- 1Oncology Research Unit, Mexican Institute for Social Security, Mexico City, Mexico
- 2Biochemistry Sciences Program, Universidad Nacional Autónoma de Mexico, Mexico City, Mexico
- 3Departamento de Biología Molecular y Biotecnología, Instituto de Investigaciones Biomédicas, Universidad Nacional Autónoma de Mexico, Mexico City, Mexico
Lineage fate decisions of hematopoietic cells depend on intrinsic factors and extrinsic signals provided by the bone marrow microenvironment, where they reside. Abnormalities in composition and function of hematopoietic niches have been proposed as key contributors of acute lymphoblastic leukemia (ALL) progression. Our previous experimental findings strongly suggest that pro-inflammatory cues contribute to mesenchymal niche abnormalities that result in maintenance of ALL precursor cells at the expense of normal hematopoiesis. Here, we propose a molecular regulatory network interconnecting the major communication pathways between hematopoietic stem and progenitor cells (HSPCs) and mesenchymal stromal cells (MSCs) within the BM. Dynamical analysis of the network as a Boolean model reveals two stationary states that can be interpreted as the intercellular contact status. Furthermore, simulations describe the molecular patterns observed during experimental proliferation and activation. Importantly, our model predicts instability in the CXCR4/CXCL12 and VLA4/VCAM1 interactions following microenvironmental perturbation due by temporal signaling from Toll like receptors (TLRs) ligation. Therefore, aberrant expression of NF-κB induced by intrinsic or extrinsic factors may contribute to create a tumor microenvironment where a negative feedback loop inhibiting CXCR4/CXCL12 and VLA4/VCAM1 cellular communication axes allows for the maintenance of malignant cells.
Introduction
Cancer is currently considered as a global child health priority (Gupta et al., 2014). The application of effective treatments to decrease overall childhood cancer mortality requires a comprehensive understanding of its origins and pathobiology, along with accurate diagnosis and early identification of high-risk groups (reviewed in Vilchis-Ordoñez et al., 2016). Strikingly, the clinical, molecular and biological heterogeneity of malignant diseases indicating an unsuspected multiclonal diversity has highlighted their complexity and the uncertainty of their cell population dynamics. Novel theoretical and experimental integrative strategies have changed our perspective of cancer, from a hierarchical, deterministic and unidirectional process to a multi-factorial network where genetics interacts with micro and macro environmental cues that contribute to the etiology and maintenance of tumor cells (Notta et al., 2011; Davila-Velderrain et al., 2015; Tomasetti and Vogelstein, 2015). Furthermore, stochastic effects associated with the number of stem cell divisions have been proposed as major contributors, often even more significant than hereditary or external factors (Tomasetti and Vogelstein, 2015).
B-cell acute lymphoblastic leukemia (B-ALL) is largely the result of a growing number of cooperating genetic and epigenetic aberrations that corrupt hematopoietic developmental pathways and ultimate lead to uncontrolled production of malignant B lymphoid precursor cells within the bone marrow (BM) (Pelayo et al., 2012; Purizaca et al., 2012). Leukemic cell infiltration and treatment failure worsen the outcome of the disease and remain the foremost cause of relapse. Recent advances suggest the ability of leukemia initiating cells to create abnormal BM microenvironments, promoting high proliferation and early differentiation arrest at the expense of normal cell fate decisions (Colmone et al., 2008; Raaijmakers, 2011; Vilchis-Ordoñez et al., 2015). Intrinsic damage and/or remodeling of cell compartments that shape the distinct BM niches may account to microenvironmental regulation of quiescence, proliferation, differentiation and blastic cell migration. Leukemic cells compete for niche resources with their normal hematopoietic counterparts (Wu et al., 2009), culminating in the displacement of the latter, as observed in xenotransplantation mice models (Colmone et al., 2008). Moreover, the marrow microenvironment provides leukemic precursors with dynamic interactions and regulatory signals that are essential for their maintenance, proliferation and survival. Although, the underlying molecular mechanisms are poorly defined, these niches protect tumor cells from chemotherapy-induced apoptosis, showing a new perspective on the evolution of chemoresistance (Ayala et al., 2009: Shain et al., 2015; Tabe and Konopleva, 2015), and emphasizing the need for new models that theoretically or experimentally replicate the interplay between tumor and stromal cells under normal and pathological settings.
As suggested by our previous findings, ALL lymphoid precursors have the ability of responding to pathogen- or damage- associated molecular patterns via Toll-like receptor signaling by secreting soluble factors and altering their differentiation potentials (Dorantes-Acosta et al., 2013). The resulting pro-inflammatory microenvironment may expose them to prolonged proliferation, contributing tumor maintenance in a self-sustaining way while prompting the NF-κB-associated proliferation of normal progenitor cells (Vilchis-Ordoñez et al., 2015, 2016). Some hematopoietic growth factors and pro-inflammatory cytokines, including granulocyte-colony stimulating factor (G-CSF), IFNα, IL-1α, IL-1β, IL-7, and TNFα were highly produced by ALL cells from a conspicuous group of patients co-expressing myeloid markers (Vilchis-Ordoñez et al., 2015). Of note, mesenchymal stromal cells (MSCs) from ALL BM have shown atypical production of pro-inflammatory factors whereas disruption of the major cell communication pathway is apparent by detriment of CXCL12 expression and biological function (Geay et al., 2005; Colmone et al., 2008; van den Berk et al., 2014).
Considering that the CXCL12/CXCR4 axis constitutes the most critical component of the perivascular and reticular BM niches supporting the hematopoietic stem and progenitor cells (HSPCs) differentiation and maintenance within the BM, as well as the early steps of B cell development (Ma et al., 1998; Tokoyoda et al., 2004; Sugiyama et al., 2006; Greenbaum et al., 2013), an obstruction of the HSPC-MSC interaction may have substantial implications in the overall stability of these processes. Whether the inflammation-derived signals provide a mechanism for leukemic cells to survive, to induce changes in lineage cell fate decisions, or to prompt niche remodeling in leukemia settings, are currently topical questions.
Mathematical model strategies have become powerful approaches to complex biological systems and may contribute to unravel the hematopoietic-microenvironment interplay that facilitates tumor cells prevalence (Altrock et al., 2015; Enciso et al., 2015). Through continuous dynamic modeling with differential equations we have learned seminal aspects of multi-compartment and multi-clonal behavior of leukemic cell populations (Stiehl and Marciniak-Czochra, 2012; Enciso et al., 2015), leading to novel proposals on disease development driven by unbalanced competition between normal and pre-leukemic cells (Swaminathan et al., 2015). Both stochastic and deterministic models have been useful to simulate cell fate decisions and predict clonal evolution (reviewed in Enciso et al., 2015). Certainly, incorporating tumor microenvironment in cancer modeling is expected to change our vision of biochemical interactions in niche remodeling-dependent hematopoietic growth, as recently demonstrated for myeloma disease (Coelho et al., 2016).
By developing and simulating a dynamic Boolean system, we now investigate the biological consequences of microenvironmental perturbation due by temporal TLR signaling on crucial communication networks between stem/progenitor cells (HSPCs) and MSCs in ALL. We propose that NF-κB dependent tumor-associated inflammation co-participate in malignant progression concomitant to normal hematopoietic failure through disruption of CXCL12/CXCR4 and VLA4/VCAM-1 communication axes.
Materials and Methods
Manual Curation Strategy
Based on the crucial and unique role of the CXCL12/CXCR4 axis in the regulation of maintenance, biological activity, and niche communication-derived cell fate decisions of seminal cells, including pluripotent embryonic stem cells and multipotent hematopoietic stem cells, construction and updating of molecular interactions of relevance involved careful manual curation of primary hematopoietic cell research. Moreover, of special interest was the attention to the hematopoietic malignancies, which in contrast to solid tumors, display a distinct CXCL12-mediated microenvironmental behavior. Thus, although the modeled signaling pathways could be considered generic to all tissues, the organ, stage of cell differentiation and surrounding microenvironment may influence the net result of interactions. Taking into account this considerations, most published work that has been used for the reconstruction of our proposed model, include data from molecular interactions in HSPCs. Some of the interactions have been reported in a number of different tissues and predicted to be conserved in the hematopoietic system. Finally, as there is not enough data to model hematopoietic-microenvironment restricted to Homo sapiens and some interactions might be crucial for the molecular connectivity of the model, we have used information from different species when needed. A detailed referencing of all reports used for the model reconstruction is provided as Supplemental Material (Tables S1, S2, and reference list).
Molecular Basis for the Network Reconstruction
The connectivity among key molecules involved in the communication between HSPCs and MSCs within the BM was inferred through the curated experimental literature. Specifically, we were interested in recovering the network components, their interactions, and the nature of the interactions (activation/positive or inactivation/negative). The resulting general network incorporates transcriptional factors, kinases, membrane receptors, interleukines, integrins, growth factors, and chemokines from Homo sapiens and Mus musculus species. Importantly, to simplify the modeling process, some groups of molecules were considered as single functional modules, thus encompassing a series of sequential steps that lead to the activation or inactivation of a certain node (e.g., PI3K/Akt). The following paragraphs summarize the principle evidence used to reconstruct the HSPC-MSC network and infer the logical rules for computational simulation of the system as a discrete dynamical model. A detailed referencing is provided as Supplemental Material (Tables S1, S2, and reference list).
The CXCR4/CXCL12 chemokine pathway was considered as the central axis for the network construction considering its essential role in homeostasis maintenance (Sugiyama et al., 2006; Tzeng et al., 2011) and B lineage support (Ma et al., 1998; Tokoyoda et al., 2004). Furthermore, recent observations suggest that this axis is disrupted by up-stream molecular deregulations both in MSC and leukemic blasts harvested from ALL patients, affecting the maintenance of hematopoietic cells within their regulatory niches (Geay et al., 2005; Colmone et al., 2008; van den Berk et al., 2014). Besides the well-studied CXCR4/CXCL12 chemotactic interaction, CXCR4 activation increases the affinity between vascular cellular adhesion molecule-1 (VCAM-1) expressed on the surface of MSC and its receptor VLA-4 on HSPC. Both pathways, CXCR4/CXCL12 and VLA-4/VCAM-1, are known to play coordinately a central role in HSPC migration, engraftment and retention within the BM (Peled et al., 2000; Ramirez et al., 2009), converge in triggering the PI3K/Akt and ERK signals, and share common up-stream regulators involving molecular factors guiding inflammatory responses.
As mentioned in the Introduction, recent evidence indicates the secretion of high levels of pro-inflammatory cytokines by a conspicuous group of ALL patients (Vilchis-Ordoñez et al., 2015), thereby presumably contributing to remodeling of the normal hematopoietic microenvironment (Colmone et al., 2008). Of note, interleukin-1α (IL-1α) and IL-1β, which were substantially elevated, play an amplification role on inflammation increasing the expression of other cytokines, like G-CSF (Majumdar et al., 2000; Allakhverdi et al., 2013), and setting a positive feedback loop with the PI3K co-activation of NF-κB (Reddy et al., 1997; Sizemore et al., 1999; Carrero et al., 2012; Bektas et al., 2014). IL-1 and G-CSF, inhibit directly and indirectly the CXCR4/CXCL12 axis. G-CSF negatively regulates CXCL12 transcription and increases the secretion of matrix metalloproteinase-9, showing the ability to degrade both CXCL12 (Lévesque et al., 2003; Semerad et al., 2005; Christopher et al., 2009; Day et al., 2015) and CXCR4 (Lévesque et al., 2003). Moreover, G-CSF promotes up-regulation of Gfi1 that at the time inhibits the transcription of CXCR4 (Zhuang et al., 2006; De La Luz Sierra et al., 2007; de la Luz Sierra et al., 2010). Thus, by considering this information from experimental data, we have included IL-1 and G-CSF as key elements of the BM microenvironment in the HSPC-MSC communication network.
