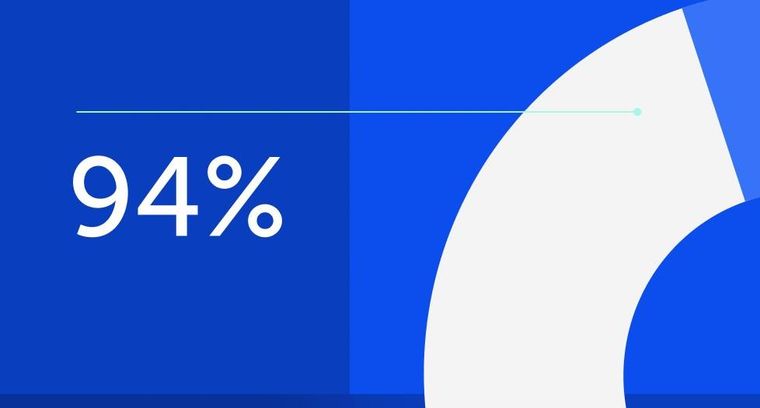
94% of researchers rate our articles as excellent or good
Learn more about the work of our research integrity team to safeguard the quality of each article we publish.
Find out more
ORIGINAL RESEARCH article
Front. Physiol., 23 March 2017
Sec. Exercise Physiology
Volume 8 - 2017 | https://doi.org/10.3389/fphys.2017.00175
This article is part of the Research TopicHypoxia and exercise - novel treatment strategy for obesity and related comorbiditiesView all 8 articles
Previous studies have investigated the effects of high-intensity interval training (HIIT) on cardiorespiratory fitness and body composition in overweight populations. However, the additive effect of HIIT and hypoxia on health parameters is not clear. This study compared the effects of HIIT under hypoxic conditions on cardiometabolic function with that under normoxia in overweight Chinese young women.
Methods: A double-blind randomized controlled experimental design was applied. Twenty-four sedentary overweight Chinese young women (weight: 68.8 ± 7.0 kg, BMI: 25.8 ± 2.3 kg·m−2) participated in the HIIT under either normoxia (NORM, n = 13, PIO2: 150 mmHg, FIO2: 0.21) or normobaric hypoxia (HYP, n = 11, PIO2: 117 mmHg, FIO2: 0.15) for 5 weeks. HIIT was composed of 60 repetitions of 8 s maximal cycling effort interspersed with 12-s recovery per day, for 4 days per week. Cardiorespiratory fitness [peak oxygen uptake (O2peak), and peak oxygen pulse (peak O2 pulse)], serum lipid profile [triglycerides (TG), total cholesterol (TC), high-density lipoprotein cholesterol (HDL-C), and low-density lipoprotein cholesterol (LDL-C)], and body composition (regional and whole-body), were assessed at pre- and post-intervention during the days beyond the self-reported menstrual phase of the participants. Habitual physical activity and diary behavior were maintained during the intervention period.
Results: With similar daily energy intake and physical activity, the increases in O2peak [NORM: 0.26 ± 0.37 L·min−1 (+11.8%) vs. HYP: 0.54 ± 0.34 L·min−1 (+26.1%)] and peak O2 pulse (NORM: +13.4% vs. HYP: +25.9%) for HYP were twice-larger than for NORM (p < 0.05). Although the 5-wk HIIT led to significant improvements in the ratios of TC/HDL-C (p = 0.035) and TG/HDL-C (p = 0.027), no significant group effects were found on the serum variables. Further, no significant changes in body composition or serum fasting leptin were observed in either group.
Conclusion: 5-wk of HIIT improved cardiorespiratory fitness and blood lipids in overweight Chinese young females, while the additive effect of the HIIT under normobaric hypoxia solely enhanced cardiorespiratory fitness, but not body composition or serum lipid profile.
Physical activity has been regarded as the most important prevention against a variety of chronic health disorders including poor cardiorespiratory fitness, coronary heart disease, metabolic syndrome and obesity (Booth et al., 2012). Although an increase in physical activity and decrease in sedentary behaviors are recommended in the majority of individuals, physical activity has been shown to undergo a dramatic decline during the transition from late adolescence to early adulthood (Anderson et al., 2011; Kwan et al., 2012).
“Lack of time” is often cited as a key barrier to regular exercise participation (Trost et al., 2002). High-intensity interval training (HIIT), characterized as achieving over 80% of maximal heart rate (HRmax) (Weston et al., 2014), has been shown to be a time-efficient paradigm to induce at least similar physiological adaptations associated with continuous moderate-intensity training (Gibala et al., 2006, 2014). It has been shown that 2–6 weeks of HIIT consisting of four to six 30-s “all-out” Wingate cycling tests separated by 4–4.5 min of recovery could improve cardiorespiratory fitness and associated metabolic function and body composition in athletes and sedentary individuals, as well as in patients with chronic diseases (Gibala et al., 2006; Rakobowchuk et al., 2008; Richards et al., 2010; Astorino et al., 2011; Gist et al., 2014; Hazell et al., 2014). However, the feasibility of cycling HIIT exercise is questionable, as such a strenuous protocol is hard to adhere to among sedentary individuals (Boutcher, 2011). This is partly due to the elicitation of antagonistic emotions during the repetition of “all-out” efforts (Saanijoki et al., 2015). As the training adaptations resulting from the low-volume HIIT effort are possibly exercise-mode specific (Rampinini et al., 2016), Trapp et al. (2008) have introduced an alternative cycling HIIT protocol which is rather brief, composed of 60 repetitions of 8-s of cycling effort interspersed with 12-s of recovery, and found that young sedentary women who participated in the specific HIIT for 15 weeks could markedly improve cardiorespiratory fitness, body composition and insulin resistance.
