- Department of Physical Education and Sport Science, Academic Center of Vitoria, Federal University of Pernambuco, Vitória de Santo Antão, Brazil
Systemic arterial hypertension (SAH) is an important risk factor for cardiovascular disease and affects worldwide population. Current environment including life style coupled with genetic programming have been attributed to the rising incidence of hypertension. Besides, environmental conditions during perinatal development such as maternal malnutrition can program changes in the integration among renal, neural, and endocrine system leading to hypertension. This phenomenon is termed phenotypic plasticity and refers to the adjustment of a phenotype in response to environmental stimuli without genetic change, following a novel or unusual input during development. Human and animal studies indicate that fetal exposure to an adverse maternal environment may alter the renal morphology and physiology that contribute to the development of hypertension. Recently, it has been shown that the maternal protein restriction alter the central control of SAH by a mechanism that include respiratory dysfunction and enhanced sympathetic-respiratory coupling at early life, which may contribute to adult hypertension. This review will address the new insights on the maternal diet induced-hypertension that include the potential role of the phenotypic plasticity, specifically the perinatal protein malnutrition, and sympathetic-respiratory overactivity.
Introduction
Hypertension is a highly prevalent and significant risk factor for the development of metabolic disease, including coronary heart disease (CHD), stroke, heart failure, aortic, and peripheral arterial disease (Landsberg et al., 2013). The etiology of hypertension includes a complex phenotype that arises from numerous genetic, environmental, behavioral, ethnic, and even social origins (Landsberg et al., 2013). However, it has been observed that the perinatal nutritional milieu during “sensitive” periods of development or in infant affects the normal growth/developing and this may be associated with adult disease (Lucas, 1998; Victora et al., 2008; Wells, 2012). This phenomenon can be understood in the context of phenotypic plasticity. Phenotypic plasticity refers to the ability of an organism to react to an internal and external environmental input with a change in the form, state, movement, or rate of activity without genetic changes (West-Eberhard, 2005).
The association between Systemic arterial hypertension (SAH) and nutritional factors has been studied by a large number of epidemiological and clinical studies (Ashton, 2000; Hemachandra et al., 2006; Antony and Laxmaiah, 2008; Conde and Monteiro, 2014; Parra et al., 2015). Indeed, perinatal malnutrition is associated with the risk of developing cardiovascular disease and co-morbidities in later life including hypertension, metabolic syndrome and diabetes, (Nuyt, 2008; Nuyt and Alexander, 2009). In humans, studies have provided support for the positive association between low birth weight and increased incidence of hypertension (Ravelli et al., 1976; Hales et al., 1991; Sawaya and Roberts, 2003; Sawaya et al., 2004).
It is well established that perinatal malnutrition environmental stimuli can contribute to the programming of subsequent risks of hypertension by mechanisms that include reduced nephron morphology and function, reduced glomerular filtration rate, and dysfunction on the renin-angiotensin-aldosterone system (Nuyt and Alexander, 2009). Recently, studies have highlighted the contribution of the sympathetic-respiratory dysfunctions on the development of the maternal diet induced-hypertension (de Brito Alves et al., 2015). Protein-restricted rats during gestation and lactation showed respiratory dysfunction, which was associated with sympathetic overactivity and enhanced carotid bodies (CB) sensitivity to hypoxia (de Brito Alves et al., 2015; Nanduri and Prabhakar, 2015; Prabhakar et al., 2015). The underlying mechanism may be associated with high levels of hypoxic inducible factor (HIF-1α) in CB peripheral chemoreceptor (Ito et al., 2011, 2012; de Brito Alves et al., 2015). Thus, the aim of this review was to address the new insights about maternal diet induced-hypertension and the concept that perinatal malnutrition may affect the ventilatory and cardiovascular control.
