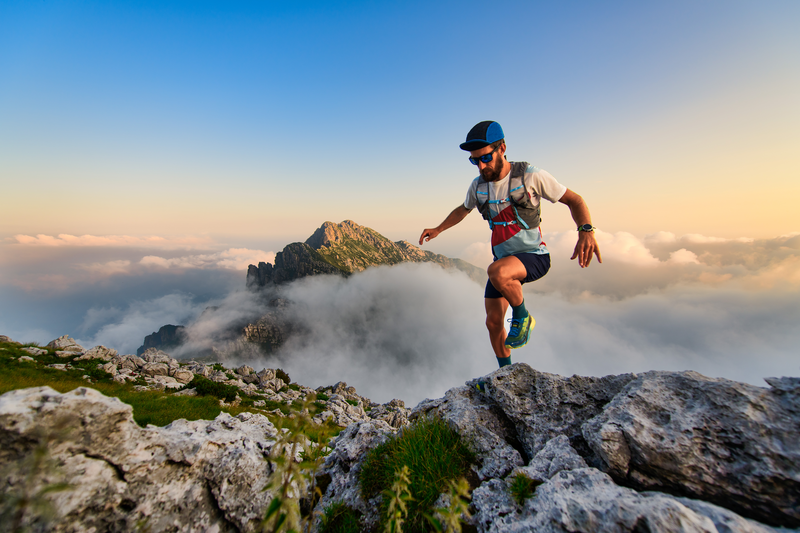
95% of researchers rate our articles as excellent or good
Learn more about the work of our research integrity team to safeguard the quality of each article we publish.
Find out more
REVIEW article
Front. Physiol. , 24 February 2016
Sec. Craniofacial Biology and Dental Research
Volume 7 - 2016 | https://doi.org/10.3389/fphys.2016.00062
This article is part of the Research Topic Dental and periodontal tissues formation and regeneration: Current approaches and future challenges View all 26 articles
Craniofacial area represent a unique district of human body characterized by a very high complexity of tissues, innervation and vascularization, and being deputed to many fundamental function such as eating, speech, expression of emotions, delivery of sensations such as taste, sight, and earing. For this reasons, tissue loss in this area following trauma or for example oncologic resection, have a tremendous impact on patients' quality of life. In the last 20 years regenerative medicine has emerged as one of the most promising approach to solve problem related to trauma, tissue loss, organ failure etc. One of the most powerful tools to be used for tissue regeneration is represented by stem cells, which have been successfully implanted in different tissue/organs with exciting results. Nevertheless, both autologous and allogeneic stem cell transplantation raise many practical and ethical concerns that make this approach very difficult to apply in clinical practice. For this reason different cell free approaches have been developed aiming to the mobilization, recruitment, and activation of endogenous stem cells into the injury site avoiding exogenous cells implant but instead stimulating patients' own stem cells to repair the lesion. To this aim many strategies have been used including functionalized bioscaffold, controlled release of stem cell chemoattractants, growth factors, BMPs, Platelet–Rich-Plasma, and other new strategies such as ultrasound wave and laser are just being proposed. Here we review all the current and new strategies used for activation and mobilization of endogenous stem cells in the regeneration of craniofacial tissue.
Regenerative medicine is the field of translational research that aims to replace and repair cells, tissues and organs to restore their normal functions (Mason and Dunnill, 2008). In the last 20 years, the possibility to use endogenous and exogenous stem cells for tissue repair has emerged producing enthusiasm in the scientific and medical community, but on the other side, raising a series of ethical and practical issues. The craniomaxillofacial complex is of fundamental importance for many different functions, such as breathing and eating, others non-vital but still important for social relationships, such as aesthetics and the delivery of senses such as sight, smell, and sound. For these reasons, craniomaxillofacial tissue damages have serious physiological and psychological consequences, and it is of paramount importance to seek solutions to optimize the life of people with maxillofacial trauma (Teo and Vallier, 2010). Many disciplines, such as biology, medicine, chemistry, and engineering, are involved in the study and development of techniques or products that regenerate the native conditions of such a complicated body region as the craniofacial tissues such as bone, muscle, cartilage and nervous tissue. The research moved toward two main different directions: one that involves the study of stem cell biology, including the development of novel techniques to isolate, characterize and transplant stem cells, the other related to the material science, which aims to the creation of new biomaterials and technologies suitable for the use with stem cells. As for stem cell biology, the research focused on stem and progenitor cells isolation, characterization, expansion, and transplantation for replacing damaged tissues (Giuliani et al., 2013) and on the study of growth-factors and genetic technology to control proliferation, migration and ability in tissue repair and regeneration (Alvarez et al., 2012; Han et al., 2014).
Many types of stem/progenitor cells have been described and proposed for their ability to rescue and repair injured tissue and partially restore organ function. Stem cells are undifferentiated cells capable to undergo an indefinite number of replications (self-renewal) and give rise to progenitor and finally to specialized cells. Therefore, stem cells differ from other types of cells in the body because they are capable of sustaining self-renewal, are unspecialized, and can give rise to differentiated cell types (La Noce et al., 2014a). In the field of regenerative medicine, two main types of stem cells are used: Embryonic stem cells (ESCs) and Adult stem cells (ASCs). The embryonic stem cells are isolated from the inner cell mass (ICM) of the blastocyst, they are able to differentiate in all the tissues deriving from the three definitive germ layers, and for this reason they are considered “pluripotent.” Adult stem cells reside in the adult tissues and they are pivotal for the tissue homeostasis (Mohanty et al., 2015); unlike ESCs, these cells are only able to differentiate into a limited group of cells types, and they are considered “multipotent.” In the recent years a new source of stem cells for regenerative and translational medicine has been created by transfecting adult cells (mainly dermal fibroblasts) with a varying number of stem-associated genes producing the so-called induced Pluripotent Stem Cells (iPSCs) (Takahashi et al., 2007). In this review, only Adult Stem Cells (ASCs) or cell-free approaches in regenerative medicine will be considered. Today, multiple sources for the isolation of adult stem cells have been identified, including heart tissue (Warejcka et al., 1996), umbilical cord blood (Campagnoli et al., 2001), skeletal muscle (Wada et al., 2002), and the dermis of skin (Toma et al., 2001). To identify a suitable cell population for craniofacial regeneration, many odontogenic stem cells, including dental pulp stem cells (Gronthos et al., 2000; Laino et al., 2005; d'Aquino et al., 2007, 2011), periodontal ligament stem cells (Seo et al., 2004), stem cells from human exfoliated deciduous teeth (Cordeiro et al., 2008), and stem cells from apical papilla (Sonoyama et al., 2008) and some non-odontogenic stem cells, including adipose-derived stem cells (Hung et al., 2011), bone marrow mesenchymal stem cells (Li et al., 2007), gingival stem cells (Hakkinen et al., 2014), embryonic stem cells (Ohazama et al., 2004), neural crest cells (Jiang et al., 2008), and even hair follicle stem cells (Wu et al., 2009) have been selected for screening. Studies have demonstrated that all odontogenic stem cells have a certain degree of multipotency in vitro and form pulp-dentin complexes combined with scaffold materials in vivo, except for PDLSCs, which tend to form bone-like tissues in vivo (Mangano et al., 2011; La Noce et al., 2014b; Naddeo et al., 2015). In contrast, non-odontogenic stem cells other than BMSCs have not yet been confirmed to have the potential for tooth regeneration. Among these cell types, postnatal DPSCs have the most potential as stem cells for endodontic tissue regeneration (Gronthos et al., 2002; Nakashima et al., 2004, 2009; Laino et al., 2005; Murray et al., 2007; Mangano et al., 2011; Paino et al., 2014; Naddeo et al., 2015). Nevertheless there is the need for craniofacial regeneration to repair tissue such as bone and soft tissue such as adipose tissue and among the different sources of stem cells bone marrow and adipose tissue seem to be the most promising. For what concerns material science, this constitutes one of the subjects most widely studied in recent years for its application in many fields of medicine, such as tissue engineering and regenerative medicine. The real paradigm shift that took place in the last years is the overlap of the material science with the biology, in an attempt to create new materials—also defined as smart materials or biomaterials—that do not only exist as passive support to living cells, but that are also able to interact and respond sensitively to environmental cues derived from these cells (Mangano et al., 2011). Moreover, the increasing understanding of the natural mechanisms involved in the development of tissues and organs has led to the creation of three-dimensional in vitro bioreactors, including nanotechnology and microfluidics-based bioreactors that recapitulate normal and pathological tissue development, structure and function (Rajan et al., 2014; Obregon et al., 2015) and pave the way to the idea of “printed organ,” a new technique used for fabrication of organ-like constructs (Choi and Kim, 2015; Ledford, 2015). These technologies have been made possible thanks to the mind-shift that has brought scientists to create synthetic matrices that recapitulate the natural properties of the extracellular matrix (ECM) of the different tissues, as a direct consequence of the evidences that the composition of the scaffold is able to influence stem cell proliferation, differentiation and angiogenesis through integrin signaling (Pittenger et al., 1999; Hynes, 2002a,b).
In last years scientific findings highlighted the importance of human cell therapy. In regenerative medicine stem cells can be used from donor that is a family member (allogeneic by family), donor unrelated (allogeneic cells from unrelated volunteer) or the patient's own (autologous). Stem cell commonly used in the therapy for craniofacial region are bone marrow stem cells(Yang et al., 2014), adipose stem cells (Griffin et al., 2014) hematopoietic stem cell (Vesterbacka et al., 2012) dental pulp stem cells (Giuliani et al., 2013). Both allogeneic and autologous cell therapies may be subdivided into two types: cell therapy for acute or for chronic conditions, the first to limit the natural progression of disease, the second for full regeneration (Sadan et al., 2008; Carpenter et al., 2009). Those approaches can be exploited in many clinical situations; therefore it is important that advantages and disadvantages of both therapies are made clear.
Allogeneic cells represent a therapeutic technology that fits easily in pharmaceutical production. So it is more efficient to produce cells from multiple patients and introduce them into a conventional quality control (QC) system, where it is ascertained the safety of the product and its characterization from the biological point of view. As autologous cells, allogeneic cells are expandable in culture, thus allowing the production of numerous batches of material and therefore their distribution on a large scale. This represents a great potential for economic development, promoting the improvement and expansion of this therapy (Mason and Dunnill, 2008). In medical emergencies the allogeneic cells have many advantages due to the rapid availability of these cells, which guarantee a good stability during storage and already occurred characterization. It is also not necessary to perform a biopsy on a person potentially sick, avoiding additional stress to the patient and saving time.
