- Department of Medical Biochemistry, Faculty of Medicine in Hradec Kralove, Charles University in Prague, Hradec Kralove, Czechia
A characteristic feature of liver cirrhosis is the accumulation of large amounts of connective tissue with the prevailing content of type I collagen. Elastin is a minor connective tissue component in normal liver but it is actively synthesized by hepatic stellate cells and portal fibroblasts in diseased liver. The accumulation of elastic fibers in later stages of liver fibrosis may contribute to the decreasing reversibility of the disease with advancing time. Elastin is formed by polymerization of tropoelastin monomers. It is an amorphous protein highly resistant to the action of proteases that forms the core of elastic fibers. Microfibrils surrounding the core are composed of fibrillins that bind a number of proteins involved in fiber formation. They include microfibril-associated glycoproteins (MAGPs), microfibrillar-associated proteins (MFAPs) and fibulins. Lysyl oxidase (LOX) and lysyl oxidase-like proteins (LOXLs) are responsible for tropoelastin cross-linking and polymerization. TGF-β complexes attached to microfibrils release this cytokine and influence the behavior of the cells in the neighborhood. The role of TGF-β as the main profibrotic cytokine in the liver is well-known and the release of the cytokines of TGF-β superfamily from their storage in elastic fibers may affect the course of fibrosis. Elastic fibers are often studied in the tissues where they provide elasticity and resilience but their role is no longer viewed as purely mechanical. Tropoelastin, elastin polymer and elastin peptides resulting from partial elastin degradation influence fibroblastic and inflammatory cells as well as angiogenesis. A similar role may be performed by elastin in the liver. This article reviews the results of the research of liver elastic fibers on the background of the present knowledge of elastin biochemistry and physiology. The regulation of liver elastin synthesis and degradation may be important for the outcome of liver fibrosis.
Introduction
Elastin is the most stable protein of the extracellular matrix (ECM) which is composed of collagen, glycoproteins, glycosaminoglycans and proteoglycans (Aumailley and Gayraud, 1998). It is present in large amounts in the organs whose elastic properties are essential for their function such as arteries and lungs. Elastin is synthesized around the birth when these organs begin to function and its synthesis subsides in the postnatal period. Most of the protein persists in the body for the lifetime (Shapiro et al., 1991). It may be degraded and resynthesized in various organs in pathological conditions. Elastic fibers appear in the scar in later stages of wound healing (Raghunath et al., 1996; Sproul and Argraves, 2013). Elastin accumulates in fibrotic livers regardless of fibrosis etiology (Liban and Ungar, 1959) and because of its low turnover it may contribute substantially to the irreversibility of the disease.
Elastic fibers are composed of the insoluble elastin core, microfibrils on the surface of the fibers and a number of attached proteins that help to assemble the fiber (Kielty et al., 2002). Elastic fibers are not an inert component of the ECM. Elastin precursor tropoelastin, elastin polymer and elastin degradation products all possess biological activity that may influence inflammatory cells and connective tissue cells in the organ (Almine et al., 2012). Moreover, the microfibrils bind the cytokines of the TGF-β superfamily that may influence the course of liver fibrosis, regeneration and cancer (Bissell et al., 2001; Dooley and ten Dijke, 2012).
The present review summarizes the results of elastin research in the liver on the background of the present knowledge of elastin biochemistry and physiology. The aim of this review is to encourage further studies of elastin and other components of elastic fibers in the liver because it may help to solve the problem of cirrhosis irreversibility.
Elastin Core of Elastic Fibers
Elastic fibers are composed of the elastin core and the microfibrillar mantle upon which is elastin deposited. The soluble monomer of elastin is tropoelastin (TE) possessing a molecular weight of about 72 kDa. TE is a single gene protein encoded by 36 exons that persist in many animal species. Exons 34 and 35 were lost during the evolution of human TE. Seven other exons may be alternatively spliced reflecting elastin function in different tissues (Mithieux and Weiss, 2005; Figure 1A). The exons vary in length from 27 to 114 base pairs and code for domains with alternating hydrophobic and hydrophilic structures. Hydrophobic regions contain nonpolar amino acids glycine, alanine, valine, proline, leucine and isoleucine, are arranged in repeating motifs such as GVGVAP and tend to form β turns. Hydrophobic regions confer elasticity to the protein. Hydrophilic domains are rich in lysine and alanine and tend to form α helices; lysine residues are separated by two or three alanine residues, e.g., AAKAAAKAA. The lysine residues in the hydrophilic regions give rise to cross-links that connect neighboring TE molecules. The interaction of four lysine residues in a specific spacial arrangement is required for the synthesis of desmosine and isodesmosine, the crosslinks characteristic of elastin (Rosenbloom, 1984; Vrhovski and Weiss, 1998; Wise et al., 2014).
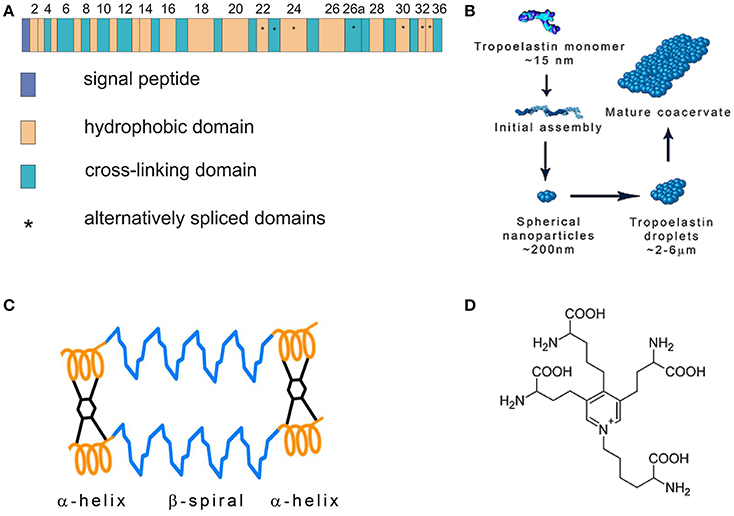
Figure 1. (A) Tropoelastin gene structure. Modified from Mithieux and Weiss (2005). (B) Tropoelastin (TE) assembly. TE molecules polymerize in a head-to-tail mode and subsequently coacervate. Modified from Wise et al. (2014). (C) Fibrillar model of elastin. Tropoelastin chains are connected with desmosine cross-links. Modified from Vrhovski and Weiss (1998). (D) Desmosine molecule.
Human TE is composed of 760 amino acid residues. It contains the N-terminal signal sequence that is removed when the newly synthesized protein enters the endoplasmic reticulum. TE interacts with the elastin binding protein (EBP) which is an enzymatically inactive variant of β-galactosidase. EBP functions as a 67 kDa chaperone that prevents intracellular TE aggregation and protects TE from proteolytic degradation. TE is packaged into secretory vesicles in Golgi apparatus (Mithieux and Weiss, 2005). After secretion into the extracellular space, TE molecules rapidly associate in a head-to-tail mode and assemble on the cell surface. This process is called coacervation (Figure 1B). The cells interact transiently with elastic fibers and globular elastin is transferred to the fibrillin containing microfibrils on the surface of the fibers (Kozel et al., 2006). The coacervation is promoted by interactions of specific regions of TE and fibrillin-1, the component of microfibrils (Clarke et al., 2005). Sialidase (neuraminidase-1) bound to EBP removes the terminal sialic acid from the carbohydrate chains of microfibrillar glycoprotein and facilitates TE binding. EBP dissociates from TE and most of it is recycled (Hinek, 1996; Debelle and Tamburro, 1999; Hinek et al., 2006).
The stabilization of coacervates on the microfibrillar scaffold requires initial oxidation of specific lysine residues and TE crosslinking (Sato et al., 2007). Protein-lysine 6-oxidase (lysyl oxidase, LOX), a copper containing enzyme, oxidizes lysine residues to α-amino adipic δ-semialdehydes (allysines) that may spontaneously interact. They form an aldol in a condensation reaction or a Schiff base when an allysine reacts with an intact lysine residue. These compounds are intermediates in the synthesis of elastin cross-links lysinonorleucine, desmosine and isodesmosine that connect neighboring TE chains (Figures 1C,D). Lysine oxidation requires enzymatic catalysis, the subsequent reactions are spontaneous (Rucker et al., 1998). TE is easily degraded by proteinases but crosslinking makes it a highly stable polymer resistant to proteolysis (Maurice et al., 2013).
Elastin in Healthy and Fibrotic Liver
Elastic fibers are found in arteries, lungs, skin, elastic ligaments and bladder—the organs subjected to stretching and expansion. However, elastin networks can also be detected in tissues whose primary function is not to provide elasticity and resilience, e.g., articular cartilage and adipose tissue (Green et al., 2014).
The use of orcein staining in histochemistry made it possible to study elastic fibers in normal and cirrhotic liver. According to Hohenemser (1895) newly formed elastic tissue in cirrhotic liver is found around the main branches of the hepatic artery and the portal vein. The fibers are less numerous in the neighborhood of fine branches of these vessels and in the acini. They surround bile ducts. Hohenemser noticed two important features of liver fibrosis. First, connective tissue in cirrhotic liver resembles the connective tissue found in the scars formed in healing wounds in the skin and other organs and elastic fibers are a result of the scar development. Second, fibrotic septa are a product of new connective tissue synthesis (Hohenemser, 1895). Thin elastic fibers are found in the liver capsule and in the portal areas of normal liver. Elastic fibers are found in enlarged portal spaces and in the internodular fibrous septa in fibrotic liver. Patches of elastin are present in the walls of sublobular and central veins. Hyperplasia of elastic fibers is increased in fibrotic liver regardless of the origin of the disease. Staining with orcein, aldehyde fuchsin, orcinol-new fuchsin and resorcin-fuchsin gives similar results (Liban and Ungar, 1959). The results yielded by classical staining can be confirmed by immunohistochemistry and electron microscopy (Porto et al., 1990a).
No elastic fibers can be detected in diseased liver when necrosis and inflammation are present. The deposition of elastic fibers is a marker of the healing of chronic active hepatitis and accompanies the appearance of large collagen bundles (Bedossa et al., 1990). There is a good correlation between the amount of elastic fibers in the liver and the duration of the illness (Thung and Gerber, 1982). New elastic fibers formed in active fibrosis can be distinguished from the areas of hepatic parenchyma collapse (Scheuer and Maggi, 1980). The deposition of orcein-positive material similar to that in cholestatic liver disease is found in the liver of patients suffering from Indian childhood cirrhosis (Portmann et al., 1978). Bundles of elastic fibers surround central venules in fibrosis accompanying Stage III of nonalcoholic steatohepatitis fibrosis (Nakayama et al., 2008). Elastic fiber deposition in the peripheral portal tracts is a characteristic feature of idiopathic portal hypertension. Sera obtained from patients with this disease induce elastin expression in vascular endothelial cells. Sera obtained from patients with chronic viral hepatitis or cirrhosis have an insignificant effect (Sato et al., 2011). Elastic tissue hyperplasia is also observed in hepatic schistosomal fibrosis (Andrade and Freitas, 1991). Hepatocellular carcinoma is a complication of decompensated cirrhosis. The accumulation of elastic fibers correlates with the development of carcinoma in advanced fibrosis (Yasui et al., 2016). The deposition of elastic fibers in human cirrhotic liver is illustrated in Figures 2A,B.