There is a general consensus that hypoxic training in athletes is an effective ergogenic aid to enhance the functional capacity of the cardiorespiratory system and associated endurance performance, and the intervention has been used for more than a half century. The ergogenic effect of hypoxic training was partly attributed to the improved blood perfusion level and resultant enhanced oxygen utilization in skeletal muscles (Faiss et al., 2013a). The underlying mechanisms for such beneficial adaptations may be associated to the compensatory vasodilation to match an increased oxygen demand at the muscular level; and the hypoxic training-induced changes in capillary-to-fiber ratio, fiber cross-sectional area, myoglobin content and oxidative enzyme activity in skeletal muscles which arise through the oxygen-sensing pathway (Vogt et al., 2001; Casey and Joyner, 2012; Faiss et al., 2013b). Moreover, hypoxic exercise augments sympathetic activation (Lippl et al., 2010) and the release of stress hormones (Shukla et al., 2005; Kayser and Verges, 2013), leading to an increase in energy expenditure (Workman and Basset, 2012) and appetite suppression (Kayser and Verges, 2013), and associated alteration of lipid metabolism (Haufe et al., 2008). Such specific physiological adaptations to hypoxic training in humans appear to be in favor of optimizing cardiometabolic function as well as body composition in sedentary individuals in comparison to those resulting from normoxic training. In our previous study, we demonstrated additive improvements in weight loss and hemodynamics following 4 weeks of intermittent hypoxic training in young obese individuals in comparison to their normoxic counterparts when the hypoxic level, training regimen, habitual dietary intake and physical activity were well controlled in a residential training camp (Kong et al., 2014). Our findings support the previous notion that hypoxia training may be an efficient strategy to tackle the health crisis of obesity and associated comorbidities (Urdampilleta et al., 2012; Kayser and Verges, 2013; Millet et al., 2016). However, the previous findings of intermittent hypoxic training-induced alterations in cardiorespiratory fitness and body composition, in spite of equivocal, were mainly resulted from moderate continuous training (Gatterer et al., 2015; Gonzalez-Muniesa et al., 2015). There is dearth of research investigating the effect of short-term, low-volume HIIT performed in hypoxia on cardiorespiratory and metabolic function in a sedentary population.
The present study was conducted, using a double-blind placebo-controlled design, to investigate whether a 5-wk HIIT regimen (60 repetitions of 8-s cycling effort interspersed with 12-s recovery, 4 sessions per week) carried out in normobaric hypoxia (HYP, FIO2 = 0.15) would improve cardiometabolic function in overweight young women in comparison to similar training in normoxia (NORM, FIO2 = 0.21).
This study was conducted in accordance with the declaration of Helsinki, and approved by the Ethical Committee of the University of Macau for the Use of Human and Animal Subjects in Research. Twenty-nine sedentary, overweight, but otherwise healthy Chinese young women were recruited based on the overweight criteria in the Asian-Pacific region recommended by the World Health Organization (WHO, 2000). The inclusion criteria were as follows: age between 18 and 30 years; body mass index (BMI) ≥ 23.0 kg·m−2; body fat (%BF) ≥ 30%; body weight that remained relatively constant (± 2 kg) in the past 3 months; residence at altitudes below 1,000 m; no prior experience of hypoxic training; no previous engagement in any structured exercise; non-smokers; not taking oral contraceptives or any medication known to influence body weight during the past 6 months. Following an explanation of the purpose and constraints of the study, all participants gave their written consent to participate.
Figure 1 shows the timeline of pre- and post-intervention measurements, as well as the 5-week HIIT intervention. Baseline measurements of body composition, cardiorespiratory fitness and fasting blood lipids were carried out 3–5 days before the intervention. Thereafter, participants with matching %BF and cardiorespiratory fitness were randomly assigned to either the NORM (n = 15) or the HYP (n = 14) HIIT group. The HIIT sessions were conducted in the 100 m2 hypoxic laboratory (Low Oxygen Systems GmbH, Germany) of the Sports Science Research Centre at Beijing Sport University. During the intervention, HIIT was performed at sea level (PIO2: 150 mmHg, FIO2: 0.21) for the NORM group or a simulated altitude of 2,500 m (PIO2: 117 mmHg, FIO2: 0.15) for the HYP group. The environment of the chamber remained constant during the intervention period with temperature of 22.2 ± 1.5°C and relative humidity of 21.9 ± 5.8%. In order to reduce the potential psychological influence, all participants and investigators were blinded to the group assignment and were not informed of the oxygen concentration in the chamber during the sessions. After intervention, measurements of body composition, cardiorespiratory fitness and fasting blood lipids, which were conducted in an identical manner to the baseline measurements, were carried out within 3–5 days following the last training session. All the pre- and post-intervention measurements were carried out during the days beyond the self-reported menstrual phase of the participants.
Figure 1. The timeline of pre-and post-intervention measurements, as well as the 5-wk intervention of high-intensity interval training (HIIT).
During the experimental period, all subjects were asked to maintain their daily activity and avoid altering their eating habits. During all testing and training sessions, a physician attended in case of emergency needs. At the end of the experiment, the awareness of group assignment was assessed by asking “Which condition do you think you trained in during the intervention?”
Accordingly to a previous study (Trapp et al., 2008), the HIIT protocol consisted of 60 repetitions of 8-s maximum effort on a Wingate testing bike ergometer (Monark 894 E, Sweden) interspersed with 12-s recovery with pedal frequency maintained between 20 and 30 rpm, 4 days per week, for 5 weeks. During a familiarization session prior to intervention, participants were requested to perform at least 10 8-s maximum efforts of the HIIT exercise, in order to ensure full understanding of the maximal involvement required during the intervention. The initial training workload was set at 1 kp for all participants. Once a participant was able to complete the 20-min full training protocol consistently at a given resistance, or demonstrated a significant decrease (≥ 5 bpm) in exercise heart rate (HR) in two consecutive sessions, the resistance was increased by 0.5 kp. During the HIIT, warm-up and cool-down exercises were standardized and identical in both groups. All participants exercised with carefully supervision. Polar HR monitor (Polar RS 800, Finland) and finger pulse oximeter (Nonin Onyx II Model 9560, USA) were used to monitor HR and peripheral oxygen saturation (SpO2), respectively, before, during and after each HIIT session. All the exercise data were concealed from participants until the completion of all experimental trials. Training load was reported via absolute and relative mean power output (MPO), percentage of HRmax (%HRmax), and training impulse (TRIMP) as previously described (Banister, 1991).
Physical activity and dietary behavior outside the study were controlled and documented. Normal dietary and lifestyle habits were maintained as consistent as possible throughout the study. To record habitual activity level apart from the HIIT, each participant wore a pedometer (Ymax SW-200 digiwalker, Japan) 3 days (2 weekdays and 1 weekend day) per week during the week prior to and after the intervention, as well as during the 5-week HIIT intervention.