The most common problem in allogeneic cell transplantation is related to the host immune response that, directed against grafted cells, can lead to strong inflammatory reaction and consequent destruction of the graft (Barker and Widner, 2004). Nevertheless, some types of stem cells as MSCs have shown immunomodulatory activity in some autoimmune disorders, such as graft-versus-host disease and systemic lupus (Sui et al., 2013; Zhao et al., 2015), but this feature seem to not be sufficient or to not apply to grafted stem cells. In fact different strategies have been proposed to overcome this problem as discussed by Guha et al. (2013). Another major problem arising with allograft is the risk of generating tumors in the host. Transplanted cells can trigger a mechanism of crosstalk with the microenvironment of the receiver, which, combined with the stem cells growth capacity, brings along the risk of uncontrolled growth after transplantation. As an example Amariglio et al. described the generation of brain benign tumor after stem cells transplantation in a patient affected by ataxia telangiectasia (Amariglio et al., 2009). This problem can also be explained by the fact that in vitro expanded stem cells bring increased genetic abnormalities, which can favor cancer initiation (Spits et al., 2008). In fact, before use for allogeneic transplantation, stem cells are extensively cultured, and the accumulation of genomic abnormalities may represent a risk to the recipient. Another issue in derivation and culturing stem cells for allogeneic transplantation is the use of animal products in cell culture (Foetal Bovine Serum for instance), increasing risk of graft rejection and transmission of zoonosis (Chavez et al., 2008).
The greatest advantage in the use of autologous cells is definitely to avoid immunological responses host-versus-graft. Furthermore during therapy with autologous cells you can prevent the immunosuppressive treatment on the patient, avoiding the risk of infections and lowering by far the costs of the therapy (Chen and Palmer, 2008). In bioaesthetic treatment this approach seems to be most suitable for the less intrusive therapies and look more natural given by the patient's own cells (Tsai et al., 2000). In the autograft, the cells used are isolated from the donor-patient and when the number of cells obtained by biopsy is sufficient for transplantation it is not necessary to amplify, avoiding the anomalies due to an excessive growth in vitro (Baker et al., 2007). In addition, they are not exposed to products of animal origin, removing from the risk of zoonosis. Finally, the patient-derived cells do not incur ethical battles and regulation, therefore their use is much simpler than that of allografts.
One of the major issues concerning the autograft is the limitation of material to engage in certain patients, such as children under 6 years of age or with particular diseases (Smith et al., 2011). In addition, craniofacial reconstruction may be even more difficult in the pediatric patient because the skull is not developed enough so the split-thickness bone grafting is not tolerated (Chenard et al., 2012).
Another complication is the side effects that occur in the donor site, which involves hematoma, infection, nerve damage, bone pain and fractures. All this may adversely affect the treatment and increase the length of hospital stay (Seiler et al., 2000; Smith et al., 2008). Moreover, the cells obtained by biopsy may not be sufficient for the graft, this involves an in vitro expansion, time consuming and often not successful. For all these reasons, this approach is not be used in emergency situations. The material used must be processed separately for each patient with customized protocols using the patient's own serum. For this reasons the use on a large scale is hardly feasible for the costs and the time required by each therapy.
The ultimate goal in the stem cell field is to find a way to translate our growing body of knowledge on stem cell biology into therapeutic applications for regenerative medicine. In that regard, most of our attention has focused upon stem-cell transplantation approaches, which have been considered above. On the other hand, the need to overcome the drawbacks associated with these approaches (mainly, the necessity of manipulating the cells before the graft takes place) has led to the development of new strategies to achieve tissue repair. The finding that many adult tissues contain stem cells that function to maintain and repair tissue damage (Su et al., 2009) paved the way to the idea that we could somehow recruit these endogenous stem cells in order to enhance tissue regeneration. Moreover, whereas the grafting strategies require the exogenous activation of stem cells, an endogenous re-activation can be speculated without the need of isolation, manipulation and grafting (Miller and Kaplan, 2012). However, though the concept of adult stem cells is an old one, the idea of their exploitation for regenerative purposes has gained attention only in the last few years. This is mainly due to the experimental evidence coming from studies showing that tissue stem cell behavior can be modulated differentially in physiologic and pathologic settings both in the animal model and in the human. For example, it is now well-established that regular exercise and brain injury can enhance neurogenesis, precursor proliferation and oligodendrogenesis in rodents (Ming and Song, 2011) and these results are comparable to those from recent imaging studies that show how aerobic exercise training enhances hippocampal volume in elderly humans (Erickson et al., 2011, 2012). Another reason we are currently approaching the idea of manipulating resident stem cells is that we now know that in some cases the factors that induce the stem cell response are the same that we have studied in other contexts, e.g., the growth factor BDNF is required for exercise-induced neurogenesis (Ming and Song, 2011). Moreover, a number of growth factors that were originally identified in stem cell culture studies have been shown to activate stem cells in vivo (Mitchell et al., 2004). We will now focus on the different approaches that have stemmed in regenerative medicine from the above considerations, with a special focus on craniofacial regeneration.
Understanding that the three-dimensional (3D) structure of the extracellular matrix (ECM) is integral for tissue formation and regeneration has led researchers to make an effort to recreate this environment when attempting to repair tissue defects, creating biocompatible matrices known as scaffolds. A scaffold is a three-dimension biomaterial designed to allow cell-biomaterial interactions, cell survival, proliferation and differentiation. That scaffold can be designed to biodegrade at a controllable rate and is characterized by a low degree of toxicity in vivo. Whether produced using synthetic or biologic materials, scaffold matrices enhance tissue growth and repair by facilitating delivery and localization of progenitor cells and growth factors to a desired location (Dhandayuthapani et al., 2011). Historically, scaffolds have been used as delivery systems in cell-based applications, whereas their use in cell-free strategies for tissue regeneration is relatively new (Bueno and Glowacki, 2009).
The use of scaffolds that accurately reproduce the structure of the native ECM (the so-called biomimetic scaffolds) is particularly useful in cranio-facial bone defects, as they play the role of template analogous for complex anatomical form while minimizing supply constraints. These types of scaffold vary in pore size, mechanical properties and degree of biodegrability, according to their chemical structure (Teven et al., 2015; see Table 1).
Table 1. Most used biomimetic scaffolds for cranio-facial bone regeneration in cell-based and cell-free applications.
Though a large amount of evidence correlates bioscaffold to increased tissue regeneration, it is still not clear whether this effect can be explained by the activation of endogenous stem cells (Zaky and Cancedda, 2009). For this reason, in the practice, scaffold-based approaches are generally combined with cell- and/or growth factor-based approaches. The former have already been discussed above (see section above), while the latter will be now presented.
Since several studies demonstrated the role of growth factors in stem cell biology (reviewed in Brizzi et al., 2012), it is almost impossible to ignore their potential provision to the therapeutic goal of tissue regeneration. Wagers previously reviewed how the use of growth factors in the clinic has already led to successful therapies in hematology and orthopedics (respectively, G-CSF analogs for chemotherapy-related neutropenia and parathyroid hormone analogs for osteoporosis; Wagers, 2012). In both these conditions, the support given to the stem cells is systemic, as it works in a tissue-specific fashion, with no privileged site of action. This is not the case in regenerative medicine, where the regeneration is usually desired only in a limited subset of body locations. For this reason, local delivery of growth factors to the site of lesion is a more suitable approach in regenerative medicine, and is usually pursued by binding growth factors to scaffolds (Figure 1). Depending on the desired release kinetic, growth factors can be either directly adsorbed to scaffolds or encapsulated in microspheres, respectively for a burst release or a sustained and delayed release. As for bone regeneration, which is the most investigated field in regenerative medicine of the craniofacial district, several growth factors have been identified with a wide range of activities in vivo (see Table 2). Bone morphogenetic proteins (BMPs) are a family of growth factors involved in embryonic development and regeneration of mesenchymal tissues (Zhao, 2003), whose roles have been investigated thoroughly in bone biology (reviewed in Luu et al., 2007). Although many isoforms of BMPs are known, only a small subset has been shown to be active in in vivo models of bone defects. BMP-2, among the others, is lethal in knockout mice model and was associated to orofacial clefting in case of haploinsufficiency in humans (Zhang and Bradley, 1996; Sahoo et al., 2011); for this reason, its use has been suggested for craniofacial bone reconstruction (Chenard et al., 2012). BMP-7 showed superior bone induction compared to autologous bone graft in an animal model of calvarial defect, when injected locally (Springer et al., 2005a,b). BMP-7 is also able to determine a widespread skeletal regeneration, and this led to its approval for use, along with BMP-2, in case of non-unions and open fractures of long bones (Gautschi et al., 2007). Other BMPs lack evidence of clinical relevance and their possible roles in the practice are reviewed by Bessa et al. (2008a,b). If, on one hand, BMPs support bone regeneration by activation of perilesional osteoprogenitor cells, several different growth factors can be used to stimulate mobilization of nearby or distant stem cells and their homing to the defect site (Herrmann et al., 2015).
Figure 1. Example strategies for bone regeneration trough Stem Cells recruitment and activation. A scaffold with control release of chemo-attractants and/or growth factors is grafted into the lesion, endogenous stem cells are attracted and home into the scaffold where they proliferate and differentiate repairing the tissue.
Table 2. Growth factors and other agents used for local activation and/or mobilization of stem cells in craniofacial and bone regeneration.