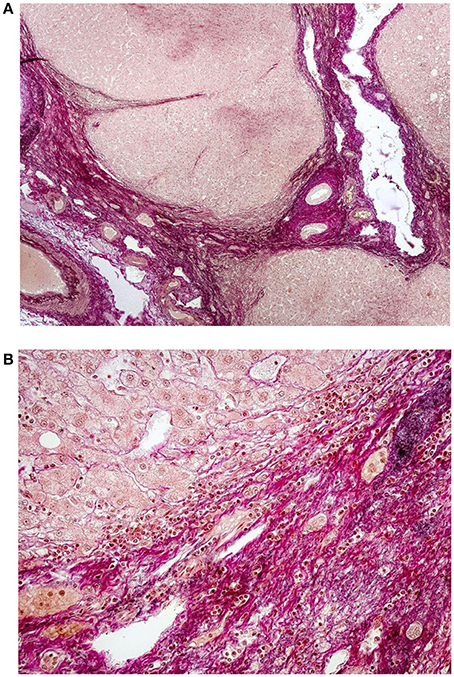
Figure 2. (A) Histology of liver cirrhosis—overview. Severe cirrhosis (magenta color) separates regenerative nodules of hepatocytes. Preexisting structures of bile ducts, portal vein branches and hepatic artery are entrapped in the fibrotic areas. Focal deposition of elastic fibers (black color) is visible; van Gieson elatic staining, 40x. (Ryška and Nová, Unpublished results). (B) Detail of marked periportal fibrosis in cirrhotic liver with deposition of fine elastic fibers. Some of the fibers irradiate into the regenerative nodules of hepatocytes; van Gieson elastic staining, 200x. (Ryška and Nová, Unpublished results).
Elastic fibers formation in the liver can be experimentally reproduced by prolonged treatment of rats with carbon tetrachloride. Elastic stain or antielastin antibody detect elastin in the septa surrounding regenerative nodules (Kanta and Bartos, 1978; Kanta et al., 2002). The ligation of the common bile duct in rats leads to the deposition of elastin in the enlarged portal zone (Lorena et al., 2004; Guyot et al., 2006). Elastin deposition is observed within a few days after bile duct obstruction, first as dots between cells and then as patches next to collagen fibers (Desmoulière et al., 1997). Sepsis induced in mice by polymicrobial polyperitonitis leads to the proliferation of elastic fibers in the portal tracts and in sublobular veins of the liver of the surviving animals (Gonnert et al., 2012). Granulomas formed in the liver of mice injected with Schistosoma mansoni eggs contain concentric orcein-positive elastic fibers (Silva et al., 2000). The content of ECM structural proteins, collagen, elastin, fibronectin and fibrillin are altered in damaged liver. The resulting changes in tissue stiffness may facilitate regeneration or fibrosis (Klaas et al., 2016).
Microfibrils of Elastic Fibers
Microscopically amorphous elastin forms the core of elastic fibers. Microfibrils 10–12 nm in diameter are located on the surface of the fiber parallel to its long axis (Mithieux and Weiss, 2005). Fibrillins are the main protein component of the microfibrils. They are glycoproteins rich in cysteine and their molecular weight is about 350 kDa. The dominating structural element of fibrillins are epidermal growth factor (EGF)-like domains, most of which bind calcium (cb-EGF domains). Calcium cations stabilize the domains and make them resistant to proteolysis. Fibrillin molecules are organized in a head-to-tail alignment (Ramirez and Sakai, 2010). The asembly of fibrillin into microfibrils requires the presence of fibronectin network. Cellular fibronectin is synthesized by fibroblasts and other cells and binds to the cell surface integrins that are connected to the actin cytoskeleton. Binding induces conformational changes in fibronectin molecules. Cellular contractility facilitates the exposing of the domains binding various ECM proteins including fibronectin itself (Mao and Schwarzbauer, 2005; Figure 3A). Isolated microfibrils have a characteristic “beads on a string” appearance. The microfibrils are stabilized by transglutaminase-formed cross-links between interbead regions of the fibrils (Kielty et al., 2002; Wang et al., 2009). Fibrillin-1 binds TE with high affinity and the two proteins may be cross-linked by transglutaminase (Rock et al., 2004). Fibrillin-2 is structurally similar to fibrillin-1 and is also implicated in extracellular tropoelastin deposition (Zhang et al., 1994; Tsuruga et al., 2005). The synthesis of fibrillin-1 parallels the growth of elastic fibers. Fibrillin-2 is produced during fetal life and tissue remodeling. Its expression is high in the fibroblasts isolated from healing wounds (Brinckmann et al., 2010; Ramirez and Sakai, 2010).
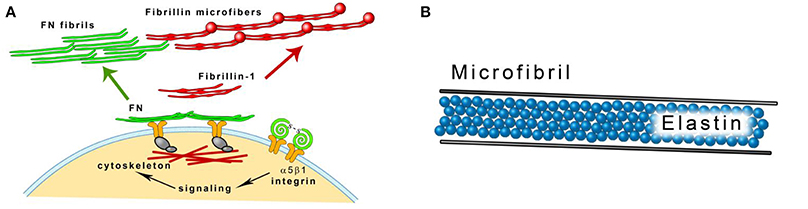
Figure 3. (A) Fibrillin microfibrils assembly. Fibrillin-1 is deposited on fibronectin (FN) template that is connected to the cell surface through integrins. Modified from Kinsey et al. (2008). (B) A scheme of an elastic fiber consisting of the elastin core and the microfibril mantle.
Three stages in the development of the elastic fiber can be distinguished by histological methods. Oxytalan fibers are composed of 10–16 nm diameter microfibrils, elaunin contains both microfibrils and amorphous material. In the mature elastic fiber, amorphous material accumulates and forms the central core (Garner and Alexander, 1986; Figure 3B). Oxytalan, elaunin and elastic fibers are all observed in developing alcoholic fibrosis of the liver (Porto et al., 1990b). In normal liver, fibrillin-1 colocalizes with elastin in vessel walls and in portal tract connective tissue. It can also be detected in the absence of elastin. In cirrhotic liver, it can be found in association with elastin in fibrotic septa surrounding regenerative nodules and alone in the perisinudoidal spaces (Dubuisson et al., 2001). Fibrillin-1 is often coexpressed with elastin in children cholestatic diseases but it has a wider distribution in the liver (Lamireau et al., 2002).
Proteins Associated with Elastic Fibers
A number of proteins are associated with fibrillin microfibrils and influence the properties of elastic fibers (Baldwin et al., 2013). Latent transforming growth factor-β binding proteins (LTBPs) are large glycoproteins (m.w. 125–240 kDa) structurally similar to fibrillin. The family of LTBPs has four members that colocalize with fibrillin microfibrils (Zilberberg et al., 2012; Robertson et al., 2015). LTBPs with the exception of LTBP-2 are secreted from most cells as a large latent complex (LLC) with the latency-associated peptide (LAP)-dimer and the transforming growth factor-β (TGF-β)-dimer (Koli et al., 2001; Figure 4A). LLC attaches to fibrillin during the assembly of microfibrils (Massam-Wu et al., 2010).
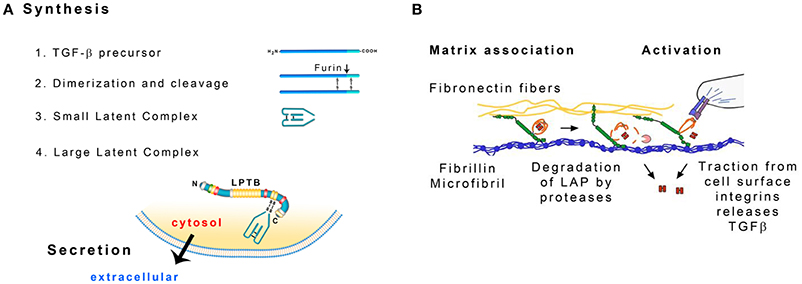
Figure 4. (A) Formation of the large latent complex (LLC). TGF-β precursors dimerize and the dimers are cleaved by furin protease. The liberated fragments form the small latent complex (SLC) that contains noncovalently bonded TGF-β. SLC is attached to the latent TGF-β binding protein (LTBP). Modified from Hayashi and Sakai (2012). (B) Possible ways to release TGF-β from the Large Latent Complex: degradation of the latency-associated peptide (LAP) by proteases and distortion of the LAP by cellular traction. Modified from Robertson et al. (2015).
LAP and TGF-β are synthesized as a single polypetide chain. Two chains associate to form a dimer that is then cleaved by the endoprotease furin (Koli et al., 2001). As a result LAP dimer (80 kDa) surrounds TGF-β dimer (25 kDa). TGF-β is noncovalently bonded to LAP and LAP is bonded to LTBP through disulfide bonds. LAP prevents TGF-β from interacting with its receptor and signaling. The complex of LAP and TGF-β is called a small latent complex (SLC) (Hayashi and Sakai, 2012; Figure 4A).
Cultured human liver myofibroblasts (MFBs) isolated from liver biopsies express all components forming the large latent complex of TGF-β: four LTBP isoforms and their splice variants, LAP and three TGF-β isoforms, suggesting the importance of TGF-β activity in diseased liver (Mangasser-Stephan et al., 2001). TGF-β1 promotes activation and myofibroblastic differentiation of hepatic stellate cells (HSCs), the key event in liver fibrogenesis (Hayashi and Sakai, 2012). LTBP-1 mRNA can be detected in fibrotic septa in rat liver. It is synthesized by HSCs transdifferentiating into myofibroblasts. TGF-β can be released from the complex with LTBP by plasmin treatment (Breitkopf et al., 2001). As TGF-β is expressed throughout the hepatic parenchyma, LTBP-1 present in the portal spaces may bind TGF-β and target it to these areas (Corchero et al., 2004). LTBP-1 knockout mice are less prone to hepatic fibrogenesis induced by bile duct ligation (Drews et al., 2008).
TGF-β needs to be activated before it exerts its biological activity. The interaction between LAP and TGF-β may be disrupted in vitro by extreme pH, chaotropic agents or heat treatment (Koli et al., 2001). LTBP may be cleaved by proteases in cell culture. Plasmin, elastase and a variety of matrix metalloproteases degrade this protein (Dallas et al., 2002). Cellular receptors integrins may serve to bring metalloproteases MT-1 MMP, MMP-9 or MMP-2 into the vicinity of the LLC that is attached to a microfibril. LTBP and LAP are then cleaved and TGFβ is released (Wipff and Hinz, 2008). Traction forces generated by the cells may also facilitate TGF-β release. The αv integrins on the cell surface recognize the arg-gly-asp (RGD) amino acid sequence present in LAP and bind the whole complex. LTBP is anchored in the mechanically resistant matrix and when MFBs contract, the conformation of LAP may change and release TGF-β (Wipff and Hinz, 2008; Figure 4B). According to an alternative mechanism, fibrillin fragments released by proteolysis may inhibit the interaction of fibrillin and LTBP-1 leading to the release of LLC and increase in active TGFβ concentration (Chaudhry et al., 2007).