In concordance, we incorporated as a “positive control condition” an input node representing the Toll-like receptor ligand (lTLR) lipopolysaccharide (LPS), that binds TLR4 and triggers the conventional and well-known NF-κB-dependent pro-inflammatory response, promoting, among other transcriptional targets, the transcription of pro-IL-1β (Jones et al., 2001; Tak and Firestein, 2001; Wang et al., 2002; Khandanpour et al., 2010; Higashikuni et al., 2013).
Downstream NF-κB, the expression of CXCR7 has been shown to be upregulated (Tarnowski et al., 2010), which in turn, down-regulates CXCR4 by heterodimerization, promoting its internalization and further degradation. In parallel, activated CXCR7 presents a higher affinity for CXCL12 and β-arrestin, reducing CXCR4 signaling in CXCR7 and CXCR4 expressing cells (Uto-Konomi et al., 2013; Coggins et al., 2014). However, CXCR7 is unable to couple with G-protein, transducing through recruitment of β-arrestin and leading to MAP kinases Akt and ERK activation (Tarnowski et al., 2010; Uto-Konomi et al., 2013; Torossian et al., 2014). As with CXCR4, CXCR7, and VLA-4 activation in HSPC, PI3K/Akt pathway is activated on HSPC and MSC, via G-CSF receptor signaling (Liu et al., 2007; Vagima et al., 2009; Ponte et al., 2012; Furmento et al., 2014), and after LPS stimulation (Guha and Mackman, 2002; Wang et al., 2009; McGuire et al., 2013). Apparently, PI3K/Akt acts at overlapping levels on the modulation of inflammation. On the one hand, it increases the production of IL-1 antagonist molecules (Williams et al., 2004; Molnarfi et al., 2005; Li and Smith, 2014) and inhibits secretion of mature IL-1β (Tapia-Abellán et al., 2014). On the other hand, it promotes nuclear translocation of the transcriptional factor Foxo3a (Brunet et al., 1999; Miyamoto et al., 2008; Park et al., 2008), down-regulating indirectly the transcription of antioxidant enzymes and enabling reactive oxygen species (ROS) accumulation, which in turn promotes maturation of pro-IL-1β and IL-1β secretion (Hsu and Wen, 2002; Yang et al., 2007; Gabelloni et al., 2013).
At the mesenchymal counterpart, in addition to a number of molecules participating in the MSC-subsystem sensitivity to microenvironmental cues, we incorporated an input node representing Gap-junction conformed by connexin-43 (Cx43) that mediates direct intercellular communication between mesenchymal cells. Strikingly, its integral activity as calcium channel conductor has been shown to be a potent positive regulator of CXCL12 transcription and secretion (Schajnovitz et al., 2011). Furthermore, Cx43 expression appears to be critically disregulated in the BM stromal cells from acute leukemia patients, suggesting an important role in the hypothetic disregulation of the hematopoietic-stromal intercellular communication (Liu et al., 2010; Zhang et al., 2012). The inclusion of GSK3β and β-catenin in both subsystems was relevant due to their roles as intermediates of signaling transduction and regulation of the main intracellular communication elements proposed for our network reconstruction. The model is available in XML format (GINML) on GINsim Model Repository page (http://ginsim.org/models_repository) (Chaouiya et al., 2012), under the title “HSPCs-MSCs. Communication pathways between Hematopoietic Stem Progenitor Cells (HSPCs) and MSCs.”
Dynamical Modeling of the HSPC-MSC Network
For the computational modeling of the HSPC-MSC complex system, we followed the standard steps to convert it into a discrete dynamical system, as described by Albert and Wang (2009) and Assman and Albert (2009). The Boolean approach is useful when quantitative and detailed kinetic information is lacking. In such a case, each node of the network is represented as a binary element, allowed only to have an “active” (ON) or “inactive” (OFF) state, numerically represented by 1 and 0, respectively. The activation state of each node is dependent on the activation state of its regulators, as described by Boolean functions, also called logical rules. The classical Boolean operators employed in Boolean functions are AND (&), OR (|) and NOT (!). The AND operator is used to represent the requirement of the conjunction of two or more nodes participating in the regulation of a certain node (e.g., VLA-4 = CXCR4 & VCAM-1 representing that VLA-4 optimal activation requires its ligand VCAM-1 and the signaling due to CXCR4 activation). When there is more than one node able to regulate another, but only one of them is sufficient to exert the effect, the OR operator is applied (e.g., PI3K/Akt = GCSF | ROS | TLR representing that the activation of the G-CSF receptor, the increase of intracelular ROS concentration or the binding of a TLR ligand may activate PI3K/Akt signaling). Finally, the NOT operator represents repression of a node over another (e.g., IL-1 = (NF-κB & ROS) & !PI3K/Akt meaning that IL-1 requires the transcriptional activation of pro-IL-1 promoted by NF-κB and the post-transcriptional maturation mediated by ROS, but its signaling is inhibited by the presence of PI3K/Akt). Detailed compiling of reviewed references for the network reconstruction and the development of the logical rules can be found in Tables S1, S2.
Given that each node in the network has an activation state, then the general state of a network at a given time t can be represented by a vector of n elements, where n is the number of nodes in the network. For example, the vector (00000010000000000100001000), represents a network state where only the 7th, 18th, and 23rd elements are active. In our model, this particular state represents the pattern of activation where only GSK3B_H, GSK3B_M, and VCAM1_M are active. Now, since we are implementing a dynamical system, it is necessary to specify how the network may evolve from a time t to t+1.
There are two possible implementations to model the transition from one state of the network to another. On one side, the synchronous scheme update the activation state of all the nodes each time-step, assuming that all the biological processes involved in the model occur at similar time scales. And on the other side, asynchronous scheme update only one of the logical rules per time step, considering a more complex behavior of biological processes where molecular signaling is likely to change at different time points depending on the nature of the interaction (Albert and Wang, 2009). Either one or another update scheme, take an initial combination of the nodes (initial state) and update the logical rules successively through an established number of time steps or until an steady state or attractor is reached. Attractors may be of a single state (fixed point attractors) or a set of states (cyclic or complex attractors depending if they have one or more possible transition paths among their constituent states). The analysis of the nodes activation pattern in the attractors give the biological significance of the computational simulations of the models (Albert and Wang, 2009; Assman and Albert, 2009).
The dynamical behavior of the network was analyzed implementing the logical rules into BoolNet (R open-source package), and obtaining its attractors (stationary states) by applying asynchronous update strategies (Müssel et al., 2010). Under the asynchronous updating scheme, the simulation was performed using 50,000 random initial states, updating the network until either a fixed point attractor or a complex attractor was reached. Confidence of the model was tested through the simulation of all possible mutants (constitutive and null activation of every node) and the comparison of the resultant attractors with experimental reports about the biological effects in vivo or in vitro after the use of antagonists or the generation of knock-in and knock-out models.
Dynamical Multicellular Approach
Assuming that every simulation beginning at a certain initial state of the network represents the dynamical profile of a single cell, Wu and collaborators proposed a “population-like” analysis for a discrete model (Wu et al., 2009). Similarly, we asynchronously ran the simulations of the network from 50,000 random initial states, and then updated for 2000 time-steps, followed by calculation of the average activation value from 50,000 simulations for each node in each time-step. Such data was plotted as multi-cellular average activation graphs. Furthermore, we evaluated the effect of a short (1 time-step) and a sustained (699 time-steps) temporary induction of lTLR in time-step 700 and 1400, and analyzed the dynamical effects in the wild type network and in some relevant mutant networks.
Results
Network Reconstruction
The inferred HSPC-MSC network (Figure 1) constitutes the first attempt to model relevant interaction axes between undifferentiated hematopoietic cells and the BM microenvironment, that may approach us to a deeper understanding of the numerous molecular signals influencing the hematopoietic system regulation during normal and malignant processes. Our current ALL network has 26 nodes and 80 interactions. Among them, twelve nodes correspond to molecules that are expressed in HSPC and involved in intracellular signaling (PI3K/Akt, Gfi1, NF-κB, GSK3β, FoxO3a, ERK, β-catenin, and ROS) or cell-membrane receptors for communication with the microenvironment (CXCR4, CXCR7, VLA-4, and TLR). Eleven nodes conform the MSC subsystem, integrated by intracellular signaling molecules (PI3K/Akt, NF-κB, GSK3β, FoxO3a, ERK, β-catenin, and ROS), a gap-junction protein regulating communication among MSC (Cx43), communication ligands with HSPC (VCAM-1 and CXCL12) and TLR. Common internal nodes in both HSPC and MSC systems are representative molecules from the most studied pathways influencing proliferation, migration, survival, and -some of them- differentiation. Finally, the microenvironmental compartment is represented by G-CSF secreted by myeloid and stromal cells (Majumdar et al., 2000; Allakhverdi et al., 2013; Tesio et al., 2013; Boettcher et al., 2014), its inductor IL-1 which is secreted by MSC and HSPC, and lTLR so as to model a homeostasis disruption that is known to drive a pro-inflammatory signaling. Model inputs are Cx43 and lTLR, while the activation value of the other 24 nodes is dependent on the network topology and the initial state of the input nodes. All logical rules used for the computational simulation with BoolNet are shown in Table 1. Note that the logical rules for the input nodes include self-regulations, but these are for computational purposes to represent their sustained activation, rather than a biological reality.