Vascular Endothelial Growth Factor (VEGF) is most known for its role in angiogenesis, but has also revealed a direct involvement in supporting bone formation (Schipani et al., 2009): for this reason, numerous preclinical bone healing models involving recombinant human VEGF delivered with various biomaterials have been tested. PLGA scaffolds delivery systems showed an increase in vascular density and bone formation in cranium and calvarial defects (Murphy et al., 2004; Kaigler et al., 2006), while collagen sponge-delivered VEGF reported similar results both in calvarial and in mandibular defects (Kleinheinz et al., 2005; Behr et al., 2012; Jin and Giannobile, 2014). Sustained release of VEGF has been achieved in different ways, such as pre-encapsulation in PLGA or gelatin microspheres or co-precipitation onto BCP, in order to support angiogenesis and bone formation for a longer period of time (Patel et al., 2008; De la Riva et al., 2009, 2010; Wernike et al., 2010; Farokhi et al., 2013, 2014). Moreover, combination of VEGF and BMP-2 showed an increased vascular density during bone regeneration but no detectable enhancement in bone formation, compared to BMP-2 alone (Young et al., 2009; Zhang et al., 2011; Ramazanoglu et al., 2013). Plateled-Derived Growth Factor (PDGF) is another angiogenetic molecule, involved in maturation of newly-formed blood vessels, but after the report of its chemoattractive action on Mesenchymal Stem Cells (MSCs) it has been studied as a homing factor in bone repair (Fiedler et al., 2004; Phipps et al., 2012). Local administration of PDGF in a critical-size calvarial defect has been shown to increase bone mineralization similarly to VEGF, but less than BMP-2 (Jin and Giannobile, 2014). Notably, Chang et al. demonstrated that gene delivery of PDGF stimulates repair of oral implant extraction socket defects in a rat model (Chang et al., 2010) moreover, PDGF has been approved for periodontal repair by FDA (Pellegrini et al., 2009). One of the most investigated signaling systems for extravasation and homing of mesenchymal cells is the SDF1-CXCR4 axis (Kitaori et al., 2009). It represents the perfect target for enhancing bone regeneration, as it is involved both in MSCs and endothelial progenitor cells (EPCs) homing dynamics, thus working both as osteoinductive and pro-angiogenetic. SDF-1-loaded scaffolds have been studied in fracture healing models (Fujio et al., 2011; Li X. et al., 2011) and in cranial defects (Ji et al., 2013; Liu et al., 2014), with excellent results (Figure 1). There is also evidence that SDF-1 may induce migration, proliferation and activation of human periodontal ligament stem cells (PLSCs; Du et al., 2012). The association of SDF-1 with BMP-2 increases bone volume in a calvarial defect model compared to SDF-1 alone (Jin and Giannobile, 2014). Another possible approach is the use of Platelet-Rich Plasma (PRP), a concentrate of blood platelets that upon activation releases various growth factors, including PDGF and VEGF (Fekete et al., 2012), and neurotransmitters that have been shown to be fundamental for tooth repair in animal model (Baudry et al., 2015). Nevertheless, its role as activator of endogenous stem cells is still controversial, this mainly due to problems in standardize the PRP production protocol (Malhotra et al., 2013); its use seems, however, more efficient in case of soft tissue healing or in case of concomitant cell transplantation (Anitua et al., 2008). In the case of periodontal regeneration, the potential use of PRP as a stem cell activator has been suggested, but not yet demonstrated (Chen et al., 2010; Del Corso et al., 2012). On the other hand, Platelet-Rich Fibrin (PRF), a polymeric (fibrin based) scaffold loaded with PRP, has shown some very interesting results in promoting craniofacial bone regeneration both in preclinical and in clinical setting (Simonpieri et al., 2012; Li et al., 2014). The use of autologous growth factors released by platelets could overcome one of the major problems associated with growth factor-mediated recruitment/activation of stem cells: the high production costs for recombinant human growth factors-loaded scaffolds. Other strategies proposed in order to stimulate the synthesis of growth factors directly at the site of the defect, such as gene transfer by plasmids, viral vectors or by genetically modified allogeneic or autologous cells, have been reviewed by Evans CH and will not be further discussed (Evans, 2014).
As considered in the previous section, the use of growth factors released at the site of defects to activate endogenous stem cells constitutes a valid and efficient approach for tissue regeneration, though it is encumbered by high production costs and short duration in time. If, on one hand, new preparation methods promise to decrease the costs of production, on the other hand new approaches for stem cells recruitment and activation are under investigation (Mallick and Cox, 2013). Old and new drugs are now being studied for their effects on bone and dental healing (Laurencin et al., 2014). Statins are the perfect example of drugs commonly used for hypercholesterolemia that have now shown a potential to induce bone formation (Mundy et al., 1999). The use of simvastatin-loaded scaffolds has been studied in calvarial defects in a rat model, proving an increase in bone healing; the mechanism of action seems to involve the local expression of BMP-2 and HIF-1α recruiting autogenous osteogenic and angiogenetic stem cells (Yueyi et al., 2013). AMD-3100 (octahydrochloride hydrate) is a new drug currently approved in USA (trade name: PlerixaforTM) for hematopoietic stem cells mobilization in patients that do not respond well to G-CSF alone. It works as a CXCR4 antagonist (or, more accurately, as a partial agonist), interfering with the SDF-1-CXCR4 axis and disrupting stem cell homing, with a primary effect on HSCs and EPCs (Pitchford et al., 2009). Several works outlined the positive effect of AMD-3100 administration after a bone lesion in animal models (Toupadakis et al., 2013) while Kumar and Ponnazhagan evidenced MSCs mobilization from bone marrow after AMD-3100 administration, and reported a significant augmentation of bone growth when it was co-administrated with IGF-1 (Kumar and Ponnazhagan, 2012). HSCs mobilization operated by G-CSF has been investigated in a phase II clinical trial in case of tibial osteotomy: in this study, the patients who received G-CSF infusion for 3 consecutive days and 4 h before surgery manifested an improved osseointegration of the bone graft compared to controls, though no difference in the quality of the newly formed bone was seen between the two groups (Marmotti et al., 2013). Local application of G-CSF with gelatin hydrogel has shown a potential use for bone regeneration as it contributes to an ideal local environment for fracture healing (Ishida et al., 2010). FTY720 is a small-molecule analog of sphingosine-1-phosphate (S1P), that works as a selective agonist on S1P1 and S1P3-5 receptors; it has proved an angiogenetic effect of microvasculature and an increase in graft osseointegration (Petrie Aronin et al., 2010; Sefcik et al., 2011). Its local delivery with PLGA-scaffolds led to statistically significant increases in bone volumes in a rat model of critical-size calvarial defects (Huang et al., 2012). Another small compound, SVAK-12, which acts as a disruptor of the binding of the E3-ligase Smurf1 with BMP-2 pathway transducers (namely, Smads -1, -5, and -8), thus increasing downstream signaling, has been found to potentiate the transdifferentiation of myoblasts toward an osteoblastic phenotype in vitro (Kato et al., 2011). In another study, a single local dose of SVAK-12 enhanced bone-healing in the presence of endogenous BMPs in an animal model of femoral fracture (Wong et al., 2013).
Pharmacological means are surely a powerful way to activate and/or mobilize stem cells, but other strategies are being developed that do not need the exogenous administration of substances. Though we are still at a seminal point in this direction, the idea of pursuing stem cells activation through physical means is revolutionary. Ultrasounds are mechanical waves (sound waves) characterized by frequencies higher than the upper audible limit of the human hearing. The use of ultrasounds skyrocketed in the last decades both in diagnostics and in therapy; as for bone healing, the most used ultrasound-based technique is LIPUS (Low-Intensity Pulsed Ultrasound Stimulation), which has been tested with various successes in case of bone fractures and non-unions (Watanabe et al., 2010; Yang et al., 2010). The biology of ultrasound effects on bone tissue has been a mystery until very recently, although the role of mechanical stresses is well-known in the scientific community since a long time (Carter, 1987); therefore, today is universally accepted that mechanical forces are a major determinant of stem cells self-renewal and fate decision, as their roles have been assessed in embryonic and mesenchymal stem cells (Li D. et al., 2011). In 2013, Kusuyama et al. demonstrated how LIPUS influences the multilineage differentiation of mesenchymal stem and progenitor cells, thus finding a proof of principle for ultrasound use in bone healing (Kusuyama et al., 2014). The effects of LIPUS on mesenchymal stem cells have been also studied with regards to chondrogenic differentiation (Cui et al., 2006) and osteogenic differentiation for tooth tissue engineering (Lim et al., 2013) in two separate in vitro studies. Light, beside the ultrasounds, is another notable mean for endogenous stem cells recruitment. In particular, in the study by Arany et al. the use of low-power lasers (LPL) induced reactive oxygen species (ROS) in a dose-dependent manner, which in turn activated latent TGF-beta via a specific methionine residue; light-activated TGF-beta was capable of differentiating human dental stem cells in vitro, and the results were confirmed in an in vivo pulp cap model in rat teeth. Moreover, in TGF-beta-RII-null animals this response to laser was abolished, confirming a central role for TGF-beta in dental regeneration (Arany et al., 2014). The last example of physical stimulus known for its effect on gene expression and stem cell fate is heat (Fan, 2012). Heat shocks constitute natural occurring events, which can be easily reproduced under specific conditions. In a series of experiments, DPSCs have been shown to be more resistant to heat stress compared to non-stem cells (Yao et al., 2011), and this paved the way for a heat-induced activation of DPSCs (Rezai Rad et al., 2013). However, these experiments were conducted in vitro, and their relative importance to endogenous stem cells activation has yet to be evidenced, and thus must be considered only speculative.
Regenerative medicine is today at a crucial point: we have already developed effective methods to improve tissue regeneration for some critical districts of the human body, such as the bones of the craniofacial complex, but these methods usually require the use of cells obtained from the same individual or from another individual (respectively, autologous or allogeneic graft), that need to be processed ex vivo before the transplant takes place. These procedures, though effective and increasingly standardized, expose the graft recipient to the risks already discussed in the previous sections. The increasing understanding of the mechanisms that regulate stem cell biology in adult tissues and their role in tissue healing in physiological and pathological conditions, along with the better comprehension of the role of the extracellular matrix in tissue dynamics, laid the foundations to newer strategies in regenerative medicine that aim to exploit the hidden potential of endogenous stem cells, such as growth factor-loaded bioscaffolds. These new approaches have been developed in order to avoid cell processing and decrease manufacturing burden, which constitute the main limitations to the spreading of the cell-based approaches. One way to reduce consistently the production costs would be the use of gene delivery strategies, in order to induce the synthesis of the growth factors directly in the host cells. However, this approach could become detrimental, as it would suffer from the same legislative and safety concerns of gene therapy (O'Reilly et al., 2015). Another critical point is that of the almost complete lack of any kind of data on human patients for growth factors-based approaches. More clinical trials are mandatory to assess the real impact of these techniques on human health, including safety assessments for the use of known mitogens. As for drug-induced stem cell activation, on the other hand, though even more molecules are under pre-clinical investigation (Kochegarov, 2009), the ones that have already proved effective in animal models should be validated for their safety and efficacy on humans. In this context, AMD-3100 could be regarded as the ideal drug to begin the clinical investigation, as it is already approved for human use, though with a different indication. Finally, concerning the physical approaches, new rigorous studies are important to understand whether these techniques can be really considered for a clinical application, as they would constitute the easiest and the less invasive way to promote tissue regeneration.
ML and VP performed the literature research and wrote the manuscript. TV and PF revised the manuscript. DA proofreading. DV and PG coordinated the work, perform the proofreading, and approve the final manuscript.