Thrombospondin-1 may bind to the SLC and the resulting ternary complex of thrombospondin, LAP and TGF-β is biologically active (Murphy-Ullrich and Poczatek, 2000). HSCs are stimulated by platelet derived growth factor (PDGF)-BB to proliferation and migration. The profibrogenic cytokine PDGF-BB stimulates the coexpression of TGF-β1 and thrombospondin-1 in these cells (Breitkopf et al., 2005). In cholestasis, latent TGF-β is activated by thrombospondin-1 whose expression in hepatocytes is induced by bile acids (Myung et al., 2007). LTBP-2 does not form covalent complexes with latent TGF-β but it competes with LTBP-1 for binding sites on fibrillin-1. It is abundant in developing, elastin containing tissues and may negatively modulate LLC storage in the ECM (Hirani et al., 2007).
TGF-β1 is a major profibrogenic cytokine in the liver. It is produced by sinusoidal endothelial cells and Kupffer cells in normal liver. Activated HSCs become the principal source of TGF-β in injured liver and are its primary target. The cytokine stimulates HSCs proliferation, their dedifferentiation to MFBs and ECM synthesis (Bissell et al., 2001; Dooley and ten Dijke, 2012). TGF-β hinders DNA synthesis in hepatocytes and causes their apoptosis or epithelial to mesenchymal transition. It has a bipartite role in hepatocellular carcinoma. It suppresses tumor growth at early stages but it promotes tumor invasiveness and metastasis later (Dooley and ten Dijke, 2012; Meindl-Beinker et al., 2012). TGF-β1 increases fibrillin-1 expression in cultured liver fibroblasts (Lorena et al., 2004).
Fibrillin binds a few members of bone morphogenetic protein (BMP) family (Sengle et al., 2008; Ramirez and Sakai, 2010). Cytokines TGF-β and BMP belong to the TGF-β superfamily. Because of the important role they play in fibrosis they are considered as potential therapeutic targets. The actions of these cytokines are mediated by the intracellular proteins Smads (Munoz-Félix et al., 2015). BMPs regulate cell proliferation, apoptosis, differentiation and migration and have been associated with liver diseases and regeneration (Sugimoto et al., 2007; Herrera et al., 2012).
Microfibril-associated glycoproteins 1 and 2 (MAGP-1, -2) attach to fibrillin. Their molecular weight is small, about 25 kDa. MAGP-1 binds TE and members of TGF-β and BMP family. It may modify TGFβ signaling and tissue homeostasis (Weinbaum et al., 2008; Mecham and Gibson, 2015). Elastin microfibril interface-located proteins (EMILINs) are a family of ECM proteins. EMILIN-1 binds to elastin ad fibulin-5 and is adhesive to cells. It is located at the surface of elastin core. Elastogenesis is impaired in EMILIN-1 deficient mice. Cells lose close contact with elastic fibers, which negatively influences their morphology (Zanetti et al., 2004). EMILIN-1 has not yet been detected in the liver. Microfibrillar-associated proteins (MFAPs) are low molecular weight proteins different from MAGPs (Mecham and Gibson, 2015). MFAP4 is a glycoprotein that binds TE and fibrillin-1 and -2 and the crosslinking amino acid desmosine in vitro. It promotes TE coacervation and is necessary for microfibril assembly (Kasamatsu et al., 2011; Pilecki et al., 2016). It is abundant in HSCs and in fibrotic septa of human cirrhotic liver (Mölleken et al., 2009). The expression of MFAP4 in fibrotic liver increases with fibrosis stage both on gene transcript level and on protein level (Bracht et al., 2015).
Fibulins are a family of glycoproteins that comprises proteins with large molecular weight, fibulin-1 (90–100 kDa) and fibulin-2 (200 kDa), and proteins much smaller, fibulin-3, -4, and -5 (50–60 kDa). Their characteristic feature is the presence of calcium-binding epidermal growth factor-like (cbEGF) domains and the fibulin-type C-terminal region. All of them bind TE but they have different roles in elastic fibers formation (Kobayashi et al., 2007). Fibulin-1 is present in the amorphous core of the elastic fiber (Roark et al., 1995), fibulin-2 colocalizes with fibrillin-1 (El-Hallous et al., 2007). The synthesis of fibulin-2 and elastin is highly coordinated (Hunzelmann et al., 2001). Fibulin-4, LOX, and TE form a ternary complex. This complex regulates LOX activation and elastin crosslinking before elastin deposition on the microfibrils. The lack of fibulin-4 causes down-regulation of TE expression in fibroblasts and the formation of irregular elastin aggregates instead of elastic fibers (McLaughlin et al., 2006). The knock-down of fibulin-4 expression in human fibroblasts is accompanied by reduced expression of TE mRNA (Chen et al., 2009). Fibulin-5 is deposited on fibrillin-1 microfibrils in the culture of human fibroblasts (Hirai et al., 2007). It associates with elastin globules and facilitates their deposition on microfibrils (Choudhury et al., 2009). Fibulin-5 gene is induced only in elastin producing cells. Fibulin-5 mRNA expression follows the expression of tropoelastin mRNA and is decreased when the cells are transfected with tropoelastin siRNA (Tsuruga et al., 2004).
Fibulin-1 and -2 are detectable in portal areas of normal rat liver. Their expression increases in acutely injured liver. Fibulin-1 is detectable in hepatocytes, activated HSCs and MFBs but fibulin-2 expression is restricted to MFBs. Fibrotic septa in cirrhotic liver are positive both for fibulin-1 and -2 (Knittel et al., 1999; Piscaglia et al., 2009). Fibulin-2 mediates the action of TGF-β in myocardial fibrosis (Khan et al., 2016) but it is not known if it functions in this way in the liver. The expression of fibulin-5 in liver biopsies of patients with hepatitis B or C associated fibrosis increases with fibrosis stage (Bracht et al., 2015). Fibulin-5 can frequently be detected in the major portal vein branches in patients with idiopathic portal hypertension and its distribution corresponds to that of elastic fibers (Sato et al., 2008).
Lysyl oxidase (LOX) is involved in crosslinking both collagen and elastin. Fibulin-4 interacts with lysyl oxidase (LOX) propetide, tethers LOX to TE and facilitates elastin crosslinking (Horiguchi et al., 2009). Fibulin-5 targets lysyl oxidase-like protein 1 (LOXL1) to the sites of elastogenesis. Binding to fibulin-5 brings LOXL1 into juxtaposition with its TE substrate. LOXL1 is closely associated with elastic fibers in contrast to LOX that is distributed more broadly (Liu et al., 2004). LOX can be detected in fibrotic septa in experimental liver fibrosis (Siegel et al., 1978) and in human viral hepatitis and primary biliary cirrhosis (Vadasz et al., 2005). Members of LOX family are present in HSCs and in portal fibroblasts of healthy and injured liver (Perepelyuk et al., 2013).
Clusterin is an extracellular chaperone that forms complexes with proteins via hydrophobic interactions and stabilizes them on their way to correct folding (Poon et al., 2002). It is present on the outside of abnormal elastic fibers in skin disorders and may protect them from degradation (Aigelsreiter et al., 2013). Clusterin is present in bile in cholestatic diseases. It colocalizes with elastic fibers in liver portal tracts but these complexes are absent from the areas of pericellular fibrosis. Collagen, hepatocytes or cholangiocytes are not stained with clusterin antibodies (Aigelsreiter et al., 2009). It is not clear if clusterin only labels elastic fibers or if it supports their deposition.
Proteoglycans contain a protein core substituted with glycosaminoglycan chains. They are categorized according to their molecular weight and the composition of the glycosaminoglycans. Proteoglycans of various composition, perlecan, versican, and decorin are attached to fibrillin microfibrils (Ramirez and Sakai, 2010). Negatively charged glycosaminoglycan chains interact with positively charged amino groups of lysine residues in TE which may change when the amino groups are oxidized by LOX (Broekelman et al., 2005). Heparan sulfate proteoglycans regulate microfibril assembly and elastin deposition on microfibrils (Cain et al., 2008). Chondroitin sulfate proteoglycans in excess cause abnormal deposition of fibrillin-1 and decreased production of TE and fibulin-5 (Ikeda et al., 2009). Small leucine-rich chondroitin sulfate proteoglycans biglycan and decorin may have a role in the formation of elastic fiber. Biglycan forms a ternary complex with TE and MAGP-1 (Reinboth et al., 2002), decorin forms a complex with fibrillin-1 and MAGP-1 (Trask et al., 2000).
Proteoglycan content in cirrhotic human liver increases significantly. The content of heparan sulfate that prevails in normal liver increases two-fold and the increase in dermatan sulfate and chondroitin sulfate is even more dramatic. Activated HSCs are the principal site of their synthesis (Gressner et al., 1994). Hepatocytes, bile ductular cells and Kupffer cells contribute to proteoglycan synthesis in cholestasis (Roskams et al., 1996). Perlecan and syndecan-1 are localized in the sinusoids of healthy human liver, perlecan is deposited in the fibrotic septa in cirrhotic liver (Tátrai et al., 2010). Perlecan and decorin are also deposited in rat liver and they are expressed in HSCs activated in vitro by culture on plastic (Gallai et al., 1996). However, the association of proteoglycans with elastin synthesis and deposition has not been studied in the liver.
Elastin Synthesis and Degradation
Elastin is expressed both in portal fibroblasts and in HSCs. Elastin can be detected in isolated portal fibroblasts by immunocytochemistry (Li et al., 2007). Elastin mRNA expression in these cells increases after bile duct ligation (Perepelyuk et al., 2013). The expression of elastin by cell lines derived from portal fibroblasts can be demostrated by mRNA determination, immunoblotting and immunocytochemistry (Fausther et al., 2015). The expression of TE mRNA in HSCs is much lower both in normal and in acutely injured liver (Perepelyuk et al., 2013). TE is expressed in primary HSCs both on mRNA and protein level and is secreted into the culture medium (Kanta et al., 2002). TE can be detected by immunocytochemistry in primary HSCs (Pellicoro et al., 2012). Ethanol stimulates HSC transdifferentiation by causing changes in chromatin structure. The expression of ECM proteins including elastin is increased in this process (Page et al., 2015). Overexpression of miR-29a leads to downregulation of elastin and other ECM proteins in HSC (Li et al., 2011).
Fibrotic septa in the liver are strongly positive for elastin that colocalizes with α-SMA containing myofibroblasts (Kanta et al., 2002; Guyot et al., 2006) but elastin is not detected in activated HSCs in the parenchyma (Lorena et al., 2004). HSCs give rise to 82–96% of myofibroblasts in models of both toxic and cholestatic fibrosis (Mederacke et al., 2013). Portal fibroblasts are the major source of myofibroblasts in early phase of cholestatic liver injury. However, their relative contribution to septa formation decreases as HSCs become activated and attracted chemotactically to the portal spaces (Kinnman and Housset, 2002; Iwaisako et al., 2014; Lua et al., 2016). The majority of elastin present in the fibrotic septa may be a product of HSC-derived MFBs.
