- 1Li Ka Shing Faculty of Medicine, School of Biomedical Sciences, University of Hong Kong, Hong Kong, China
- 2School of Medicine, Imperial College London, London, UK
- 3Department of Medicine and Therapeutics, The Chinese University of Hong Kong, Hong Kong, China
- 4Department of Epidemiology and Preventive Medicine, Monash University, Melbourne, VIC, Australia
Bayés syndrome is an under-recognized clinical condition characterized by inter-atrial block (IAB). This is defined electrocardiographically as P-wave duration > 120 ms and can be categorized into first, second and third degree IAB. It can be caused by inflammatory conditions such as systemic sclerosis and rheumatoid arthritis, abnormal protein deposition in cardiac amyloidosis, or neoplastic processes invading the inter-atrial conduction system, such as primary cardiac lymphoma. It may arise transiently during volume overload, autonomic dysfunction or electrolyte disturbances from vomiting. In other patients without an obvious cause, the predisposing factors are diabetes mellitus, hypertensive heart disease, and hypercholesterolemia. IAB has a strong association with atrial arrhythmogenesis, left atrial enlargement (LAE), and electro-mechanical discordance, increasing the risk of cerebrovascular accidents as well as myocardial and mesenteric ischemia. The aim of this review article is to synthesize experimental evidence on the pathogenesis of IAB and its underlying molecular mechanisms. Current medical therapies include anti-fibrotic, anti-arrhythmic and anti-coagulation agents, whereas interventional options include atrial resynchronization therapy by single or multisite pacing. Future studies will be needed to elucidate the significance of the link between IAB and atrial tachyarrhythmias in patients with different underlying etiologies and optimize the management options in these populations.
Introduction
The first case of inter-atrial block (IAB) was first described by Bachmann (1941), who recognized the significance of P-wave splitting on the ECG, some 25 years after he described the anatomy of Bachmann's bundle (Bachmann, 1916). Dr Bayés de Luna was the first who provided a clear description of atrial conduction block in 1979, classifying them into either inter- and intra-atrial (Bayés de Luna, 1979). In recognition of his numerous contributions to the understanding of IAB (Bayes de Luna et al., 1985), this disease was later named Bayés syndrome (Conde and Baranchuk, 2014).
The cardiac conduction system starts at the sinoatrial node, which is the pacemaker responsible for initiating action potentials (APs) that are conducted through the right atrium via three distinct inter-nodal pathways to the atrioventricular node. These are the anterior, middle (Wenckebach) and posterior (Thorel) pathways (Figure 1; Conde et al., 2015). Inter-atrial conduction from the right to left atrium occurs most frequently along Bachmann's bundle, which branches from the anterior internodal pathway (James, 1963; Racker, 1989), but it can also pass through the coronary sinus or the fossa ovalis (Tapanainen et al., 2009).
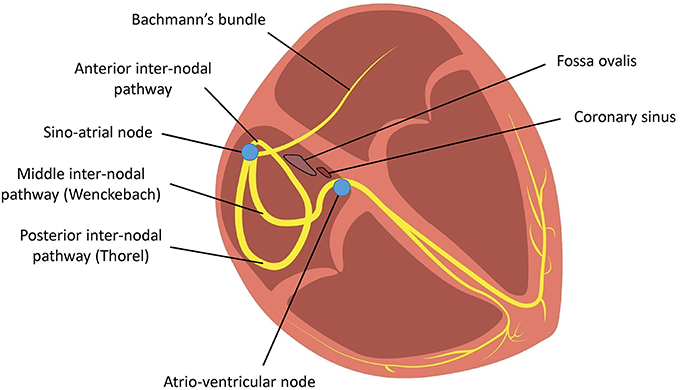
Figure 1. Conduction of action potentials from the sinoatrial node across the right atrium to the atrioventricular node occurs via three inter-nodal tracts. Conduction from the right atrium to the left atrium occurs via Bachmann's bundle.
IAB is caused by impaired conduction along Bachmann's bundle. Two definitions of IAB have been proposed. The classification adopted in the consensus report divides it into either partial (P wave duration > 120 ms) or advanced (P wave duration > 120 ms with biphasic morphology in the inferior leads; Bayés de Luna, 1979). Alternatively, similar to sinoatrial or atrioventricular block, it can be divided into first (partial), second (first degree with intermittent conduction through Bachmann's bundle) or third degree (advanced; Figure 2; Bayes de Luna et al., 2012; Chhabra et al., 2014). Partial or first degree IAB is characterized by prolonged P wave duration of >120 ms, with bifid (notching) with dome-and-spike morphology on the ECG. The definition of a normal P-wave duration is a contentious issue because 120 ms has been considered by some clinicians to be normal, yet the upper limit was defined by the World Health Organization and the International Society and Federation of Cardiology Task Force to be 110 ms (Willems et al., 1985). This may arise from difficulty in accurately measuring the P-wave duration (Baranchuk et al., 2016), which is in part due to baseline noise (Magnani et al., 2010). Intra- and inter-observer variability also contributes to inconsistent reported values (Dilaveris et al., 1999). Nevertheless, for first degree or partial IAB, a recent consensus report agreed on the definition of P-wave duration > 120 ms (Bayes de Luna et al., 2012). This is in keeping with a previous study demonstrating the modal P-wave duration to be 120 ms in first-degree IAB (Ariyarajah et al., 2006b).
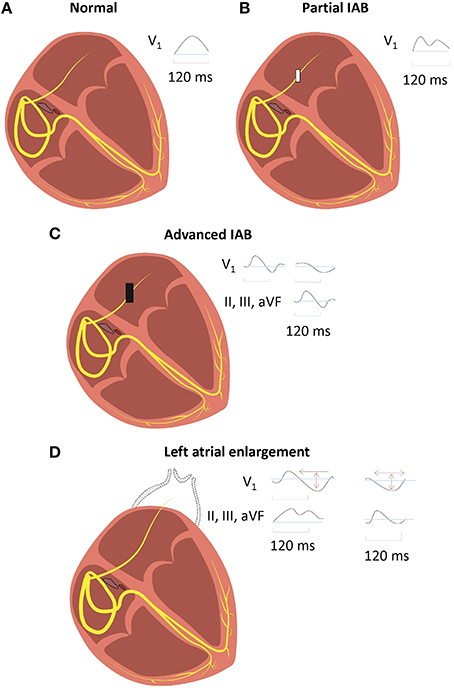
Figure 2. Normal inter-atrial conduction (A), partial (B), and advanced (C) inter-atrial block (IAB), with distinct electrocardiographic findings. IAB should be distinguished from left atrial enlargement (LAE) (D), where there is conduction delay from lengthening of Bachmann's bundle in the absence of block.
In second degree IAB, the P-wave shows an initial invariant component but a second component with varying morphology within the same ECG. This is commonly seen in atrial aberrancy (Chung, 1970), with concealed atrial conduction from post-ectopic inhibition (Chung's phenomenon; Chung and Chung, 1972). Bachmann's bundle has a long effective refractory period (ERP; Vollmann et al., 2005), during which Na+ channels cannot be reactivated. Therefore, a premature AP will not be able to pass through this bundle, but has to take another and potentially longer path. Second degree IAB can occur in an absence of a premature atrial beat. A limitation of the definition of second degree IAB is that to diagnose intermittent conduction through Bachmann's bundle, a constant interval between two P-waves is needed. If this interval is not constant, then it is possible that variable morphologies can be explained by APs with different initiation sites, for example, opposite sides of the crista terminalis.
In advanced or third degree IAB, there is a biphasic P-wave in the inferior leads with a negative terminal deflection below the isoelectric line. As conduction via Bachmann's bundle is completely blocked, the AP wave must pass through another pathway, such as the coronary sinus. This may result in retrograde activation of the left atrium in the caudo-cranial direction, producing a negative terminal deflection (Bayes de Luna et al., 1988; Cosio et al., 2004). However, if the wave passes through the rim of the fossa ovalis, then retrograde activation may not necessarily occur.
Advanced IAB is clinically important as there is a high chance of developing supraventricular tachycardia if it is left untreated (Bayes de Luna et al., 1999). Left atrial enlargement (LAE) is often associated with, but should be distinguished from, IAB (Mehrzad and Spodick, 2014). LAE leads to prolonged inter-atrial conduction times because of increased stretch and lengthening of Bachmann's bundle (Boineau, 2005). This delay is due to increased distance of conduction rather than block in the bundle per se. In the inferior leads, there are biphasic P-waves but without the terminal negative deflections seen in third degree IAB. LAE can be diagnosed on the ECG by a biphasic P-wave on V1 together with an area under the curve for the second phase < 40 mm.ms (Chhabra et al., 2014).