The diet of each participant was recorded on 3 days per week (2 weekdays and 1 weekend day) during the week prior to and after the intervention, as well as during the 5-week HIIT intervention, according to the guidelines of the Sports Nutrition Centre of the National Research Institute of Sports Medicine (NRISM) in China. The dietary records and corresponding energy intake were analyzed using the NRISM dietary and nutritional analysis system (version 3.1), designed for Chinese athletes and the general population. All participants were recommended to maintain a dietary macronutrient distribution of 60% carbohydrates, 35% fat and 15% protein.
Participants performed a graded cycling test on the same bike ergometer (Monark 894 E, Sweden) 3 to 4 days pre- and post-intervention for measuring O2peak via a breath-by-breath modular metabolic system (Max-II, AEI Technologies, PA, USA). Following a 3-min warm up, the graded cycling test began at 50 W and the power output increased by 25 W every 3 min until exhaustion. The plateau of oxygen uptake was used as the criterion for O2peak despite increased workload, along with a respiratory exchange ratio of ≥1.1. The average of the highest O2 values for 15 consecutive seconds during the last stage was recorded as O2peak. HR was measured with Polar HR monitor (Polar F4M BLK, Finland) and the HRmax was defined as the highest value attained during the test. The peak O2 pulse was calculated as the O2peak divided by the maximal HR and expressed as ml·beat−1.
Participants reported to the laboratory in the morning (7:00 a.m.) after a minimum 12 h overnight fast. Subjects were asked to refrain from physical exercise and alcohol ingestion for at least 48 h before each session. Following 30 min of rest on a chair, 8 mL venous blood from the antecubital fossa was collected. After static clotting and centrifugation, serum samples were removed and stored at −80°C until later analysis.
Serum lipids, including high-density lipoprotein cholesterol (HDL-C), low-density lipoprotein cholesterol (LDL-C), total cholesterol (TC) and total triglycerides (TG), were measured using an automatic biochemical analyzer (Olympus AU400, Japan). The intra-assay coefficients of variation (CV) for blood lipid assays were all within 5.0% (Deyi Biomedical Technology Co., Ltd., Beijing, China). Serum leptin was measured by a commercial enzyme-linked immunosorbent assay kit (Abcam, Cambridge, UK). The lower detection limits and intra-assay CVs were 5.3 pg·ml−1 and 2.4% for leptin, respectively (KingMed Center for Clinical Laboratory Co. Ltd, Guangzhou, China).
Height was determined using standard methods with a stadiometer to the nearest 0.1 cm. Whole-body and regional fat mass and lean mass measurements were carried out 30 min following the venous blood collection. Subjects were scanned in a supine position by a dual-energy X-ray absorptiometry scanner (Norland XR-36 DXA densitometer, Norland Corporation, Fort Atkinson, WS, USA) and images analyzed using dedicated software (3.7.4/2.1.0; Norland Corporation). Lean mass and fat mass were calculated from the total and regional analysis of the whole body scan. The instrument was calibrated daily with the phantoms provided by the manufacturer.
Statistical analysis was carried out using SPSS software (Version 20.0, IBM, New York, USA). All variables were checked by parametric statistics regarding conformation to a normal distribution by the Kolmogorov-Smirnov test. Independent sample t-tests were performed to determine the differences in training parameters and SpO2 between the two groups. A two-way repeated measures analysis of variance (group × time) was used to determine differences in pre- and post-training variables. Significant effects were subsequently analyzed using the Tukey post hoc test. As effect size measures of the main effect and the interaction effect, partial η2 was considered small if η2 < 0.01 and large if η2 > 0.14. Pearson product-moment correlation coefficients were computed to examine the relationships between variables. The sample size of subjects estimated for the power of 0.8 to detect a significant increase after HIIT was 8–12 for the primary outcome of O2peak. All tests for statistical significance were standardized at an alpha level of p ≤ 0.05, and all results were expressed as mean ± standard deviation.
During the intervention, two participants in NORM and three in HYP withdrew from the HIIT intervention for personal reasons that were not exercise-related. Accordingly, the data from 13 participants in the NORM group, and 11 subjects in the HYP group were valid for subsequent analyses. Among the participants who completed the study, compliance with the exercise intervention was 100% in the NORM and HYP groups. Eleven (46%) of the 24 participants provided an incorrect answer about the group assignment during the intervention period suggesting that the participants were blinded to the inhaled oxygen concentration during their exercise. No adverse events were reported during testing or training in either group.
For the SpO2 of NORM and HYP groups, the main effects of time (p < 0.001, partial η2 = 0.849), group (p < 0.001, partial η2 = 0.823) and interaction (p < 0.001, partial η2 = 0.780) effects were significant. Throughout the 5-week HIIT intervention, SpO2 remained constant in the NORM group, while it increased progressively in the HYP group in the first 2 weeks, and plateaued in the subsequent weeks. The SpO2 of the NORM group in each week was significantly higher than the corresponding value of the HYP group (p < 0.05) (Figure 2).
Figure 2. The changes in peripheral oxygen saturation (SpO2) of participants during the 5-wk HIIT under normoxia (NORM) and normobaric hypoxia (HYP) are shown [partial η2 = 0.849 (time); 0.823 (group); 0.780 (time × group)]. †Significantly different from the corresponding values of the NORM group (p < 0.05). aSignificantly different from the wk1 value of the HYP group (p < 0.05); bSignificantly different from the wk 2 value of the HYP group (p < 0.05); HIIT, high-intensity interval training.
Table 1 shows the HIIT details of NORM and HYP groups in each week during the intervention. During the 5-wk HIIT, the average exercise duration reduced progressively (p < 0.05), and the difference in the reduction of the exercise duration between the NORM and HYP groups was not significant (p > 0.05). In contrast, the average workload increased progressively across the 5-wk HIIT (p < 0.05) without differing between groups (p > 0.05). Likewise, the MPO of NORM and HYP groups were increased in similar magnitude, except during the 5th week, in which the MPO of NORM was slightly higher than that of HYP group (p < 0.05). For the TRIMP and % HRmax, slightly reductions were observed across the 5-wk HIIT in both groups (p < 0.05), while the changes in the two variables of the HIIT were not significant (p > 0.05).