The authors declare that the research was conducted in the absence of any commercial or financial relationships that could be construed as a potential conflict of interest.
Alvarez, P., Hee, C. K., Solchaga, L., Snel, L., Kestler, H. K., Lynch, S. E., et al. (2012). Growth factors and craniofacial surgery. J. Craniofac. Surg. 23, 20–29. doi: 10.1097/SCS.0b013e318240c6a8
Amariglio, N., Hirshberg, A., Scheithauer, B. W., Cohen, Y., Loewenthal, R., Trakhtenbrot, L., et al. (2009). Donor-derived brain tumor following neural stem cell transplantation in an ataxia telangiectasia patient. PLoS Med. 17:e1000029. doi: 10.1371/journal.pmed.1000029
Anitua, E., Aguirre, J. J., Algorta, J., Ayerdi, E., Cabezas, A. I., Orive, G., et al. (2008). Effectiveness of autologous preparation rich in growth factors for the treatment of chronic cutaneous ulcers. J. Biomed. Mater. Res. B Appl. Biomater. 84, 415–421. doi: 10.1002/jbm.b.30886
Arany, P. R., Cho, A., Hunt, T. D., Sidhu, G., Shin, K., Hahm, E., et al. (2014). Photoactivation of endogenous latent transforming growth factor-β1 directs dental stem cell differentiation for regeneration. Sci. Trans. Med. 6:238ra269. doi: 10.1126/scitranslmed.3008234
Bae, S. C., Lee, K. S., Zhang, Y. W., and Ito, Y. (2001). Intimate relationship between TGF-β/BMP signaling and runt domain transcription factor, PEBP2/CBF. J. Bone Joint Surg. Am. 83-A(Suppl. 1), S48–S55.
Baker, D. E., Harrison, N. J., Maltby, E., Smith, K., Moore, H. D., Shaw, P. J., et al. (2007). Adaptation to culture of human embryonic stem cells and oncogenesis in vivo. Nat. Biotechnol. 25, 207–215. doi: 10.1038/nbt1285
Barker, R. A., and Widner, H. (2004). Immune problems in central nervous system cell therapy. NeuroRx 1, 472–481. doi: 10.1602/neurorx.1.4.472
Baudry, A., Alleaume-Butaux, A., Dimitrova-Nakov, S., Goldberg, M., Schneider, B., Launay, J. M., et al. (2015). Essential roles of dopamine and serotonin in tooth repair: functional interplay between odontogenic stem cells and platelets. Stem Cells 33, 2586–2595. doi: 10.1002/stem.2037
Baykan, E., Koc, A., Eser Elcin, A., and Murat Elcin, Y. (2014). Evaluation of a biomimetic poly(epsilon-caprolactone)/β-tricalcium phosphate multispiral scaffold for bone tissue engineering: in vitro and in vivo studies. Biointerphases 9, 029011. doi: 10.1116/1.4870781
Behr, B., Sorkin, M., Lehnhardt, M., Renda, A., Longaker, M. T., and Quarto, N. (2012). A comparative analysis of the osteogenic effects of BMP-2, FGF-2, and VEGFA in a calvarial defect model. Tissue Eng. Part A 18, 1079–1086. doi: 10.1089/ten.TEA.2011.0537
Bessa, P. C., Casal, M., and Reis, R. L. (2008a). Bone morphogenetic proteins in tissue engineering: the road from laboratory to clinic, part II (BMP delivery). J. Tissue Eng. Regen. Med. 2, 81–96. doi: 10.1002/term.74
Bessa, P. C., Casal, M., and Reis, R. L. (2008b). Bone morphogenetic proteins in tissue engineering: the road from the laboratory to the clinic, part I (basic concepts). J. Tissue Eng. Regen. Med. 2, 1–13. doi: 10.1002/term.63
Bose, S., Roy, M., and Bandyopadhyay, A. (2012). Recent advances in bone tissue engineering scaffolds. Trends Biotechnol. 30, 546–554. doi: 10.1016/j.tibtech.2012.07.005
Brizzi, M. F., Tarone, G., and Defilippi, P. (2012). Extracellular matrix, integrins, and growth factors as tailors of the stem cell niche. Curr. Opin. Cell Biol. 24, 645–651. doi: 10.1016/j.ceb.2012.07.001
Bueno, E. M., and Glowacki, J. (2009). Cell-free and cell-based approaches for bone regeneration. Nat. Rev. Rheumatol. 5, 685–697. doi: 10.1038/nrrheum.2009.228
Campagnoli, C., Roberts, I. A., Kumar, S., Bennett, P. R., Bellantuono, I., and Fisk, N. M. (2001). Identification of mesenchymal stem/progenitor cells in human first-trimester fetal blood, liver, and bone marrow. Blood 98, 2396–2402. doi: 10.1182/blood.V98.8.2396
Carpenter, M. K., Frey-Vasconcells, J., and Rao, M. S. (2009). Developing safe therapies from human pluripotent stem cells. Nat. Biotechnol. 27, 606–613. doi: 10.1038/nbt0709-606
Carter, D. R. (1987). Mechanical loading history and skeletal biology. J. Biomech. 20, 1095–1109. doi: 10.1016/0021-9290(87)90027-3
Chang, P. C., Seol, Y. J., Cirelli, J. A., Pellegrini, G., Jin, Q., Franco, L. M., et al. (2010). PDGF-B gene therapy accelerates bone engineering and oral implant osseointegration. Gene Ther. 17, 95–104. doi: 10.1038/gt.2009.117
Chavez, S. L., Meneses, J. J., Nguyen, H. N., Kim, S. K., and Pera, R. A. (2008). Characterization of six new human embryonic stem cell lines (HSF7, -8, -9, -10, -12, and -13) derived under minimal-animal component conditions. Stem Cells Dev. 17, 535–546. doi: 10.1089/scd.2007.0216
Chen, F. M., Zhang, M., and Wu, Z. F. (2010). Toward delivery of multiple growth factors in tissue engineering. Biomaterials 31, 6279–6308. doi: 10.1016/j.biomaterials.2010.04.053
Chen, Z., and Palmer, T. D. (2008). Cellular repair of CNS disorders: an immunological perspective. Hum. Mol. Genet. 17, R84–R92. doi: 10.1093/hmg/ddn104
Chenard, K. E., Teven, C. M., He, T. C., and Reid, R. R. (2012). Bone morphogenetic proteins in craniofacial surgery: current techniques, clinical experiences, and the future of personalized stem cell therapy. J. Biomed. Biotechnol. 2012:601549. doi: 10.1155/2012/601549
Chesnutt, B. M., Yuan, Y., Buddington, K., Haggard, W. O., and Bumgardner, J. D. (2009). Composite chitosan/nano-hydroxyapatite scaffolds induce osteocalcin production by osteoblasts in vitro and support bone formation in vivo. Tissue Eng. Part A 15, 2571–2579. doi: 10.1089/ten.tea.2008.0054
Choi, J. W., and Kim, N. (2015). Clinical application of three-dimensional printing technology in craniofacial plastic surgery. Arch. Plast. Surg. 42, 267–277. doi: 10.5999/aps.2015.42.3.267
Chung, E. J., Sugimoto, M. J., and Ameer, G. A. (2011). The role of hydroxyapatite in citric acid-based nanocomposites: surface characteristics, degradation, and osteogenicity in vitro. Acta Biomater. 7, 4057–4063. doi: 10.1016/j.actbio.2011.07.001
Cordeiro, M. M., Dong, Z., Kaneko, T., Zhang, Z., Miyazawa, M., Shi, S., et al. (2008). Dental pulp tissue engineering with stem cells from exfoliated deciduous teeth. J. Endod. 34, 962–969. doi: 10.1016/j.joen.2008.04.009
Costello, L. C., Franklin, R. B., Reynolds, M. A., and Chellaiah, M. (2012). The important role of osteoblasts and citrate production in bone formation: “osteoblast citration” as a new concept for an old relationship. Open Bone J. 4, 27–34. doi: 10.2174/1876525401204010027
Cui, J. H., Park, K., Park, S. R., and Min, B. H. (2006). Effects of low-intensity ultrasound on chondrogenic differentiation of mesenchymal stem cells embedded in polyglycolic acid: an in vivo study. Tissue Eng. 12, 75–82. doi: 10.1089/ten.2006.12.75
d'Aquino, R., Graziano, A., Sampaolesi, M., Laino, G., Pirozzi, G., De Rosa, A., et al. (2007). Human postnatal dental pulp cells co-differentiate into osteoblasts and endotheliocytes: a pivotal synergy leading to adult bone tissue formation. Cell Death Diff. 14, 1162–1171. doi: 10.1038/sj.cdd.4402121
d'Aquino, R., Tirino, V., Desiderio, V., Studer, M., De Angelis, G. C., Laino, L., et al. (2011). Human neural crest-derived postnatal cells exhibit remarkable embryonic attributes either in vitro or in vivo. Eur. Cell. Mater. 21, 304–316.