The prevalence of IAB is age-dependent, increasing from 5.4% at < 20 years old to 60% at >50 (Jairath and Spodick, 2001; Asad and Spodick, 2003; Ariyarajah et al., 2005; Gialafos et al., 2007; Martinez-Selles et al., 2016b). This is likely the result of aging-related fibrosis, which would result in impaired AP conduction through the atria (Gramley et al., 2009; Fleg and Strait, 2012). The risk factors for developing IAB are coronary artery disease, hypertension, diabetes mellitus and hypercholesterolemia (Figure 3; Ariyarajah et al., 2006a). IAB can be caused by structural defects of the conducting pathway, such as atrial septal defect (Thilen et al., 2007) or aneurysm (Okutucu et al., 2010), or infiltration of the bundle from cardiac lymphoma (Engelen et al., 2005; Peyrou et al., 2013) or amyloidosis (Rocken et al., 2002). Alternatively, inflammation can induce cardiac structural remodeling, which can occur in hypertrophic cardiomyopathy (Szili-Torok et al., 2014) or systemic inflammatory diseases such as scleroderma and rheumatoid arthritis (Mizuno et al., 1999; Acar et al., 2009). Transient IAB may be related to autonomic dysfunction, increased atrial strain or electrolyte abnormalities. For example, it was observed in decompensated heart failure with increased atrial strain from volume overload, which disappeared after its resolution using diuretic therapy (Song et al., 2002; Proietti et al., 2012), or hemodialysis patients with vomiting (Enriquez et al., 2015). In terms of disease progression, it takes around 66 months to progress from a normal P-wave duration to advanced IAB (Ariyarajah et al., 2007b). IAB is important because of increased risks of atrial arrhythmias (tachycardia, flutter, fibrillation) complicated by LAE and electro-mechanical discordance (Ariyarajah et al., 2007a). This predisposes to increased thrombosis in obstructive sleep apnea (Can et al., 2009; Cagirci et al., 2011; Maeno et al., 2013) and to myocardial ischemia (Myrianthefs et al., 1991), cerebral vascular accidents (Lorbar et al., 2005), and mesenteric ischemia (Chhabra et al., 2012).
IAB can be managed with pharmacological therapy, such as angiotensin-converting enzyme (ACE) inhibitors, which can delay the progression from first degree to third degree IAB (Ariyarajah et al., 2007b). It can also be managed by interventional procedures, such as multi-site or single site pacing at the triangle of Koch or Bachmann's bundle. It is unclear whether there is any benefit in early treatment. Atrial resynchronization therapy can be used to correct for left-sided atrioventricular dyssynchrony arising from IAB (Daubert et al., 2004).
Electrophysiological Mechanisms of Bayés Syndrome
To understand the electrophysiological mechanisms of IAB and how it increases atrial arrhythmic risk, the determinants of AP conduction through the myocardium must first be considered (Kléber and Rudy, 2004; Tse and Yeo, 2015; Tse et al., 2016b). This can be described by the core conduction theory (Barr et al., 2003). Conduction velocity (CV) depends on both passive and active electrical properties. Passive properties refer to the resistive and capacitive components and the architecture of the myocardium. They include the axial resistance (ri) of both the myoplasm (Thomas et al., 2003) and the gap junctions between cardiomyocytes (Rohr et al., 1998; Chen et al., 2007), resistance of the extracellular space (ro), and the membrane capacitance (Cm). Active membrane properties refer to the voltage-gated ionic conductances: the most important conductance is that of Na+ channels, which mediates INa and determine the AP upstroke. Reduced CV can arise from increased ri, ro or Cm, decreased maximum upstroke velocity (dV/dtmax, from reduced Na+ current density, Nattel, 2008; Tse et al., 2016g) or decreased myocardial excitability given by 1/(Threshold Potential–Resting Membrane Potential). Cardiac fibrosis can decrease CV by the following mechanisms: reduced cardiomyocyte-cardiomyocyte coupling, which increases ri, or increased fibroblast-cardiomyocyte coupling, which increases Cm (Tse and Yeo, 2015). Moreover, fibroblast-cardiomyocyte coupling can depolarize cardiomyocytes (Rohr, 2012; Kohl and Gourdie, 2014; Thomsen and Calloe, 2016), leading to Na+ channel inactivation and reduced dV/dtmax. It is increasingly recognized that passive and active properties are not independent of each other, since gap junctions and Na+ channels co-localize in the connexome, and their close proximity to each other could enable ephaptic conduction (Rhett and Gourdie, 2012; Veeraraghavan et al., 2012, 2014a,b,c, 2015; Rhett et al., 2013; George et al., 2015). Increased risk of arrhythmogenesis by either circus-type or spiral wave reentry can be explained by a reduction in excitation wavelength (λ) given by CV × ERP (Wiener and Rosenblueth, 1946; Smeets et al., 1986; Vaidya et al., 1999; Tse, 2015; Tse et al., 2016f, in press). Selective atrial fibrosis could increase the heterogeneity of conduction by allowing micro-reentry to take place in smaller areas in atrial fibrosis (Spach and Josephson, 1994; Verheule et al., 2004).
Inter-Atrial Block Can Arise from Abnormal Function or Expression of Sodium Channels and Gap Junctions
The conditions predisposing or causing IAB can affect any of these parameters. Animals have been extensively used to study cardiac arrhythmogenesis in a number of clinical conditions because of their amenability to genetic and pharmacological manipulation (Chen et al., in press; Choy et al., 2016). In these systems, electrical activity can be recorded using different techniques such as monophasic action potential and bipolar electrogram methods, optical mapping and surface electrocardiography (Vigmond and Leon, 1999; van Rijen et al., 2001; Vigmond, 2005; Vigmond et al., 2009; Tse et al., 2016c,h). Although few mouse models have been generated specifically for studying IAB, experiments in different systems have increased our understanding on the molecular determinants of AP conduction (Tse et al., 2012, 2016d,e,i; George et al., 2015; Veeraraghavan et al., 2015) and how abnormalities in ion channels or cardiac remodeling lead to intra- or inter-atrial conduction defects (Figure 4).
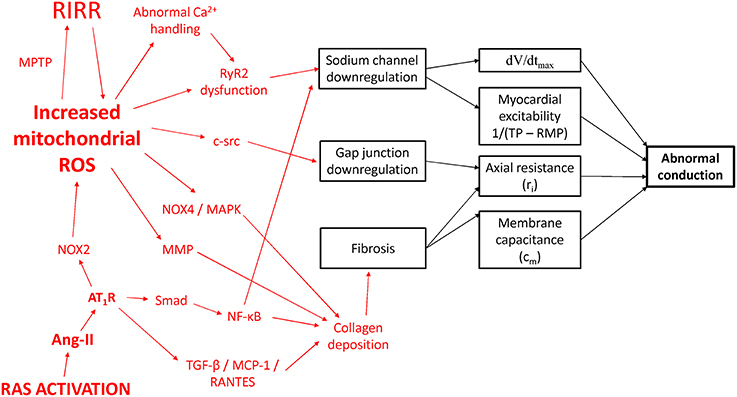
Figure 4. Potential molecular mechanisms leading to conduction abnormalities in IAB. RAS, renin-angiotensin system; Ang-II, angiotensin II; AT1R, angiotensin II receptor isoform 1; NOX, NADPH oxidase; MAPK, mitogen-activated protein kinase; MCP-1, monocyte chemoattractant protein-1; MMP, matrix metalloproteinase; MPTP, mitochondrial permeability transition pore; NF-κB, nuclear factor kappa-light-chain-enhancer of activated B cells; RANTES, Regulated on Activation, Normal T Cell Expressed and Secreted; ROS, reactive oxygen species; RIRR, ROS-induced ROS release; TGF-β, transforming growth factor-beta; RyR2, ryanodine receptor isoform 2.