Elastin expression is regulated mostly on transcriptional and posttrancriptional level. A number of cytokines participate in elastin regulation in developing and diseased organs. TGF-β1 is one of the most important (Sproul and Argraves, 2013). Mechanical forces play a role in the regulation of elastin synthesis. Collagen and elastin synthesis increases in pulmonary arteries during a period of hypoxic pulmonary hypertension. These changes are reversible but persist if the hypertension becomes chronic (Poiani et al., 1990; Durmowicz et al., 1994). Stretching of aortic smooth muscle cells (SMCs) on culture dishes with deformable bottoms or centrifugation of fibroblasts in cell culture flasks leads to the activation of tropolastin gene and increased tropoelastin synthesis (Sutcliffe and Davidson, 1990; Redlich et al., 2004). Liver stiffness reflects the progression of liver fibrosis. Median area ratios of collagen and elastin fibers correlate well with increasing liver stiffness measured by transient elastography (Abe et al., 2013). Increased stiffness of damaged liver which contributes to HSC activation initially results from a combination of hepatocyte edema, bilirubin elevation and intrahepatic collagen deposition (Dechêne et al., 2010). β-aminopropionitrile, the inhibitor of LOX, partially abolishes the increase in liver stiffness and α-SMA expression (Georges et al., 2007). Elastin fibers are formed later (Klaas et al., 2016). Fibrillin-1 expression in portal fibroblasts and in liver MFBs cultured in restrained collagen gel lattices is larger than that in the cells embedded in freely floating lattices (Lorena et al., 2004).
Elastin is synthesized during fetal life and in the postnatal period. As a result of crosslinking it becomes an insoluble and extremely stable protein. It is the most stable component of ECM. In spite of that it is slowly degraded and small amounts of elastin peptides (micrograms/ml) circulate in the blood sera of healthy individuals (Shapiro et al., 1991; Robert and Labat-Robert, 2014). ECM remodeling is mediated primarily by matrix metalloproteinases (MMPs) containing zinc in their active center. MMP-2 (gelatinase A), -7 (matrilysin), -9 (gelatinase B), and -12 (elastase) are active against elastin. Neutrophil elastase is a serine protease, most cathepsins cleaving elastin belong to cysteine proteases (Qin, 2015). Proteases degrading elastin often cleave fibrillin microfibrils (Sherratt, 2009). Physiological elastin synthesis and degradation in the adulthood occurs in the involuting uterus (Starcher and Percival, 1985). Large amounts of elastin are degraded and elastin synthesis may be reactivated in pathological conditions, e.g., in lung emphysema. Elastin fragments produced by the action of macrophage elastase, MMP-12, attract peripheral blood monocytes (Houghton et al., 2006). Desmosine content in rat cirrhotic liver, which is a measure of mature elastin, increases but elastase-like activity in the organ does not change (Velebný et al., 1983). CCl4 and thioacetamide cause severe liver injury both in WT and MMP-12−∕− mice but the accumulation of elastin is much more pronounced in MMP-12 knockout mice. Elastase produced by a subset of hepatic macrophages degrades elastin in the liver and its deficiency leads to enhanced elastin accumulation in experimental liver fibrosis. No spontaneous fibrosis is observed in either group of animals but, after long-term treatment with thioacetamide, increased accumulation of collagen is observed in knockout compared with WT mice (Pellicoro et al., 2012).
Regulatory Role of Elastin
Elastic fibers provide elasticity and resilience to the organs that are subject to repetitive distension and physiological stress (aorta, lungs, skin). However, this is not their only function. The regulatory role of TE, elastin polymer and products of elastin degradation have become prominent in recent years (Lannoy et al., 2014). Elastin acts as an autocrine factor that inhibits the proliferation of vascular SMCs in arteries and keeps them in a quiescent contractile state. Mice lacking elastin gene (Eln−/−) die from occlusive fibrocellular pathology caused by subendothelial proliferation and accumulation of vascular SMCs in early neonatal life. SMCs isolated from newborn Eln−/− pups proliferate at a much higher rate than Eln+/+ cells. Eln−/− SMCs show a remarkable paucity of actin stress fibers that can be reversed by recombinant TE treatment. TE inhibits SMC migration and proliferation in culture (Karnik et al., 2003a). Insoluble elastin incorporated into collagen scaffolds reduces their stiffness in a concentration dependent manner and modulates SMC phenotype toward a contractile state (Ryan and O'Brien, 2015; Nguyen et al., 2016). Eln+/+ cells have a spindle-shaped morphology with prominent stress fibers in contrast to the epitheloid-like morphology of Eln−/− cells (Karnik et al., 2003b).
Hydrophilic domains of tropoelastin rich in lysine are often involved in cross-link formation and the products of elastolytic cleavage are fragments of the hydrophobic parts of the molecule containing the hexapeptide val-gly-val-ala-pro-gly (VGVAPG) and other peptides containing GXXPG sequence where X is a hydrophobic amino acid. These peptides are biologically active (Almine et al., 2010). Elastin peptides (EP) sometimes called elastokines may bind to the elastin receptor complex consisting of EBP, neuraminidase and protective protein-cathepsin A and located on the cell surface (Adair-Kirk and Senior, 2008; Maurice et al., 2013). Integrin αvβ3 binds the sequence RKRK at the C-terminal of TE (Rodgers and Weiss, 2004; Almine et al., 2013). Galectin-3 occurring on breast carcinoma cells can be regarded as the third elastin binding receptor (Ochieng et al., 1999). EP undergo phase transition and self-assembly above a certain critical temperature which increases EP concentration and makes their action more effective (Yuan and Koria, 2016).
Fragments of ECM including elastin are chemotactic for monocytes and polymorphonuclear leucocytes (Hauck et al., 1995; Houghton et al., 2006). They induce changes in gene expression of inflammatory cells (Adair-Kirk and Senior, 2008). EP stimulate MMP-12 secretion by fibroblasts. This proteinase may in turn produce more elastokines (Almine et al., 2013). The expression of proinflammatory cytokines TNF-α, IL-1β, and IL-6 in monocytes is suppressed by EP and elastin fragments thus modify inflammation (Baranek et al., 2007).
Elastin hydrolysate and EP stimulate the proliferation of fibroblasts and SMC (Chatron-Colliet et al., 2015; Shiratsuchi et al., 2016) and enhance angiogenesis (Robinet et al., 2005). EP stimulate production of some MMPs (Robinet et al., 2005; Duca et al., 2007) and decrease elastin synthesis (Wachi et al., 1995). Protein kinases ERK1/2 are involved in MMP regulation (Duca et al., 2007). TE modulates the release of vascular endothelial growth factor (VEGF) and connective tissue growth factor (CTGF) from SMC stimulated by TGF-β1 (Reddel et al., 2013).
Elastic Fibers in the Diagnosis of Liver Diseases
Elastin that accumulates in the liver with advancing fibrosis can be used to diagnose the disease. Magnetic resonance with an elastin-specific contrast agent containing gadolinium may be used as a noninvasive diagnostic technique (Ehling et al., 2013). Accumulation of elastic fibers in the liver is a major predictor for the development of hepatocellular carcinoma, independently of collagen fibers (Yasui et al., 2016). MFAP4 expression is low in the liver compared to elastic tissues (Wulf-Johansson et al., 2013). However, MAFP4 concentration in the plasma of patients with chronic hepatitis and cirrhosis is increased and positively correlates with liver stiffness measured by transient elastography. The increase in plasma MFAP4 level in patients with liver disease is higher than that observed in patients with heart or other diseases (Sækmose et al., 2013, 2015).
ECM markers, degraded collagen III, collagen V and elastin, correlate with galactose elimination capacity and with the degree of portal hypertension (Leeming et al., 2013). The concentration of circulating EP may reflect ECM remodeling and predict survival time in patients with transjugular intrahepatic porto-systemic shunt (Nielsen et al., 2015). Clusterin is present in serum. Its concentration decreases in patients with alcoholic cirrhosis and is correlated with their survival (Høgåsen et al., 1996). The presence of desmosines, degradation products of elastin, in urine is a potential diagnostic marker of liver fibrosis (Afdhal et al., 1997; Stone, 2000).
Conclusions
The formation of fibrotic septa is regarded as a negative outcome of liver diseases. Elastic fibers contribute to the stability of the septa. All aspects of the presence of elastic fibers in the liver are not known, however. The synthesis of individual components of elastic fibers in the liver and their assembly is likely to follow the general pattern of elastic fibers formation in the organism. The knowledge of the regulation of elastogenesis obtained in the studies done in other organs may provide a useful inspiration for the study of elastogenesis in the liver. The emerging regulatory role of fibulins is an example. The research done in human cells should be given a priority because of the differences in the splicing of the primary TE gene transcript in various animal species. Elastin and other components of elastic fibers may also be useful as diagnostic tools in hepatology.
Author Contributions
The author confirms being the sole contributor of this work and approved it for publication.
Conflict of Interest Statement
The author declares that the research was conducted in the absence of any commercial or financial relationships that could be construed as a potential conflict of interest.
The reviewer PK and handling Editor declared their shared affiliation, and the handling Editor states that the process nevertheless met the standards of a fair and objective review.
Acknowledgments
This research was supported by Charles University grant PRVOUK P37/01. The author wants to express his thanks to Dr. Aleš Ryška and Dr. Markéta Nová for providing histological pictures and to Dr. Miloš Hroch for his help with preparing Figures.
References
Abe, T., Hashiguchi, A., Yamazaki, K., Ebinuima, H., Saito, H., Kumada, H., et al. (2013). Quantification of collagen and elastic fibers using whole-slide images of liver biopsy specimens. Pathol. Int. 63, 305–310. doi: 10.1111/pin.12064
Adair-Kirk, T. L., and Senior, R. M. (2008). Fragments of extracellular matrix as mediators of inflammation. Int. J. Biochem. Cell Biol. 40, 1101–1110. doi: 10.1016/j.biocel.2007.12.005
Afdhal, N. H., Keaveny, A. P., Cohen, S. B., Nunes, D. P., Maldonado, N., O'Brien, M., et al. (1997). Urinary assays for desmosine and hydroxylysylpyridinoline in the detection of cirrhosis. J. Hepatol. 27, 993–1002.
Aigelsreiter, A., Janig, E., Sostaric, J., Pichler, M., Unterthor, D., Halasz, J., et al. (2009). Clusterin expression in cholestasis, hepatocellular carcinoma and liver fibrosis. Histopathology 54, 561–570. doi: 10.1111/j.1365-2559.2009.03258.x
Aigelsreiter, A., Pichler, M., Pixner, T., Janig, E., Schuller, M., Lackner, C., et al. (2013). Clusterin expression in elastofibroma dorsi. Histol. Histopathol. 28, 597–603.