Table 1. Training parameters of participants of the 5-wk high-intensity interval training regimen under normoxia (NORM) and normobaric hypoxia (HYP).
The habitual energy intake and activity level of the participants during the week prior to and after the intervention, as well as during the 5-week HIIT intervention, are depicted in Table 2. No significant difference was noted within and between groups before, during and after intervention for habitual energy intake (p > 0.05); similar results were also found in the estimated habitual physical activity level (p > 0.05).
Table 2. Habitual energy intake and physical activity level during the 1-week prior to the intervention (Pre), the 5 weeks of the intervention, and the 1 week after the intervention (Post) in NORM and HYP groups.
Table 3 shows the variables of cardiorespiratory fitness, body composition and serum lipid profile pre- and post-intervention. The significant main effect of time in absolute and relative O2peak and peak O2 pulse (p < 0.001) suggested that there were significant increases in these three variables in both NORM and HYP groups. The significant interaction effect (p ≤ 0.05) further revealed that the increases in these three variables in the NORM group were significantly lesser in comparison to corresponding variables in the HYP group (Figure 3). Furthermore, the increase in time for which the participants sustained the exercise at the intensity corresponding to ≥ 90% of their HRmax between the last and first training session (NORM: 4.4 ± 3.6 min vs. 7.2 ± 8.3 min, HYP: 4.1 ± 3.4 min vs. 7.9 ± 8.8 min, p < 0.05) was positively correlated to the increase in absolute O2peak (r = 0.55, n = 24, p < 0.01).
Table 3. Cardiorespiratory fitness, body composition and serum lipid profile prior to (Pre) and after the intervention (Post) in NORM and HYP groups.
Figure 3. The changes in (A) O2peak[partial η2 = 0.626 (time); 0.144 (group); 0.177 (time × group)] and (B) Peak O2 pulse [partial η2 = 0.736 (time); 0.229 (group); 0.216 (time × group)] in individual participants (solid lines) and groups (bars) after 5-wk HIIT under normoxia (NORM, n = 13) and normobaric hypoxia (HYP, n = 11). †Significantly different from corresponding NORM value (p < 0.05); HIIT: high-intensity interval training.
Following the 5-wk HIIT intervention, there were no significant changes in whole-body and regional body composition in either NORM or HYP groups (p > 0.05). For the serum lipid profile, there were no significant changes in TG, TC, HDL-C, and LDL-C between pre- and post-intervention in both groups, while significant improvements in TC/HDL-C and TG/HDL-C were observed (p < 0.05) over time. Serum leptin was also unchanged between pre- and post-intervention in either group (p > 0.05).
To the best of our knowledge, this is the first study to investigate the effects of short-term hypoxic HIIT on cardiometabolic health in overweight Chinese sedentary young women. The main findings of this study were that 20 brief HIIT sessions over 5 weeks improved the cardiorespiratory fitness and blood lipids in both NORM and HYP groups. Moreover, the 5-wk HIIT carried out in HYP could boost further the training effect on the cardiorespiratory fitness, but not on the whole-body and regional body composition or serum lipid profile of the participants.
O2peak and peak O2 pulse are the variables frequently used in assessment of an individual's cardiorespiratory fitness in both research and clinical settings (Oliveira et al., 2009). In the present study, we found that the hypoxic HIIT led to a 2-fold increase in absolute O2peak in comparison to that resulting from an identical normoxic training. Similar results were also found in peak O2 pulse (NORM: 13.4%; HYP: 25.9%). The current findings are in line with the results of previous studies where 3 to 4 weeks of hypoxic HIIT led to a further enhancement in aerobic capacity in athletes (Dufour et al., 2006; Czuba et al., 2011, 2013) as well in healthy young males (Bailey et al., 2000; Dufour et al., 2006). During the 5-wk hypoxic HIIT, despite the progressive rise in SpO2 of the HYP participants from the initial stage, possibly due to improved ventilation-perfusion inequality of the lung (Stoneham and Pethybridge, 1993), they were still suffering from hypoxia during the exercise. Nevertheless, the overall training impulse of the NORM group during the HIIT did not differ from that of the HYP group. Moreover, the duration of exercise at the intensity corresponding to ≥ 90% of HRmax tended to be longer in HYP group. The increase in duration of exercise at the aforementioned intensity from the initial to the final session of the HIIT accounted for 30.1% of the changes in absolute O2peak. This scenario suggests that the hypoxic HIIT might have overloaded the cardiovascular system additionally, and thus potentiated greater adaptation. It further provides supportive evidence that the short-term HIIT in a hypoxic environment is feasible in overweight and obese individuals to enhance aerobic fitness, and would result in an additive effect on cardioprotection when compared with the same training in normoxia.
It has been demonstrated that the activation of hematological adaptive mechanisms with intermittent hypoxic training requires the provision of sufficient training stimulus with the duration of hypoxic exercise last consecutively for 90 min for a single session (Rodriguez et al., 2000). In the present study, the additive increase in O2peak resulting from the brief hypoxic HIIT regimen (≤ 20 min) is possibly associated with non-hematological adaptive mechanisms occurring in muscular and systemic portions. In fact, certain physiological adaptations in muscle tissues in response to hypoxic training, including increases in skeletal muscle mitochondrial density, capillary-to-fiber ratio, and fiber cross-sectional area, have been demonstrated to be larger than those resulting from traditional normoxic training in untrained individuals (Vogt et al., 2001; Zoll et al., 2006; Faiss et al., 2013b). Such discrepancy in training adaptations in muscles might be associated with an increase in hypoxia inducible factor-1α, which has been shown to be essential in regulating the cardiovascular and respiratory responses to hypoxia (Semenza, 2004). In addition, the additive increase in O2peak in the HYP group is concomitant with a greater peak O2 pulse. The specific adaptations in the cardiovascular system to hypoxic training, including increased stroke volume, might lead to more effective systemic circulation for optimizing muscle oxygenation (Czuba et al., 2011).