Conduction between successive cardiomyocytes occurs via gap junctions. Each gap junction consists of two connexons, each of which is a hexamer of proteins called connexins (Cx). Cx40 and 43 are the isoforms expressed in the atria (Beyer et al., 1987; Gourdie et al., 1993a,b). Cx40−∕− mice showed intra-atrial conduction delay (Hagendorff et al., 1999; Verheule et al., 1999). Mice with cardiomyocyte-directed expression of CREM-IbΔC-X, an isoform of transcription factor CREM (Kirchhof et al., 2013), showed evidence of fibrosis, atrial dilatation and IAB, associated with downregulation of Cx40 and ryanodine receptor 2 (RyR2)-mediated Ca2+ leak from the sarcoplasmic reticulum (Li et al., 2014). Increased leak could have a knock on effect by downregulating Na+ channels and decreasing INa (Curran and Louch, 2015).
Liver kinase B1, which has been termed the master upstream kinase, normally activates AMP-activated protein kinase (AMPK) and other related kinases (Gan and Li, 2014). Its deletion led to downregulation of both Cx40 and Na+ channels, resulting in complete absence of inter-atrial conduction (Kim et al., 2015). This was later complicated by atrial enlargement and fibrosis without inflammation, hypertrophy or apoptosis. Interestingly, mice with knockout of regulator of G-protein signaling 5 (Rgs5−∕−), a negative regulator of G protein-mediated signaling, showed increased P-wave duration in the absence of atrial dilatation or fibrosis (Qin et al., 2012). These two experiments on mouse model support the notion that although IAB and LAE frequently co-exist, they have different underlying pathologies.
Increased Oxidative Stress, Renin-Angiotensin System Activation and IAB
For cardio-metabolic disorders such as hypertension and diabetes, the common link appears to involve increased reactive oxygen species (ROS) production leading to excess oxidative stress (Tse et al., 2016a; Zhang et al., 2016). In hypertension, there is increased renin-angiotensin system (RAS) activation with elevated levels of angiotensin II (Murugan et al., 2015; Zhang et al., 2015). Mice infused with angiotensin II showed inter-atrial conduction delay, which was dependent on the leptin signaling pathway (Fukui et al., 2013). This resulted in upregulation of transforming growth factor beta, Monocyte Chemoattractant Protein-1 and RANTES, ultimately leading to fibrosis from deposition of collagen types 1 and 3. Ang-II has been shown to mediate cardiac fibrosis and inflammation via the Smad/NF-κB pathway (Wei et al., 2013). NF-κB can bind to the promoter region of the gene encoding for the Na+ channel (Shang and Dudley, 2005) to reduce its transcriptional activity (Shang et al., 2008).
Moreover, cardiac-restricted ACE overexpression produced conduction defects and reduced expression of atrial connexin 40 (Cx40) and connexin 43 (Cx43) proteins. Activation of the AT1 receptor by Ang-II stimulates Nox2 to generate oxygen free radicals, which can diffuse to and promote further ROS release at the mitochondria, by activation of the mitochondrial permeability transition (MPT) to mediate ROS-induced ROS release (RIRR; Zorov et al., 2000). Mitochondrial ROS can decrease the expression of Cx43 via c-src activation (Sovari et al., 2013), as well as induce myocardial fibrosis via NOX4/MAPK signaling (Aragno et al., 2006; Kuroda et al., 2010). Diabetes produces a cardiomyopathy characterized by diastolic dysfunction and structural remodeling. Cardiac fibrosis is observed in many models of diabetes. Thus, OVE26 mice modeling type 1 diabetes mellitus showed increased nuclear factor-κB and matrix metalloproteinase (MMP) activities and cardiac fibrosis (Li et al., 2011).
Leptin-deficient ob/ob mice modeling human diabetes mellitus showed reduced pro-MMP-8, -9, and -13 gene expression and increased stimulation of pro-collagen Iα, resulting in cardiac fibrosis (Zibadi et al., 2011). Leptin receptor-deficient db/db mice similarly show increased fibrosis (Cox and Marsh, 2014). In diabetes, RyR2 gating is abnormal due to channel oxidation by ROS (Eager et al., 1997; Xu et al., 1998; Bidasee et al., 2003) and phosphorylation by Ca2+/calmodulin-dependent protein kinase II (Witcher et al., 1991; Hain et al., 1995; Wehrens et al., 2004), which would lead to INa downregulation as discussed above. Taken together, inflammation or infiltration lead to electrophysiological remodeling of Na+ channel and gap junction downregulation, as well as structural remodeling of fibrosis. Together, these produce conduction abnormalities that underlie conduction block in Bayés syndrome.
Future Perspective
Our understanding of Bayés syndrome has increased significantly due to the development of mapping systems and the use of genetic and pharmacological mouse models for studying cardiac electrophysiology. Despite its clinical significance, this condition is under-diagnosed. Raising the awareness of IAB in healthcare professionals could improve its diagnostic rates (Baranchuk and Bayes de Luna, 2015). Risk stratification is important for determining individuals who are most at risk of cardiac arrhythmias (Tse, 2016a,b,c; Tse and Yan, 2016), and should include patients with Bayés syndrome (Martinez-Selles et al., 2016a). Measurement of magnetic fields in the heart has been useful for characterizing cardiac structural abnormalities (Vassiliou et al., 2014; Tse et al., 2015a,b), which can be useful for detecting atrial fibrosis. Magnetocardiography can be used to diagnose and predict the risk of cardiac arrhythmias in clinical practice (Steinhoff et al., 2004; Kuijpers et al., 2011; Sato et al., 2012; Kwong et al., 2013; Ito et al., 2014; Yoshida et al., 2015) and has the potential for early detection of IAB (Jurkko et al., 2009).
IAB results in delayed and asynchronous activation of the left atrium (Agarwal et al., 2003; Budeus et al., 2005; Caldwell et al., 2014). IAB, particularly in its advanced form, is frequently associated with supraventricular tachy-arrhythmias (Bayes de Luna et al., 1999; Conde et al., 2015) and higher cardiovascular and all-cause mortality (Ariyarajah et al., 2007c; Magnani et al., 2011). The pathogenesis of AF in the context of IAB has been studied in detail, demonstrating the occurrence of the following event sequence: abnormal atrial activation can lead to increased atrial pressure, with subsequent electrophysiological and structural remodeling, such as atrial dilatation and fibrosis. Furthermore, endothelial damage and dysfunction, together with impaired atrial mechanical activity, is thrombogenic (Martinez-Selles et al., 2016a). Regarding the optimal management of IAB and AF, anti-arrhythmic treatment can reduce the recurrence rate of atrial fibrillation (AF) associated with IAB (Bayes de Luna et al., 1988, 1989). Anti-coagulation is needed to reduce the risk of thrombo-embolic complications. Anti-coagulation is likely to be beneficial for patients with IAB, even before the development of AF (Martinez-Selles et al., 2016a). A recent paper proposed that anticoagulation treatment should be initiated based on the following criteria: P wave duration ≥ 160 ms, structural heart disease, >40 atrial premature beats/h and/or runs in Holter monitoring and CHA2DS2-VASc score ≥ 2. ACE inhibitors are now increasingly recognized for their anti-fibrotic effects and trials should be conducted to determine their relative efficacies in reducing arrhythmic risk, morbidity and mortality in patients with IAB. Interventional management, such as synchronous biatrial pacing, can be used to prevent the recurrences of AF associated with IAB (D'Allonnes et al., 2000). However, resynchronization therapy may be difficult in situations such as hypertrophic cardiomyopathy or heart failure, where patients will have abnormal cardiac hemodynamics. Thus, future studies will be needed for its clarification.
Author Contributions
GT: Design of manuscript; drafted and critically revised the manuscript for important intellectual content; preparation of figures. EL: Acquired and interpreted primary research papers; critically revised the manuscript for important intellectual content; preparation of figures. JY: Analyzed and interpreted primary research papers; critically revised the manuscript for important intellectual content. BY: drafted and critically revised the manuscript for important intellectual content. All authors approved the final version, ensured that the text is accurate and agreed to be accountable for all aspects of the work.
Conflict of Interest Statement
The authors declare that the research was conducted in the absence of any commercial or financial relationships that could be construed as a potential conflict of interest.
Acknowledgments
GT was awarded a BBSRC Doctoral Training Award at the University of Cambridge for his PhD.
References
Acar, G., Sayarlioglu, M., Akcay, A., Sokmen, A., Sokmen, G., Yalcintas, S., et al. (2009). Evaluation of atrial electromechanical delay and left atrial mechanical functions in patients with rheumatoid arthritis. Turk Kardiyol. Dern. Ars. 37, 447–453.