Davies, E., Muller, K. H., Wong, W. C., Pickard, C. J., Reid, D. G., Skepper, J. N., et al. (2014). Citrate bridges between mineral platelets in bone. Proc. Natl. Acad. Sci. U.S.A. 111, E1354–E1363. doi: 10.1073/pnas.1315080111
De la Riva, B., Nowak, C., Sanchez, E., Hernandez, A., Schulz-Siegmund, M., Pec, M. K., et al. (2009). VEGF-controlled release within a bone defect from alginate/chitosan/PLA-H scaffolds. Eur. J. Pharm. Biopharm. 73, 50–58. doi: 10.1016/j.ejpb.2009.04.014
De la Riva, B., Sanchez, E., Hernandez, A., Reyes, R., Tamimi, F., Lopez-Cabarcos, E., et al. (2010). Local controlled release of VEGF and PDGF from a combined brushite-chitosan system enhances bone regeneration. J. Control. Release 143, 45–52. doi: 10.1016/j.jconrel.2009.11.026
Del Corso, M., Vervelle, A., Simonpieri, A., Jimbo, R., Inchingolo, F., Sammartino, G., et al. (2012). Current knowledge and perspectives for the use of platelet-rich plasma (PRP) and platelet-rich fibrin (PRF) in oral and maxillofacial surgery part 1: Periodontal and dentoalveolar surgery. Curr. Pharm. Biotechnol. 13, 1207–1230. doi: 10.2174/138920112800624391
Dhandayuthapani, B., Yoshida, Y., Maekawa, T., and Kumar, D. S. (2011). Polymeric scaffolds in tissue engineering application: a review. Int. J. Polymer Sci. 2011, 19. doi: 10.1155/2011/290602
Du, L., Yang, P., and Ge, S. (2012). Stromal cell-derived factor-1 significantly induces proliferation, migration, and collagen type I expression in a human periodontal ligament stem cell subpopulation. J. Periodontol. 83, 379–388. doi: 10.1902/jop.2011.110201
Erickson, K. I., Miller, D. L., and Roecklein, K. A. (2012). The aging hippocampus: interactions between exercise, depression, and BDNF. Neuroscientist 18, 82–97. doi: 10.1177/1073858410397054
Erickson, K. I., Voss, M. W., Prakash, R. S., Basak, C., Szabo, A., Chaddock, L., et al. (2011). Exercise training increases size of hippocampus and improves memory. Proc. Natl. Acad. Sci. U.S.A. 108, 3017–3022. doi: 10.1073/pnas.1015950108
Evans, C. (2014). Using genes to facilitate the endogenous repair and regeneration of orthopaedic tissues. Int. Orthop. 38, 1761–1769. doi: 10.1007/s00264-014-2423-x
Fan, G. C. (2012). Role of heat shock proteins in stem cell behavior. Prog. Mol. Biol. Transl. Sci. 111, 305–322. doi: 10.1016/B978-0-12-398459-3.00014-9
Farokhi, M., Mottaghitalab, F., Ai, J., and Shokrgozar, M. A. (2013). Sustained release of platelet-derived growth factor and vascular endothelial growth factor from silk/calcium phosphate/PLGA based nanocomposite scaffold. Int. J. Pharm. 454, 216–225. doi: 10.1016/j.ijpharm.2013.06.080
Farokhi, M., Mottaghitalab, F., Shokrgozar, M. A., Ai, J., Hadjati, J., and Azami, M. (2014). Bio-hybrid silk fibroin/calcium phosphate/PLGA nanocomposite scaffold to control the delivery of vascular endothelial growth factor. Mater. Sci. Eng. C Mater. Biol. Appl. 35, 401–410. doi: 10.1016/j.msec.2013.11.023
Fekete, N., Gadelorge, M., Furst, D., Maurer, C., Dausend, J., Fleury-Cappellesso, S., et al. (2012). Platelet lysate from whole blood-derived pooled platelet concentrates and apheresis-derived platelet concentrates for the isolation and expansion of human bone marrow mesenchymal stromal cells: production process, content and identification of active components. Cytotherapy 14, 540–554. doi: 10.3109/14653249.2012.655420
Ferreira, A. M., Gentile, P., Chiono, V., and Ciardelli, G. (2012). Collagen for bone tissue regeneration. Acta Biomater. 8, 3191–3200. doi: 10.1016/j.actbio.2012.06.014
Fiedler, J., Etzel, N., and Brenner, R. E. (2004). To go or not to go: migration of human mesenchymal progenitor cells stimulated by isoforms of PDGF. J. Cell. Biochem. 93, 990–998. doi: 10.1002/jcb.20219
Fujio, M., Yamamoto, A., Ando, Y., Shohara, R., Kinoshita, K., Kaneko, T., et al. (2011). Stromal cell-derived factor-1 enhances distraction osteogenesis-mediated skeletal tissue regeneration through the recruitment of endothelial precursors. Bone 49, 693–700. doi: 10.1016/j.bone.2011.06.024
Gautschi, O. P., Frey, S. P., and Zellweger, R. (2007). Bone morphogenetic proteins in clinical applications. ANZ J. Surg. 77, 626–631. doi: 10.1111/j.1445-2197.2007.04175.x
Gentile, P., Chiono, V., Carmagnola, I., and Hatton, P. V. (2014). An overview of poly(lactic-co-glycolic) acid (PLGA)-based biomaterials for bone tissue engineering. Int. J. Mol. Sci. 15, 3640–3659. doi: 10.3390/ijms15033640
Giannobile, W. V., Ryan, S., Shih, M. S., Su, D. L., Kaplan, P. L., and Chan, T. C. (1998). Recombinant human osteogenic protein-1 (OP-1) stimulates periodontal wound healing in class III furcation defects. J. Periodontol. 69, 129–137. doi: 10.1902/jop.1998.69.2.129
Giuliani, A., Manescu, A., Langer, M., Rustichelli, F., Desiderio, V., Paino, F., et al. (2013). Three years after transplants in human mandibles, histological and in-line holotomography revealed that stem cells regenerated a compact rather than a spongy bone: biological and clinical implications. Stem Cells Trans. Med. 2, 316–324. doi: 10.5966/sctm.2012-0136
Griffin, M., Kalaskar, D. M., Butler, P. E., and Seifalian, A. M. (2014). The use of adipose stem cells in cranial facial surgery. Stem Cell Rev. 10, 671–685. doi: 10.1007/s12015-014-9522-3
Gronthos, S., Brahim, J., Li, W., Fisher, L. W., Cherman, N., Boyde, A., et al. (2002). Stem cell properties of human dental pulp stem cells. J. Dent. Res. 81, 531–535. doi: 10.1177/154405910208100806
Gronthos, S., Mankani, M., Brahim, J., Robey, P. G., and Shi, S. (2000). Postnatal human dental pulp stem cells (DPSCs) in vitro and in vivo. Proc. Natl. Acad. Sci. U.S.A. 97, 13625–13630. doi: 10.1073/pnas.240309797
Guha, P., Morgan, J. W., Mostoslavsky, G., Rodrigues, N. P., and Boyd, A. S. (2013). Lack of immune response to differentiated cells derived from syngeneic induced pluripotent stem cells. Cell Stem Cell 12, 407–412. doi: 10.1016/j.stem.2013.01.006
Hakkinen, L., Larjava, H., and Fournier, B. P. (2014). Distinct phenotype and therapeutic potential of gingival fibroblasts. Cytotherapy 16, 1171–1186. doi: 10.1016/j.jcyt.2014.04.004
Han, A., Zhao, H., Li, J., Pelikan, R., and Chai, Y. (2014). ALK5-mediated transforming growth factor β signaling in neural crest cells controls craniofacial muscle development via tissue-tissue interactions. Mol. Cell. Biol. 34, 3120–3131. doi: 10.1128/MCB.00623-14
Herrmann, M., Verrier, S., and Alini, M. (2015). Strategies to stimulate mobilization and homing of endogenous stem and progenitor cells for bone tissue repair. Front. Bioeng. Biotechnol. 3:79. doi: 10.3389/fbioe.2015.00079
Huang, C., Das, A., Barker, D., Tholpady, S., Wang, T., Cui, Q., et al. (2012). Local delivery of FTY720 accelerates cranial allograft incorporation and bone formation. Cell Tissue Res. 347, 553–566. doi: 10.1007/s00441-011-1217-3
Hung, C. N., Mar, K., Chang, H. C., Chiang, Y. L., Hu, H. Y., Lai, C. C., et al. (2011). A comparison between adipose tissue and dental pulp as sources of MSCs for tooth regeneration. Biomaterials 32, 6995–7005. doi: 10.1016/j.biomaterials.2011.05.086
Hynes, R. O. (2002a). Integrins: bidirectional, allosteric signaling machines. Cell 110, 673–687. doi: 10.1016/S0092-8674(02)00971-6
Hynes, R. O. (2002b). A reevaluation of integrins as regulators of angiogenesis. Nat. Med. 8, 918–921. doi: 10.1038/nm0902-918
Ishida, K., Matsumoto, T., Sasaki, K., Mifune, Y., Tei, K., Kubo, S., et al. (2010). Bone regeneration properties of granulocyte colony-stimulating factor via neovascularization and osteogenesis. Tissue Eng. Part A 16, 3271–3284. doi: 10.1089/ten.tea.2009.0268
Ji, W., Yang, F., Ma, J., Bouma, M. J., Boerman, O. C., Chen, Z., et al. (2013). Incorporation of stromal cell-derived factor-1alpha in PCL/gelatin electrospun membranes for guided bone regeneration. Biomaterials 34, 735–745. doi: 10.1016/j.biomaterials.2012.10.016
Jiang, H. B., Tian, W. D., Liu, L., Tang, W., Zheng, X. H., and Chen, X. Z. (2008). [Odontoblast-like cell phenotype differentiation of cranial neural crest stem cell in vivo]. Sichuan da Xue Xue Bao Yi Xue Ban 39, 276–278.
Jin, Q., and Giannobile, W. V. (2014). SDF-1 enhances wound healing of critical-sized calvarial defects beyond self-repair capacity. PLoS ONE 9:e97035. doi: 10.1371/journal.pone.0097035
Kaigler, D., Wang, Z., Horger, K., Mooney, D. J., and Krebsbach, P. H. (2006). VEGF scaffolds enhance angiogenesis and bone regeneration in irradiated osseous defects. J. Bone Miner. Res. 21, 735–744. doi: 10.1359/jbmr.060120
Kamitakahara, M., Ohtsuki, C., and Miyazaki, T. (2008). Review paper: behavior of ceramic biomaterials derived from tricalcium phosphate in physiological condition. J. Biomater. Appl. 23, 197–212. doi: 10.1177/0885328208096798
Kang, Q., Sun, M. H., Cheng, H., Peng, Y., Montag, A. G., Deyrup, A. T., et al. (2004). Characterization of the distinct orthotopic bone-forming activity of 14 BMPs using recombinant adenovirus-mediated gene delivery. Gene Ther. 11, 1312–1320. doi: 10.1038/sj.gt.3302298
Kato, S., Sangadala, S., Tomita, K., Titus, L., and Boden, S. D. (2011). A synthetic compound that potentiates bone morphogenetic protein-2-induced transdifferentiation of myoblasts into the osteoblastic phenotype. Molecular Cell. Biochem. 349, 97–106. doi: 10.1007/s11010-010-0664-6
Kim, S. S., Sun Park, M., Jeon, O., Yong Choi, C., and Kim, B. S. (2006). Poly(lactide-co-glycolide)/hydroxyapatite composite scaffolds for bone tissue engineering. Biomaterials 27, 1399–1409. doi: 10.1016/j.biomaterials.2005.08.016
Kitaori, T., Ito, H., Schwarz, E. M., Tsutsumi, R., Yoshitomi, H., Oishi, S., et al. (2009). Stromal cell-derived factor 1/CXCR4 signaling is critical for the recruitment of mesenchymal stem cells to the fracture site during skeletal repair in a mouse model. Arthritis Rheum. 60, 813–823. doi: 10.1002/art.24330
Kleinheinz, J., Stratmann, U., Joos, U., and Wiesmann, H. P. (2005). VEGF-activated angiogenesis during bone regeneration. J. Oral Maxillofac. Surg. 63, 1310–1316. doi: 10.1016/j.joms.2005.05.303
Kobayashi, K., Anada, T., Handa, T., Kanda, N., Yoshinari, M., Takahashi, T., et al. (2014). Osteoconductive property of a mechanical mixture of octacalcium phosphate and amorphous calcium phosphate. ACS Appl. Mater. Interfaces 6, 22602–22611. doi: 10.1021/am5067139
Kochegarov, A. (2009). Small molecules for stem cells. Expert Opinion Ther. Pat. 19, 275–281. doi: 10.1517/13543770802709010
Kumar, S., and Ponnazhagan, S. (2012). Mobilization of bone marrow mesenchymal stem cells in vivo augments bone healing in a mouse model of segmental bone defect. Bone 50, 1012–1018. doi: 10.1016/j.bone.2012.01.027
Kusuyama, J., Bandow, K., Shamoto, M., Kakimoto, K., Ohnishi, T., and Matsuguchi, T. (2014). Low intensity pulsed ultrasound (LIPUS) influences the multilineage differentiation of mesenchymal stem and progenitor cell lines through ROCK-Cot/Tpl2-MEK-ERK signaling pathway. J. Biol. Chem. 289, 10330–10344. doi: 10.1074/jbc.M113.546382
Laino, G., d'Aquino, R., Graziano, A., Lanza, V., Carinci, F., Naro, F., et al. (2005). A new population of human adult dental pulp stem cells: a useful source of living autologous fibrous bone tissue (LAB). J. Bone Miner. Res. 20, 1394–1402. doi: 10.1359/JBMR.050325
La Noce, M., Mele, L., Tirino, V., Paino, F., De Rosa, A., Naddeo, P., et al. (2014a). Neural crest stem cell population in craniomaxillofacial development and tissue repair. Eur. Cell. Mater. 28, 348–357.
