/jre.12088
Petelin, M., Pavlica, Z., Ivanusa, T., Sentjurc, M., and Skaleric, U. (2000). Local delivery of liposome-encapsulated superoxide dismutase and catalase suppress periodontal inflammation in beagles. J. Clin. Periodontol. 27, 918–925. doi: 10.1034/j.1600-051x.2000.027012918.x
Rana, T., Schultz, M. A., Freeman, M. L., and Biswas, S. (2012). Loss of Nrf2 accelerates ionizing radiation-induced bone loss by upregulating RANKL. Free Radic. Biol. Med. 53, 2298–2307. doi: 10.1016/j.freeradbiomed.2012.10.536
Saglam, M., Koseoglu, S., Hatipoglu, M., Esen, H. H., and Koksal, E. (2015). Effect of sumac extract on serum oxidative status, RANKL/OPG system and alveolar bone loss in experimental periodontitis in rats. J. Appl. Oral Sci. 23, 33–41. doi: 10.1590/1678-775720140288
Sakai, E., Shimada-Sugawara, M., Yamaguchi, Y., Sakamoto, H., Fumimoto, R., Fukuma, Y., et al. (2013). Fisetin inhibits osteoclastogenesis through prevention of RANKL-induced ROS production by Nrf2-mediated up-regulation of phase II antioxidant enzymes. J. Pharmacol. Sci. 121, 288–298. doi: 10.1254/jphs.12243FP
Sasaki, H., Yamamoto, H., Tominaga, K., Masuda, K., Kawai, T., Teshima-Kondo, S., et al. (2009a). NADPH oxidase-derived reactive oxygen species are essential for differentiation of a mouse macrophage cell line (RAW264.7) into osteoclasts. J. Med. Invest. 56, 33–41. doi: 10.2152/jmi.56.33
Sasaki, H., Yamamoto, H., Tominaga, K., Masuda, K., Kawai, T., Teshima-Kondo, S., et al. (2009b). Receptor activator of nuclear factor- κB ligand-induced mouse osteoclast differentiation is associated with switching between NADPH oxidase homologues. Free Radic. Biol. Med. 47, 189–199. doi: 10.1016/j.freeradbiomed.2009.04.025
Sawamoto, Y., Sugano, N., Tanaka, H., and Ito, K. (2005). Detection of periodontopathic bacteria and an oxidative stress marker in saliva from periodontitis patients. Oral Microbiol. Immunol. 20, 216–220. doi: 10.1111/j.1399-302X.2005.00215.x
Schreck, R., Rieber, P., and Baeuerle, P. A. (1991). Reactive oxygen intermediates as apparently widely used messengers in the activation of the NF-kappa B transcription factor and HIV-1. EMBO J. 10, 2247–2258.
Sheikhi, M., Bouhafs, R. K., Hammarstrom, K. J., and Jarstrand, C. (2001). Lipid peroxidation caused by oxygen radicals from Fusobacterium-stimulated neutrophils as a possible model for the emergence of periodontitis. Oral Dis. 7, 41–46.
Sheikhi, M., Gustafsson, A., and Jarstrand, C. (2000). Cytokine, elastase and oxygen radical release by Fusobacterium nucleatum-activated leukocytes: a possible pathogenic factor in periodontitis. J. Clin. Periodontol. 27, 758–762. doi: 10.1034/j.1600-051x.2000.027010758.x
Sima, C., Aboodi, G. M., Lakschevitz, F. S., Sun, C., Goldberg, M. B., and Glogauer, M. (2016). Nuclear factor erythroid 2-related factor 2 down-regulation in oral neutrophils is associated with periodontal oxidative damage and severe chronic periodontitis. Am. J. Pathol. 186, 1417–1426. doi: 10.1016/j.ajpath.2016.01.013
Singh, N., Chander Narula, S., Kumar Sharma, R., Tewari, S., and Kumar Sehgal, P. (2014). Vitamin E supplementation, superoxide dismutase status, and outcome of scaling and root planing in patients with chronic periodontitis: a randomized clinical trial. J Periodontol. 85, 242–249. doi: 10.1902/jop.2013.120727
Sobaniec, H., and Sobaniec-Lotowska, M. E. (2000). Morphological examinations of hard tissues of periodontium and evaluation of selected processes of lipid peroxidation in blood serum of rats in the course of experimental periodontitis. Med. Sci. Monit. 6, 875–881.