Agarwal, Y. K., Aronow, W. S., Levy, J. A., and Spodick, D. H. (2003). Association of interatrial block with development of atrial fibrillation. Am. J. Cardiol. 91, 882. doi: 10.1016/S0002-9149(03)00027-4
Aragno, M., Mastrocola, R., Medana, C., Catalano, M. G., Vercellinatto, I., Danni, O., et al. (2006). Oxidative stress-dependent impairment of cardiac-specific transcription factors in experimental diabetes. Endocrinology 147, 5967–5974. doi: 10.1210/en.2006-0728
Ariyarajah, V., Apiyasawat, S., Moorthi, R., and Spodick, D. H. (2006a). Potential clinical correlates and risk factors for interatrial block. Cardiology 105, 213–218. doi: 10.1159/000091642
Ariyarajah, V., Apiyasawat, S., Najjar, H., Mercado, K., Puri, P., and Spodick, D. H. (2007a). Frequency of interatrial block in patients with sinus rhythm hospitalized for stroke and comparison to those without interatrial block. Am. J. Cardiol. 99, 49–52. doi: 10.1016/j.amjcard.2006.07.060
Ariyarajah, V., Asad, N., Tandar, A., and Spodick, D. H. (2005). Interatrial block: pandemic prevalence, significance, and diagnosis. Chest 128, 970–975. doi: 10.1378/chest.128.2.970
Ariyarajah, V., Frisella, M. E., and Spodick, D. H. (2006b). Reevaluation of the criterion for interatrial block. Am. J. Cardiol. 98, 936–937. doi: 10.1016/j.amjcard.2006.04.036
Ariyarajah, V., Kranis, M., Apiyasawat, S., and Spodick, D. H. (2007b). Potential factors that affect electrocardiographic progression of interatrial block. Ann. Noninvasive Electrocardiol. 12, 21–26. doi: 10.1111/j.1542-474X.2007.00134.x
Ariyarajah, V., Puri, P., Apiyasawat, S., and Spodick, D. H. (2007c). Interatrial block: a novel risk factor for embolic stroke? Ann. Noninvasive Electrocardiol. 12, 15–20. doi: 10.1111/j.1542-474X.2007.00133.x
Asad, N., and Spodick, D. H. (2003). Prevalence of interatrial block in a general hospital population. Am. J. Cardiol. 91, 609–610. doi: 10.1016/S0002-9149(02)03320-9
Bachmann, G. (1916). The inter-auricular time interval. Am. J. Physiology – Legacy Content 41, 309–320.
Bachmann, G. (1941). The significance of splitting of the P-wave in the electrocardiogram*. Ann. Intern. Med. 14, 1702–1709. doi: 10.7326/0003-4819-14-9-1702
Baranchuk, A., and Bayes de Luna, A. (2015). The P-wave morphology: what does it tell us? Herzschrittmacherther. Elektrophysiol. 26, 192–199. doi: 10.1007/s00399-015-0385-3
Baranchuk, A., de Luna, A. B., and Breithardt, G. (2016). To the Editor— The role of advanced interatrial block pattern as a predictor of atrial fibrillation. Heart Rhythm 13, e87. doi: 10.1016/j.hrthm.2015.11.024
Barr, R. C., Plonsey, R., and Johnson, C. R. (2003). Membrane current from transmembrane potentials in complex core-conductor models. IEEE Trans. Biomed. Eng. 50, 405–411. doi: 10.1109/TBME.2003.809508
Bayes de Luna, A., Cladellas, M., Oter, R., Torner, P., Guindo, J., Marti, V., et al. (1988). Interatrial conduction block and retrograde activation of the left atrium and paroxysmal supraventricular tachyarrhythmia. Eur. Heart J. 9, 1112–1118.
Bayes de Luna, A., Fort de Ribot, R., Trilla, E., Julia, J., Garcia, J., Sadurni, J., et al. (1985). Electrocardiographic and vectorcardiographic study of interatrial conduction disturbances with left atrial retrograde activation. J. Electrocardiol. 18, 1–13. doi: 10.1016/S0022-0736(85)80029-7
Bayes de Luna, A., Guindo, J., Vinolas, X., Martinez-Rubio, A., Oter, R., and Bayes-Genis, A. (1999). Third-degree inter-atrial block and supraventricular tachyarrhythmias. Europace 1, 43–46. doi: 10.1053/eupc.1998.0006
Bayes de Luna, A., Oter, M. C., and Guindo, J. (1989). Interatrial conduction block with retrograde activation of the left atrium and paroxysmal supraventricular tachyarrhythmias: influence of preventive antiarrhythmic treatment. Int. J. Cardiol. 22, 147–150. doi: 10.1016/0167-5273(89)90061-2
Bayes de Luna, A., Platonov, P., Cosio, F. G., Cygankiewicz, I., Pastore, C., Baranowski, R., et al. (2012). Interatrial blocks. A separate entity from left atrial enlargement: a consensus report. J. Electrocardiol. 45, 445–451. doi: 10.1016/j.jelectrocard.2012.06.029
Beyer, E. C., Paul, D. L., and Goodenough, D. A. (1987). Connexin43: a protein from rat heart homologous to a gap junction protein from liver. J. Cell Biol. 105, 2621–2629. doi: 10.1083/jcb.105.6.2621
Bidasee, K. R., Nallani, K., Besch, H. R. Jr., and Dincer, U. D. (2003). Streptozotocin-induced diabetes increases disulfide bond formation on cardiac ryanodine receptor (RyR2). J. Pharmacol. Exp. Ther. 305, 989–998. doi: 10.1124/jpet.102.046201
Boineau, J. (2005). The prolonged P wave and interatrial block. Time to consider a broader concept and different terminology. J. Electrocardiol. 38, 327–329. doi: 10.1016/j.jelectrocard.2005.05.002
Budeus, M., Hennersdorf, M., Perings, C., Wieneke, H., Erbel, R., and Sack, S. (2005). Prediction of the recurrence of atrial fibrillation after successful cardioversion with P wave signal-averaged ECG. Ann. Noninvasive Electrocardiol. 10, 414–419. doi: 10.1111/j.1542-474X.2005.00059.x
Cagirci, G., Cay, S., Gulsoy, K. G., Bayindir, C., Vural, M. G., Firat, H., et al. (2011). Tissue Doppler atrial conduction times and electrocardiogram interlead P-wave durations with varying severity of obstructive sleep apnea. J. Electrocardiol. 44, 478–482. doi: 10.1016/j.jelectrocard.2011.03.009
Caldwell, J., Koppikar, S., Barake, W., Redfearn, D., Michael, K., Simpson, C., et al. (2014). Prolonged P-wave duration is associated with atrial fibrillation recurrence after successful pulmonary vein isolation for paroxysmal atrial fibrillation. J. Interv. Card. Electrophysiol. 39, 131–138. doi: 10.1007/s10840-013-9851-1
Can, I., Aytemir, K., Demir, A. U., Deniz, A., Ciftci, O., Tokgozoglu, L., et al. (2009). P-wave duration and dispersion in patients with obstructive sleep apnea. Int. J. Cardiol. 133, e85–e89. doi: 10.1016/j.ijcard.2007.11.037
Chen, W., Potse, M., and Vinet, A. (2007). Dynamics of sustained reentry in a loop model with discrete gap junction resistances. Phys. Rev. E Stat. Nonlin. Soft Matter Phys. 76:021928. doi: 10.1103/PhysRevE.76.021928
Chen, Z., Sun, B., Tse, G., Jiang, J., and Xu, W. (in press). Reversibility of both sinus node dysfunction and reduced HCN4 mRNA expression level in an atrial tachycardia pacing model of tachycardia-bradycardia syndrome in rabbit hearts. Int. J. Clin. Exp. Pathol. 9.
Chhabra, L., Devadoss, R., Chaubey, V. K., and Spodick, D. H. (2014). Interatrial block in the modern era. Curr. Cardiol. Rev. 10, 181–189. doi: 10.2174/1573403X10666140514101748
Chhabra, L., Srinivasan, I., Sareen, P., Anand, C., and Spodick, D. H. (2012). Interatrial block - a novel risk factor for acute mesenteric ischemia. Indian J. Gastroenterol. 31, 191–194. doi: 10.1007/s12664-012-0194-0
Choy, L., Yeo, J. M., Tse, V., Chan, S. P., and Tse, G. (2016). Cardiac disease and arrhythmogenesis: mechanistic insights from mouse models. Int. J. Cardiol. Heart Vasc. 12, 1–10. doi: 10.1016/j.ijcha.2016.05.005
Chung, D. K., and Chung, E. K. (1972). Post-ectopic inhibition phenomenon. W. V. Med. J. 68, 168–169.