It is known that exposure to hypoxia could facilitate lipid metabolism through the transcriptional coactivator peroxisome proliferator-activated receptor-1, which is triggered by the hypoxia inducible factor-1 (Zoll et al., 2006; Millet et al., 2010). Modifications of blood lipid profile with short-term hypoxic training have been demonstrated in untrained individuals. Haufe et al. (2008) reported that 4-wk hypoxic training in untrained healthy men reduced blood TG to a greater extent in comparison to training in normoxia. Such beneficial effects of hypoxic training on blood lipid profile were also observed in healthy normal-weight males following a 10-day intervention of normobaric hypoxic (FIO2 = 14%) confinement (Debevec et al., 2014). However, the 5-wk hypoxic HIIT in the present study did not lead to significant additive improvements in serum lipids in the HYP group. The current findings were consistent with those found previously in overweight or obese subjects following a 4-wk moderate-intensity treadmill training regimen under hypoxia (Wiesner et al., 2010). The comparable post-intervention profile of serum lipids between NORM and HYP groups was in accordance with the previous notion that short-term moderate hypoxic training does not cause significant additive lipolytic effect to that invoked by normoxic training, specifically, when the body mass of participants remains constant and their diet and physical activity levels are controlled (Bailey et al., 2000). Differences in training mode and regimens (low-to-moderate aerobic training vs. HIIT), the body fat status of participants (normal vs. overweight vs. obese), and their dietary control may definitely cause contradictory findings.
Improved body composition has been reported during or after acute high altitude exposure (Lippl et al., 2010). Combining hypoxic exposure with exercise training has also been reported to potentiate weight loss in overweight and obese individuals (Netzer et al., 2008; Wiesner et al., 2010; Kong et al., 2014). For instance, low-intensity exercise performed three times per week for 90 min with normobaric hypoxia (FIO2 = 15%) for 8 weeks could lead to greater body weight reduction in obese individuals than resulted from training under sham hypoxia (Netzer et al., 2008). Similar results were also noted from a relatively brief training regimen which was composed of three sessions of 60 min hypoxic endurance exercises per week for 4 weeks in overweight/obese subjects (Wiesner et al., 2010). However, greater improvement was not observed in body composition and fasting serum leptin level in our HYP participants participating in the 5-wk hypoxic HIIT compared with the NORM group. The current training outcomes, in contrast to the previous findings, might have been derived from differences in training mode and intensities which have been shown to play essential roles in regulating free-fatty acid mobilization (Romijn et al., 1993). It is well known that blood flow increases in subcutaneous adipose tissue in response to low-intensity exercise, and plateaus when exercise intensity is further increased (Heinonen et al., 2012). This plateau in adipose tissue blood flow during high-intensity exercise is considered an important mechanism for decreasing free fatty acid mobilization during the exercise (Romijn et al., 1993), and alternatively, increasing the contribution of aerobic/anaerobic glycolysis to ATP generation. Recently, it was found that adipose tissue blood flow is further reduced during exercise with hypoxic breathing, possibly due to hypoxia-triggered enhanced sympathetic vasoconstriction in adipose tissue vasculature (Heinonen et al., 2014). Although the present study did not examine fat metabolism during the 5-wk HIIT, it is reasonable to postulate that a marked increase in fat metabolism in the HYP group in comparison to that of NORM group is unlikely to occur during the intervention period. In fact, the beneficial effects of hypoxic training on body composition in most previous studies were with low-to-moderate intensity continuous exercise (Haufe et al., 2008; Netzer et al., 2008; Wiesner et al., 2010; Kong et al., 2014). Similar findings regarding body fat control with HIIT under hypoxia either in healthy or obese individuals are limited. In addition, increased serum levels of the satiety-signaling hormone leptin and the resultant anorexia response are generally associated with altitude sojourn or hypoxic training (Shukla et al., 2005). Nevertheless, the unchanged serum leptin found in the HYP group following the 5-wk hypoxic HIIT is in line with previous findings in short-term hypoxia exposure (Debevec et al., 2014) or hypoxic training (Haufe et al., 2008) in healthy individuals, and support the notion that hypoxia does not necessarily always affect one's serum leptin and associated appetite regulation (Debevec et al., 2014). Apart from potential intensity difference which may partly explain the contrary findings in body fat reduction, participants' initial adiposity level may also impact changes in body fat resulting from an exercise intervention (Forbes, 2000). It is known that participants with a higher BMI appear to have more fat loss after an intervention compared with those possessing a lower BMI (Hansen et al., 2001). In the present study, the initial BMI (~25 kg·m−2) of the Chinese female participants appeared to be lower than that (~30 kg·m−2) reported in previous studies (Haufe et al., 2008; Netzer et al., 2008; Wiesner et al., 2010; Kong et al., 2014). It is therefore conceivable to consider the relative low initial BMI as an apparent disadvantage in revealing the impact of the 5-wk HIIT on body fat loss.
In the present study, there are several caveats which deserve discussion. First, the habitual calorie intake and energy expenditure of participants in this study were estimated based on the self-reported dietary intake, and the data of activity monitoring, respectively, recorded 3 days per week. Moreover, the lead-in period for the dietary and physical activity assessments was only 1 week in advance of the intervention. Although our findings provide reasonable information regarding the daily energy intake and output during the intervention, further interpretation of the current findings is therefore limited by the potential estimation errors. In avoidance of the potential errors, participants accommodating in a hypoxic training camp with strict control in nutritional intake and physical activity is recommended. Such a maneuver had been successfully implemented in our previous study (Kong et al., 2014). Secondly, a gradual increase in SpO2, possibly due to ventilation-perfusion adaptation in the lungs (Stoneham and Pethybridge, 1993), demonstrated the progressive attenuation of hypoxic stress in participants during the intervention period. It is reasonable to hypothesize the further improvement would be observed in the resultant aerobic capacity, as well as the body composition and serum lipid profile in HYP participants if the initial SpO2 and associated hypoxic stress could be maintained throughout the intervention period. These points await further investigation. Moreover, the improvements in the cardiorespiratory fitness and serum lipids resulting from HIIT under mild hypoxia could possibly be augmented by increasing the severity of hypoxia. However, more severe hypoxia might not be well tolerated by all individuals. It might concomitantly induce physiological detrimental effects on bodily function including inflammatory responses in adipose tissue of obese persons (Heinonen et al., 2016). A balance of these factors appears to be the prerequisite for achieving potential health benefits of hypoxia on humans. Lastly, as Chinese population generally possess a lower level of adiposity than their European counterpart (Lesser et al., 2013), such ethnical difference in body fat accumulation and distribution would lead to inconsistent hypoxic HIIT effects on body composition.