La Noce, M., Paino, F., Spina, A., Naddeo, P., Montella, R., Desiderio, V., et al. (2014b). Dental pulp stem cells: state of the art and suggestions for a true translation of research into therapy. J. Dent. 42, 761–768. doi: 10.1016/j.jdent.2014.02.018
Laurencin, C. T., Ashe, K. M., Henry, N., Kan, H. M., and Lo, K. W. (2014). Delivery of small molecules for bone regenerative engineering: preclinical studies and potential clinical applications. Drug Discov. Today 19, 794–800. doi: 10.1016/j.drudis.2014.01.012
Ledford, H. (2015). The printed organs coming to a body near you. Nature 520:273. doi: 10.1038/520273a
Li, D., Zhou, J., Chowdhury, F., Cheng, J., Wang, N., and Wang, F. (2011). Role of mechanical factors in fate decisions of stem cells. Regen. Med. 6, 229–240. doi: 10.2217/rme.11.2
Li, Q., Reed, D. A., Min, L., Gopinathan, G., Li, S., Dangaria, S. J., et al. (2014). Lyophilized platelet-rich fibrin (PRF) promotes craniofacial bone regeneration through Runx2. Int. J. Mol. Sci. 15, 8509–8525. doi: 10.3390/ijms15058509
Li, X., Gao, Z., and Wang, J. (2011). Single percutaneous injection of stromal cell-derived factor-1 induces bone repair in mouse closed tibial fracture model. Orthopedics 34, 450. doi: 10.3928/01477447-20110427-19
Li, X., Yu, X., Lin, Q., Deng, C., Shan, Z., Yang, M., et al. (2007). Bone marrow mesenchymal stem cells differentiate into functional cardiac phenotypes by cardiac microenvironment. J. Mol. Cell. Cardiol. 42, 295–303. doi: 10.1016/j.yjmcc.2006.07.002
Liao, H. T., Lee, M. Y., Tsai, W. W., Wang, H. C., and Lu, W. C. (2013). Osteogenesis of adipose-derived stem cells on polycaprolactone-β-tricalcium phosphate scaffold fabricated via selective laser sintering and surface coating with collagen type I. J. Tissue Eng. Regen. Med. doi: 10.1002/term.1811. [Epub ahead of print].
Lim, K., Kim, J., Seonwoo, H., Park, S. H., Choung, P. H., and Chung, J. H. (2013). In vitro effects of low-intensity pulsed ultrasound stimulation on the osteogenic differentiation of human alveolar bone-derived mesenchymal stem cells for tooth tissue engineering. Biomed. Res. Int. 2013:269724. doi: 10.1155/2013/269724
Lin, H. R., Kuo, C. J., Yang, C. Y., Shaw, S. Y., and Wu, Y. J. (2002). Preparation of macroporous biodegradable PLGA scaffolds for cell attachment with the use of mixed salts as porogen additives. J. Biomed. Mater. Res. 63, 271–279. doi: 10.1002/jbm.10183
Liu, Y. S., Ou, M. E., Liu, H., Gu, M., Lv, L. W., Fan, C., et al. (2014). The effect of simvastatin on chemotactic capability of SDF-1alpha and the promotion of bone regeneration. Biomaterials 35, 4489–4498. doi: 10.1016/j.biomaterials.2014.02.025
Luo, G., Hofmann, C., Bronckers, A. L., Sohocki, M., Bradley, A., and Karsenty, G. (1995). BMP-7 is an inducer of nephrogenesis, and is also required for eye development and skeletal patterning. Genes Dev. 9, 2808–2820. doi: 10.1101/gad.9.22.2808
Luu, H. H., Song, W. X., Luo, X., Manning, D., Luo, J., Deng, Z. L., et al. (2007). Distinct roles of bone morphogenetic proteins in osteogenic differentiation of mesenchymal stem cells. J. Orthop. Res. 25, 665–677. doi: 10.1002/jor.20359
Malhotra, A., Pelletier, M. H., Yu, Y., and Walsh, W. R. (2013). Can platelet-rich plasma (PRP) improve bone healing? A comparison between the theory and experimental outcomes. Arch. Orthop. Trauma Surg. 133, 153–165. doi: 10.1007/s00402-012-1641-1
Mallick, K. K., and Cox, S. C. (2013). Biomaterial scaffolds for tissue engineering. Front. Biosci. 5:341–360. doi: 10.2741/E620
Mangano, C., Paino, F., d'Aquino, R., De Rosa, A., Iezzi, G., Piattelli, A., et al. (2011). Human dental pulp stem cells hook into biocoral scaffold forming an engineered biocomplex. PLoS ONE 6:e18721. doi: 10.1371/journal.pone.0018721
Marmotti, A., Castoldi, F., Rossi, R., Marenco, S., Risso, A., Ruella, M., et al. (2013). Bone marrow-derived cell mobilization by G-CSF to enhance osseointegration of bone substitute in high tibial osteotomy. Knee Surg. Sports Traumatol. Arthrosc 21, 237–248. doi: 10.1007/s00167-012-2150-z
Mason, C., and Dunnill, P. (2008). A brief definition of regenerative medicine. Regen. Med. 3, 1–5. doi: 10.2217/17460751.3.1.1
Miller, F. D., and Kaplan, D. R. (2012). Mobilizing endogenous stem cells for repair and regeneration: are we there yet? Cell Stem Cell 10, 650–652. doi: 10.1016/j.stem.2012.05.004
Ming, G. L., and Song, H. (2011). Adult neurogenesis in the mammalian brain: significant answers and significant questions. Neuron 70, 687–702. doi: 10.1016/j.neuron.2011.05.001
Mitchell, B. D., Emsley, J. G., Magavi, S. S., Arlotta, P., and Macklis, J. D. (2004). Constitutive and induced neurogenesis in the adult mammalian brain: manipulation of endogenous precursors toward CNS repair. Dev. Neurosci. 26, 101–117. doi: 10.1159/000082131
Mohanty, P., Prasad, N. K., Sahoo, N., Kumar, G., Mohanty, D., and Sah, S. (2015). Reforming craniofacial orthodontics via stem cells. J. Int. Soc. Prev. Community Dent. 5, 13–18. doi: 10.4103/2231-0762.151966
Mundy, G., Garrett, R., Harris, S., Chan, J., Chen, D., Rossini, G., et al. (1999). Stimulation of bone formation in vitro and in rodents by statins. Science 286, 1946–1949. doi: 10.1126/science.286.5446.1946
Murphy, W. L., Simmons, C. A., Kaigler, D., and Mooney, D. J. (2004). Bone regeneration via a mineral substrate and induced angiogenesis. J. Dent. Res. 83, 204–210. doi: 10.1177/154405910408300304
Murray, P. E., Garcia-Godoy, F., and Hargreaves, K. M. (2007). Regenerative endodontics: a review of current status and a call for action. J. Endodon. 33, 377–390. doi: 10.1016/j.joen.2006.09.013
Muzzarelli, R. A., Mattioli-Belmonte, M., Tietz, C., Biagini, R., Ferioli, G., Brunelli, M. A., et al. (1994). Stimulatory effect on bone formation exerted by a modified chitosan. Biomaterials 15, 1075–1081. doi: 10.1016/0142-9612(94)90093-0
Naddeo, P., Laino, L., La Noce, M., Piattelli, A., De Rosa, A., Iezzi, G., et al. (2015). Surface biocompatibility of differently textured titanium implants with mesenchymal stem cells. Dent. Mater. 31, 235–243. doi: 10.1016/j.dental.2014.12.015
Nakashima, M., Iohara, K., Ishikawa, M., Ito, M., Tomokiyo, A., Tanaka, T., et al. (2004). Stimulation of reparative dentin formation by ex vivo gene therapy using dental pulp stem cells electrotransfected with growth/differentiation factor 11 (Gdf11). Hum. Gene Ther. 15, 1045–1053. doi: 10.1089/hum.2004.15.1045
Nakashima, M., Iohara, K., and Sugiyama, M. (2009). Human dental pulp stem cells with highly angiogenic and neurogenic potential for possible use in pulp regeneration. Cytokine Growth Factor Rev. 20, 435–440. doi: 10.1016/j.cytogfr.2009.10.012