Almine, J. F., Bax, D. V., Mithieux, S. M., Nivison-Smith, L., Rnjak, J., Waterhouse, A., et al. (2010). Elastin-based materials. Chem. Soc. Rev. 39, 3371–3379. doi: 10.1039/b919452p
Almine, J. F., Wise, S. G., Hiob, M., Singh, N. K., Tiwari, K. K., Vali, S., et al. (2013). Elastin sequences trigger transient proinflammatory responses by human dermal fibroblasts. FASEB J. 27, 3455–3465. doi: 10.1096/fj.13-231787
Almine, J. F., Wise, S. G., and Weiss, A. S. (2012). Elastin signaling in wound repair. Birth Defects Res. Part C 96, 248–257. doi: 10.1002/bdrc.21016
Andrade, Z. A., and Freitas, L. A. R. (1991). Hyperplasia of elastic tissue in hepatic schistosomal fibrosis. Mem. Inst. Oswaldo Cruz 86, 447–456. doi: 10.1590/S0074-02761991000400014
Aumailley, M., and Gayraud, B. (1998). Structure and biological activity of the extracellular matrix. J. Mol. Med. 76, 253–265. doi: 10.1007/s001090050215
Baldwin, A. K., Simpson, A., Steer, R., Cain, S. A., and Kielty, C. M. (2013). Elastic fibres in health and disease. Expert Rev. Mol. Med. 15:e8. doi: 10.1017/erm.2013.9
Baranek, T., Debret, R., Antonicelli, F., Lamkhioued, B., Balaaouaj, A., Hornebeck, W., et al. (2007). Elastin receptor (spliced galactosidase) occupancy by elastin peptides counteracts proinflammatory cytokine expression in lipopolysaccharide-stimulated human monocytes through NF-κB down-regulation. J. Immunol. 179, 6184–6192. doi: 10.4049/jimmunol.179.9.6184
Bedossa, P., Lemaigre, G., Paraf, F., and Martin, E. (1990). Deposition and remodelling of elastic fibres in chronic hepatitis. Virchows Arch. A Pathol. Anat. 417, 159–162. doi: 10.1007/BF02190534
Bissell, D. M., Roulot, D., and George, J. (2001). Transforming growth factor β and the liver. Hepatology 34, 859–867. doi: 10.1053/jhep.2001.28457
Bracht, T., Schweinsberg, V., Trippler, M., Kohl, M., Ahrens, M., Padden, J., et al. (2015). Analysis of disease-associated protein expression using quantitative proteomics—fibulin-5 is expressed in association with hepatic fibrosis. J. Proteome Res. 14, 2278–2286. doi: 10.1021/acs.jproteome.5b00053
Breitkopf, K., Lahme, B., Tag, C. G., and Gressner, A. M. (2001). Expression and matrix deposition of latent transforming growth factor β binding proteins in normal and fibrotic rat liver and transdifferentiating hepatic stellate cells in culture. Hepatology 33, 387–396. doi: 10.1053/jhep.2001.21996
Breitkopf, K., Sawitza, I., Westhoff, J. H., Wickert, L., Dooley, S., and Gressner, A. M. (2005). Thrombospondin 1 acts as a strong promoter of transforming growth factor β effects via two distinct mechanisms in hepatic stellate cells. Gut 54, 673–681. doi: 10.1136/gut.2004.042911
Brinckmann, J., Hunzelmann, N., Kahle, B., Rohwedel, J., Kramer, J., Gibson, M. A., et al. (2010). Enhanced fibrillin-2 expression is a general feature of wound healing and sclerosis: potential alteration of cell attachment and storage of TGF-β. Lab. Invest 90, 739–752. doi: 10.1038/labinvest.2010.49
Broekelman, T. J., Kozel, B. A., Ishibashi, H., Werneck, C. C., Keeley, F. W., Zhang, L., et al. (2005).Tropoelastin interacts with cell-surface glycosaminoglycans via its COOH-terminal domain. J. Biol. Chem. 280, 40939–40947. doi: 10.1074/jbc.M507309200
Cain, S. A., Baldwin, A. K., Mahalingam, Y., Raynal, B., Jowitt, T. A., Shuttleworth, C. A., et al. (2008). Heparan sulfate regulates fibrillin-1 N- and C-terminal interactions. J. Biol. Chem. 283, 27017–27027. doi: 10.1074/jbc.M803373200
Chatron-Colliet, A., Lalun, N., Terryn, C., Kurdykowski, S., Lorenzato, M., Rusciani, A., et al. (2015). The elastin peptide (VGVAPG)3 induces the 3D reorganisation of PML-NBs and SC35 speckles architecture, and accelerates proliferation of fibroblasts and melanoma cells. Histochem. Cell Biol. 143, 245–258. doi: 10.1007/s00418-014-1274-2
Chaudhry, S. S., Cain, S. A., Morgan, A., Dallas, S. L., Shuttleworth, C. A., and Kielty, C. M. (2007). Fibrillin-1 regulates the bioavailability of TGFβ1. J. Cell Biol. 176, 355–367. doi: 10.1083/jcb.200608167
Chen, Q., Zhang, T., Roshetsky, J. F., Ouyang, J., Essers, J., Fan, C., et al. (2009). Fibulin-4 regulates expression of the tropoelastin gene and consequent elastic-fibre formation by human fibroblasts. Biochem. J. 423, 79–89. doi: 10.1042/BJ20090993
Choudhury, R., McGovern, A., Ridley, C., Cain, S. A., Baldwin, A., Wang, M.-C., et al. (2009). Differential regulation of elastic fiber formation by fibulin-4 and -5. J. Biol. Chem. 284, 24553–24567. doi: 10.1074/jbc.M109.019364
Clarke, A. W., Wise, S. G., Cain, S. A., Kielty, C. M., and Weiss, A. S. (2005). Coacervation is promoted by molecular interactions between the PF2 segment of fibrillin-1 and the domain 4 region of tropoelastin. Biochemistry 44, 10271–10281. doi: 10.1021/bi050530d
Corchero, J., Martín-Partido, G., Dallas, L., and Fernández-Salguerro, P. M. (2004). Liver portal fibrosis in dioxin receptor-null mice that overexpress the latent transforming growth factor-β-binding protein-1. Int. J. Exp. Path. 85, 295–302. doi: 10.1111/j.0959-9673.2004.00397.x
Dallas, S. L., Rosser, J. L., Mundy, G. R., and Bonewald, L. F. (2002). Proteolysis of latent transforming growth factor-β (TGF-β)-binding protein-1 by osteoclasts. A cellular mechanism for release of TGF-β from bone matrix. J. Biol. Chem. 277, 21352–21360. doi: 10.1074/jbc.M111663200
Debelle, L., and Tamburro, A. M. (1999). Elastin: molecular description and function. Int. J. Biochem. Cell Biol. 31, 261–272. doi: 10.1016/S1357-2725(98)00098-3
Dechêne, A., Sowa, J. P., Gieseler, R. K., Jochum, C., Bechmann, L. P., El Fouly, A., et al. (2010). Acute liver failure is associated with elevated liver stiffness and hepatic cell activation. Hepatology 52, 1008–1016. doi: 10.1002/hep.23754
Desmoulière, A., Darby, I., Costa, A. M. A., Raccurt, M., Tuchweber, B., Sommer, P., et al. (1997). Extracellular matrix deposition, lysyl oxidase expression, and myofibroblastic differentiation during the initial stages of cholestatic fibrosis in the rat. Lab. Invest. 76, 765–778.
Dooley, S., and ten Dijke, P. (2012). TGF-β in progression of liver disease. Cell Tissue Res. 347, 245–256. doi: 10.1007/s00441-011-1246-y
Drews, F., Knobel, S., Moser, M., Muhlack, K. G., Mohren, S., Stoll, C., et al. (2008). Disruption of the latent transforming growth factor-β binding protein-1 gene causes alteration in facial structure and influences TGF-β bioavailability. Biochim. Biophys. Acta 1783, 34–48. doi: 10.1016/j.bbamcr.2007.08.004
Dubuisson, L., Lepreux, S., Bioulac-Sage, P., Balabaud, C., Costa, A. M. A., Rosenbaum, J., et al. (2001). Expression and cellular localization of fibrillin-1 in normal and pathological human liver. J. Hepatol. 34, 514–522. doi: 10.1016/S0168-8278(00)00048-9
Duca, L., Blanchevoye, C., Cantarelli, B., Ghoneim, C., Dedieu, S., Delacoux, F., et al. (2007). The elastin receptor complex transduces signals through the catalytic activity of its Neu-1 subunit. J. Biol. Chem. 282, 12484–12491. doi: 10.1074/jbc.M609505200
Durmowicz, A. G., Parks, W. C., Hyde, D. M., Mecham, R. P., and Stenmark, K. R. (1994). Persistence, re-expression, and induction of pulmonary arterial fibronectin, tropoelastin, and type I procollagen mRNA expression in neonatal hypoxic pulmonary hypertension. Am. J. Pathol. 145, 1411–1420.
Ehling, J., Bartneck, M., Fech, V., Butznach, B., Cesati, R., Botnar, R., et al. (2013). Elastin-based molecular MRI of liver fibrosis. Hepatology 58, 1517–1518. doi: 10.1002/hep.26332
El-Hallous, E., Sasaki, T., Hubmacher, D., Getie, M., Tiedemann, K., Brinckmann, J., et al. (2007). Fibrillin-1 interactions with fibulins depend on the first hybrid domain and provide an adaptor function to tropoelastin. J. Biol. Chem. 282, 8935–8946. doi: 10.1074/jbc.M608204200
Fausther, M., Goree, J. R., Lavoie, É. G., Graham, A. L., Sévigny, J., and Dranoff, J. A. (2015). Establishment and characterization of rat portal myofibroblast cell lines. PLoS ONE 10:e0121161. doi: 10.1371/journal.pone.0121161
Gallai, M., Kovalszky, I., Knittel, T., Neubauer, K., Ambrust, T., and Ramadori, G. (1996). Expresion of extracellular matrix proteoglycans perlecan and decorin in carbon-tetrachloride-injured rat liver and in isolated liver cells. Am. J. Pathol. 148, 1463–1471.
Garner, A., and Alexander, R. A. (1986). Histochemistry of elastic and related fibres in the human eye in health and disease. Histochem. J. 18, 405–412. doi: 10.1007/BF01675332
Georges, P. C., Hui, J.-J., Gombos, Z., McCormick, M. E., Wang, A. Y., Uemura, M., et al. (2007). Increased stiffness of rat liver precedes matrix deposition: implications for fibrosis. Am. J. Physiol. Gastrointest. Liver Physiol. 293, G1147–G1154. doi: 10.1152/ajpgi.00032.2007
Gonnert, F. A., Kunisch, E., Gajda, M., Lambeck, S., Weber, M., Claus, R. A., et al. (2012). Hepatic fibrosis in a long-term murine model of sepsis. Shock 37, 399–407. doi: 10.1097/SHK.0b013e31824a670b
Green, E. M., Mansfield, J. C., Bell, J. S., and Winlove, C. P. (2014). The structure and micromechanics of elastic tissue. Interface Focus 4:20130058. doi: 10.1098/rsfs.2013.0058
Gressner, A. M., Krull, N., and Bachem, M. G. (1994). Regulation of proteoglycan expression in fibrotic liver and cultured fat-storing cells. Path. Res. Pract. 190, 864–882. doi: 10.1016/S0344-0338(11)80990-8
Guyot, C., Combe, C., and Desmouliére, A. (2006). The common bile duct ligation in rat: a relevant in vivo model to study the role of mechanical stress on cell and matrix behaviour. Histochem. Cell Biol. 126, 517–523. doi: 10.1007/s00418-006-0185-2
Hauck, M., Seres, I., Kiss, I., Saulnier, J., Mohacsi, A., Wallach, J., et al. (1995). Effects of synthesized elastin peptides on human leukocytes. Biochem. Mol. Biol. Int. 37, 45–55.
Hayashi, H., and Sakai, T. (2012). Biological significance of local TGF-β activation in liver diseases. Front. Physiol. Gastrointest. Sci. 3:12. doi: 10.3389/fphys.2012.00012
Herrera, B., Sánchez, A., and Fabregar, I. (2012). BMPS and liver: more questions than answers. Curr. Pharm. Design 18, 4114–4125. doi: 10.2174/138161212802430503
Hinek, A. (1996). Biological roles of the non-integrin elastin/laminin receptor. Biol. Chem. 377, 471–480.