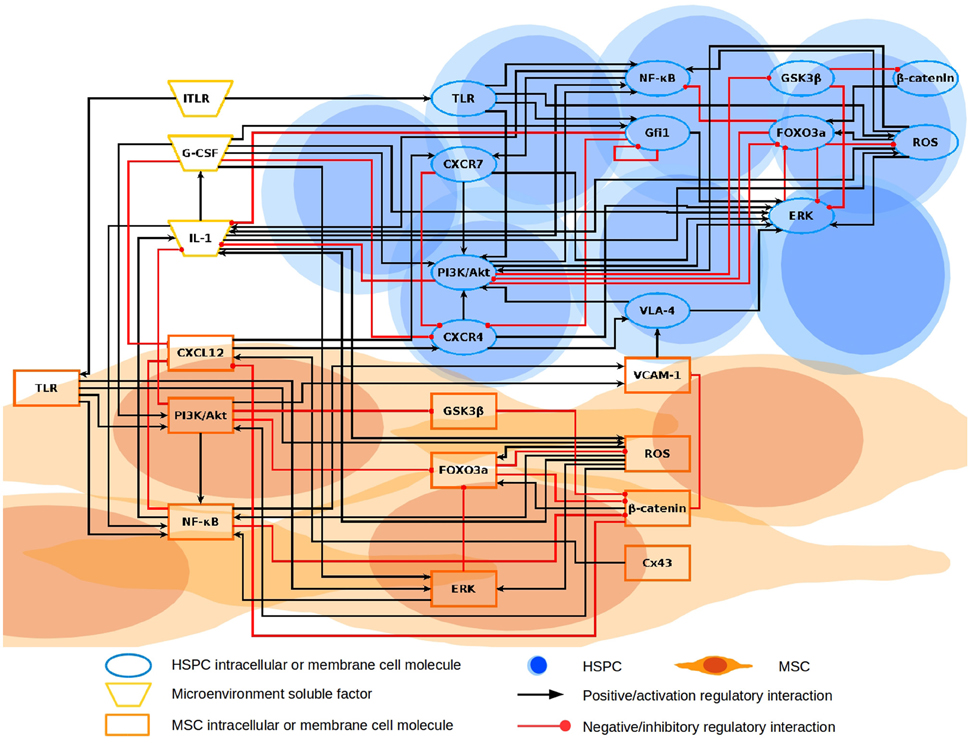
Figure 1. Regulatory HSPC-MSC network. The network is constituted by three compartments represented with different geometric shapes: HSPC, MSC, and microenvironmental soluble factors. HSPC and MSC have intracellular nodes regulating the response and expression of elements mediating the communication between them. CXCR4-CXCL12 and VLA-4/VCAM-1 axes are suggested to be the most crucial communicating elements. HSPC and MSC are both susceptible of TLR stimulation with lTLR input. HSPC, hematopoietic stem and progenitor cell; MSC, mesenchymal stromal cell.
Attractors of the Wild-Type Network: Searching for the Relevance of TLR in the Biology of CXCL12
The asynchronous simulation of the Boolean model returned 4 attractors: 2 fixed points and 2 complex attractors (Figure 2). The first two attractors, fixed point attractor 1 and 2, were identified with the physiological detached and attached state of the HSPC with its MSC counterpart, respectively.
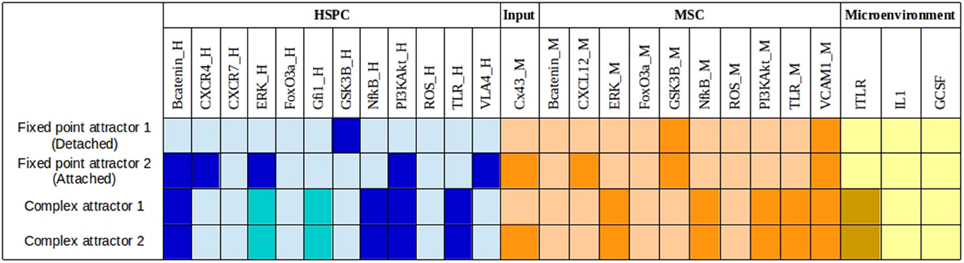
Figure 2. Asynchronous attractors from the wild type network. Dark color denotes an activation value of 1, while light color represents an activation value = 0. The blue, orange, and yellow colors distinguish the nodes in the three compartments in the HSPC-MSC network corresponding to HSPC, MSC, and microenvironmental factors, respectively. The last two attractors obtained when the initial states for the asynchronous simulation had lTLR value = 1, have two nodes (ERK_H and Gfi1_H) whose activation values oscillate and are responsible of the complex attractor. Oscillatory values are represented by intermediate blue color. Nodes representing molecules in HSPC are denoted with “_H” at the end of the node name, while nodes representing molecules in MSC are denoted with “_M.”
Both fixed point attractors will depend on the initial states of both, TLR and Cx43. Thus, in the absence of lTLR, the final fates will depend on the initial activation state of Cx43. However, once TLR is activated, final fates are not contributed anymore from the activation state of Cx43.
Loss of HSPC-MSC communication corresponding to a detachment state, is due to the absence of Cx43 and the consequent inactivation of CXCL12. In the activation pattern of this attractor, only VCAM-1 accompanied by GSK3β in both sub systems remained active (Tabe et al., 2007). On the contrary, when Cx43 is active (as in fixed point attractor 2), CXL12 is expressed by the MSC, which in turn positively regulates the CXCR4 receptor required for the activation of the VLA-4/VCAM-1 axis. The pattern in HSPC, correspond to ERK and PI3K/Akt activation, well-described elements downstream CXCR4 and VLA-4 (Tabe et al., 2007). β-catenin, a subject of debate about its function on stem cell maintenance, is turned on as a consequence of the GSK3β inhibition by PI3K/Akt (Dao et al., 2007).
Complex attractors 1 and 2 share the same activation values in all nodes, except for the initial state of Cx43 which is an input and therefore may be consistently either active or inactive through simulation. Importantly, these two attractors have the node for ITLR active, so that under induced pro-inflammatory conditions the resultant perturbation of CXCR4/CXCL12 and VLA-4/VCAM-1 is exclusively dependent on CXCL12 down regulation in MSC by NF-κB. The network attractors are concordant with experimental observations (Ueda et al., 2004; Wang et al., 2012; Yi et al., 2012) with the exception of IL1 and GCSF inactivation although lTLR-induced NF-κB signaling in hematopoietic and mesenchymal compartments. In order to explain this discrepancy we may remark that an attractor is a stable network state or set of states, reached after the network went through a sequence of transient states where, in most biological systems, there is cross-pathway communication for modulating cellular response (Williams et al., 2004; Tapia-Abellán et al., 2014), so IL1 and GCSF could be activated in some transient states but down-regulated by other pathways responding to lTLR activation. Due to the existence of regulatory circuits among pathways, in the presence of ITLR there is an oscillatory behavior of ERK and Gfi1. Therefore, we applied the dynamic multicellular approach described by Wu et al. (2009) in order to have a deeper understanding of the HSPC-MSC model upon perturbations. The average activation value of 50,000 simulations for all nodes within the HSPC-MSC network was plotted and presented in Figure 3. The plots represent a qualitative approach for the analysis of the cell population trend under specific conditions. Considering that the initial activation values are randomly chosen, with exception of lTLR, TLR_M, and TLR_H which activation value was set to 0, the average initial activation value for the rest of the nodes correspond to 0.5. From time-step 0 to time-step 499 correspond to the stabilization of the dynamics. Of note, the plateau obtained around time-steps 500-699 corresponds to the average of the two fixed point attractors.
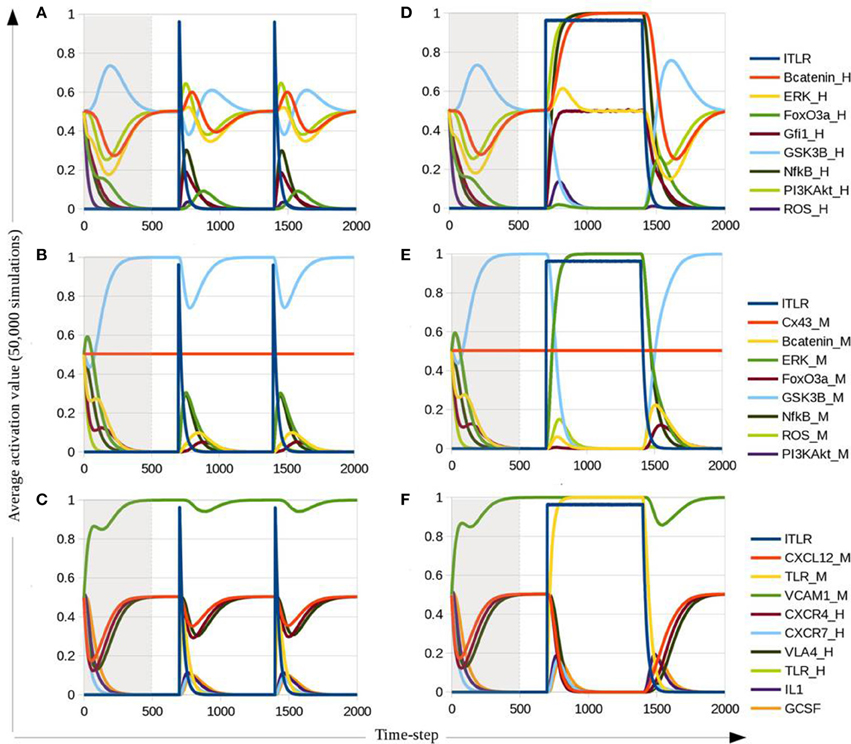
Figure 3. Average activation value for intracellular HSPC nodes (A,D), intracellular MSC nodes (B,E) and communication axes among HSPC, MSC, and microenvironment (C,F). (A–C) Correspond to simulations with a short (1 time-step) stimulation of lTLR at time-steps 700 and 1400. (D–F) Correspond to simulations with lTLR stimulation at time-step 700 with a length of 699 time-steps. Nodes representing molecules in HSPC are denoted with “_H” at the end of the node name, and nodes representing molecules in MSC are denoted with “_M.” Gray area covers the stabilization time steps until attractors are reached.
Analysis of Transitory States Applied to a Multicellular Approach: from Pro-inflammatory Signals to CXCL12 Downregulation
The short lTLR stimulation at time-step 700 and 1400 (Figures 3A–C) induces up-regulation of Gfi1 in HSPC (Figure 3A), and of NF-κB and PI3K/Akt in both HSPC and MSC compartments (Figures 3A,B). These nodes maintain a sustained activation as long as the lTLR is present (Figures 3D–F). In contrast, ERK, ROS and FoxO3a showed an increase but are regulated by other nodes, providing a feedback to basal values. Accompanying the cross-regulation of intracellular pathways, a decrease on CXCR4, CXCL12, VLA-4, and VCAM-1 activation is observed. As expected, there is positive signaling of the pro-inflammatory cytokines with a parallel co-increase of CXCR7, signals damped by PI3K/Akt and CXCL12 down-regulation, respectively.
Model Validation by Mutant Analysis
Listed in Table 2 are the observations from comparisons between the resultant attractors of simulations with null (“loss of function”) and constitutive expression mutants (“gain of function”), against the wild-type model. We focused on the activation value changes in the two axes of interest –CXCR4/CXCL12 and VCAM-1/VLA-4. Even though the nodes included in the reconstruction of the present model are well-studied elements of cell fate related-pathways, there is a lack of experiments correlating their perturbation with microenvironment modifications that impact HSPC behavior (Table 2, Table S4). Due to this missing data, and in order to validate the model, we now used available information of general alterations in hematopoiesis in the presence of lTLR.
MSC ERK, FoxO3a, and PI3K/Akt nodes participating in CXCR4/CXCL12 and VCAM-1 VLA-4 axes regulation were not found in the revised literature. β-catenin in MSC has a role on osteoblastogenesis and its constitutive induced expression in osteoblasts in a mice model results in acute myeloid leukemia (AML) induction (Kode et al., 2014). The constitutive expression of β-catenin showed an outcome where, under non-induced inflammation, the CXCR4/CXCL12 axis is disrupted. This gives support to our hypothesis that CXCR4/CXCL12 is probably involved in the maintenance of leukemic cells. Furthermore, the dynamic multicellular approach in the gain of function of β-catenin in MSC, reproduced the recovery of VCAM-1 expression upon stimulation of lTLR as reported by Kincade in OP9 cells (Figure S1; Malhotra and Kincade, 2009).