Conde, D., and Baranchuk, A. (2014). Interatrial block as anatomical-electrical substrate for supraventricular arrhythmias: Bayes syndrome. Arch. Cardiol. Mex. 84, 32–40. doi: 10.1016/j.acmx.2013.10.004
Conde, D., Seoane, L., Gysel, M., Mitrione, S., Bayes de Luna, A., and Baranchuk, A. (2015). Bayes' syndrome: the association between interatrial block and supraventricular arrhythmias. Expert Rev. Cardiovasc. Ther. 13, 541–550. doi: 10.1586/14779072.2015.1037283
Cosio, F. G., Martin-Penato, A., Pastor, A., Nunez, A., Montero, M. A., Cantale, C. P., et al. (2004). Atrial activation mapping in sinus rhythm in the clinical electrophysiology laboratory: observations during Bachmann's bundle block. J. Cardiovasc. Electrophysiol. 15, 524–531. doi: 10.1046/j.1540-8167.2004.03403.x
Cox, E. J., and Marsh, S. A. (2014). A systematic review of fetal genes as biomarkers of cardiac hypertrophy in rodent models of diabetes. PLoS ONE 9:e92903. doi: 10.1371/journal.pone.0092903
Curran, J., and Louch, W. E. (2015). Linking ryanodine receptor Ca(2+) leak and Na(+) current in heart: a day in the life of flecainide. Acta Physiol. (Oxf). 214, 300–302. doi: 10.1111/apha.12526
D'Allonnes, G. R., Pavin, D., Leclercq, C., Ecke, J. E., Jauvert, G., Mabo, P., et al. (2000). Long-term effects of biatrial synchronous pacing to prevent drug-refractory atrial tachyarrhythmia: a nine-year experience. J. Cardiovasc. Electrophysiol. 11, 1081–1091. doi: 10.1111/j.1540-8167.2000.tb01752.x
Daubert, J. C., Pavin, D., Jauvert, G., and Mabo, P. (2004). Intra- and interatrial conduction delay: implications for cardiac pacing. Pacing Clin. Electrophysiol. 27, 507–525. doi: 10.1111/j.1540-8159.2004.00473.x
Dilaveris, P., Batchvarov, V., Gialafos, J., and Malik, M. (1999). Comparison of different methods for manual P wave duration measurement in 12-lead electrocardiograms. Pacing Clin. Electrophysiol. 22, 1532–1538. doi: 10.1111/j.1540-8159.1999.tb00358.x
Eager, K. R., Roden, L. D., and Dulhunty, A. F. (1997). Actions of sulfhydryl reagents on single ryanodine receptor Ca(2+)-release channels from sheep myocardium. Am. J. Physiol. 272, C1908–C1918.
Engelen, M. A., Juergens, K. U., Breithardt, G., and Eckardt, L. (2005). Interatrial conduction delay and atrioventricular block due to primary cardiac lymphoma. J. Cardiovasc. Electrophysiol. 16, 926. doi: 10.1046/j.1540-8167.2005.50003.x
Enriquez, A., Marano, M., D'Amato, A., de Luna, A. B., and Baranchuk, A. (2015). Second-degree interatrial block in hemodialysis patients. Case Rep. Cardiol. 2015:468493. doi: 10.1155/2015/468493
Fleg, J. L., and Strait, J. (2012). Age-associated changes in cardiovascular structure and function: a fertile milieu for future disease. Heart Fail. Rev. 17, 545–554. doi: 10.1007/s10741-011-9270-2
Fukui, A., Takahashi, N., Nakada, C., Masaki, T., Kume, O., Shinohara, T., et al. (2013). Role of leptin signaling in the pathogenesis of angiotensin II-mediated atrial fibrosis and fibrillation. Circ. Arrhythm. Electrophysiol. 6, 402–409. doi: 10.1161/CIRCEP.111.000104
Gan, R. Y., and Li, H. B. (2014). Recent progress on liver kinase B1 (LKB1): expression, regulation, downstream signaling and cancer suppressive function. Int. J. Mol. Sci. 15, 16698–16718. doi: 10.3390/ijms150916698
George, S. A., Sciuto, K. J., Lin, J., Salama, M. E., Keener, J. P., Gourdie, R. G., et al. (2015). Extracellular sodium and potassium levels modulate cardiac conduction in mice heterozygous null for the Connexin43 gene. Pflugers Arch. 467, 2287–2297. doi: 10.1007/s00424-015-1698-0
Gialafos, E., Psaltopoulou, T., Papaioannou, T. G., Synetos, A., Dilaveris, P., Andrikopoulos, G., et al. (2007). Prevalence of interatrial block in young healthy men < 35 years of age. Am. J. Cardiol. 100, 995–997. doi: 10.1016/j.amjcard.2007.04.041
Gourdie, R. G., Green, C. R., Severs, N. J., Anderson, R. H., and Thompson, R. P. (1993a). Evidence for a distinct gap-junctional phenotype in ventricular conduction tissues of the developing and mature avian heart. Circ. Res. 72, 278–289. doi: 10.1161/01.RES.72.2.278
Gourdie, R. G., Severs, N. J., Green, C. R., Rothery, S., Germroth, P., and Thompson, R. P. (1993b). The spatial distribution and relative abundance of gap-junctional connexin40 and connexin43 correlate to functional properties of components of the cardiac atrioventricular conduction system. J. Cell Sci. 105(Pt 4), 985–991.
Gramley, F., Lorenzen, J., Knackstedt, C., Rana, O. R., Saygili, E., Frechen, D., et al. (2009). Age-related atrial fibrosis. Age (Dordr) 31, 27–38. doi: 10.1007/s11357-008-9077-9
Hagendorff, A., Schumacher, B., Kirchhoff, S., Lüderitz, B., and Willecke, K. (1999). Conduction disturbances and increased atrial vulnerability in Connexin40-deficient mice analyzed by transesophageal stimulation. Circulation 99, 1508–1515. doi: 10.1161/01.CIR.99.11.1508
Hain, J., Onoue, H., Mayrleitner, M., Fleischer, S., and Schindler, H. (1995). Phosphorylation modulates the function of the calcium release channel of sarcoplasmic reticulum from cardiac muscle. J. Biol. Chem. 270, 2074–2081. doi: 10.1074/jbc.270.5.2074
Ito, Y., Shiga, K., Yoshida, K., Ogata, K., Kandori, A., Inaba, T., et al. (2014). Development of a magnetocardiography-based algorithm for discrimination between ventricular arrhythmias originating from the right ventricular outflow tract and those originating from the aortic sinus cusp: a pilot study. Heart Rhythm 11, 1605–1612. doi: 10.1016/j.hrthm.2014.05.032
Jairath, U. C., and Spodick, D. H. (2001). Exceptional prevalence of interatrial block in a general hospital population. Clin. Cardiol. 24, 548–550. doi: 10.1002/clc.4960240805
James, T. N. (1963). The connecting pathways between the Sinus node and a-V Node and between the right and the left atrium in the human heart. Am. Heart J. 66, 498–508. doi: 10.1016/0002-8703(63)90382-X
Jurkko, R., Mantynen, V., Tapanainen, J. M., Montonen, J., Vaananen, H., Parikka, H., et al. (2009). Non-invasive detection of conduction pathways to left atrium using magnetocardiography: validation by intra-cardiac electroanatomic mapping. Europace 11, 169–177. doi: 10.1093/europace/eun335
Kim, G. E., Ross, J. L., Xie, C., Su, K. N., Zaha, V. G., Wu, X., et al. (2015). LKB1 deletion causes early changes in atrial channel expression and electrophysiology prior to atrial fibrillation. Cardiovasc. Res. 108, 197–208. doi: 10.1093/cvr/cvv212
Kirchhof, P., Marijon, E., Fabritz, L., Li, N., Wang, W., Wang, T., et al. (2013). Overexpression of cAMP-response element modulator causes abnormal growth and development of the atrial myocardium resulting in a substrate for sustained atrial fibrillation in mice. Int. J. Cardiol. 166, 366–374. doi: 10.1016/j.ijcard.2011.10.057
Kléber, A. G., and Rudy, Y. (2004). Basic mechanisms of cardiac impulse propagation and associated arrhythmias. Physiol. Rev. 84, 431–488. doi: 10.1152/physrev.00025.2003
Kohl, P., and Gourdie, R. G. (2014). Fibroblast–myocyte electrotonic coupling: does it occur in native cardiac tissue? J. Mol. Cell. Cardiol. 70, 37–46. doi: 10.1016/j.yjmcc.2013.12.024
Kuijpers, N. H., Potse, M., van Dam, P. M., ten Eikelder, H. M., Verheule, S., Prinzen, F. W., et al. (2011). Mechanoelectrical coupling enhances initiation and affects perpetuation of atrial fibrillation during acute atrial dilation. Heart Rhythm 8, 429–436. doi: 10.1016/j.hrthm.2010.11.020
Kuroda, J., Ago, T., Matsushima, S., Zhai, P., Schneider, M. D., and Sadoshima, J. (2010). NADPH oxidase 4 (Nox4) is a major source of oxidative stress in the failing heart. Proc. Natl. Acad. Sci. U.S.A. 107, 15565–15570. doi: 10.1073/pnas.1002178107
Kwong, J. S., Leithauser, B., Park, J. W., and Yu, C. M. (2013). Diagnostic value of magnetocardiography in coronary artery disease and cardiac arrhythmias: a review of clinical data. Int. J. Cardiol. 167, 1835–1842. doi: 10.1016/j.ijcard.2012.12.056