Hinek, A., Pshezhetsky, A. V., von Itzstein, M., and Starcher, B. (2006). Lysosomal sialidase (neuraminidase-1) is targeted to the cell surface in a mutiprotein complex that facilitates elastic fiber assembly. J. Biol. Chem. 281, 3698–3710. doi: 10.1074/jbc.M508736200
Hirai, M., Horiguchi, M., Ohbayashi, T., Kita, T., Chien, K. R., and Nakamura, T. (2007). Latent TGF-β-binding protein 2 binds to DANCE/fibulin-5 and regulates elastic fiber assembly. EMBO J. 26, 3283–3295. doi: 10.1038/sj.emboj.7601768
Hirani, R., Hanssen, E., and Gibson, M. A. (2007). LTBP-2 specifically interacts with the amino- terminal region of fibrillin-1 and competes with LTBP-1 for binding to this microfibrillar protein. Matrix Biol. 26, 213–223. doi: 10.1016/j.matbio.2006.12.006
Høgåsen, K., Homann, C., Mollnes, T. E., Graudal, N., Hogasen, A. K. M., Hasselqvist, P., et al. (1996). Serum clusterin and vitronectin in alcoholic cirrhosis. Liver 16, 140–146. doi: 10.1111/j.1600-0676.1996.tb00719.x
Hohenemser, A. (1895). Ueber das Vorkommen von elastischern Fasern bei cirrhotischen Prozessen der Leber und Niere. Arch. Path. Anat. Physiol. Klin. Med. 140, 192–197. doi: 10.1007/BF02116132
Horiguchi, M., Inoue, T., Ohbayashi, T., Hirai, M., Noda, K., Marmorstein, L. Y., et al. (2009). Fibulin-4 conducts proper elastogenesis via interaction with cross-linking enzyme lysyl oxidase. Proc. Natl. Acad. Sci. U.S.A. 106, 19029–19034. doi: 10.1073/pnas.0908268106
Houghton, A. M., Quintero, P. A., Perkins, D. L., Kobayashi, D.K., Kelley, D. G., Marconcini, L. A., et al. (2006). Elastin fragments drive disease progression in a murine model of emphysema. J. Clin. Invest. 116, 753–759. doi: 10.1172/JCI25617
Hunzelmann, N., Nischt, R., Brenneisen, P., Eickert, A., and Krieg, T. (2001). Increased deposition of fibulin-2 in solar elastosis and its colocalization with elastic fibres. Br. J. Dermatol. 145, 217–222. doi: 10.1046/j.1365-2133.2001.04337.x
Ikeda, M., Naitoh, M., Kubota, H., Ishiko, T., Yoshikawa, K., Yamawaki, S., et al. (2009). Elastic fiber assembly is disrupted by excessive accumulation of chondroitin sulfate in the dermal fibrotic disease, keloid. Biochem. Biophys. Res. Commun. 390, 1221–1228. doi: 10.1016/j.bbrc.2009.10.125
Iwaisako, K., Jiang, C., Zhang, M., Cong, M., Moore-Morris, T. J., Park, T. J., et al. (2014). Origin of myofibroblasts in the fibrotic liver in mice. Proc. Natl. Acad. Sci. U.S.A. 111, E3297–E3305. doi: 10.1073/pnas.1400062111
Kanta, J., and Bartos, F. (1978). Elastin fibers formation in the liver of carbon tetrachloride-treated rats. Acta Hepato Gastroenterol. 25, 350–352.
Kanta, J., Dooley, S., Delvoux, B., Breuer, S., D'Amico, T., and Gressner, A. M. (2002). Tropoelastin expression is up-regulated during activation of hepatic stellate cells and in the livers of CCl4-cirrhotic rats. Liver 22, 220–227. doi: 10.1046/j.0106-9543.2002.01573.x
Karnik, S. K., Brooke, B. S., Bayes-Genis, A., Sorensen, L., Wythe, J. D., Schwartz, R. S., et al. (2003a). A critical role for elastin signaling in vascular morphogenesis and disease. Development 130, 411–423. doi: 10.1242/dev.00223
Karnik, S. K., Wythe, J. D., Sorensen, L., Brooke, B. S., Urness, L. D., and Li, D. Y. (2003b). Elastin induces myofibrillogenesis via a specific domain, VGVAPG. Matrix Biol. 22, 409–425. doi: 10.1016/S0945-053X(03)00076-3
Kasamatsu, S., Hachiya, A., Fujimura, T., Sriwiriyanont, P., Haketa, K., Visscher, M. O., et al. (2011). Essential role of microfibrillar-associated protein 4 in human cutaneous homeostasis and in its photoprotection. Sci. Rep. 1:164. doi: 10.1038/srep00164
Khan, S. A., Dong, H., Joyce, J., Sasaki, T., Chu, M.-L., and Tsuda, T. (2016). Fibulin-2 is essential for angiotensin II-induced myocardial fibrosis mediated by transforming growth factor (TGF)-β. Lab. Invest. 96, 773–783. doi: 10.1038/labinvest.2016.52
Kielty, C. M., Sherratt, M. J., and Shuttleworth, C. A. (2002). Elastic fibres. J. Cell Sci. 115, 2817–2828.
Kinnman, N., and Housset, C. (2002). Peribiliary myofibroblasts in biliary type liver fibrosis. Front. Biosci. 47, 496–503. doi: 10.2741/A790
Kinsey, R., Williamson, M. R., Chaudhry, S., Mellody, K. T., McGovern, A, Takahashi, S., et al. (2008). Fibrillin-1 microfibril deposition is dependent on fibronectin assembly. J. Cell Sci. 121, 2696–2704. doi: 10.1242/jcs.029819
Klaas, M., Kangur, T., Viil, J., Mäemets-Allas, K., Minajeva, A., Vadi, K., et al. (2016). The alterations in the extracellular matrix composition guide the repair of damaged liver tissue. Sci. Rep. 6:27398. doi: 10.1038/srep27398
Knittel, T., Kobold, D., Piscaglia, F., Saile, B., Neubauer, K., Mehde, M., et al. (1999). Localization of liver myofibroblasts and hepatic stellate cells in normal and diseased rat livers: distinct roles of (myo-)fibroblast subpopulations in hepatic tissue repair. Histochem. Cell Biol. 112, 387–401. doi: 10.1007/s004180050421
Kobayashi, N., Kostka, G., Garbe, J. H. O., Keene, D. R., Bächinger, H. P., Hanisch, F.-G., et al. (2007). A comparative analysis of the fibulin protein family. Biochemical characterization, binding interactions, and tissue localization. J. Biol. Chem. 282, 11805–11816. doi: 10.1074/jbc.M611029200
Koli, K., Saharinen, J., Hyytiäinen, M., Penttinen, C., and Keski-Oja, J. (2001). Latency, activation, and binding proteins of TGF-β. Microsc. Res. Tech. 52, 354–362. doi: 10.1002/1097-0029(20010215)52:4<354::AID-JEMT1020>3.0.CO;2-G
Kozel, B. A., Rongish, B. J., Czirok, A., Zach, J., Little, C. D., Davis, E. C., et al. (2006). Elastic fiber formation: a dynamic view of extracellular matrix assembly using timer reporters. J. Cell. Physiol. 207, 87–96. doi: 10.1002/jcp.20546
Lamireau, T., Dubuisson, L., Lepreux, S., Bioulac-Sage, P., Fabre, M., Rosenbaum, J., et al. (2002). Abnormal hepatic expression of fibrillin-1 in children with cholestasis. Am. J. Surg. Pathol. 26, 637–646. doi: 10.1097/00000478-200205000-00010
Lannoy, M., Slove, S., and Jacob, M.-P. (2014). The function of elastic fibers in the arteries: beyond elasticity. Pathol. Biol. 62, 79–83. doi: 10.1016/j.patbio.2014.02.011
Leeming, D. J., Karsdal, M. A., Byrjalsen, I., Bendtsen, F., Trebicka, J., Nielsen, M. J., et al. (2013). Novel serological neo-epitope markers of extracellular matrix proteins for the detection of portal hypertension. Aliment. Pharmacol. Ther. 38, 1086–1096. doi: 10.1111/apt.12484
Liban, E., and Ungar, H. (1959). Elastosis in fibrotic and cirrhotic processes of the liver. AMA Arch. Pathol. 68, 331–341.