Squier, T. C. (2001). Oxidative stress and protein aggregation during biological aging. Exp. Gerontol. 36, 1539–1550. doi: 10.1016/S0531-5565(01)00139-5
Squier, T. C., and Bigelow, D. J. (2000). Protein oxidation and age-dependent alterations in calcium homeostasis. Front. Biosci. 5:D504–D526. doi: 10.2741/A530
Sukhtankar, L., Kulloli, A., Kathariya, R., and Shetty, S. (2013). Effect of non-surgical periodontal therapy on superoxide dismutase levels in gingival tissues of chronic periodontitis patients: a clinical and spectophotometric analysis. Dis. Markers 34, 305–311. doi: 10.1155/2013/269027
Sun, Y. X., Li, L., Corry, K. A., Zhang, P., Yang, Y., Himes, E., et al. (2015). Deletion of Nrf2 reduces skeletal mechanical properties and decreases load-driven bone formation. Bone 74, 1–9. doi: 10.1016/j.bone.2014.12.066
Tamaki, N., Cristina Orihuela-Campos, R., Inagaki, Y., Fukui, M., Nagata, T., and Ito, H. O. (2014). Resveratrol improves oxidative stress and prevents the progression of periodontitis via the activation of the Sirt1/AMPK and the Nrf2/antioxidant defense pathways in a rat periodontitis model. Free Radic. Biol. Med. 75, 222–229. doi: 10.1016/j.freeradbiomed.2014.07.034
Tan, P., Guan, H., Xie, L., Mi, B., Fang, Z., Li, J., et al. (2015). FOXO1 inhibits osteoclastogenesis partially by antagnozing MYC. Sci. Rep. 5:16835. doi: 10.1038/srep16835
Teitelbaum, S. L. (2000). Bone resorption by osteoclasts. Science 289, 1504–1508. doi: 10.1126/science.289.5484.1504
Thannickal, V. J., and Fanburg, B. L. (2000). Reactive oxygen species in cell signaling. Am. J. Physiol. Lung Cell. Mol. Physiol., 279, L1005–L1028.
Thimmulappa, R. K., Mai, K. H., Srisuma, S., Kensler, T. W., Yamamoto, M., and Biswal, S. (2002). Identification of Nrf2-regulated genes induced by the chemopreventive agent sulforaphane by oligonucleotide microarray. Cancer Res. 62, 5196–5203.
Tothova, L., Kamodyova, N., Cervenka, T., and Celec, P. (2015). Salivary markers of oxidative stress in oral diseases. Front. Cell. Infect. Microbiol. 5:73. doi: 10.3389/fcimb.2015.00073
Udagawa, N., Takahashi, N., Akatsu, T., Tanaka, H., Sasaki, T., Nishihara, T., et al. (1990). Origin of osteoclasts: mature monocytes and macrophages are capable of differentiating into osteoclasts under a suitable microenvironment prepared by bone marrow-derived stromal cells. Proc. Natl. Acad. Sci. U.S.A. 87, 7260–7264. doi: 10.1073/pnas.87.18.7260
Waddington, R. J., Moseley, R., and Embery, G. (2000). Reactive oxygen species: a potential role in the pathogenesis of periodontal diseases. Oral Dis. 6, 138–151. doi: 10.1111/j.1601-0825.2000.tb00325.x
Wang, Q., Sztukowska, M., Ojo, A., Scott, D. A., Wang, H., and Lamont, R. J. (2015). FOXO responses to Porphyromonas gingivalis in epithelial cells. Cell. Microbiol. 17, 1605–1617. doi: 10.1111/cmi.12459
Wang, Y., Lebowitz, D., Sun, C., Thang, H., Grynpas, M. D., and Glogauer, M. (2008). Identifying the relative contributions of Rac1 and Rac2 to osteoclastogenesis. J. Bone Miner. Res. 23, 260–270. doi: 10.1359/jbmr.071013