GSK3β inhibition in MSC has been known to function in the regulation of osteoblast and adipocyte differentiation. Besides, experimental effect of a GSK3β-inhibitor on osteoblastogenesis has shown that the decrease of this kinase induces down-regulation of CXCL12 expression (Satija et al., 2013). The model is consistent with the unsteadiness of CXCL12 activation in the simulation of the mutant (Figures S2A,B).
According to our hypothesis, a pro-inflammatory-induced CXCR4/CXCL12 disruption results in leukemic progression support. In the proposed model, overexpression of NF-κB disrupts the HSPC-MSC communication (Figure S2C). This is in agreement with the reported leukocytosis associated to up-regulation of NF-κB within BM MSCs from a mice model of high-fat diet (Cortez et al., 2013). Finally, modeling of a gain of function mutation in ROS resulted in the blocking of CXCL12 activation (Figure S2D). This is also in accordance of the recent report of oxidative damage induced by iron in MSC, resulting in down-regulation of CXCL12 expression and reduction of their hematopoietic supporting function (Zhang et al., 2015). Moreover, the iron-induced hematopoietic alterations previously observed by other groups, are attenuated by the treatment with ROS inhibitors (Lu et al., 2013).
Nodes in HSPC which have been experimentally reported as dispensable for hematopoiesis, which did not show any alterations in the CXCR4/CXCL12 and VLA-4/VCAM-1 axes on the mutant simulations, are β-catenin (Figures S3A–D; Cobas et al., 2004; Jeannet et al., 2008) and CXCR7 (Figures S3E,F). However, even though in vivo β-catenin null mutant HSPC does not lose long-term reconstitution capacity or multipotentiallity, its overexpression produces lose of stemness and differentiation blockage to erythroid and lymphoid lineages (Kirstetter et al., 2006; Scheller et al., 2006). Simulations of the gain of function of β-catenin resulted in the appearance of additional attractors where FoxO3a and GSK3β are increased (Figures S4A,B, S5B), suggesting a reduction in proliferation and/or apoptosis induction (Maurer et al., 2006; Yamazaki et al., 2006). In turn, the simulation of overexpression of FoxO3a showed a down-regulation of ERK and PI3K (Figures S4C, S5C). Also reported as proliferative repressors in HSPC (Hock et al., 2004; Zeng et al., 2004; Holmes et al., 2008), Gfi1 and GSK3β overexpression mutants inhibited ERK activation, and additionally Gfi1 induce the downregulation of PI3K/Akt node, CXCR4/CXCL12 and VLA-4/VCAM-1 axes (Figures S4 and S5). Disagreeing with experimental data (Holmes et al., 2008), GSK3β null mutant outcome result in an additional attractor where PI3K/Akt and ERK are inactive, notwithstanding CXCR4 and VLA4 activation (Figure S6).
Of interest, NF-κB (Figure 4) and ROS (Figures S4F, S5F) constitutive expression in HSPC induce additional attractors with activation of IL-1 and G-CSF, and inhibition of axes regulating HSPC-MSC contact. A number of investigations on cancer cells report a correlation of NF-κB increased levels and CXCR4 (Richmond, 2002; Ayala et al., 2009; Shin et al., 2014). Nonetheless, a recent study in human leukemic cell lines has shown that LPS treatment increases MMP-9 activity, a metalloproteinase known to efficiently degrade CXCR4 and CXCL12 (Hajighasemi and Gheini, 2015).
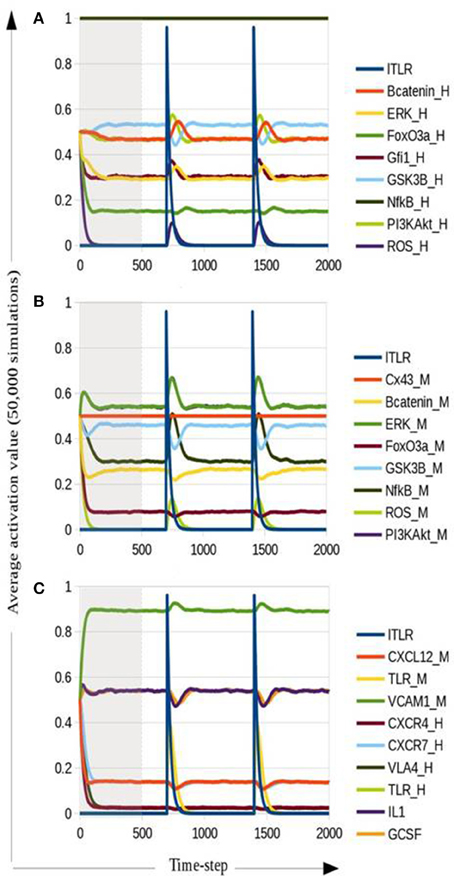
Figure 4. Dynamic multicellular simulation for a ALL simplified model addressed by NF-κB gain of function in HSPC. Average activation for intracellular HSPC nodes (A), intracellular MSC nodes (B) and communication axes among HSPC, MSC, and microenvironment (C). Nodes representing molecules in HSPC are denoted with “_H” at the end of the node name, while nodes representing molecules in MSC are denoted with “_M.” Gray area covers the stabilization time steps until attractors are reached.
NF-κB Gain of Function Mutant as ALL Simplified Model
How common alterations in ALL cells may induce BM microenvironment remodeling, regardless of the underlying genetic aberration, was investigated by running a dynamic multicellular simulation using the mutant network for NF-κB gain of function within the HSPC sub-system. The results shown in Figure 4 confirm that NF-κB mutation in HSPC may perturb HSPC-MSC communication in parallel with the induction of other alterations previously reported in ALL cells, such as the increase of Gfi1 expression (Purizaca et al., 2013) and a pro-inflammatory milieu (Vilchis-Ordoñez et al., 2015). IL1 and G-CSF activation by HSPC up-regulate ERK, NF-κB and PI3K/Akt in MSC. As consequence of PI3K/Akt increase in MSC, β-catenin is up-regulated through the inhibition of GSK3β. Strikingly, the sustained activation of CXCR7 resulted as a consequence of NF-κB constitutive expression in HSPC and CXCL12 residual expression from MSC. CXCR7/CXCL12 axis was recently reported to be increased in ALL cells and a possible participation in abnormal cell migration was suggested (Melo et al., 2014).
Discussion
According to the classical model of hematopoiesis, normal blood cells are replenished throughout life by stem and early progenitor populations undergoing stepwise differentiation processes in the context of intersinusoidal specialized niches (Purizaca et al., 2012; Vadillo et al., 2013). Cell cycle status, self-renewing capability and the central cell fate decisions depend, in great part, on the microanatomic organization and signals from the BM environment. Endosteal, perivascular and reticular niches provide support by cell-cell interactions and growth/differentiation factors that control the expression of lineage-specific transcription factors, among other elements. Within the reticular niche, mainly composed by CXCL12-abundant reticular cells (CARs), a special category of MSCs, the chemokine CXCL12 and its receptor CXCR4 play a pivotal role in the regulation of lymphopoiesis from the earliest stages of the pathway (Tokoyoda et al., 2004; Nagasawa, 2015). The transcription factor Foxc1 governs CXCL12 and stem cell factor expression, allowing the CAR niche formation for maintenance of HSC, common lymphoid progenitors, B cells, NK and plasmacytoid dendritic cells (Omatsu et al., 2014). The net balance of its disruption is instability of adaptive and innate immune cell production. Recent findings suggest that elevation of cytokines and growth factors, including G-CSF and TNFα, due to infectious stress, substantially reduce the expression of CXCL12, SCF and VCAM-1, further impairing primitive cell maintenance and prompting their proliferation and migration (Kobayashi et al., 2015, 2016).
Much remains to be unraveled about CXCL12-related mechanisms of intercommunication damage that may favor growth of cancer cells at the expense of healthy hematopoiesis during biological contingencies such as hematological malignancies and biological stress. Although, genetic heterogeneity may be co-responsible for differences in ALL overall survival, response to treatment, differentiation-stage arrest or even predisposition to metastasis, a common need might be the development of biological features that provide pre-malignant cells decisive advantage over normal cells to compete for the same ecological niche. Given the importance of CXCR4/CXCL12 axis for homeostatic hematopoiesis and of its presumptive disruption in ALL BM, we now propose a Boolean model reconstructed with some of the most studied elements upstream and downstream this key communication axis. Our model shows its capacity to simulate several phenotypes relevant to ALL. According to previous experimental research, the major assumption made from this model is that the integrity of CXCR4/CXCL12 signaling, promoting the required activation of the VLA-4/VCAM-1 integrins interaction, is absolutely necessary for HSPC retention in the mesenchymal niche and in consequence, indispensable for optimal hematopoiesis regulation (Lévesque et al., 2003; Lua et al., 2012; Greenbaum et al., 2013; Park et al., 2013). The HSPC-MSC model asynchronous simulation in the absence of lTLR returned two attractors corresponding to HSPC attachment and detachment to MSC. The ‘attachment’ status, represented by the induction of CXCR4/CXCL12 and/or VLA-4/VCAM-1 axes, also exhibited PI3K/Akt and β-catenin activation within the HSPC compartment. Although there is some controversy about the β-catenin role in HSC regulation (Kirstetter et al., 2006; Duinhouwer et al., 2015), the co-activation of PI3K/Akt and β-catenin is known to promote self-renewal and HSC expansion (Perry et al., 2011). Two core pathways downstream CXCR4/CXCL12 binding are PI3K/Akt and ERK, both promoters of cell survival and regulators of proliferation. Considering that the mesenchymal stromal niche has being identified as the interface between the quiescence promoting osteoblastic niche and the vascular niche regulating final lineage commitment and cell migration, the signals provided by mesenchymal cells should tightly regulate proliferation/expansion in order to further allow differentiation. According to this statement, the attractor representing the detached state conducts to pro-apoptosis signaling in the absence of aberrant expression of NF-κB, that relies on cytochrome C release-associated normal functions of GSK3β in HSPC (Maurer et al., 2006).
By using elegant mice disease models and controlled culture systems, a wealth body of studies has recently highlighted the co-participation of inflammation and infectious stress in the HSPC exit from quiescence status, as well as in cancer etiology and progression (Baldridge et al., 2011; Vilchis-Ordoñez et al., 2015). Chronic inflammation and carcinogenesis have been closely connected via either a oncogenes-derived intrinsic pathway or through an extrinsic pathway from external factors that promote latent inflammatory responses involving signaling pathways such as MyD88, NF-κB, and STAT3 (Mantovani et al., 2008; Krawczyk et al., 2014).
Interestingly, pattern recognition receptors (PRRs), including Toll-like receptors (TLRs) are functionally expressed from the most primitive stages of hematopoiesis and contribute to emergent cell replenishment in response to life-threatening infections or disease-associated cell damage (Nagai et al., 2006; Welner et al., 2008; Dorantes-Acosta et al., 2013; Vadillo et al., 2014). This phenomenon is called emergency hematopoiesis and is regulated at the most primitive cell level (Kobayashi et al., 2015, 2016).