Li, N., Chiang, D. Y., Wang, S., Wang, Q., Sun, L., Voigt, N., et al. (2014). Ryanodine receptor-mediated calcium leak drives progressive development of an atrial fibrillation substrate in a transgenic mouse model. Circulation 129, 1276–1285. doi: 10.1161/CIRCULATIONAHA.113.006611
Li, Y., Ma, J., Zhu, H., Singh, M., Hill, D., Greer, P. A., et al. (2011). Targeted inhibition of calpain reduces myocardial hypertrophy and fibrosis in mouse models of type 1 diabetes. Diabetes 60, 2985–2994. doi: 10.2337/db10-1333
Lorbar, M., Levrault, R., Phadke, J. G., and Spodick, D. H. (2005). Interatrial block as a predictor of embolic stroke. Am. J. Cardiol. 95, 667–668. doi: 10.1016/j.amjcard.2004.10.059
Maeno, K., Kasai, T., Kasagi, S., Kawana, F., Ishiwata, S., Ohno, M., et al. (2013). Relationship between atrial conduction delay and obstructive sleep apnea. Heart Vessels 28, 639–645. doi: 10.1007/s00380-012-0288-8
Magnani, J. W., Gorodeski, E. Z., Johnson, V. M., Sullivan, L. M., Hamburg, N. M., Benjamin, E. J., et al. (2011). P wave duration is associated with cardiovascular and all-cause mortality outcomes: the National Health and Nutrition Examination Survey. Heart Rhythm 8, 93–100. doi: 10.1016/j.hrthm.2010.09.020
Magnani, J. W., Johnson, V. M., Sullivan, L. M., Lubitz, S. A., Schnabel, R. B., Ellinor, P. T., et al. (2010). P-wave indices: derivation of reference values from the Framingham Heart Study. Ann. Noninvasive Electrocardiol. 15, 344–352. doi: 10.1111/j.1542-474X.2010.00390.x
Martinez-Selles, M., Fernandez Lozano, I., Baranchuk, A., Bayes-Genis, A., and Bayes de Luna, A. (2016a). Should we anticoagulate patients at high risk of atrial fibrillation? Rev. Esp. Cardiol. (Engl. Ed). 69, 374–376. doi: 10.1016/j.rec.2016.01.008
Martinez-Selles, M., Masso-van Roessel, A., Alvarez-Garcia, J., Garcia de la Villa, B., Cruz-Jentoft, A. J., Vidan, M. T., et al. (2016b). Interatrial block and atrial arrhythmias in centenarians: prevalence, associations, and clinical implications. Heart Rhythm 13, 645–651. doi: 10.1016/j.hrthm.2015.10.034
Mehrzad, R., and Spodick, D. H. (2014). Interatrial block: a virtual pandemic requiring attention. Iran. J. Med. Sci. 39, 84–93.
Mizuno, R., Fujimoto, S., Nakano, H., Nakajima, T., Kimura, A., Nakagawa, Y., et al. (1999). Atrial involvement in patients with progressive systemic sclerosis: relationship between ultrasonic tissue characterization of the atrium and interatrial conduction. Cardiology 91, 134–139. doi: 10.1159/000006893
Murugan, D., Lau, Y. S., Lau, W. C., Mustafa, M. R., and Huang, Y. (2015). Angiotensin 1-7 protects against angiotensin II-induced endoplasmic reticulum stress and endothelial dysfunction via mas receptor. PLoS ONE 10:e0145413. doi: 10.1371/journal.pone.0145413
Myrianthefs, M. M., Ellestad, M. H., Startt-Selvester, R. H., and Crump, R. (1991). Significance of signal-averaged P-wave changes during exercise in patients with coronary artery disease and correlation with angiographic findings. Am. J. Cardiol. 68, 1619–1624. doi: 10.1016/0002-9149(91)90319-G
Nattel, S. (2008). Effects of heart disease on cardiac ion current density versus current amplitude: important conceptual subtleties in the language of arrhythmogenic ion channel remodeling. Circ. Res. 102, 1298–1300. doi: 10.1161/CIRCRESAHA.108.178087
Okutucu, S., Evranos, B., Aytemir, K., Kaya, E. B., Deveci, O. S., Deniz, A., et al. (2010). Relationship between atrial septal aneurysms and atrial electromechanical delay. Int. J. Cardiovasc. Imaging 27, 505–513. doi: 10.1007/s10554-010-9700-6
Peyrou, J., Park, C. I., Cikirikcioglu, M., Shah, D., and Muller, H. (2013). A rare cause of Bachmann interatrial block. Eur. Heart J. Cardiovasc. Imaging 14, 1131. doi: 10.1093/ehjci/jet099
Proietti, R., Mafrici, A., and Spodick, D. H. (2012). Dynamic variations of P-wave duration in a patient with acute decompensated congestive heart failure. Cardiol. J. 19, 95–97. doi: 10.5603/CJ.2012.0017
Qin, M., Huang, H., Wang, T., Hu, H., Liu, Y., Gu, Y., et al. (2012). Atrial tachyarrhythmia in Rgs5-null mice. PLoS ONE 7:e46856. doi: 10.1371/journal.pone.0046856
Racker, D. K. (1989). Atrioventricular node and input pathways: a correlated gross anatomical and histological study of the canine atrioventricular junctional region. Anat. Rec. 224, 336–354. doi: 10.1002/ar.1092240303
Rhett, J. M., and Gourdie, R. G. (2012). The perinexus: a new feature of Cx43 gap junction organization. Heart Rhythm 9, 619–623. doi: 10.1016/j.hrthm.2011.10.003
Rhett, J. M., Veeraraghavan, R., Poelzing, S., and Gourdie, R. G. (2013). The perinexus: sign-post on the path to a new model of cardiac conduction? Trends Cardiovasc. Med. 23, 222–228. doi: 10.1016/j.tcm.2012.12.005
Rocken, C., Peters, B., Juenemann, G., Saeger, W., Klein, H. U., Huth, C., et al. (2002). Atrial amyloidosis: an arrhythmogenic substrate for persistent atrial fibrillation. Circulation 106, 2091–2097. doi: 10.1161/01.CIR.0000034511.06350.DF
Rohr, S. (2012). Arrhythmogenic implications of fibroblast-myocyte interactions. Circulation Arrhythm. Electrophysiol. 5, 442–452. doi: 10.1161/circep.110.957647
Rohr, S., Kucera, J. P., and Kleber, A. G. (1998). Slow conduction in cardiac tissue, I: effects of a reduction of excitability versus a reduction of electrical coupling on microconduction. Circ. Res. 83, 781–794. doi: 10.1161/01.RES.83.8.781
Sato, Y., Yoshida, K., Ogata, K., Inaba, T., Tada, H., Sekiguchi, Y., et al. (2012). An increase in right atrial magnetic strength is a novel predictor of recurrence of atrial fibrillation after radiofrequency catheter ablation. Circ. J. 76, 1601–1608. doi: 10.1253/circj.CJ-11-1419
Shang, L. L., and Dudley, S. C. Jr. (2005). Tandem promoters and developmentally regulated 5′- and 3′-mRNA untranslated regions of the mouse Scn5a cardiac sodium channel. J. Biol. Chem. 280, 933–940. doi: 10.1074/jbc.M409977200
Shang, L. L., Sanyal, S., Pfahnl, A. E., Jiao, Z., Allen, J., Liu, H., et al. (2008). NF-kappaB-dependent transcriptional regulation of the cardiac scn5a sodium channel by angiotensin II. Am. J. Physiol. Cell Physiol. 294, C372–C379. doi: 10.1152/ajpcell.00186.2007
Smeets, J. L., Allessie, M. A., Lammers, W. J., Bonke, F. I., and Hollen, J. (1986). The wavelength of the cardiac impulse and reentrant arrhythmias in isolated rabbit atrium. The role of heart rate, autonomic transmitters, temperature, and potassium. Circ Res. 58, 96–108. doi: 10.1161/01.RES.58.1.96
Song, J., Kalus, J. S., Caron, M. F., Kluger, J., and White, C. M. (2002). Effect of diuresis on P-wave duration and dispersion. Pharmacotherapy 22, 564–568. doi: 10.1592/phco.22.8.564.33213
Sovari, A. A., Rutledge, C. A., Jeong, E. M., Dolmatova, E., Arasu, D., Liu, H., et al. (2013). Mitochondria oxidative stress, connexin43 remodeling, and sudden arrhythmic death. Circ. Arrhythm. Electrophysiol. 6, 623–631. doi: 10.1161/CIRCEP.112.976787
Spach, M. S., and Josephson, M. E. (1994). Initiating reentry: the role of nonuniform anisotropy in small circuits. J. Cardiovasc. Electrophysiol. 5, 182–209. doi: 10.1111/j.1540-8167.1994.tb01157.x
Steinhoff, U., Knappe-Grueneberg, S., Schnabel, A., Trahms, L., Smith, F., Langley, P., et al. (2004). Magnetocardiography for pharmacology safety studies requiring high patient throughput and reliability. J. Electrocardiol. 37(Suppl.), 187–192. doi: 10.1016/j.jelectrocard.2004.08.055
Szili-Torok, T., Akca, F., Caliskan, K., Ten Cate, F., Theuns, D., and Michels, M. (2014). Extreme interatrial conduction delay and regularization of atrial arrhythmias in a subgroup of patients with hypertrophic cardiomyopathy. IJC Heart Vessels 4, 46–52.