Wei, D., Zhang, X. L., Wang, Y. Z., Yang, C. X., and Chen, G. (2010). Lipid peroxidation levels, total oxidant status and superoxide dismutase in serum, saliva and gingival crevicular fluid in chronic periodontitis patients before and after periodontal therapy. Aust. Dent. J. 55, 70–78. doi: 10.1111/j.1834-7819.2009.01123.x
Wells, P. G., McCallum, G. P., Chen, C. S., Henderson, J. T., Lee, C. J. J., Perstin, J., et al. (2009). Oxidative stress in developmental origins of disease: teratogenesis, neurodevelopmental deficits, and cancer. Toxicol. Sci. 108, 4–18. doi: 10.1093/toxsci/kfn263
White, P. C., Chicca, I. J., Cooper, P. R., Milward, M. R., and Chapple, I. L. (2016). Neutrophil extracellular traps in periodontitis: a web of intrigue. J. Dent. Res. 95, 26–34. doi: 10.1177/0022034515609097
Wild, C., Gipp, J. J., and Mulcahy, T. (1998). Overlapping antioxidant response element and PMA response element sequences mediate basal and β-naphthoflavone-induced expression of the human γ-glutamylcysteine synthetase catalytic subunit gene. Biochem. J. 332(Pt 2), 373–381. doi: 10.1042/bj3320373
Wong, B. R., Josien, R., Lee, S. Y., Vologodskaia, M., Steinman, R. M., and Choi, Y. (1998). The TRAF family of signal transducers mediates NF- κB activation by the TRANCE receptor. J. Biol. Chem. 273, 28355–28359. doi: 10.1074/jbc.273.43.28355
Yasuda, H., Shima, N., Nakagawa, N., Yamaguchi, K., Kinosaki, M., Mochizuki, S., et al. (1998). Osteoclast differentiation factor is a ligand for osteoprotegerin/osteoclastogenesis-inhibitory factor and is identical to TRANCE/RANKL. Proc. Natl. Acad. Sci. U.S.A. 95, 3597–3602. doi: 10.1073/pnas.95.7.3597
Yu, J. Y., Lee, S. Y., Son, Y. O., Shi, X., Park, S. S., and Lee, J. C. (2012). Continuous presence of H(2)O(2) induces mitochondrial-mediated, MAPK- and caspase-independent growth inhibition and cytotoxicity in human gingival fibroblasts. Toxicol. In vitro 26, 561–570. doi: 10.1016/j.tiv.2012.01.022
Keywords: oxidative stress, cytoprotective enzymes, ROS, osteoclast, periodontitis
Citation: Kanzaki H, Wada S, Narimiya T, Yamaguchi Y, Katsumata Y, Itohiya K, Fukaya S, Miyamoto Y and Nakamura Y (2017) Pathways that Regulate ROS Scavenging Enzymes, and Their Role in Defense Against Tissue Destruction in Periodontitis. Front. Physiol. 8:351. doi: 10.3389/fphys.2017.00351
Received: 31 January 2017; Accepted: 15 May 2017;
Published: 30 May 2017.
Edited by:
Alexandrina L. Dumitrescu, Dental Private Practice, RomaniaReviewed by:
Chun-An Chen, Ohio State University Columbus, United StatesNicolás Serafín, Autonomous University of Baja California, Mexico
Copyright © 2017 Kanzaki, Wada, Narimiya, Yamaguchi, Katsumata, Itohiya, Fukaya, Miyamoto and Nakamura. This is an open-access article distributed under the terms of the Creative Commons Attribution License (CC BY). The use, distribution or reproduction in other forums is permitted, provided the original author(s) or licensor are credited and that the original publication in this journal is cited, in accordance with accepted academic practice. No use, distribution or reproduction is permitted which does not comply with these terms.
*Correspondence: Hiroyuki Kanzaki, a2FuemFraS1oQHRzdXJ1bWktdS5hYy5qcA==