Using a randomized, double-blind, placebo-controlled design in overweight Chinese young women, the present study demonstrates that the specific 5-wk HIIT regimen could improve cardiorespiratory fitness and blood lipids in the participants in both NORM and HYP groups. Nevertheless, the additive effect of the HIIT under mild hypoxia (FIO2 = 0.15) only appears to enhance cardiorespiratory fitness, but not the whole-body and regional body composition, or serum lipid profile.
The ethical group in the University of Macau. Overweight healthy young females were recruited to participate in the study through local advertisements. After the initial screening, the qualified participants were informed verbally and in writing of the experimental procedures and associated risks in detail, all participants gave their written consent to participate in the study. During the intervention, all participants can withdraw from the study at any time without any reasons. In order to avoid any unexpected risks, a physician was in attendance during all tests and training sessions associated with the study.
The contributions of the authors were as follows: research design: ZK, QS, JN, TT, and YH; data collection: LY, LS, and YH; data analysis and interpretion: ZK, QS, JN, and TT; manuscript drafting: ZK, QS, and LS; manuscript revision: JN, TT, LY, and YH.
This study was supported by a research grant received by ZK from the University of Macau (MYRG027(Y1-L1)FED11-KZW), which made no contribution toward research design, data collection or decision to publish.
The authors declare that the research was conducted in the absence of any commercial or financial relationships that could be construed as a potential conflict of interest.
We would like to thank Dr. Wang Xintang for her assistance in data collection. Also, we are very appreciated Dr. Robert Chapman's kind comments on our final manuscript. At last, but not the least, we are very grateful for the patience and cooperations of all participants.
BF, body fat; BMI, body mass index; HDL-C, high-density lipoprotein cholesterol; FM, fat mass; HIIT, high-intensity interval training; HR, heart rate; HYP, normobaric hypoxia; LDL-C, low-density lipoprotein cholesterol; LM, lean mass; NORM, normoxia; SpO2, peripheral oxygen saturation; TC, total cholesterol; TG, triglycides; TC/HDL-C, ratio of TC and HDL-C; TG/HDL-C, ratio of TG and HDL-C; O2, oxygen consumption; O2peak: peak oxygen uptake.
Anderson, G. J., Cipolla, C., and Kennedy, R. T. (2011). Western blotting using capillary electrophoresis. Anal. Chem. 83, 1350–1355. doi: 10.1021/ac102671n
Astorino, T. A., Allen, R. P., Roberson, D. W., Jurancich, M., Lewis, R., McCarthy, K., et al. (2011). Adaptations to high-intensity training are independent of gender. Eur. J. Appl. Physiol. 111, 1279–1286. doi: 10.1007/s00421-010-1741-y
Bailey, D. M., Davies, B., and Baker, J. (2000). Training in hypoxia: modulation of metabolic and cardiovascular risk factors in men. Med. Sci. Sports Exerc. 32, 1058–1066. doi: 10.1097/00005768-200006000-00004
Banister, E. W. (1991). “Modeling elite athletic performance,” in Physiological Testing of the High-Performance Athlete, 2nd Edn., eds J. D. MacDougall, H. L. Wenger, and H. J. Green (Champaign, IL: Human Kinetics), 403–425.
Booth, F. W., Roberts, C. K., and Laye, M. J. (2012). Lack of exercise is a major cause of chronic diseases. Compr. Physiol. 2, 1143–1211. doi: 10.1002/cphy.c110025
Boutcher, S. H. (2011). High-intensity intermittent exercise and fat loss. J. Obes. 2011:868305. doi: 10.1155/2011/868305
Casey, D. P., and Joyner, M. J. (2012). Compensatory vasodilatation during hypoxic exercise: mechanisms responsible for matching oxygen supply to demand. J. Physiol. 590, 6321–6326. doi: 10.1113/jphysiol.2012.242396
Czuba, M., Waskiewicz, Z., Zajac, A., Poprzecki, S., Cholewa, J., and Roczniok, R. (2011). The effects of intermittent hypoxic training on aerobic capacity and endurance performance in cyclists. J. Sports Sci. Med. 10, 175–183.