Tapanainen, J. M., Jurkko, R., Holmqvist, F., Husser, D., Kongstad, O., Makijarvi, M., et al. (2009). Interatrial right-to-left conduction in patients with paroxysmal atrial fibrillation. J. Interv. Card. Electrophysiol. 25, 117–122. doi: 10.1007/s10840-008-9359-2
Thilen, U., Carlson, J., Platonov, P. G., Havmoller, R., and Olsson, S. B. (2007). Prolonged P wave duration in adults with secundum atrial septal defect: a marker of delayed conduction rather than increased atrial size? Europace 9(Suppl. 6), vi105–vi108. doi: 10.1093/europace/eum214
Thomas, S. P., Kucera, J. P., Bircher-Lehmann, L., Rudy, Y., Saffitz, J. E., and Kleber, A. G. (2003). Impulse propagation in synthetic strands of neonatal cardiac myocytes with genetically reduced levels of connexin43. Circ. Res. 92, 1209–1216. doi: 10.1161/01.RES.0000074916.41221.EA
Thomsen, M. B., and Calloe, K. (2016). Human atrial fibroblasts and their contribution to supraventricular arrhythmia. Physiol. Rep. 4:e12711. doi: 10.14814/phy2.12711
Tse, G. (2015). Mechanisms of cardiac arrhythmias. J Arrhythm. 32, 75–81. doi: 10.1016/j.joa.2015.11.003
Tse, G. (2016a). Both transmural dispersion of repolarization and transmural dispersion of refractoriness are poor predictors of arrhythmogenicity: a role for the index of Cardiac Electrophysiological Balance (QT/QRS)? J. Geriatr. Cardiol.
Tse, G. (2016b). Novel conduction-repolarization indices for the stratification of arrhythmic risk. J. Geriatr. Cardiol.
Tse, G. (2016c). (Tpeak-Tend)/QRS and (Tpeak-Tend)/(QT x QRS): novel markers for predicting arrhythmic risk in Brugada syndrome. Europace.
Tse, G., Ali, A., Alpendurada, F., Prasad, S., Raphael, C. E., and Vassiliou, V. (2015a). Tuberculous constrictive pericarditis. Res. Cardiovasc. Med. 4:e29614. doi: 10.5812/cardiovascmed.29614
Tse, G., Ali, A., Prasad, S. K., Vassiliou, V., and Raphael, C. E. (2015b). Atypical case of post-partum cardiomyopathy: an overlap syndrome with arrhythmogenic right ventricular cardiomyopathy? BJR Case Rep. 1:20150182. doi: 10.1259/bjrcr.20150182
Tse, G., Hothi, S. S., Grace, A. A., and Huang, C. L. (2012). Ventricular arrhythmogenesis following slowed conduction in heptanol-treated, Langendorff-perfused mouse hearts. J. Physiol. Sci. 62, 79–92. doi: 10.1007/s12576-011-0187-2
Tse, G., Lai, E. T., Tse, V., and Yeo, J. M. (2016a). Molecular and electrophysiological mechanisms underlying cardiac arrhythmogenesis in diabetes mellitus. J. Diabetes Res.
Tse, G., Lai, T. H., Yeo, J. M., Tse, V., and Wong, S. H. (2016b). Mechanisms of electrical activation and conduction in the gastrointestinal system: lessons from cardiac electrophysiology. Front. Physiol. 7:182. doi: 10.3389/fphys.2016.00182
Tse, G., Sun, B., Wong, S. T., Tse, V., and Yeo, J. M. (2016c). Ventricular anti-arrhythmic effects of hypercalcaemia treatment in hyperkalaemic, Langendorff-perfused mouse hearts. Biomed Rep. doi: 10.3892/br.2016.577
Tse, G., Tse, V., and Yeo, J. M. (2016d). Ventricular anti-arrhythmic effects of heptanol in hypokalaemic, Langendorff-perfused mouse hearts. Biomed Rep. 4, 313–324. doi: 10.3892/br.2016.577
Tse, G., Tse, V., Yeo, J. M., and Sun, B. (2016e). Atrial anti-arrhythmic effects of heptanol in Langendorff-perfused mouse hearts. PLoS ONE 11:e0148858. doi: 10.1371/journal.pone.0148858
Tse, G., Wong, S. T., Tse, V., Lee, Y. T., Lin, H. Y., and Yeo, J. M. (2016f). Cardiac dynamics: alternans and arrhythmogenesis. J. Arrhythm. doi: 10.1016/j.joa.2016.02.009
Tse, G., Wong, S. T., Tse, V., and Yeo, J. M. (2016g). Depolarization vs.repolarization: what is the mechanism of ventricular arrhythmogenesis underlying sodium channel haploinsufficiency in mouse hearts? Acta Physiol. (Oxf). doi: 10.1111/apha.12694. [Epub ahead of print].
Tse, G., Wong, S. T., Tse, V., and Yeo, J. M. (in press). Determination of action potential wavelength restitution in Scn5a+/− mouse hearts modelling human Brugada syndrome. J. Physiol.
Tse, G., Wong, S. T., Tse, V., and Yeo, J. M. (2016h). Monophasic action potential recordings: which is the recording electrode? J. Basic Clin. Physiol. Pharmacol. doi: 10.1515/jbcpp-2016-0007. [Epub ahead of print].
Tse, G., Wong, S. T., Tse, V., and Yeo, J. M. (2016i). Restitution analysis of alternans using dynamic pacing and its comparison with S1S2 restitution in heptanol-treated, hypokalaemic Langendorff-perfused mouse hearts. Biomed Rep. 4, 673–680. doi: 10.3892/br.2016.659
Tse, G., and Yan, B. P. (2016). Novel arrhythmic risk markers incorporating QRS dispersion: QRSd x (Tpeak-Tend) / QRS and QRSd x (Tpeak-Tend)/(QT x QRS). Ann. Noninvasive Electrocardiol.