Li, J., Zhang, Y., Kuruba, R., Gao, X., Gandhi, C. R., Xie, W., et al. (2011). Roles of microRNA-29a in the antifibrotic effect of farnesoid X receptor in hepatic stellate cells. Mol. Pharmacol. 80, 191–200. doi: 10.1124/mol.110.068247
Li, Z., Dranoff, J. A., Chan, E., Uemuira, M., Sévigny, J., and Wells, R. G. (2007). Transforming growth factor-β and substrate stiffness regulate portal fibroblast activation in culture. Hepatology 46, 1246–1256. doi: 10.1002/hep.21792
Liu, X., Zhao, Y., Gao, J., Pawlyk, B., Starcher, B., Spencer, J. A., et al. (2004). Elastic fiber homeostasis requires lysyl oxidase-like 1 protein. Nat. Genet. 36, 178–182. doi: 10.1038/ng1297
Lorena, D., Darby, I. A., Reinhardt, D. P., Sapin, V., Rosenbaum, J., and Desmouliére, A. (2004). Fibrillin-1 expression in normal and fibrotic rat liver and in cultured hepatic fibroblastic cells: modulation by mechanical stress and role in cell adhesion. Lab. Invest. 84, 203–212. doi: 10.1038/labinvest.3700023
Lua, I., Li, Y., Zagory, J. A., Wang, K. S., French, S. W., Sévigny, J., et al. (2016). Characterization of hepatic stellate cells, portal fibroblasts, and mesothelial cells in normal and fibrotic livers. J. Hepatol. 64, 1137–1146. doi: 10.1016/j.jhep.2016.01.010
Mangasser-Stephan, K., Gartung, C., Lahme, B., and Gressner, A. M. (2001). Expression of isoforms and splice variants of the latent transforming growth factor β binding protein (LTBP) in cultured human liver myofiobroblasts. Liver 21, 105–113. doi: 10.1034/j.1600-0676.2001.021002105.x
Mao, Y., and Schwarzbauer, J. E. (2005). Fibronectin fibrogenesis, a cell-mediated matrix assembly process. Matrix Biol. 24, 389–399. doi: 10.1016/j.matbio.2005.06.008
Massam-Wu, T., Chiu, M., Choudhury, R., Chaudhry, S. S., Baldwin, A. K., McGovern, A., et al. (2010). Assembly of fibrillin microfibrils governs extracellular deposition of latent TGF-β. J. Cell Sci. 123, 3006–3018. doi: 10.1242/jcs.073437
Maurice, P., Blaise, S., Gayral, S., Debelle, S., Laffargue, M., Hornebeck, W., et al. (2013). Elastin fragmentation and atherosclerosis progression: the elastokine concept. Trends Cardiovasc. Med. 23, 211–221. doi: 10.1016/j.tcm.2012.12.004
McLaughlin, P. J., Chen, Q., Horiguchi, M., Starcher, B. C., Stanton, J. B., Broekelmann, T. J., et al. (2006). Targeted disruption of fibulin-4 abolishes elastogenesis and causes perinatal lethality in mice. Mol. Cell. Biol. 26, 1700–1709. doi: 10.1128/MCB.26.5.1700-1709.2006
Mecham, R. P., and Gibson, M. A. (2015). The microfibril-associated glycoproteins (MAGPs) and the microfibrillar niche. Matrix Biol. 47, 13–35. doi: 10.1016/j.matbio.2015.05.003
Mederacke, I., Hsu, C. C., Troeger, J. S., Huebener, P., Mu, X., Dapito, D. H., et al. (2013). Fate-tracing reveals hepatic stellate cells as dominant contributors to liver fibrosis independent of its etiology. Nat. Commun. 4:2823. doi: 10.1038/ncomms3823
Meindl-Beinker, N. M., Matsuzaki, K., and Dooley, S. (2012). TGF-β signaling in onset and progression of hepatocellular carcinoma. Dig. Dis. 30, 514–523. doi: 10.1159/000341704
Mithieux, S. M., and Weiss, A. S. (2005). Elastin. Adv. Protein Chem. 70, 437–461. doi: 10.1016/j.jdermsci.2013.03.004
Mölleken, C., Sitek, B., Henkel, C., Poschmann, G., Sipos, B., Wiese, S., et al. (2009). Detection of novel biomarkers of liver cirrhosis by proteomic analysis. Hepatology 49, 1257–1266. doi: 10.1002/hep.22764
Munoz-Félix, J. M., González-Núnez, M., Martinez-Salgado, C., and López-Novoa, J. M. (2015). TGF-β/BMP proteins as therapeutic targets in renal fibrosis. Where have we arrived after 25 years of trials and tribulations? Pharmacol. Ther. 156, 44–58. doi: 10.1016/j.pharmthera.2015.10.003
Murphy-Ullrich, J. E., and Poczatek, M. (2000). Activation of latent TGF-β bythrombospondin-1: mechanisms and physiology. Cytokine Growth Factor Rev. 11, 59–69. doi: 10.1016/S1359-6101(99)00029-5
Myung, S. J., Yoon, J.-H., Gwak, G.-Y., Kim, W., Yang, J. I., Lee, S. H., et al. (2007). Bile acid-mediated thrombospondin-1 induction in hepatocytes leads to transforming growth factor-β-dependent hepatic cell activation. Biochem. Biophys. Res. Commun. 353, 1091–1096. doi: 10.1016/j.bbrc.2006.12.157
Nakayama, H., Itoh, H., Kunita, S., Kuroda, N., Hiroi, M., Matsuura, H., et al. (2008). Presence of perivenular elastic fibers in nonalcoholic steatohepatitis fibrosis stage III. Histol. Histopathol. 23, 407–409.
Nguyen, T.-U., Bashur, C. A., and Kishore, V. (2016). Impact of elastin incorporation into electrochemically aligned collagen fibers on mechanical properties and muscle cell phenotype. Biomed. Mater. 11:025008. doi: 10.1088/1748-6041/11/2/025008
Nielsen, M. J., Lehmann, J., Leeming, D. J., Schierwagen, R., Klein, S., Jansen, C., et al. (2015). Circulating elastin fragments are not affected by hepatic, renal and hemodynamic changes, but reflect survival in cirrhosis with TIPS. Dig. Dis. Sci. 60, 3456–3464. doi: 10.1007/s10620-015-3783-9
Ochieng, J., Warfield, P., Green-Jarvis, B., and Fentie, I. (1999). Galectin-3 regulates the adhesive interaction between breast carcinoma cells and elastin. J. Cell. Biochem. 75, 505–514.
Page, A., Paoli, P. P., Hill, S. J., Howarth, R., Wu, R., Kweon, S.-M., et al. (2015). Alcohol directly stimulates epigenetic modifications in hepatic stellate cells. J. Hepatol. 62, 388–397. doi: 10.1016/j.jhep.2014.09.033
Pellicoro, A., Aucott, R. L., Ramachandran, P., Robson, A. J., Fallowfield, J. A., Snowdon, V. K., et al. (2012). Elastin accumulation is regulated at the level of degradation by macrophage metalloelastase (MMP-12) during experimental liver fibrosis. Hepatology 55, 1965–1975. doi: 10.1002/hep.25567
Perepelyuk, M., Terajima, M., Wang, A. Y., Georges, P. C., Janmey, P. A., Yamauchi, M., et al. (2013). Hepatic stellate cells and portal fibroblasts are the major cellular sources of collagens and lysyl oxidases in normal liver and early after injury. Am. J. Physiol. Gastrointest. Liver Physiol. 304, G605–G614. doi: 10.1152/ajpgi.00222.2012
Pilecki, B., Holm, A. T., Schlosser, A., Moeller, J. B., Wohl, A. P., Zuk, A. V., et al. (2016). Characterization of microfibrillar-associated protein 4 (MFAP4) as a tropoelastin- and fibrillin-binding protein involved in elastic fiber formation. J. Biol. Chem. 291, 1103–1114. doi: 10.1074/jbc.M115.681775
Piscaglia, F., Dudás, J., Knittel, T., Di Rocco, P., Kobold, D., Saile, B., et al. (2009). Expression of ECM proteins fibulin-1 and -2 in acute and chronic liver disease and in cultured rat liver cells. Cell Tissue Res. 337, 449–462. doi: 10.1007/s00441-009-0823-9
Poiani, G. J., Tozzi, C. A., Yohn, S. E., Pierce, R. A., Belsky, S. A., Berg, R. A., et al. (1990). Collagen and elastin metabolism in hypertensive pulmonary arteries of rats. Circulation Res. 66, 968–978. doi: 10.1161/01.RES.66.4.968
Poon, S., Treweek, T. M., Wilson, M. R., Easterbrook-Smith, S. B., and Carver, J. A. (2002). Clusterin is an extracellular chaperone that specifically interacts with slowly aggregating proteins on their off-folding pathway. FEBS Lett. 513, 259–266. doi: 10.1016/S0014-5793(02)02326-8
Portmann, B., Tanner, M. S., Mowat, A. P., and Williams, R. (1978). Orcein-positive liver deposits in Indian childhood cirrhosis. Lancet 1, 1338–1340. doi: 10.1016/S0140-6736(78)92407-8
Porto, L. C., Chevallier, M., Guerret, S., Hartmann, D. J., and Grimaud, J.-A. (1990a). Elastin in alcoholic liver disease: an immunohistochemical and immunoelectron microscopic study. Path. Res. Pract. 186, 668–679. doi: 10.1016/S0344-0338(11)80232-3
Porto, L. C., Chevallier, M., Peyrol, S., Guerret, S., and Grimaud, J.-A. (1990b). Elastin in human, baboon, and mouse liver: an immunohistochemical and immunoelectron microscopic study. Anat. Rec. 228, 392–404. doi: 10.1002/ar.1092280405
Qin, Z. (2015). Soluble elastin peptides in cardiovascular homeostasis: foe or ally. Peptides 67, 64–73. doi: 10.1016/j.foodchem.2016.09.028
Raghunath, M., Bächi, T., Meuli, M., Altermatt, S., Gobet, R., Bruckner-Tuderman, L., et al. (1996). Fibrillin and elastin expression in skin regenerating from cultured keratinocyte autografts: morphogenesis of microfibrils begins at the dermo-epidermal junction and precedes elastic fiber formation. J. Invest. Dermatol. 106, 1090–1095. doi: 10.1111/1523-1747.ep12339373
Ramirez, F., and Sakai, L. Y. (2010). Biogenesis and function of fibrillin assemblies. Cell Tissue Res. 339, 71–82. doi: 10.1007/s00441-009-0822-x
Reddel, C. J., Cultrone, D., Rnjak-Kovacina, J., Weiss, A. S., and Burgess, J. K. (2013). Tropoelastin modulates TGF-β1-induced expression of VEGF and CTGF in airway smooth muscle cells. Matrix Biol. 32, 407–413. doi: 10.1016/j.matbio.2013.04.003
Redlich, M., Roos, H. A., Reichenberg, E., Zaks, B., Mussig, D., Baumert, U., et al. (2004). Expression of tropoelastin in human periodontal ligament fibroblasts after simulation of orthodontic force. Arch. Oral Biol. 49, 119–124. doi: 10.1016/j.archoralbio.2003.08.002
Reinboth, B., Hanssen, E., Cleary, E. G., and Gibson, M. A. (2002). Molecular interactions of biglycan and decorin with elastic fiber components. Biglycan forms a ternary complex with tropoelastin and microfibril-associated glycoprotein 1. J. Biol. Chem. 277, 3950–3957. doi: 10.1074/jbc.M109540200
Roark, E. F., Keene, D. R., Haudenschild, C. C., Godyna, S., Little, C. D., and Argraves, W. S. (1995). The association of human fibulin-1 with elastic fibers: an immunohistological, ultrastructural, and RNA study. J. Histochem. Cytochem. 43, 401–411. doi: 10.1177/43.4.7534784
Robert, L., and Labat-Robert, J. (2014). Circulating elastin peptides, role in vascular pathology. Pathol. Biol. 62, 337–341. doi: 10.1016/j.patbio.2014.05.020
Robertson, I. B., Horiguchi, M., Zilberberg, L., Dabovic, B., Hadjiolova, K., and Rifkin, D. B. (2015). Latent TGF-β-binding proteins. Matrix Biol. 47, 44–53. doi: 10.1016/j.matbio.2015.05.005
Robinet, A., Fahem, A., Cauchard, J.-H., Huet, E., Vincent, L., Lorimier, S., et al. (2005). Elastin-derived peptides enhance angiogenesis by promoting endothelial cell migration and tubulogenesis through upregulation of MT1-MMP. J. Cell Sci. 118, 343–356. doi: 10.1242/jcs.01613
Rock, M. J., Cain, S. A., Freeman, L. J., Morgan, A., Mellody, K., Marson, A., et al. (2004). Molecular basis of elastic fiber formation. Critical interactions and a tropoelastin-fibrillin-1 cross-link. J. Biol. Chem. 279, 23748–23758. doi: 10.1074/jbc.M400212200
Rodgers, U. R., and Weiss, A. S. (2004). Integrin αvβ3 binds a unique non-RGD site near the C-terminus of human tropoelastin. Biochimie 86, 173–178. doi: 10.1016/j.biochi.2004.03.002
Rosenbloom, J. (1984). Elastin: relation of protein and gene structure to disease. Lab. Invest. 51, 605–623.
Roskams, T., Rosenbaum, J., De Vos, R., David, G., and Desmet, V. (1996). Heparan sulfate proteoglycan expression in chronic cholestatic human liver diseases. Hepatology 24, 524–532. doi: 10.1002/hep.510240310
Rucker, R. B., Kosonen, T., Clegg, M. S., Mitchell, A. E., Rucker, B. R., Uriu-Hare, J. Y., et al. (1998). Copper, lysyl oxidase, and extracellular matrix protein cross-linking. Am. J. Clin. Nutr. 67(Suppl.), 996S–1002S.