The potential relevance of this mechanism in leukemogenesis was the focus of this investigation, and our model allowed for the analysis of most behaviors observed under experimental settings. The discrete simulation of NF-κB constitutive expression mutant on HSPC, gave further support to our hypothesis on the perturbation of CXCR4/CXCL12 communication axis induced by pro-inflammatory microenvironment. The single mutation of NF-κB was sufficient to remodel the dynamical behavior of the three sub-systems represented, which was an unexpected behavior of the model. The dynamic analysis of the ALL-like network, also suggested the activation of an alternative communication pathway mediated by CXCR7 binding CXCL12. Inhibition of CXCL12 within the mesenchymal niche, may be fundamental for cell migration to adjacent BM structures unable to sustain proper differentiation or even to extramedullar tissues, accounting for a predictable role of this axis in metastasis.
Concluding Remarks
The proposed HSPC-MSC model is the first systemic approximation to understand the intercommunication pathways underlying primitive cell retention/proliferation in the mesenchymal niche as a determinant factor for progression of hematological hyperproliferative diseases. We applied conventional discrete dynamical modeling and non-conventional population-like approaches as an average behavior of the network model. Future improvement of discrete dynamical modeling for ALL system will provide a powerful tool for investigation of unbalanced competitions between leukemic and normal hematopoietic cells within the BM. Overall, systems biology will advance our comprehensive view of the mechanisms involved in the pathogenesis of leukemic niches that may illuminate therapeutic strategies based on cell-to-cell crosstalk manipulation.
Author Contributions
JE designed the work; generated, analyzed and interpreted data; wrote the paper. HM interpreted data; revised the work for intellectual content; wrote the paper. LM designed the work; interpreted data; revised the work for intellectual content; wrote the paper. RP designed the work; interpreted data; revised the work for intellectual content; wrote the paper.
Conflict of Interest Statement
The authors declare that the research was conducted in the absence of any commercial or financial relationships that could be construed as a potential conflict of interest.
Acknowledgments
This work was supported by the National Council of Science and Technology (CONACyT) (Grant CB-2010-01-152695 to RP), by the Mexican Institute for Social Security (IMSS) (Grant FIS/IMSS/PROT/G14/1289 to RP) and by the “Red Temática de Células Troncales y Medicina Regenerativa” from CONACyT. LM acknowledges the sabbatical scholarships from PASPA-DAPA UNAM and CONACyT 251420. JE is scholarship holder from CONACyT and IMSS, and was awarded by the PRODESI IMSS Program.
Supplementary Material
The Supplementary Material for this article can be found online at: http://journal.frontiersin.org/article/10.3389/fphys.2016.00349
References
Albert, R., and Wang, R. S. (2009). Discrete dynamic modeling of cellular signaling networks. Methods Enzymol. 467, 281–306. doi: 10.1016/S0076-6879(09)67011-7
Allakhverdi, Z., Comeau, M. R., Armant, M., Agrawal, R., Woodfolk, J. A, Sehmi, R., et al. (2013). Mast cell-activated bone marrow mesenchymal stromal cells regulate proliferation and lineage commitment of CD34+ progenitor cells. Front. Immunol. 4:461. doi: 10.3389/fimmu.2013.00461
Altrock, P. M., Liu, L. L., and Michor, F. (2015). The mathematics of cancer : integrating quantitative models. Nat. Publ. Gr. 15, 730–745. doi: 10.1038/nrc4029
Assman, S. M., and Albert, R. (2009). Discrete dynamic modelling with asynchronous update or, how to model complex systems in the absence of quantitative information. Methods Mol. Biol. 553, 207–225. doi: 10.1007/1-4020-0613-6_16551
Ayala, F., Dewar, R., Kieran, M., and Kalluri, R. (2009). Contribution of bone microenvironment to leukemogenesis and leukemia progression. Leukemia 23, 2233–2241. doi: 10.1038/leu.2009.175
Baldridge, M. T., King, K. Y., and Goodell, M. A. (2011). Inflammatory signals regulate hematopoietic stem cells. Trends Immunol. 32, 57–65. doi: 10.1016/j.it.2010.12.003.Inflammatory
Bektas, A., Zhang, Y., Lehmann, E., Wood. W. H. III, Becker, K. G., Ferrucci, L., et al. (2014). Age-associated changes in basal NF-κB function in human CD4+ T lymphocytes via dysregulation of PI3 kinase. Aging (Albany. NY). 6, 957–974. doi: 10.18632/aging.100705
Boettcher, S., Gerosa, R. C., Radpour, R., Bauer, J., Ampenberger, F., Heikenwalder, M., et al. (2014). Endothelial cells translate pathogen signals into G-CSF-driven emergency granulopoiesis. Blood 124, 1393–1403. doi: 10.1182/blood-2014-04-570762
Brunet, A., Bonni, A., Zigmond, M. J., Lin, M. Z., Juo, P., Hu, L. S., et al. (1999). Akt promotes cell survival by phosphorylating and inhibiting a forkhead transcription factor. Cell 96, 857–868.
Carrero, R., Cerrada, I., Lledó, E., Dopazo, J., García-García, F., Rubio, M.-P., et al. (2012). IL1β induces mesenchymal stem cells migration and leucocyte chemotaxis through NF-κB. Stem Cell Rev. 8, 905–916. doi: 10.1007/s12015-012-9364-9
Champelovier, P., El Atifi, M., Pautre, V., Rostaing, B., Berger, F., and Seigneurin, D. (2008). Specific inhibition of basal mitogen-activated protein kinases and phosphatidylinositol 3 kinase activities in leukemia cells: a possible therapeutic role for the kinase inhibitors. Exp. Hematol. 36, 28–36. doi: 10.1016/j.exphem.2007.08.027
Chaouiya, C., Naldi, A., and Thieffry, D. (2012). Logical modelling of gene regulatory networks with GINsim. Methods Mol. Biol. 804, 463–479. doi: 10.1007/978-1-61779-361-5_23
Christopher, M. J., Liu, F., Hilton, M. J., Long, F., and Link, D. C. (2009). Suppression of CXCL12 production by bone marrow osteoblasts is a common and critical pathway for cytokine-induced mobilization. Blood 114, 1331–1339. doi: 10.1182/blood-2008-10-184754
Cobas, M., Wilson, A., Ernst, B., Mancini, S. J. C., MacDonald, H. R., Kemler, R., et al. (2004). β-catenin is dispensable for hematopoiesis and lymphopoiesis. J. Exp. Med. 199, 221–229. doi: 10.1084/jem.20031615
Coelho, R. M., Lemos, J. M., Alho, I., Valério, D., Ferreira, A. R., Costa, L., et al. (2016). Dynamic modeling of bone metastasis, microenvironment and therapy: integrating parathyroid hormone (PTH) effect, anti-resorptive and anti-cancer therapy. J. Theor. Biol. 391, 1–12. doi: 10.1016/j.jtbi.2015.11.024
Coggins, N. L., Trakimas, D., Chang, S. L., Ehrlich, A., Ray, P., Luker, K. E., et al. (2014). CXCR7 controls competition for recruitment of β-arrestin 2 in cells expressing both CXCR4 and CXCR7. PLoS ONE 9:e98328. doi: 10.1371/journal.pone.0098328
Colmone, A., Amorim, M., Pontier, A. L., Wang, S., Jablonski, E., and Sipkins, D. A. (2008). Leukemic cells create bone marrow niches that disrupt the behavior of normal hematopoietic progenitor cells. Science 322, 1861–1865. doi: 10.1126/science.1164390
Cortez, M., Carmo, L. S., Rogero, M. M., Borelli, P., and Fock, R. A. (2013). A high-fat diet increases IL-1, IL-6, and TNF-α production by increasing NF-κb and attenuating PPAR-γ expression in bone marrow mesenchymal stem cells. Inflammation 36, 379–386. doi: 10.1007/s10753-012-9557-z
Dao, M. A., Creer, M. H., Nolta, J. A., and Verfaillie, C. M. (2007). Biology of umbilical cord blood progenitors in bone marrow niches. Blood 110, 74–81. doi: 10.1182/blood-2006-08-034447
Davila-Velderrain, J., Martinez-Garcia, J. C., and Alvarez-Buylla, E. R. (2015). Modeling the epigenetic attractors landscape: toward a post-genomic mechanistic understanding of development. Front. Genet. 6:160. doi: 10.3389/fgene.2015.00160
Day, R. B., Bhattacharya, D., Nagasawa, T., and Link, D. C. (2015). Granulocyte colony-stimulating factor reprograms bone marrow stromal cells to actively suppress B lymphopoiesis in mice. Blood. doi: 10.1182/blood-2015-02-629444
De La Luz Sierra, M., Gasperini, P., McCormick, P. J., Zhu, J., and Tosato, G. (2007). Transcription factor Gfi-1 induced by G-CSF is a negative regulator of CXCR4 in myeloid cells. Blood 110, 2276–2285. doi: 10.1182/blood-2007-03-081448
de la Luz Sierra, M., Sakakibara, S., Gasperini, P., Salvucci, O., Jiang, K., McCormick, P. J., et al. (2010). The transcription factor Gfi1 regulates G-CSF signaling and neutrophil development through the Ras activator RasGRP1. Blood 115, 3970–3979. doi: 10.1182/blood-2009-10-246967
Delgado-Martín, C., Escribano, C., Pablos, J. L., Riol-Blanco, L., and Rodríguez-Fernández, J. L. (2011). Chemokine CXCL12 uses CXCR4 and a signaling core formed by bifunctional akt, Extracellular Signal-regulated Kinase (ERK)1/2, and Mammalian Target of Rapamycin Complex 1 (mTORC1) proteins to control chemotaxis and survival simultaneously in mature dendriti. J. Biol. Chem. 286, 37222–37236. doi: 10.1074/jbc.M111.294116