Czuba, M., Zajac, A., Maszczyk, A., Roczniok, R., Poprzecki, S., Garbaciak, W., et al. (2013). The effects of high intensity interval training in normobaric hypoxia on aerobic capacity in basketball players. J. Hum. Kinet. 39, 103–114. doi: 10.2478/hukin-2013-0073
Debevec, T., Simpson, E. J., Macdonald, I. A., Eiken, O., and Mekjavic, I. B. (2014). Exercise training during normobaric hypoxic confinement does not alter hormonal appetite regulation. PLoS ONE 9:e98874. doi: 10.1371/journal.pone.0098874
Dufour, S. P., Ponsot, E., Zoll, J., Doutreleau, S., Lonsdorfer-Wolf, E., Geny, B., et al. (2006). Exercise training in normobaric hypoxia in endurance runners. I. Improvement in aerobic performance capacity. J. Appl. Physiol. 100, 1238–1248. doi: 10.1152/japplphysiol.00742.2005
Faiss, R., Girard, O., and Millet, G. P. (2013a). Advancing hypoxic training in team sports: from intermittent hypoxic training to repeated sprint training in hypoxia. Br. J. Sports Med. 47(Suppl. 1), i45–i50. doi: 10.1136/bjsports-2013-092741
Faiss, R., Leger, B., Vesin, J. M., Fournier, P. E., Eggel, Y., Deriaz, O., et al. (2013b). Significant molecular and systemic adaptations after repeated sprint training in hypoxia. PLoS ONE 8:e56522. doi: 10.1371/journal.pone.0056522
Forbes, G. B. (2000). Body fat content influences the body composition response to nutrition and exercise. Ann. N. Y. Acad. Sci. 904, 359–365. doi: 10.1111/j.1749-6632.2000.tb06482.x
Gatterer, H., Haacke, S., Burtscher, M., Faulhaber, M., Melmer, A., Ebenbichler, C., et al. (2015). normobaric intermittent hypoxia over 8 months does not reduce body weight and metabolic risk factors–a randomized, single blind, placebo-controlled study in normobaric hypoxia and normobaric sham hypoxia. Obes. Facts 8, 200–209. doi: 10.1159/000431157
Gibala, M. J., Gillen, J. B., and Percival, M. E. (2014). Physiological and health-related adaptations to low-volume interval training: influences of nutrition and sex. Sports Med. 44(Suppl. 2), S127–S137. doi: 10.1007/s40279-014-0259-6
Gibala, M. J., Little, J. P., van Essen, M., Wilkin, G. P., Burgomaster, K. A., Safdar, A., et al. (2006). Short-term sprint interval versus traditional endurance training: similar initial adaptations in human skeletal muscle and exercise performance. J. Physiol. Lond. 575, 901–911. doi: 10.1113/jphysiol.2006.112094
Gist, N. H., Fedewa, M. V., Dishman, R. K., and Cureton, K. J. (2014). Sprint interval training effects on aerobic capacity: a systematic review and meta-analysis. Sports Med. 44, 269–279. doi: 10.1007/s40279-013-0115-0
Gonzalez-Muniesa, P., Lopez-Pascual, A., de Andres, J., Lasa, A., Portillo, M. P., Aros, F., et al. (2015). Impact of intermittent hypoxia and exercise on blood pressure and metabolic features from obese subjects suffering sleep apnea-hypopnea syndrome. J. Physiol. Biochem. 71, 589–599. doi: 10.1007/s13105-015-0410-3
Hansen, D., Astrup, A., Toubro, S., Finer, N., Kopelman, P., Hilsted, J., et al. (2001). Predictors of weight loss and maintenance during 2 years of treatment by sibutramine in obesity. Results from the European multi-centre STORM trial. Sibutramine Trial of Obesity Reduction and Maintenance. Int. J. Obes. Relat. Metab. Disord. 25, 496–501. doi: 10.1038/sj.ijo.0801481
Haufe, S., Wiesner, S., Engeli, S., Luft, F. C., and Jordan, J. (2008). Influences of normobaric hypoxia training on metabolic risk markers in human subjects. Med. Sci. Sports Exerc. 40, 1939–1944. doi: 10.1249/MSS.0b013e31817f1988
Hazell, T. J., Hamilton, C. D., Olver, T. D., and Lemon, P. W. (2014). Running sprint interval training induces fat loss in women. Appl. Physiol. Nutr. Metab. 39, 944–950. doi: 10.1139/apnm-2013-0503
Heinonen, I., Boushel, R., and Kalliokoski, K. (2016). The Circulatory and metabolic responses to hypoxia in humans - with special reference to adipose tissue physiology and obesity. Front. Endocrinol. (Lausanne) 7:116. doi: 10.3389/fendo.2016.00116
Heinonen, I., Bucci, M., Kemppainen, J., Knuuti, J., Nuutila, P., Boushel, R., et al. (2012). Regulation of subcutaneous adipose tissue blood flow during exercise in humans. J. Appl. Physiol. 112, 1059–1063. doi: 10.1152/japplphysiol.00732.2011
Heinonen, I., Kemppainen, J., Kaskinoro, K., Knuuti, J., Boushel, R., and Kalliokoski, K. K. (2014). Capacity and hypoxic response of subcutaneous adipose tissue blood flow in humans. Circ. J. 78, 1501–1506. doi: 10.1253/circj.CJ-13-1273
Kayser, B., and Verges, S. (2013). Hypoxia, energy balance and obesity: from pathophysiological mechanisms to new treatment strategies. Obes. Rev. 14, 579–592. doi: 10.1111/obr.12034
Kong, Z., Zang, Y., and Hu, Y. (2014). Normobaric hypoxia training causes more weight loss than normoxia training after a 4-week residential camp for obese young adults. Sleep Breath 18, 591–597. doi: 10.1007/s11325-013-0922-4
Kwan, M. Y., Cairney, J., Faulkner, G. E., and Pullenayegum, E. E. (2012). Physical activity and other health-risk behaviors during the transition into early adulthood: a longitudinal cohort study. Am. J. Prev. Med. 42, 14–20. doi: 10.1016/j.amepre.2011.08.026
Lesser, I. A., Gasevic, D., and Lear, S. A. (2013). The effect of body fat distribution on ethnic differences in cardiometabolic risk factors of Chinese and Europeans. Appl. Physiol. Nutr. Metab. 38, 701–706. doi: 10.1139/apnm-2012-0125
Lippl, F. J., Neubauer, S., Schipfer, S., Lichter, N., Tufman, A., Otto, B., et al. (2010). Hypobaric hypoxia causes body weight reduction in obese subjects. Obesity (Silver. Spring) 18, 675–681. doi: 10.1038/oby.2009.509
Millet, G. P., Debevec, T., Brocherie, F., Malatesta, D., and Girard, O. (2016). Therapeutic use of exercising in hypoxia: promises and limitations. Front. Physiol. 7:224. doi: 10.3389/fphys.2016.00224
Millet, G. P., Roels, B., Schmitt, L., Woorons, X., and Richalet, J. P. (2010). Combining hypoxic methods for peak performance. Sports Med. 40, 1–25. doi: 10.2165/11317920-000000000-00000
Netzer, N. C., Chytra, R., and Kupper, T. (2008). Low intense physical exercise in normobaric hypoxia leads to more weight loss in obese people than low intense physical exercise in normobaric sham hypoxia. Sleep Breath. 12, 129–134. doi: 10.1007/s11325-007-0149-3
Oliveira, R. B., Myers, J., Araujo, C. G., Abella, J., Mandic, S., and Froelicher, V. (2009). Maximal exercise oxygen pulse as a predictor of mortality among male veterans referred for exercise testing. Eur. J. Cardiovasc. Prev. Rehabil. 16, 358–364. doi: 10.1097/HJR.0b013e3283292fe8
Rakobowchuk, M., Tanguay, S., Burgomaster, K. A., Howarth, K. R., Gibala, M. J., and MacDonald, M. J. (2008). Sprint interval and traditional endurance training induce similar improvements in peripheral arterial stiffness and flow-mediated dilation in healthy humans. Am. J. Physiol. Regul. Integr. Comp. Physiol. 295, R236–R242. doi: 10.1152/ajpregu.00069.2008
Rampinini, E., Connolly, D. R., Ferioli, D., La Torre, A., Alberti, G., and Bosio, A. (2016). Peripheral neuromuscular fatigue induced by repeated-sprint exercise: cycling vs. running. J. Sports Med. Phys. Fitness 56, 49–59.