Ryan, A. J., and O'Brien, F. J. (2015). Insoluble elastin reduces collagen scaffold stiffness, improves viscoelastic properties, and induces a contractile phenotype in smooth muscle cells. Biomaterials 73, 296–307. doi: 10.1016/j.biomaterials.2015.09.003
Sækmose, S. G., Mössner, B., Christensen, P. B., Lindvig, K., Schlosser, A., Holst, R., et al. (2015). Microfibrillar-associated protein 4: a potential biomarker for screening for liver fibrosis in a mixed patient cohort. PLoS ONE 10:e0140418. doi: 10.1371/journal.pone.0140418
Sækmose, S. G., Schlosser, A., Holst, R., Johansson, S. L., Wulf-Johansson, H., Tornøe, I., et al. (2013). Enzyme-linked immunosorbent assay characterization of basal variation and heritability of systemic microfibrillar-associated protein 4. PLoS ONE 8:e82383. doi: 10.1371/journal.pone.0082383
Sato, F., Wachi, H., Ishida, M., Nonaka, R., Onoue, S., Urban, Z., et al. (2007). Distinct steps of cross-linking, self-association, and maturation of tropoelastin are necessary for elastic fiber formation. J. Mol. Biol. 369, 841–851. doi: 10.1016/j.jmb.2007.03.060
Sato, Y., Ren, X. S., Harada, K., Sasaki, M., Morikawa, H., Shiomi, S., et al. (2011). Induction of elastin expression in vascular endothelial cells relates to hepatoportal sclerosis in idiopathic portal hypertension: possible link to serum anti-endothelial cell antibodies. Clin. Exp. Immunol. 167, 532–542. doi: 10.1111/j.1365-2249.2011.04530.x
Sato, Y., Sawada, S., and Nakanuma, Y. (2008). Fibulin-5 is involved in phlebosclerosis of major portal vein branches associated with elastic fiber deposition in idiopathic portal hypertension. Hepatol. Res. 38, 166–173. doi: 10.1111/j.1872-034X.2007.00253.x
Scheuer, P. J., and Maggi, G. (1980). Hepatic fibrosis and collapse: histological distinction by orcein staining. Histopathology 4, 487–490. doi: 10.1111/j.1365-2559.1980.tb02943.x
Sengle, G., Charbonneau, N. L., Ono, R. N., Sasaki, T., Alvarez, J., Keene, D. R., et al. (2008). Targeting of bone morphogenetic protein growth factor complexes to fibrillin. J. Biol. Chem. 283, 13874–13888. doi: 10.1074/jbc.M707820200
Shapiro, S. D., Endicott, S. K., Province, M. A., Pierce, J. A., and Campbell, E. J. (1991). Marked longevity of human lung parenchymal elastic fibers deduced from prevalence of D-aspartate and nuclear weapons-related radiocarbon. J. Clin. Invest. 87, 1828–1834. doi: 10.1172/JCI115204
Sherratt, M. J. (2009). Tissue elasticity and the ageing elastic fibre. AGE 31, 305–325. doi: 10.1007/s11357-009-9103-6
Shiratsuchi, E., Nakaba, M., and Yamada, M. (2016). Elastin hydrolysate derived from fish enhances proliferation of human skin fbroblasts and elastin synthesis in human skin fibroblasts and improves the skin conditions. J. Sci. Food Agric. 96, 1672–1677. doi: 10.1002/jsfa.7270
Siegel, R. C., Chen, K. H., Greenspan, J. S., and Aguiar, J. M. (1978). Biochemical and immunochemical study of lysyl oxidase in experimental hepatic fibrosis in the rat. Proc. Natl. Acad. Sci. U.S.A 75, 2945–2949. doi: 10.1073/pnas.75.6.2945
Silva, L. M., Fernandes, A. L. M., Barbosa, A. Jr., Oliveira, I. R., and Andrade, Z. A. (2000). Significance of schistosomal granuloma modulation. Mem. Inst. Oswaldo Cruz 95, 353–361. doi: 10.1590/S0074-02762000000300010
Sproul, E. P., and Argraves, W. S. (2013). A cytokine axis regulates elastin formation and degradation. Matrix Biol. 32, 86–94. doi: 10.1016/j.matbio.2012.11.004
Starcher, B., and Percival, S. (1985). Elastin turnover in the rat uterus. Connect Tisue Res 13, 207–215. doi: 10.3109/03008208509152400
Stone, P. J. (2000). Potential use of collagen and elastin degradation markers for monitoring liver fibrosis in schistosomiasis. Acta Trop. 77, 97–99. doi: 10.1016/S0001-706X(00)00118-2
Sugimoto, H., Yang, C., LeBleu, V. S., Soubasakos, M. A., Giraldo, M., Zeisberg, M., et al. (2007). BMP-7 functions as a novel hormone to facilitate liver regeneration. FASEB J. 21, 256–264. doi: 10.1096/fj.06-6837com
Sutcliffe, M. C., and Davidson, J. M. (1990). Effect of static stretching on elastin production by porcine aortic smooth muscle cells. Matrix 10, 148–153. doi: 10.1016/S0934-8832(11)80163-0
Tátrai, P., Egedi, K., Somorácz, A., van Kuppevelt, T. H., Ten Dam, G., Lyon, M., et al. (2010). Quantitative and qualitative alterations of heparan sulfate in fibrogenic liver diseases and hepatocellular cancer. J. Histochem. Cytochem. 58, 429–441. doi: 10.1369/jhc.2010.955161
Thung, S. N., and Gerber, M. A. (1982). The formation of elastic fibers in livers with massive hepatic necrosis. Arch. Pathol. Lab. Med. 106, 468–469.
Trask, B. C., Trask, T. M., Broekelmann, T., and Mecham, R. P. (2000). The microfibrillar proteins MAGP-1 and fibrillin-1 form a ternary complex with the chondroitin sulfate proteoglycan decorin. Mol. Biol. Cell 11, 1499–1507. doi: 10.1091/mbc.11.5.1499
Tsuruga, E., Yajima, T., and Irie, K. (2004). Induction of fibulin-5 gene is regulated by tropoelastin gene, and correlated with tropoelastin accumulation in vitro. Int. J. Biochem. Cell Biol. 36, 395–400. doi: 10.1016/S1357-2725(03)00238-3
Tsuruga, E., Yajima, T., and Irie, K. (2005). Microfibril-associated glycoprotein-1 and fibrilin-2 are associated with tropoelastin deposition in vitro. Int. J. Biochem. Cell Biol. 37, 120–129. doi: 10.1016/j.biocel.2004.06.002
Vadasz, Z., Kessler, O., Akiri, G., Gengrinovitch, S., Kagan, H. M., Baruch, Y., et al. (2005). Abnormal deposition of collagen around hepatocytes in Wilson's disease is associated with hepatocyte specific expression of lysyl oxidase and lysyl oxidase like protein-2. J. Hepatol. 43, 499–507. doi: 10.1016/j.jhep.2005.02.052
Velebný, V., Kasafírek, E., and Kanta, J. (1983). Desmosine and isodesmosine contents and elastase activity in normal and cirrhotic rat liver. Biochem. J. 214, 1023–1025. doi: 10.1042/bj2141023
Vrhovski, B., and Weiss, A. S. (1998). Biochemistry of tropoelastin. Eur. J. Biochem. 258, 1–18. doi: 10.1046/j.1432-1327.1998.2580001.x
Wachi, H., Seyama, Y., Yamashita, S., Suganami, H., Uemura, Y., Okamoto, K., et al. (1995). Stimulation of cell proliferation and autoregulation of elastin expression by elastin peptide VPGVG in cultured chick vascular smooth muscle cells. FEBS Lett. 368, 215–219. doi: 10.1016/0014-5793(95)00641-L
Wang, M.-C., Lu, Y., and Baldock, C. (2009). Fibrillin microfibrils: a key role for the interbead region in elasticity. Mol. Biol. 388, 168–179. doi: 10.1016/j.jmb.2009.02.062
Weinbaum, J. S., Broekelman, T. J., Pierce, R. A., Werneck, C. C., Segade, F., Craft, C. S., et al. (2008). Deficiency in microfibril-associated glycoprotein-1 leads to complex phenotypes in multiple organ systems. J. Biol. Chem. 283, 25533–25543. doi: 10.1074/jbc.M709962200
Wipff, P.-J., and Hinz, B. (2008). Integrins and the activation of latent transforming growth factor β1 – an intimate relationship. Eur. J. Cell Biol. 87, 601–615. doi: 10.1016/j.ejcb.2008.01.012
Wise, S. G., Yeo, G. C., Hiob, M. A., Rnjak-Kovacina, J., Kaplan, D. L., Ng, M. K., et al. (2014). Tropoelastin: a versaile, bioactive assembly module. Acta Biomater. 10, 1532–1541. doi: 10.1016/j.actbio.2013.08.003
Wulf-Johansson, H., Johansson, S. L., Schlosser, A., Holm, A. T., Rasmussen, L. M., Mickley, H., et al. (2013). Localization of microfibrillar-associated protein 4 (MFAP4) in human tissues: clinical evaluation of serum MFAP4 and its association with various cardiovascular conditions. PLoS ONE 8:e82243. doi: 10.1371/journal.pone.0082243
Yasui, Y., Abe, T., Kurosaki, M., Higuchi, M., Komiyama, Y., Yoshida, T., et al. (2016). Elastin fiber accumulation in liver correlates with the development of hepatocellular carcinoma. PLoS ONE 11:e0154558. doi: 10.1371/journal.pone.0160133
Yuan, Y., and Koria, P. (2016). Proliferative activity of elastin-like-peptides depends on charge and phase transition. J. Biomed. Mater. Res. 104, 697–706. doi: 10.1002/jbm.a.35609
Zanetti, M., Braghetta, P., Sabatelli, P., Mura, I., Doliana, R., Colombatti, A., et al. (2004). EMILIN-1 deficiency induces elastogenesis and vascular cell defects. Mol. Cell. Biol. 24, 638–650. doi: 10.1128/MCB.24.2.638-650.2004
Zhang, H., Apfelroth, S. D., Hu, W., Davis, E. C., Sanguinetti, C., Bonadio, J., et al. (1994). Structure and expression of fibrillin-2, a novel microfibrillar component preferentially located in elastic matrices. J. Cell Biol. 124, 855–863. doi: 10.1083/jcb.124.5.855
Keywords: elastic fibers, microfibrils, elastin, fibulin, hepatic stellate cells, portal fibroblasts, TGF-β, liver fibrosis
Citation: Kanta J (2016) Elastin in the Liver. Front. Physiol. 7:491. doi: 10.3389/fphys.2016.00491
Received: 05 August 2016; Accepted: 10 October 2016;
Published: 25 October 2016.
Edited by:
Ali Canbay, University Hospital Essen, GermanyReviewed by:
Katja Breitkopf-Heinlein, Medical Faculty Mannheim at Heidelberg University, GermanyPeri Kocabayoglu, University Hospital Essen, Germany
Copyright © 2016 Kanta. This is an open-access article distributed under the terms of the Creative Commons Attribution License (CC BY). The use, distribution or reproduction in other forums is permitted, provided the original author(s) or licensor are credited and that the original publication in this journal is cited, in accordance with accepted academic practice. No use, distribution or reproduction is permitted which does not comply with these terms.
*Correspondence: Jiří Kanta, a2FudGFAbGZoay5jdW5pLmN6