Obregon, F., Vaquette, C., Ivanovski, S., Hutmacher, D. W., and Bertassoni, L. E. (2015). Three-Dimensional Bioprinting for Regenerative Dentistry and Craniofacial Tissue Engineering. J. Dent. Res. 94, 143S–152S. doi: 10.1177/0022034515588885
Ohazama, A., Modino, S. A., Miletich, I., and Sharpe, P. T. (2004). Stem-cell-based tissue engineering of murine teeth. J. Dent. Res. 83, 518–522. doi: 10.1177/154405910408300702
O'Reilly, M., Jambou, R., Rosenthal, E., Montgomery, M., Hassani, M., Gargiulo, L., et al. (2015). The national institutes of health oversight of human gene transfer research: enhancing science and safety. Adv. Exp. Med. Biol. 871, 31–47. doi: 10.1007/978-3-319-18618-4_2
Paino, F., La Noce, M., Tirino, V., Naddeo, P., Desiderio, V., Pirozzi, G., et al. (2014). Histone deacetylase inhibition with valproic acid downregulates osteocalcin gene expression in human dental pulp stem cells and osteoblasts: evidence for HDAC2 involvement. Stem Cells 32, 279–289. doi: 10.1002/stem.1544
Panda, N. N., Biswas, A., Pramanik, K., and Jonnalagadda, S. (2015). Enhanced osteogenic potential of human mesenchymal stem cells on electrospun nanofibrous scaffolds prepared from eri-tasar silk fibroin. J. Biomed. Mater. Res. Part B Appl. Biomater. 103, 971–982. doi: 10.1002/jbm.b.33272
Patel, Z. S., Ueda, H., Yamamoto, M., Tabata, Y., and Mikos, A. G. (2008). In vitro and in vivo release of vascular endothelial growth factor from gelatin microparticles and biodegradable composite scaffolds. Pharm. Res. 25, 2370–2378. doi: 10.1007/s11095-008-9685-1
Pellegrini, G., Seol, Y. J., Gruber, R., and Giannobile, W. V. (2009). Pre-clinical models for oral and periodontal reconstructive therapies. J. Dent. Res. 88, 1065–1076. doi: 10.1177/0022034509349748
Peng, H., Wright, V., Usas, A., Gearhart, B., Shen, H. C., Cummins, J., et al. (2002). Synergistic enhancement of bone formation and healing by stem cell-expressed VEGF and bone morphogenetic protein-4. J. Clin. Invest. 110, 751–759. doi: 10.1172/JCI15153
Petrie Aronin, C. E., Shin, S. J., Naden, K. B., Rios, P. D. Jr., Sefcik, L. S., Zawodny, S. R., et al. (2010). The enhancement of bone allograft incorporation by the local delivery of the sphingosine 1-phosphate receptor targeted drug FTY720. Biomaterials 31, 6417–6424. doi: 10.1016/j.biomaterials.2010.04.061
Phipps, M. C., Xu, Y., and Bellis, S. L. (2012). Delivery of platelet-derived growth factor as a chemotactic factor for mesenchymal stem cells by bone-mimetic electrospun scaffolds. PLoS ONE 7:e40831. doi: 10.1371/journal.pone.0040831
Pitchford, S. C., Furze, R. C., Jones, C. P., Wengner, A. M., and Rankin, S. M. (2009). Differential mobilization of subsets of progenitor cells from the bone marrow. Cell Stem Cell 4, 62–72. doi: 10.1016/j.stem.2008.10.017
Pittenger, M. F., Mackay, A. M., Beck, S. C., Jaiswal, R. K., Douglas, R., Mosca, J. D., et al. (1999). Multilineage potential of adult human mesenchymal stem cells. Science 284, 143–147. doi: 10.1126/science.284.5411.143
Qi, X. N., Mou, Z. L., Zhang, J., and Zhang, Z. Q. (2014). Preparation of chitosan/silk fibroin/hydroxyapatite porous scaffold and its characteristics in comparison to bi-component scaffolds. J. Biomed. Mater. Res. Part A 102, 366–372. doi: 10.1002/jbm.a.34710
Rajan, A., Eubanks, E., Edwards, S., Aronovich, S., Travan, S., Rudek, I., et al. (2014). Optimized cell survival and seeding efficiency for craniofacial tissue engineering using clinical stem cell therapy. Stem Cells Trans. Med. 3, 1495–1503. doi: 10.5966/sctm.2014-0039
Ramazanoglu, M., Lutz, R., Rusche, P., Trabzon, L., Kose, G. T., Prechtl, C., et al. (2013). Bone response to biomimetic implants delivering BMP-2 and VEGF: an immunohistochemical study. J. Craniomaxillofac. Surg 41, 826–835. doi: 10.1016/j.jcms.2013.01.037
Rezai Rad, M., Wise, G. E., Brooks, H., Flanagan, M. B., and Yao, S. (2013). Activation of proliferation and differentiation of dental follicle stem cells (DFSCs) by heat stress. Cell Prolif. 46, 58–66. doi: 10.1111/cpr.12004
Rezwan, K., Chen, Q. Z., Blaker, J. J., and Boccaccini, A. R. (2006). Biodegradable and bioactive porous polymer/inorganic composite scaffolds for bone tissue engineering. Biomaterials 27, 3413–3431. doi: 10.1016/j.biomaterials.2006.01.039
Riccio, M., Maraldi, T., Pisciotta, A., La Sala, G. B., Ferrari, A., Bruzzesi, G., et al. (2012). Fibroin scaffold repairs critical-size bone defects in vivo supported by human amniotic fluid and dental pulp stem cells. Tissue Eng. Part A 18, 1006–1013. doi: 10.1089/ten.tea.2011.0542
Sadan, O., Shemesh, N., Barzilay, R., Bahat-Stromza, M., Melamed, E., Cohen, Y., et al. (2008). Migration of neurotrophic factors-secreting mesenchymal stem cells toward a quinolinic acid lesion as viewed by magnetic resonance imaging. Stem Cells 26, 2542–2551. doi: 10.1634/stemcells.2008-0240
Sahoo, T., Theisen, A., Sanchez-Lara, P. A., Marble, M., Schweitzer, D. N., Torchia, B. S., et al. (2011). Microdeletion 20p12.3 involving BMP2 contributes to syndromic forms of cleft palate. A. J. Med. Genet. Part A 155A, 1646–1653. doi: 10.1002/ajmg.a.34063
Schantz, J. T., Hutmacher, D. W., Lam, C. X., Brinkmann, M., Wong, K. M., Lim, T. C., et al. (2003a). Repair of calvarial defects with customised tissue-engineered bone grafts II. Evaluation of cellular efficiency and efficacy in vivo. Tissue Eng. 9(Suppl. 1), S127–S139. doi: 10.1089/10763270360697030
Schantz, J. T., Teoh, S. H., Lim, T. C., Endres, M., Lam, C. X., and Hutmacher, D. W. (2003b). Repair of calvarial defects with customized tissue-engineered bone grafts I. Evaluation of osteogenesis in a three-dimensional culture system. Tissue Eng. 9(Suppl. 1), S113–S126. doi: 10.1089/10763270360697021
Schipani, E., Maes, C., Carmeliet, G., and Semenza, G. L. (2009). Regulation of osteogenesis-angiogenesis coupling by HIFs and VEGF. J. Bone Miner. Res. 24, 1347–1353. doi: 10.1359/jbmr.090602
Schliephake, H., Weich, H. A., Dullin, C., Gruber, R., and Frahse, S. (2008). Mandibular bone repair by implantation of rhBMP-2 in a slow release carrier of polylactic acid–an experimental study in rats. Biomaterials 29, 103–110. doi: 10.1016/j.biomaterials.2007.09.019
Sefcik, L. S., Aronin, C. E., Awojoodu, A. O., Shin, S. J., Mac Gabhann, F., MacDonald, T. L., et al. (2011). Selective activation of sphingosine 1-phosphate receptors 1 and 3 promotes local microvascular network growth. Tissue Eng. Part A 17, 617–629. doi: 10.1089/ten.tea.2010.0404
Seiler, J. G. III, Casey, P. J., and Binford, S. H. (2000). Compartment syndromes of the upper extremity. J. South. Orthop. Assoc. 9, 233–247.