Tse, G., and Yeo, J. M. (2015). Conduction abnormalities and ventricular arrhythmogenesis: the roles of sodium channels and gap junctions. Int. J. Cardiol. Heart Vasc. 9, 75–82. doi: 10.1016/j.ijcha.2015.10.003
Vaidya, D., Morley, G. E., Samie, F. H., and Jalife, J. (1999). Reentry and fibrillation in the mouse heart. A challenge to the critical mass hypothesis. Circ. Res. 85, 174–181. doi: 10.1161/01.RES.85.2.174
van Rijen, H. V., van Veen, T. A., van Kempen, M. J., Wilms-Schopman, F. J., Potse, M., Krueger, O., et al. (2001). Impaired conduction in the bundle branches of mouse hearts lacking the gap junction protein connexin40. Circulation 103, 1591–1598. doi: 10.1161/01.CIR.103.11.1591
Vassiliou, V., Chin, C., Perperoglou, A., Tse, G., Ali, A., Raphael, C., et al. (2014). 93 ejection fraction by cardiovascular magnetic resonance predicts adverse outcomes post aortic valve replacement. Heart 100, A53–A54. doi: 10.1136/heartjnl-2014-306118.93
Veeraraghavan, R., Lin, J., Hoeker, G. S., Keener, J. P., Gourdie, R. G., and Poelzing, S. (2015). Sodium channels in the Cx43 gap junction perinexus may constitute a cardiac ephapse: an experimental and modeling study. Pflugers Arch. 467, 2093–2105. doi: 10.1007/s00424-014-1675-z
Veeraraghavan, R., Poelzing, S., and Gourdie, R. G. (2014a). Intercellular electrical communication in the heart: a new, active role for the intercalated disk. Cell Commun. Adhes. 21, 161–167. doi: 10.3109/15419061.2014.905932
Veeraraghavan, R., Poelzing, S., and Gourdie, R. G. (2014b). Novel ligands for zipping and unzipping the intercalated disk: today's experimental tools, tomorrow's therapies? Cardiovasc. Res. 104, 229–230. doi: 10.1093/cvr/cvu216
Veeraraghavan, R., Poelzing, S., and Gourdie, R. G. (2014c). Old cogs, new tricks: a scaffolding role for connexin43 and a junctional role for sodium channels? FEBS Lett. 588, 1244–1248. doi: 10.1016/j.febslet.2014.01.026
Veeraraghavan, R., Salama, M. E., and Poelzing, S. (2012). Interstitial volume modulates the conduction velocity-gap junction relationship. Am. J. Physiol. Heart Circ. Physiol. 302, H278–H286. doi: 10.1152/ajpheart.00868.2011
Verheule, S., Sato, T., Everett, T. IV, Engle, S. K., Otten, D., Rubart-von der Lohe, M., et al. (2004). Increased vulnerability to atrial fibrillation in transgenic mice with selective atrial fibrosis caused by overexpression of TGF-beta1. Circ. Res. 94, 1458–1465. doi: 10.1161/01.RES.0000129579.59664.9d
Verheule, S., van Batenburg, C. A., Coenjaerts, F. E., Kirchhoff, S., Willecke, K., and Jongsma, H. J. (1999). Cardiac conduction abnormalities in mice lacking the gap junction protein connexin40. J. Cardiovasc. Electrophysiol. 10, 1380–1389. doi: 10.1111/j.1540-8167.1999.tb00194.x
Vigmond, E. J. (2005). The electrophysiological basis of MAP recordings. Cardiovasc. Res. 68, 502–503. doi: 10.1016/j.cardiores.2005.07.020
Vigmond, E. J., and Leon, L. J. (1999). Electrophysiological basis of mono-phasic action potential recordings. Med. Biol. Eng. Comput. 37, 359–365. doi: 10.1007/BF02513313
Vigmond, E. J., Tsoi, V., Yin, Y., Page, P., and Vinet, A. (2009). Estimating atrial action potential duration from electrograms. IEEE Trans. Biomed. Eng. 56, 1546–1555. doi: 10.1109/TBME.2009.2014740
Vollmann, D., Blaauw, Y., Neuberger, H.-R., Schotten, U., and Allessie, M. (2005). Long-term changes in sequence of atrial activation and refractory periods: No evidence for “atrial memory”. Heart Rhythm 2, 155–161. doi: 10.1016/j.hrthm.2004.11.013
Wehrens, X. H., Lehnart, S. E., Reiken, S. R., and Marks, A. R. (2004). Ca2+/calmodulin-dependent protein kinase II phosphorylation regulates the cardiac ryanodine receptor. Circ. Res. 94, e61–e70. doi: 10.1161/01.RES.0000125626.33738.E2
Wei, L. H., Huang, X. R., Zhang, Y., Li, Y. Q., Chen, H. Y., Heuchel, R., et al. (2013). Deficiency of Smad7 enhances cardiac remodeling induced by angiotensin II infusion in a mouse model of hypertension. PLoS ONE 8:e70195. doi: 10.1371/journal.pone.0070195
Wiener, N., and Rosenblueth, A. (1946). The mathematical formulation of the problem of conduction of impulses in a network of connected excitable elements, specifically in cardiac muscle. Arch. Inst. Cardiol. Mex. 16, 205–265.
Willems, J. L., Robles de Medina, E. O., Bernard, R., Coumel, P., Fisch, C., Krikler, D., et al. (1985). Criteria for intraventricular conduction disturbances and pre-excitation. World Health Organizational/International Society and Federation for Cardiology Task Force Ad Hoc. J. Am. Coll. Cardiol. 5, 1261–1275. doi: 10.1016/S0735-1097(85)80335-1
Witcher, D. R., Kovacs, R. J., Schulman, H., Cefali, D. C., and Jones, L. R. (1991). Unique phosphorylation site on the cardiac ryanodine receptor regulates calcium channel activity. J. Biol. Chem. 266, 11144–11152.
Xu, L., Eu, J. P., Meissner, G., and Stamler, J. S. (1998). Activation of the cardiac calcium release channel (ryanodine receptor) by poly-S-nitrosylation. Science 279, 234–237. doi: 10.1126/science.279.5348.234
Yoshida, K., Ogata, K., Inaba, T., Nakazawa, Y., Ito, Y., Yamaguchi, I., et al. (2015). Ability of magnetocardiography to detect regional dominant frequencies of atrial fibrillation. J. Arrhythm. 31, 345–351. doi: 10.1016/j.joa.2015.05.003
Zhang, H., Liu, J., Qu, D., Wang, L., Luo, J. Y., Lau, C. W., et al. (2016). Inhibition of miR-200c restores endothelial function in diabetic mice through suppression of COX-2. Diabetes 65, 1196–1207. doi: 10.2337/db15-1067
Zhang, Y., Liu, J., Luo, J. Y., Tian, X. Y., Cheang, W. S., Xu, J., et al. (2015). Upregulation of angiotensin (1-7)-mediated signaling preserves endothelial function through reducing oxidative stress in diabetes. Antioxid. Redox Signal. 23, 880–892. doi: 10.1089/ars.2014.6070
Zibadi, S., Cordova, F., Slack, E. H., Watson, R. R., and Larson, D. F. (2011). Leptin's regulation of obesity-induced cardiac extracellular matrix remodeling. Cardiovasc. Toxicol. 11, 325–333. doi: 10.1007/s12012-011-9124-0
Keywords: Bayés syndrome, inter-atrial block, intra-atrial block, conduction, electrophysiological remodeling, structural remodeling
Citation: Tse G, Lai ETH, Yeo JM and Yan BP (2016) Electrophysiological Mechanisms of Bayés Syndrome: Insights from Clinical and Mouse Studies. Front. Physiol. 7:188. doi: 10.3389/fphys.2016.00188
Received: 31 March 2016; Accepted: 10 May 2016;
Published: 31 May 2016.
Edited by:
Mark Potse, Inria Bordeaux Sud-Ouest, FranceReviewed by:
Jan Pavel Kucera, University of Bern, SwitzerlandAdrian Marco Baranchuk, Queen's University, Canada
Copyright © 2016 Tse, Lai, Yeo and Yan. This is an open-access article distributed under the terms of the Creative Commons Attribution License (CC BY). The use, distribution or reproduction in other forums is permitted, provided the original author(s) or licensor are credited and that the original publication in this journal is cited, in accordance with accepted academic practice. No use, distribution or reproduction is permitted which does not comply with these terms.
*Correspondence: Gary Tse, Z2FyeS50c2VAZG9jdG9ycy5vcmcudWs=;
Bryan P. Yan, YnJ5YW4ueWFuQGN1aGsuZWR1Lmhr