Richards, J. C., Johnson, T. K., Kuzma, J. N., Lonac, M. C., Schweder, M. M., Voyles, W. F., et al. (2010). Short-term sprint interval training increases insulin sensitivity in healthy adults but does not affect the thermogenic response to beta-adrenergic stimulation. J. Physiol. 588(Pt 15), 2961–2972. doi: 10.1113/jphysiol.2010.189886
Rodriguez, F. A., Ventura, J. L., Casas, M., Casas, H., Pages, T., Rama, R., et al. (2000). Erythropoietin acute reaction and haematological adaptations to short, intermittent hypobaric hypoxia. Eur. J. Appl. Physiol. 82, 170–177. doi: 10.1007/s004210050669
Romijn, J. A., Coyle, E. F., Sidossis, L. S., Gastaldelli, A., Horowitz, J. F., Endert, E., et al. (1993). Regulation of endogenous fat and carbohydrate metabolism in relation to exercise intensity and duration. Am. J. Physiol. 265(3 Pt 1), E380–E391.
Saanijoki, T., Nummenmaa, L., Eskelinen, J. J., Savolainen, A. M., Vahlberg, T., Kalliokoski, K. K., et al. (2015). Affective responses to repeated sessions of high-intensity interval training. Med. Sci. Sports Exerc. 47, 2604–2611. doi: 10.1249/MSS.0000000000000721
Semenza, G. L. (2004). O2-regulated gene expression: transcriptional control of cardiorespiratory physiology by HIF-1. J. Appl. Physiol. 96, 1173–1177. discussion: 1170–1172. doi: 10.1152/japplphysiol.00770.2003
Shukla, V., Singh, S. N., Vats, P., Singh, V. K., Singh, S. B., and Banerjee, P. K. (2005). Ghrelin and leptin levels of sojourners and acclimatized lowlanders at high altitude. Nutr. Neurosci. 8, 161–165. doi: 10.1080/10284150500132823
Stoneham, M. D., and Pethybridge, R. J. (1993). Acclimatization to altitude: effects on arterial oxygen saturation and pulse rate during prolonged exercise at altitude. J. R. Nav. Med. Serv. 79, 3–9.
Trapp, E. G., Chisholm, D. J., Freund, J., and Boutcher, S. H. (2008). The effects of high-intensity intermittent exercise training on fat loss and fasting insulin levels of young women. Int. J. Obes. 32, 684–691. doi: 10.1038/sj.ijo.0803781
Trost, S. G., Owen, N., Bauman, A. E., Sallis, J. F., and Brown, W. (2002). Correlates of adults' participation in physical activity: review and update. Med. Sci. Sports Exerc. 34, 1996–2001. doi: 10.1097/00005768-200212000-00020
Urdampilleta, A., Gonzalez-Muniesa, P., Portillo, M. P., and Martinez, J. A. (2012). Usefulness of combining intermittent hypoxia and physical exercise in the treatment of obesity. J. Physiol. Biochem. 68, 289–304. doi: 10.1007/s13105-011-0115-1
Vogt, M., Puntschart, A., Geiser, J., Zuleger, C., Billeter, R., and Hoppeler, H. (2001). Molecular adaptations in human skeletal muscle to endurance training under simulated hypoxic conditions. J. Appl. Physiol. 91, 173–182.
Weston, M., Taylor, K. L., Batterham, A. M., and Hopkins, W. G. (2014). Effects of low-volume high-intensity interval training (HIT) on fitness in adults: a meta-analysis of controlled and non-controlled trials. Sports Med. 44, 1005–1017. doi: 10.1007/s40279-014-0180-z
Wiesner, S., Haufe, S., Engeli, S., Mutschler, H., Haas, U., Luft, F. C., et al. (2010). Influences of normobaric hypoxia training on physical fitness and metabolic risk markers in overweight to obese subjects. Obesity (Silver. Spring) 18, 116–120. doi: 10.1038/oby.2009.193
Workman, C., and Basset, F. A. (2012). Post-metabolic response to passive normobaric hypoxic exposure in sedendary overweight males: a pilot study. Nutr. Metab. 9:103. doi: 10.1186/1743-7075-9-103
Keywords: normobaric hypoxia, high-intensity interval training, maximal oxygen uptake, blood lipids, leptin, body composition
Citation: Kong Z, Shi Q, Nie J, Tong TK, Song L, Yi L and Hu Y (2017) High-Intensity Interval Training in Normobaric Hypoxia Improves Cardiorespiratory Fitness in Overweight Chinese Young Women. Front. Physiol. 8:175. doi: 10.3389/fphys.2017.00175
Received: 15 September 2016; Accepted: 07 March 2017;
Published: 23 March 2017.
Edited by:
Tadej Debevec, Jožef Stefan Institute, SloveniaReviewed by:
Franck Brocherie, Institut National du Sport, de l'Expertise et de la Performance, FranceCopyright © 2017 Kong, Shi, Nie, Tong, Song, Yi and Hu. This is an open-access article distributed under the terms of the Creative Commons Attribution License (CC BY). The use, distribution or reproduction in other forums is permitted, provided the original author(s) or licensor are credited and that the original publication in this journal is cited, in accordance with accepted academic practice. No use, distribution or reproduction is permitted which does not comply with these terms.
*Correspondence: Yang Hu, aHl5cjFAMTYzLmNvbQ==
Disclaimer: All claims expressed in this article are solely those of the authors and do not necessarily represent those of their affiliated organizations, or those of the publisher, the editors and the reviewers. Any product that may be evaluated in this article or claim that may be made by its manufacturer is not guaranteed or endorsed by the publisher.
Research integrity at Frontiers
Learn more about the work of our research integrity team to safeguard the quality of each article we publish.