Doan, L. L., Porter, S. D., Duan, Z., Flubacher, M. M., Montoya, D., Tsichlis, P. N., et al. (2004). Targeted transcriptional repression of Gfi1 by GFI1 and GFI1B in lymphoid cells. Nucleic Acids Res. 32, 2508–2519. doi: 10.1093/nar/gkh570
Dorantes-Acosta, E., Vadillo, E., Contreras-Quiroz, A., Balandrán, J. C., Arriaga-Pizano, L., Purizaca, J., et al. (2013). TLR stimulation of bone marrow lymphoid precursors from childhood Acute Leukemia modifies their differentiation potentials. Biomed Res. Int. 2013:846724. doi: 10.1155/2013/846724
Duinhouwer, L. E., Tüysüz, N., Rombouts, E. W. J. C., Ter Borg, M. N. D., Mastrobattista, E., Spanholtz, J., et al. (2015). Wnt3a protein reduces growth factor-driven expansion of human hematopoietic stem and progenitor cells in serum-free cultures. PLoS ONE 10:e0119086. doi: 10.1371/journal.pone.0119086
Enciso, J., Mendoza, L., and Pelayo, R. (2015). Normal vs. Malignant hematopoiesis: the complexity of acute leukemia through systems biology. Front. Genet. 6:290. doi: 10.3389/fgene.2015.00290
Essers, M. A. G., de Vries-Smits, L. M. M., Barker, N., Polderman, P. E., Burgering, B. M. T., and Korswagen, H. C. (2005). Functional interaction between β-catenin and FOXO in oxidative stress signaling. Science 308, 1181–1184. doi: 10.1126/science.1109083
Finn, N. A., and Kemp, M. L. (2012). Pro-oxidant and antioxidant effects of N-acetylcysteine regulate doxorubicin-induced NF-kappa B activity in leukemic cells. Mol. Biosyst. 8, 650–662. doi: 10.1039/c1mb05315a
Furmento, V. A., Marino, J., Blank, V. C., and Roguin, L. P. (2014). The granulocyte colony-stimulating factor (G-CSF) upregulates metalloproteinase-2 and VEGF through PI3K/Akt and Erk1/2 activation in human trophoblast Swan 71 cells. Placenta 35, 937–946. doi: 10.1016/j.placenta.2014.09.003
Gabelloni, M. L., Sabbione, F., Jancic, C., Bass, J. F., Keitelman, I., Iula, L., et al. (2013). NADPH oxidase derived reactive oxygen species are involved in human neutrophil IL-1β secretion but not in inflammasome activation. Eur. J. Immunol. 43, 3324–3335. doi: 10.1002/eji.201243089
Geay, J.-F., Buet, D., Zhang, Y., Foudi, A., Jarrier, P., Berthebaud, M., et al. (2005). p210BCR-ABL inhibits SDF-1 chemotactic response via alteration of CXCR4 signaling and down-regulation of CXCR4 expression. Cancer Res. 65, 2676–2683. doi: 10.1158/0008-5472.CAN-04-2152
Gorbunov, N. V., Garrison, B. R., McDaniel, D. P., Zhai, M., Liao, P. J., Nurmemet, D., et al. (2013). Adaptive redox response of mesenchymal stromal cells to stimulation with lipopolysaccharide inflammagen: mechanisms of remodeling of tissue barriers in sepsis. Oxid. Med. Cell. Longev. 2013:186795. doi: 10.1155/2013/186795
Greenbaum, A., Hsu, Y.-M. S., Day, R. B., Schuettpelz, L. G., Christopher, M. J., Borgerding, J. N., et al. (2013). CXCL12 in early mesenchymal progenitors is required for haematopoietic stem-cell maintenance. Nature 495, 227–230. doi: 10.1038/nature11926
Guha, M., and Mackman, N. (2002). The phosphatidylinositol 3-kinase-Akt pathway limits lipopolysaccharide activation of signaling pathways and expression of inflammatory mediators in human monocytic cells. J. Biol. Chem. 277, 32124–32132. doi: 10.1074/jbc.M203298200
Gupta, S., Rivera-Luna, R., Ribeiro, R. C., and Howard, S. C. (2014). Pediatric oncology as the next global child health priority: the need for national childhood cancer strategies in low- and middle-income countries. PLoS Med. 11:e1001656. doi: 10.1371/journal.pmed.1001656
Hajighasemi, F., and Gheini, M. H. (2015). Lipopolysaccharide effect on vascular endothelial factor and matrix metalloproteinases in leukemic cell lines In vitro. Iran. J. Cancer Prev. 8:e2327. doi: 10.17795/ijcp2327
Higashikuni, Y., Tanaka, K., Kato, M., Nureki, O., Hirata, Y., Nagai, R., et al. (2013). Toll-like receptor-2 mediates adaptive cardiac hypertrophy in response to pressure overload through interleukin-1β upregulation via nuclear factor κB activation. J. Am. Heart Assoc. 2:e000267. doi: 10.1161/JAHA.113.000267
Hock, H., Hamblen, M. J., Rooke, H. M., Schindler, J. W., Saleque, S., Fujiwara, Y., et al. (2004). Gfi-1 restricts proliferation and preserves functional integrity of haematopoietic stem cells. Nature 431, 1002–1007. doi: 10.1038/nature02994
Holmes, T., O'Brien, T. A., Knight, R., Lindeman, R., Shen, S., Song, E., et al. (2008). Glycogen synthase kinase-3β inhibition preserves hematopoietic stem cell activity and inhibits leukemic cell growth. Stem Cells 26, 1288–1297. doi: 10.1634/stemcells.2007-0600
Hsu, H.-Y., and Wen, M.-H. (2002). Lipopolysaccharide-mediated reactive oxygen species and signal transduction in the regulation of interleukin-1 gene expression. J. Biol. Chem. 277, 22131–22139. doi: 10.1074/jbc.M111883200
Jeannet, G., Scheller, M., Scarpellino, L., Duboux, S., Gardiol, N., Back, J., et al. (2008). Long-term, multilineage hematopoiesis occurs in the combined absence of β-catenin and γ-catenin. Blood 111, 142–149. doi: 10.1182/blood-2007-07-102558
Jones, B. W., Means, T. K., Heldwein, K. A., Keen, M. A., Hill, P. J., Belisle, J. T., et al. (2001). Different Toll-like receptor agonists induce distinct macrophage responses. J. Leukoc. Biol. 69, 1036–1044.
Khandanpour, C., Phelan, J. D., Vassen, L., Schütte, J., Chen, R., Horman, S. R., et al. (2013). Growth factor independence-1 antagonizes a p53-induced DNA damage response pathway in lymphoblastic leukemia. Cancer Cell 23, 200–214. doi: 10.1016/j.ccr.2013.01.011
Khandanpour, C., Sharif-askari, E., Vassen, L., Gaudreau, M. C., Zhu, J., Paul, W. E., et al. (2010). Evidence that Growth factor independence 1b regulates dormancy and peripheral blood mobilization of hematopoietic stem cells Evidence that Growth factor independence 1b regulates dormancy and peripheral blood mobilization of hematopoietic stem cells. Blood 116, 5149–5161. doi: 10.1182/blood-2010-04-280305
Kirstetter, P., Anderson, K., Porse, B. T., Jacobsen, S. E. W., and Nerlov, C. (2006). Activation of the canonical Wnt pathway leads to loss of hematopoietic stem cell repopulation and multilineage differentiation block. Nat. Immunol. 7, 1048–1056. doi: 10.1038/ni1381
Kobayashi, H., Kobayashi, C. I., Nakamura-Ishizu, A., Karigane, D., Haeno, H., Yamamoto, K. N., et al. (2015). Bacterial c-di-GMP affects hematopoietic Stem/progenitors and their niches through STING. Cell Rep. 11, 71–84. doi: 10.1016/j.celrep.2015.02.066
Kobayashi, H., Suda, T., and Takubo, K. (2016). How hematopoietic stem/progenitors and their niche sense and respond to infectious stress. Exp. Hematol. 44, 92–100. doi: 10.1016/j.exphem.2015.11.008
Kode, A., Manavalan, J. S., Mosialou, I., Bhagat, G., Rathinam, C. V, Luo, N., et al. (2014). Leukemogenesis induced by an activating β-catenin mutation in osteoblasts. Nature 506, 240–244. doi: 10.1038/nature11130
Krawczyk, J., O'Dwyer, M., Swords, R., Freeman, C., and Giles, F. J. (2014). “The role of inflammation in leukaemia,” in Inflammation and Cancer, Vol. 816, Advances in Experimental Medicine and Biology, eds B. B. Aggarwal, B. Sung, and S. C. Gupta (Basel: Springer), 335–360. doi: 10.1007/978-3-0348-0837-8_13
Lévesque, J., Hendy, J., Takamatsu, Y., Simmons, P. J., and Bendall, L. J. (2003). Disruption of the CXCR4 / CXCL12 chemotactic interaction during hematopoietic stem cell mobilization induced by GCSF or cyclophosphamide. J. Clin. Invest. 111, 187–196. doi: 10.1172/JCI200315994
Li, B., and Smith, T. J. (2014). PI3K/AKT pathway mediates induction of IL-1RA by TSH in fibrocytes: modulation by PTEN. J. Clin. Endocrinol. Metab. 99, 3363–3372. doi: 10.1210/jc.2014-1257
Liu, H., Mihara, K., and Song, G. (2007). Interferon- γ attenuates the survival activity of G-CSF through PI3K / Akt signaling pathway in mouse multipotent progenitor cells. Ann. Hematol. 86, 547–555. doi: 10.1007/s00277-007-0308-4
Liu, Y., Zhang, X., Li, Z., and Chen, X. (2010). Up-regulation of Cx43 expression and GJIC function in acute leukemia bone marrow stromal cells post-chemotherapy. Leuk. Res. 34, 631–640. doi: 10.1016/j.leukres.2009.10.013
Lu, W., Zhao, M., Rajbhandary, S., Xie, F., Chai, X., Mu, J., et al. (2013). Free iron catalyzes oxidative damage to hematopoietic cells/mesenchymal stem cells in vitro and suppresses hematopoiesis in iron overload patients. Eur. J. Haematol. 91, 249–261. doi: 10.1111/ejh.12159
Lua, J., Suna, Y., Nombela-Arrietaa, C., Dua, K. P., Parka, S.-Y., Chaib, L., et al. (2012). Fak depletion in both hematopoietic and non-hematopoietic niche cells leads to hematopoietic stem cell expansion. Exp. Hematol. 40, 307–317. doi: 10.1016/j.biotechadv.2011.08.010
Ma, Q., Jones, D., Borghesani, P. R., Segal, R. A., Nagasawa, T., Kishimoto, T., et al. (1998). Impaired B-lymphopoiesis, myelopoiesis, and derailed cerebellar neuron migration in CXCR4- and SDF-1-deficient mice. Proc. Natl. Acad. Sci. U.S.A. 95, 9448–9453.