Seo, B. M., Miura, M., Gronthos, S., Bartold, P. M., Batouli, S., Brahim, J., et al. (2004). Investigation of multipotent postnatal stem cells from human periodontal ligament. Lancet 364, 149–155. doi: 10.1016/S0140-6736(04)16627-0
Seol, Y. J., Lee, J. Y., Park, Y. J., Lee, Y. M., Young, K., Rhyu, I. C., et al. (2004). Chitosan sponges as tissue engineering scaffolds for bone formation. Biotechnol. Lett. 26, 1037–1041. doi: 10.1023/B:BILE.0000032962.79531.fd
Simonpieri, A., Del Corso, M., Vervelle, A., Jimbo, R., Inchingolo, F., Sammartino, G., et al. (2012). Current knowledge and perspectives for the use of platelet-rich plasma (PRP) and platelet-rich fibrin (PRF) in oral and maxillofacial surgery part 2: Bone graft, implant and reconstructive surgery. Curr. Pharm. Biotechnol. 13, 1231–1256. doi: 10.2174/138920112800624472
Smith, D. M., Cooper, G. M., Afifi, A. M., Mooney, M. P., Cray, J., Rubin, J. P., et al. (2011). Regenerative surgery in cranioplasty revisited: the role of adipose-derived stem cells and BMP-2. Plast. Reconst. Surg. 128, 1053–1060. doi: 10.1097/PRS.0b013e31822b65e4
Smith, D. M., Cooper, G. M., Mooney, M. P., Marra, K. G., and Losee, J. E. (2008). Bone morphogenetic protein 2 therapy for craniofacial surgery. J. Craniofac. Surg. 19, 1244–1259. doi: 10.1097/SCS.0b013e3181843312
Solloway, M. J., Dudley, A. T., Bikoff, E. K., Lyons, K. M., Hogan, B. L., and Robertson, E. J. (1998). Mice lacking Bmp6 function. Dev. Genet. 22, 321–339. doi: 10.1002/(SICI)1520-6408(1998)22:4%3C321::AID-DVG3%3E3.0.CO;2-8
Sonoyama, W., Liu, Y., Yamaza, T., Tuan, R. S., Wang, S., Shi, S., et al. (2008). Characterization of the apical papilla and its residing stem cells from human immature permanent teeth: a pilot study. J. Endod. 34, 166–171. doi: 10.1016/j.joen.2007.11.021
Spits, C., Mateizel, I., Geens, M., Mertzanidou, A., Staessen, C., Vandeskelde, Y., et al. (2008). Recurrent chromosomal abnormalities in human embryonic stem cells. Nat. Biotechnol. 26, 1361–1363. doi: 10.1038/nbt.1510
Springer, I. N., Acil, Y., Kuchenbecker, S., Bolte, H., Warnke, P. H., Abboud, M., et al. (2005a). Bone graft versus BMP-7 in a critical size defect–cranioplasty in a growing infant model. Bone 37, 563–569. doi: 10.1016/j.bone.2005.05.010
Springer, I. N., Acil, Y., Spies, C., Jepsen, S., Warnke, P. H., Bolte, H., et al. (2005b). RhBMP-7 improves survival and eruption in a growing tooth avulsion trauma model. Bone 37, 570–577. doi: 10.1016/j.bone.2005.04.037
Stadlinger, B., Pilling, E., Huhle, M., Mai, R., Bierbaum, S., Scharnweber, D., et al. (2008). Evaluation of osseointegration of dental implants coated with collagen, chondroitin sulphate and BMP-4: an animal study. Int. J. Oral Maxillofac. Surg. 37, 54–59. doi: 10.1016/j.ijom.2007.05.024
Su, X., Paris, M., Gi, Y. J., Tsai, K. Y., Cho, M. S., Lin, Y. L., et al. (2009). TAp63 prevents premature aging by promoting adult stem cell maintenance. Cell Stem Cell 5, 64–75. doi: 10.1016/j.stem.2009.04.003
Sui, W., Hou, X., Che, W., Chen, J., Ou, M., Xue, W., et al. (2013). Hematopoietic and mesenchymal stem cell transplantation for severe and refractory systemic lupus erythematosus. Clin. Immunol. 148, 186–197. doi: 10.1016/j.clim.2013.05.014
Takahashi, K., Tanabe, K., Ohnuki, M., Narita, M., Ichisaka, T., Tomoda, K., et al. (2007). Induction of pluripotent stem cells from adult human fibroblasts by defined factors. Cell 131, 861–872. doi: 10.1016/j.cell.2007.11.019
Teo, A. K., and Vallier, L. (2010). Emerging use of stem cells in regenerative medicine. Biochem. J. 428, 11–23. doi: 10.1042/BJ20100102
Teven, C. M., Fisher, S., Ameer, G. A., He, T. C., and Reid, R. R. (2015). Biomimetic approaches to complex craniofacial defects. Ann. Maxillofac. Surg. 5, 4–13. doi: 10.4103/2231-0746.161044
Tevlin, R., McArdle, A., Atashroo, D., Walmsley, G. G., Senarath-Yapa, K., Zielins, E. R., et al. (2014). Biomaterials for craniofacial bone engineering. J. Dent. Res. 93, 1187–1195. doi: 10.1177/0022034514547271
Toma, J. G., Akhavan, M., Fernandes, K. J., Barnabe-Heider, F., Sadikot, A., Kaplan, D. R., et al. (2001). Isolation of multipotent adult stem cells from the dermis of mammalian skin. Nat. Cell Biol. 3, 778–784. doi: 10.1038/ncb0901-778
Toupadakis, C. A., Granick, J. L., Sagy, M., Wong, A., Ghassemi, E., Chung, D. J., et al. (2013). Mobilization of endogenous stem cell populations enhances fracture healing in a murine femoral fracture model. Cytotherapy 15, 1136–1147. doi: 10.1016/j.jcyt.2013.05.004
Tsai, R. J., Li, L. M., and Chen, J. K. (2000). Reconstruction of damaged corneas by transplantation of autologous limbal epithelial cells. N. Engl. J. Med. 13, 86–93. doi: 10.1056/NEJM200007133430202
Vesterbacka, M., Ringden, O., Remberger, M., Huggare, J., and Dahllof, G. (2012). Disturbances in dental development and craniofacial growth in children treated with hematopoietic stem cell transplantation. Orthod. Craniofac. Res. 15, 21–29. doi: 10.1111/j.1601-6343.2011.01533.x
Wada, M. R., Inagawa-Ogashiwa, M., Shimizu, S., Yasumoto, S., and Hashimoto, N. (2002). Generation of different fates from multipotent muscle stem cells. Development 129, 2987–2995.
Wagers, A. J. (2012). The stem cell niche in regenerative medicine. Cell Stem Cell 10, 362–369. doi: 10.1016/j.stem.2012.02.018
Warejcka, D. J., Harvey, R., Taylor, B. J., Young, H. E., and Lucas, P. A. (1996). A population of cells isolated from rat heart capable of differentiating into several mesodermal phenotypes. J. Surg. Res. 62, 233–242. doi: 10.1006/jsre.1996.0201
Watanabe, Y., Matsushita, T., Bhandari, M., Zdero, R., and Schemitsch, E. H. (2010). Ultrasound for fracture healing: current evidence. J. Orthop. Trauma 24(Suppl. 1), S56–S61. doi: 10.1097/BOT.0b013e3181d2efaf
Wernike, E., Montjovent, M. O., Liu, Y., Wismeijer, D., Hunziker, E. B., Siebenrock, K. A., et al. (2010). VEGF incorporated into calcium phosphate ceramics promotes vascularisation and bone formation in vivo. Eur. Cell. Mater.19, 30–40.
Wong, E., Sangadala, S., Boden, S. D., Yoshioka, K., Hutton, W. C., Oliver, C., et al. (2013). A novel low-molecular-weight compound enhances ectopic bone formation and fracture repair. J. Bone Joint Surg. Am. 6, 454–461. doi: 10.2106/JBJS.L.00275
Wu, G., Deng, Z. H., Fan, X. J., Ma, Z. F., Sun, Y. J., Ma, D. D., et al. (2009). Odontogenic potential of mesenchymal cells from hair follicle dermal papilla. Stem Cells Dev. 18, 583–589. doi: 10.1089/scd.2008.0066
Yang, M., Zhang, H., and Gangolli, R. (2014). Advances of mesenchymal stem cells derived from bone marrow and dental tissue in craniofacial tissue engineering. Curr. Stem Cell Res. Ther. 9, 150–161.
Yang, M. H., Lim, K. T., Choung, P. H., Cho, C. S., and Chung, J. H. (2010). Application of ultrasound stimulation in bone tissue engineering. Int. J. Stem Cells 3, 74–79.
Yao, S., Gutierrez, D. L., He, H., Dai, Y., Liu, D., and Wise, G. E. (2011). Proliferation of dental follicle-derived cell populations in heat-stress conditions. Cell Prolif. 44, 486–493. doi: 10.1111/j.1365-2184.2011.00778.x
Yoon, E., Dhar, S., Chun, D. E., Gharibjanian, N. A., and Evans, G. R. (2007). In vivo osteogenic potential of human adipose-derived stem cells/poly lactide-co-glycolic acid constructs for bone regeneration in a rat critical-sized calvarial defect model. Tissue Eng. 13, 619–627. doi: 10.1089/ten.2006.0102
Yoshikawa, H., and Myoui, A. (2005). Bone tissue engineering with porous hydroxyapatite ceramics. J. Artif. Organs 8, 131–136. doi: 10.1007/s10047-005-0292-1
Young, S., Patel, Z. S., Kretlow, J. D., Murphy, M. B., Mountziaris, P. M., Baggett, L. S., et al. (2009). Dose effect of dual delivery of vascular endothelial growth factor and bone morphogenetic protein-2 on bone regeneration in a rat critical-size defect model. Tissue Eng. Part A 15, 2347–2362. doi: 10.1089/ten.tea.2008.0510
Yueyi, C., Xiaoguang, H., Jingying, W., Quansheng, S., Jie, T., Xin, F., et al. (2013). Calvarial defect healing by recruitment of autogenous osteogenic stem cells using locally applied simvastatin. Biomaterials 34, 9373–9380. doi: 10.1016/j.biomaterials.2013.08.060
Zaky, S. H., and Cancedda, R. (2009). Engineering craniofacial structures: facing the challenge. J. Dent. Res. 88, 1077–1091. doi: 10.1177/0022034509349926
Zhang, H., and Bradley, A. (1996). Mice deficient for BMP2 are nonviable and have defects in amnion/chorion and cardiac development. Development 122, 2977–2986.
Zhang, W., Wang, X., Wang, S., Zhao, J., Xu, L., Zhu, C., et al. (2011). The use of injectable sonication-induced silk hydrogel for VEGF(165) and BMP-2 delivery for elevation of the maxillary sinus floor. Biomaterials 32, 9415–9424. doi: 10.1016/j.biomaterials.2011.08.047
Zhang, W., Zhu, C., Wu, Y., Ye, D., Wang, S., Zou, D., et al. (2014). VEGF and BMP-2 promote bone regeneration by facilitating bone marrow stem cell homing and differentiation. Eur. Cell. Mater. 27, 1–11; discussion 11–12. doi: 10.1016/j.eurpolymj.2014.08.007
Zhao, G. Q. (2003). Consequences of knocking out BMP signaling in the mouse. Genesis 35, 43–56. doi: 10.1002/gene.10167
Keywords: craniofacial abnormalities, regenerative medicine, stem cell transplantation, stem cells and regenerative medicine, stem cells recruitment, SDF1, bioscaffold, BMP signaling
Citation: Mele L, Vitiello PP, Tirino V, Paino F, De Rosa A, Liccardo D, Papaccio G and Desiderio V (2016) Changing Paradigms in Cranio-Facial Regeneration: Current and New Strategies for the Activation of Endogenous Stem Cells. Front. Physiol. 7:62. doi: 10.3389/fphys.2016.00062
Received: 04 January 2016; Accepted: 09 February 2016;
Published: 24 February 2016.
Edited by:
Thimios Mitsiadis, University of Zurich, SwitzerlandReviewed by:
Ariane Berdal, UMRS 1138 Institut National de la Santé et de la Recherche Médicale, Université Paris Diderot - Paris 7, FranceCopyright © 2016 Mele, Vitiello, Tirino, Paino, De Rosa, Liccardo, Papaccio and Desiderio. This is an open-access article distributed under the terms of the Creative Commons Attribution License (CC BY). The use, distribution or reproduction in other forums is permitted, provided the original author(s) or licensor are credited and that the original publication in this journal is cited, in accordance with accepted academic practice. No use, distribution or reproduction is permitted which does not comply with these terms.
*Correspondence: Papaccio Gianpaolo, Z2lhbnBhb2xvLnBhcGFjY2lvQHVuaW5hMi5pdA==
Disclaimer: All claims expressed in this article are solely those of the authors and do not necessarily represent those of their affiliated organizations, or those of the publisher, the editors and the reviewers. Any product that may be evaluated in this article or claim that may be made by its manufacturer is not guaranteed or endorsed by the publisher.
Research integrity at Frontiers
Learn more about the work of our research integrity team to safeguard the quality of each article we publish.