Majumdar, M. K., Thiede, M. A., Haynesworth, S. E., Bruder, S. P., and Gerson, S. L. (2000). Human marrow-derived mesenchymal stem cells (MSCs) express hematopoietic cytokines and support long-term hematopoiesis when differentiated toward stromal and osteogenic lineages. J. Hematother. Stem Cell Res. 9, 841–848. doi: 10.1089/152581600750062264
Malhotra, S., and Kincade, P. W. (2009). Canonical Wnt pathway signaling suppresses VCAM-1 expression by marrow stromal and hematopoietic cells. Exp. Hematol. 37, 19–30. doi: 10.1016/j.exphem.2008.08.008
Mantovani, A., Allavena, P., Sica, A., and Balkwill, F. (2008). Cancer-related inflammation. Nature 454, 444. doi: 10.1038/nature07205
Maurer, U., Charvet, C., Wagman, A. S., Dejardin, E., and Green, D. R. (2006). Glycogen synthase kinase-3 regulates mitochondrial outer membrane permeabilization and apoptosis by destabilization of MCL-1. Mol. Cell 21, 749–760. doi: 10.1016/j.molcel.2006.02.009
McGuire, V. A., Gray, A., Monk, C. E., Santos, S. G., Lee, K., Aubareda, A., et al. (2013). Cross talk between the Akt and p38α pathways in macrophages downstream of Toll-like receptor signaling. Mol. Cell. Biol. 33, 4152–4165. doi: 10.1128/MCB.01691-12
Melo, R. C. C., Longhini, A. L., Louzao Bigarella, C., Ozello Baratti, M., Traina, F., Favaro, P., et al. (2014). CXCR7 is highly expressed in acute lymphoblastic leukemia and potentiates CXCR4 response to CXCL12. PLoS ONE 9:e85926. doi: 10.1371/journal.pone.0085926
Miyamoto, K., Miyamoto, T., Kato, R., Yoshimura, A., Motoyama, N., and Suda, T. (2008). FoxO3a regulates hematopoietic homeostasis through a negative feedback pathway in conditions of stress or aging. Blood 112, 4485–4493. doi: 10.1182/blood-2008-05-159848
Molnarfi, N., Hyka-Nouspikel, N., Gruaz, L., Dayer, J.-M., and Burger, D. (2005). The production of IL-1 receptor antagonist in IFN- -stimulated human monocytes depends on the activation of Phosphatidylinositol 3-kinase but not of STAT1. J. Immunol. 174, 2974–2980. doi: 10.4049/jimmunol.174.5.2974
Müssel, C., Hopfensitz, M., and Kestler, H. A. (2010). BoolNet-an R package for generation, reconstruction and analysis of Boolean networks. Bioinformatics 26, 1378–1380. doi: 10.1093/bioinformatics/btq124
Nagai, Y., Garrett, K. P., Ohta, S., Bahrun, U., Kouro, T., Akira, S., et al. (2006). Toll-like receptors on hematopoietic progenitor cells stimulate innate immune system replenishment. Immunity 24, 801–812. doi: 10.1016/j.immuni.2006.04.008
Notta, F., Mullighan, C. G., Wang, J. C., Poeppl, A., Doulatov, S., Phillips, L. A., et al. (2011). Evolution of human BCR-ABL1 lymphoblastic leukaemia-initiating cells. Nature 469, 362–367. doi: 10.1038/nature09733nature09733 [pii]
Omatsu, Y., Seike, M., Sugiyama, T., Kume, T., and Nagasawa, T. (2014). Foxc1 is a critical regulator of haematopoietic stem/progenitor cell niche formation. Nature 508, 536–540. doi: 10.1038/nature13071
Park, S., Guo, J., Kim, D., and Cheng, J. Q. (2008). Identification of 24p3 as a direct target of Foxo3a regulated by Interleukin-3 through the phosphoinositide 3-Kinase/Akt pathway. J. Biol. Chem. 284, 2187–2193. doi: 10.1074/jbc.M806131200
Park, S.-Y., Wolfram, P., Canty, K., Harley, B., Nombela-Arrieta, C., Pivarnik, G., et al. (2013). Focal adhesion kinase regulates the localization and retention of pro-B cells in bone marrow microenvironments. J. Immunol. 190, 1094–1102. doi: 10.4049/jimmunol.1202639
Pelayo, R., Dorantes-Acosta, E., Vadillo, E., and Fuentes-Panana, E. (2012). “From HSC to B-lymphoid cells in normal and malignant hematopoiesis,” in Advances in Hematopoietic Stem Cell Research, ed R. Pelayo (InTech), 277–298. doi: 10.5772/32213
Peled, A., Kollet, O., Ponomaryov, T., Petit, I., Franitza, S., Grabovsky, V., et al. (2000). The chemokine SDF-1 activates the integrins LFA-1, VLA-4, and VLA-5 on immature human CD34(+) cells: role in transendothelial/stromal migration and engraftment of NOD/SCID mice. Blood 95, 3289–3296.
Perry, J. M., He, X. C., Sugimura, R., Grindley, J. C., Haug, J. S., Ding, S., et al. (2011). Cooperation between both Wnt/β-catenin and PTEN/PI3K/Akt signaling promotes primitive hematopoietic stem cell self-renewal and expansion. Genes Dev. 25, 1928–1942. doi: 10.1101/gad.17421911
Ponte, A. L., Ribeiro-Fleury, T., Chabot, V., Gouilleux, F., Langonné, A., Hérault, O., et al. (2012). Granulocyte-colony-stimulating factor stimulation of bone marrow mesenchymal stromal cells promotes CD34+ Cell migration via a matrix Metalloproteinase-2-Dependent mechanism. Stem Cells Dev. 21, 3162–3172. doi: 10.1089/scd.2012.0048
Purizaca, J., Contreras-Quiroz, A., Dorantes-Acosta, E., Vadillo, E., Arriaga-Pizano, L., Fuentes-Figueroa, S., et al. (2013). Lymphoid progenitor cells from childhood acute lymphoblastic leukemia are functionally deficient and express high levels of the transcriptional repressor gfi-1. Clin. Dev. Immunol. 2013:349067. doi: 10.1155/2013/349067
Purizaca, J., Meza, I., and Pelayo, R. (2012). Early lymphoid development and microenvironmental cues in B-cell acute lymphoblastic leukemia. Arch. Med. Res. 43, 89–101. doi: 10.1016/j.arcmed.2012.03.005
Raaijmakers, M. H. G. P. (2011). Niche contributions to oncogenesis: emerging concepts and implications for the hematopoietic system. Haematologica 96, 1041–1048. doi: 10.3324/haematol.2010.028035
Ramirez, P., Rettig, M. P., Uy, G. L., Deych, E., Holt, M. S., Ritchey, J. K., et al. (2009). BIO5192, a small molecule inhibitor of VLA-4, mobilizes hematopoietic stem and progenitor cells. Blood 114, 1340–1343. doi: 10.1182/blood-2008-10-184721
Reddy, S. A., G., Huang, J. H., and Liao, W. S.-L. (1997). Phosphatidylinositol 3-Kinase in Interleukin 1 Signaling: PHYSICAL INTERACTION WITH THE INTERLEUKIN 1 RECEPTOR AND REQUIREMENT IN NF B AND AP-1 ACTIVATION. J. Biol. Chem. 272, 29167–29173. doi: 10.1074/jbc.272.46.29167
Richmond, A. (2002). NF-κB, chemokine gene transcription and tumour growth. Nat. Rev. Immunol. 2, 664–674. doi: 10.1038/nri887
Satija, N. K., Sharma, D., Afrin, F., Tripathi, R. P., and Gangenahalli, G. (2013). High throughput transcriptome profiling of lithium stimulated human mesenchymal stem cells reveals priming towards Osteoblastic lineage. PLoS ONE 8:55769. doi: 10.1371/journal.pone.0055769
Schajnovitz, A., Itkin, T., D'Uva, G., Kalinkovich, A., Golan, K., Ludin, A., et al. (2011). CXCL12 secretion by bone marrow stromal cells is dependent on cell contact and mediated by connexin-43 and connexin-45 gap junctions. Nat. Immunol. 12, 391–398. doi: 10.1038/ni.2017
Scheller, M., Huelsken, J., Rosenbauer, F., Taketo, M. M., Birchmeier, W., Tenen, D. G., et al. (2006). Hematopoietic stem cell and multilineage defects generated by constitutive β-catenin activation. Nat. Immunol. 7, 1037–1047. doi: 10.1038/ni1387
Schofield, K. P., Humphries, M. J., de Wynter, E., Testa, N., and Gallagher, J. (1998). The Effect of α4β1-Integrin binding sequences of fibronectin on growth of cells from human hematopoietic progenitors. Blood 91, 3230–3238.
Scott, L. M., Priestley, G. V, and Papayannopoulou, T. (2003). Deletion of α4 integrins from adult hematopoietic cells reveals roles in homeostasis, regeneration, and homing. Mol. Cell. Biol. 23, 9349–9360. doi: 10.1128/MCB.23.24.9349-9360.2003
Semerad, C. L., Christopher, M. J., Liu, F., Short, B., Simmons, P. J., Winkler, I., et al. (2005). G-CSF potently inhibits osteoblast activity and CXCL12 mRNA expression in the bone marrow. Blood 106, 3020–3027. doi: 10.1182/blood-2004-01-0272
Shain, K. H., Dalton, W. S., and Tao, J. (2015). The tumor microenvironment shapes hallmarks of mature B-cell malignancies. Oncogene 34, 1–10. doi: 10.1038/onc.2014.403
Shalapour, S., Hof, J., Kirschner-Schwabe, R., Bastian, L., Eckert, C., Prada, J., et al. (2011). High VLA-4 expression is associated with adverse outcome and distinct gene expression changes in childhood B-cell precursor acute lymphoblastic leukemia at first relapse. Haematologica 96, 1627–1635. doi: 10.3324/haematol.2011.047993
Shin, H. C., Seo, J., Kang, B. W., Moon, J. H., Chae, Y. S., and Lee, S. J. (2014). Clinical significance of nuclear factor κ B and chemokine receptor CXCR4 expression in patients with diffuse large B-cell lymphoma who received rituximab-based therapy. Korean J. Intern. Med. 29, 785–792. doi: 10.3904/kjim.2014.29.6.785
Sierro, F., Biben, C., Martínez-Muñoz, L., Mellado, M., Ransohoff, R. M., Li, M., et al. (2007). Disrupted cardiac development but normal hematopoiesis in mice deficient in the second CXCL12/SDF-1 receptor, CXCR7. Proc. Natl. Acad. Sci. U.S.A. 104, 14759–14764. doi: 10.1073/pnas.0702229104
Sizemore, N., Leung, S., and Stark, G. R. (1999). Activation of Phosphatidylinositol 3-Kinase in Response to Interleukin-1 Leads to Phosphorylation and Activation of the NF- κB p65/RelA Subunit. Mol. Cell. Biol. 19, 4798–4805.
Stiehl, T., and Marciniak-Czochra, A. (2012). Mathematical Modeling of Leukemogenesis and Cancer Stem Cell Dynamics. Math. Model. Nat. Phenom. 7, 166–202. doi: 10.1051/mmnp/20127199
Sugiyama, T., Kohara, H., Noda, M., and Nagasawa, T. (2006). Maintenance of the hematopoietic stem cell pool by CXCL12-CXCR4 chemokine signaling in bone marrow stromal cell niches. Immunity 25, 977–988. doi: 10.1016/j.immuni.2006.10.016
Swaminathan, S., Klemm, L., Park, E., Papaemmanuil, E., Ford, A., Kweon, S.-M., et al. (2015). Mechanisms of clonal evolution in childhood acute lymphoblastic leukemia. Nat. Immunol. 16, 766–774. doi: 10.1038/ni.3160
Tabe, Y., Jin, L., Tsutsumi-Ishii, Y., Xu, Y., McQueen, T., Priebe, W., et al. (2007). Activation of integrin-linked kinase is a critical prosurvival pathway induced in leukemic cells by bone marrow-derived stromal cells. Cancer Res. 67, 684–694. doi: 10.1158/0008-5472.CAN-06-3166
Tabe, Y., and Konopleva, M. (2015). Role of microenvironment in resistance to therapy in AML. Curr. Hematol. Malig. Rep. 10, 96–103. doi: 10.1007/s11899-015-0253-6
Tapia-Abellán, A., Ruiz-Alcaraz, A. J., Antón, G., Miras-López, M., Francés, R., Such, J., et al. (2014). Regulatory role of PI3K-protein kinase B on the release of interleukin-1β in peritoneal macrophages from the ascites of cirrhotic patients. Clin. Exp. Immunol. 178, 525–536. doi: 10.1111/cei.12428
Tak, P. P., and Firestein, G. S. (2001). NF- κB: a key role in inflammatory diseases. J. Clin. Invest. 107, 7–11. doi: 10.1172/JCI11830
Tarnowski, M., Liu, R., Wysoczynski, M., Ratajczak, J., Kucia, M., and Ratajczak, M. Z. (2010). CXCR7: a new SDF-1-binding receptor in contrast to normal CD34+ progenitors is functional and is expressed at higher level in human malignant hematopoietic cells. Eur. J. Haematol. 85, 472–483. doi: 10.1111/j.1600-0609.2010.01531.x
Tesio, M., Oser, G. M., Baccelli, I., Blanco-Bose, W., Wu, H., Göthert, J. R., et al. (2013). Pten loss in the bone marrow leads to G-CSF-mediated HSC mobilization. J. Exp. Med. 210, 2337–2349. doi: 10.1084/jem.20122768
Tokoyoda, K., Egawa, T., Sugiyama, T., Choi, B.-I., and Nagasawa, T. (2004). Cellular niches controlling B lymphocyte behavior within bone marrow during development. Immunity 20, 707–718. doi: 10.1016/j.immuni.2004.05.001
Tomasetti, C., and Vogelstein, B. (2015). Variation in cancer risk among tissues can be explained by the number of stem cell divisions. Science 347, 78–81. doi: 10.1126/science.1260825
Torossian, F., Anginot, A., Chabanon, A., Clay, D., Guerton, B., Desterke, C., et al. (2014). CXCR7 participates in CXCL12-induced CD34+ cell cycling through b-arrestin–dependent Akt activation. Blood 123, 191–202. doi: 10.1182/blood-2013-05-500496
Tzeng, Y.-S., Li, H., Kang, Y.-L., Chen, W.-C., Cheng, W.-C., and Lai, D.-M. (2011). Loss of Cxcl12/Sdf-1 in adult mice decreases the quiescent state of hematopoietic stem/progenitor cells and alters the pattern of hematopoietic regeneration after myelosuppression. Blood 117, 429–439. doi: 10.1182/blood-2010-01-266833
Ueda, Y., Yang, K., Foster, S. J., Kondo, M., and Kelsoe, G. (2004). Inflammation controls B lymphopoiesis by regulating chemokine CXCL12 expression. J. Exp. Med. 199, 47–58. doi: 10.1084/jem.20031104
Uto-Konomi, A., McKibben, B., Wirtz, J., Sato, Y., Takano, A., Nanki, T., et al. (2013). CXCR7 agonists inhibit the function of CXCL12 by down-regulation of CXCR4. Biochem. Biophys. Res. Commun. 431, 772–776. doi: 10.1016/j.bbrc.2013.01.032
Vadillo, E., Dorantes-Acosta, E., Arriaga-Pizano, L., Chavez-Gonzalez, A., Reyes-Maldonado, E., Garrett, K. P., et al. (2014). Adult, but not neonatal, human lymphoid progenitors respond to TLR9 ligation by producing functional NK-like cells. Exp. Hematol. 42, 562–573. doi: 10.1016/j.exphem.2014.03.008
Vadillo, E., Dorantes-acosta, E., and Pelayo, R. (2013). “Regulation of hematopoietic stem / progenitor cell development by inflammation cues,” in Molecular Aspects of Inflammation, eds L. Perez-Martinez, G. Pedraza-Alva, and E. F. Osorio (Kerala: Research Signpost), 71–87.
Vagima, Y., Avigdor, A., Goichberg, P., Shivtiel, S., Tesio, M., Kalinkovich, A., et al. (2009). MT1-MMP and RECK are involved in human CD34+ progenitor cell retention, egress, and mobilization. J. Clin. Invest. 119, 492–503. doi: 10.1172/JCI36541
van den Berk, L. C. J., van der Veer, A., Willemse, M. E., Theeuwes, M. J. G., a, Luijendijk, M. W., Tong, W. H., et al. (2014). Disturbed CXCR4/CXCL12 axis in paediatric precursor B-cell acute lymphoblastic leukaemia. Br. J. Haematol. 166, 240–249. doi: 10.1111/bjh.12883
Vilchis-Ordoñez, A., Contreras-Quiroz, A., Vadillo, E., Dorantes-Acosta, E., Reyes-López, A., Quintela-Nuñez del Prado, H. M., et al. (2015). Bone marrow cells in acute lymphoblastic leukemia create a proinflammatory microenvironment influencing normal hematopoietic differentiation fates. Biomed Res. Int. 2015:386165. doi: 10.1155/2015/386165
Vilchis-Ordoñez, A., Dorantes-Acosta, E., Vadillo, E., López-Martínez, B., and Pelayo, R. (2016). “Early hematopoietic differentiation in acute lymphoblastic leukemia: the interplay between leukemia-initiating cells and abnormal bone marrow microenvironment,” in Etiology of Acute Leukemias in Children ed J. M. Mejía-Aranguré (Cham: Springer International publishing), 291–318.
Wang, L., You, L., Ni, W., Ma, Q., Tong, Y., Mao, L., et al. (2013). β-Catenin and AKT are promising targets for combination therapy in acute myeloid leukemia. Leuk. Res. 37, 1329–1340. doi: 10.1016/j.leukres.2013.06.023
Wang, M. W., Consoli, U., Lane, C. M., Durett, A., Lauppe, M. J., Champlin, R., et al. (1998). Rescue from apoptosis in early (CD34-selected) versus late (non-CD34-selected) human hematopoietic cells by very late antigen 4- and vascular cell adhesion molecule (VCAM) 1-dependent adhesion to bone marrow stromal cells. Cell Growth Differ. 9, 105–112.
Wang, T., Zou, J., Cunningham, C., and Secombes, C. J. (2002). Cloning and functional characterisation of the interleukin-1 β1 promoter of rainbow trout (Oncorhynchus mykiss). Biochim. Biophys. Acta 1575, 108–116. doi: 10.1016/S0167-4781(02)00235-X
Wang, X., Cheng, Q., Li, L., Wang, J., Xia, L., Xu, X., et al. (2012). Toll-like receptors 2 and 4 mediate the capacity of mesenchymal stromal cells to support the proliferation and differentiation of CD34+ cells. Exp. Cell Res. 318, 196–206. doi: 10.1016/j.yexcr.2011.11.001
Wang, Z. J., Zhang, F. M., Wang, L. S., Yao, Y. W., Zhao, Q., and Gao, X. (2009). Lipopolysaccharides can protect mesenchymal stem cells (MSCs) from oxidative stress-induced apoptosis and enhance proliferation of MSCs via Toll-like receptor(TLR)-4 and PI3K/Akt. Cell Biol. Int. 33, 665–674. doi: 10.1016/j.cellbi.2009.03.006
Welner, R. S., Pelayo, R., Nagai, Y., Garrett, K. P., Wuest, T. R., Carr, D. J., et al. (2008). Lymphoid precursors are directed to produce dendritic cells as a result of TLR9 ligation during herpes infection. Blood 112, 3753–3761. doi: 10.1182/blood-2008-04-151506
Williams, D. L., Li, C., Ha, T., Ozment-Skelton, T., Kalbfleisch, J. H., Preiszner, J., et al. (2004). Modulation of the phosphoinositide 3-kinase pathway alters innate resistance to polymicrobial sepsis. J. Immunol. 172, 449–456. doi: 10.4049/jimmunol.172.1.449
Wu, M., Yang, X., and Chan, C. (2009). A dynamic analysis of IRS-PKR signaling in liver cells: a discrete modeling approach. PLoS ONE 4:e8040. doi: 10.1371/journal.pone.0008040
Xu, J., Qian, J., Xie, X., Lin, L., Zou, Y., Fu, M., et al. (2012). High density lipoprotein protects mesenchymal stem cells from oxidative stress-induced apoptosis via activation of the PI3K/Akt pathway and suppression of reactive oxygen species. Int. J. Mol. Sci. 13, 17104–17120. doi: 10.3390/ijms131217104
Yamazaki, S., Iwama, A., Takayanagi, S., Morita, Y., Eto, K., Ema, H., et al. (2006). Cytokine signals modulated via lipid rafts mimic niche signals and induce hibernation in hematopoietic stem cells. EMBO J. 25, 3515–3523. doi: 10.1038/sj.emboj.7601236
Yang, D., Elner, S. G., Bian, Z. M., Till, G. O., Petty, H. R., and Elner, V. M. (2007). Pro-inflammatory cytokines increase reactive oxygen species through mitochondria and NADPH oxidase in cultured RPE cells. Exp. Eye Res. 85, 462–472. doi: 10.1016/j.exer.2007.06.013
Yi, L., Chandrasekaran, P., and Venkatesan, S. (2012). TLR signaling paralyzes monocyte chemotaxis through synergized effects of p38 MAPK and global rap-1 activation. PLoS ONE 7:30404. doi: 10.1371/journal.pone.0030404
Zeng, H., Yücel, R., Kosan, C., Klein-Hitpass, L., and Möröy, T. (2004). Transcription factor Gfi1 regulates self-renewal and engraftment of hematopoietic stem cells. EMBO J. 23, 4116–4125. doi: 10.1038/sj.emboj.7600419
Zhang, X., Liu, Y., Si, Y., Chen, X., Li, Z., Gao, L., et al. (2012). Effect of Cx43 gene-modified leukemic bone marrow stromal cells on the regulation of Jurkat cell line in vitro. Leuk. Res. 36, 198–204. doi: 10.1016/j.leukres.2011.10.001
Zhang, Y., Zhai, W., Zhao, M., Li, D., Chai, X., Cao, X., et al. (2015). Effects of Iron overload on the bone marrow microenvironment in mice. PLoS ONE 10:e0120219. doi: 10.1371/journal.pone.0120219
Keywords: cancer systems biology, acute lymphoblastic leukemia, tumor microenvironment, CXCL12, pro-inflammatory bone marrow, early hematopoiesis, network modeling, dynamical systems
Citation: Enciso J, Mayani H, Mendoza L and Pelayo R (2016) Modeling the Pro-inflammatory Tumor Microenvironment in Acute Lymphoblastic Leukemia Predicts a Breakdown of Hematopoietic-Mesenchymal Communication Networks. Front. Physiol. 7:349. doi: 10.3389/fphys.2016.00349
Received: 22 March 2016; Accepted: 02 August 2016;
Published: 19 August 2016.
Edited by:
Christian Diener, National Institute of Genomic Medicine, MexicoReviewed by:
Oksana Sorokina, University of Edinburgh, UKMarcio Luis Acencio, Norwegian University of Science and Technology, Norway
Copyright © 2016 Enciso, Mayani, Mendoza and Pelayo. This is an open-access article distributed under the terms of the Creative Commons Attribution License (CC BY). The use, distribution or reproduction in other forums is permitted, provided the original author(s) or licensor are credited and that the original publication in this journal is cited, in accordance with accepted academic practice. No use, distribution or reproduction is permitted which does not comply with these terms.
*Correspondence: Luis Mendoza, bG1lbmRvemFAYmlvbWVkaWNhcy51bmFtLm14
Rosana Pelayo, cm9zYW5hcGVsYXlvQGdtYWlsLmNvbQ==