New Insights on the Perinatal Origin of Hypertension: The Role of Phenotypic Plasticity
One of the best-known attempts to understand the association between early nutrition and SAH is the “thrifty phenotype hypothesis” proposed by Hales and Barker in 1992. This hypothesis is extensively used to consider cardiovascular disease as a “programmed” effect of nutritional restriction during early phases of growth and development, followed by a recovery of the diet during lifespan (Hales and Barker, 1992). This phenomenon can be understood in the context of the phenotypic plasticity (Barker et al., 2005; West-Eberhard, 2005; Labayen et al., 2006; Andersen et al., 2009; Biosca et al., 2011). Phenotypic plasticity is defined as the ability of an organism to react to an environmental stimuli with a adaptive mutual adjustment, without genetic change, among variable aspects of the phenotype, following a novel or unusual input during development (West-Eberhard, 2005). Epigenetic alterations as DNA methylation, histone acetylation and microRNA expression are the molecular basis of the phenotypic plasticity (Wells, 2011). Maternal low-protein diet model during gestation and/or lactation is one of the most extensively studied animal models of phenotypic plasticity (Ozanne and Hales, 2004; Costa-Silva et al., 2009; Falcão-Tebas et al., 2012; Fidalgo et al., 2013; de Brito Alves et al., 2014). An overview about the environmental influence during development on early appearance of SAH is shown in Figure 1. Feeding a low-protein diet (8% casein) during gestation followed by the consumption of a normocaloric diet throughout lactation is associated with growth restriction, asymmetric reduction in organ growth, elevated systolic blood pressure, and increased fasting plasma insulin concentrations (Ozanne and Hales, 2004; Fidalgo et al., 2013; de Brito Alves et al., 2014). Recently, it was demonstrated that adult animals subjected to maternal protein restriction presented mainly an increase in the cardiovascular sympathetic tone and increased low frequency (LF) bands of the SAH, suggesting autonomic misbalance, and sympathetic predominance on the cardiovascular system of these animals (Barros et al., 2015).
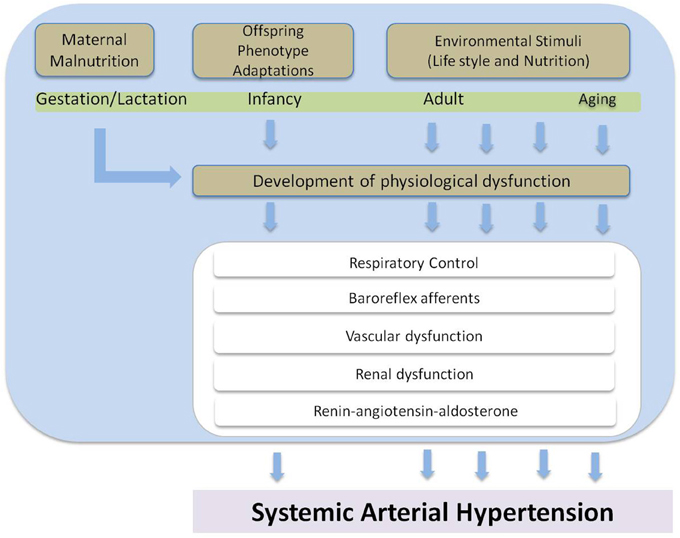
Figure 1. Overview about the environmental influence during development on early appearance of systemic arterial hypertension.
It is known that the rhythmicity of the sympathetic nervous system can modulate the arterial pressure (AP) and the heart rate at regular frequencies (Tseng et al., 2009). These rhythmic fluctuations in the cardiovascular variables suggest a measurement of cardiovascular autonomic balance (Japundzic-Zigon, 1998). Accordingly, the LF oscillations of the systolic arterial pressure (SAP) are typically enhanced during states of sympathetic activation (Julien, 2006) and are increased in the offspring from dams subjected to protein restriction during perinatal period and may contribute to the development of arterial hypertension in this experimental model (de Brito Alves et al., 2015).
Although the relationship between maternal protein restriction and sympathetic overactivity have been suggested (Johansson et al., 2007; Franco et al., 2008; Barros et al., 2015), less is known about the physiological dysfunctions responsible for producing these effects. In this context, it is described that a baroreflex dysfunction could lead to a sympathetic overactivity and subsequent development of hypertension (Souza et al., 2001; Heusser et al., 2010; Tsyrlin et al., 2013). However, the hypothesis that maternal protein restriction leads to baroreflex dysfunction has not been proved yet.
Nowadays, it is well accepted that perinatal protein malnutrition raise risks of hypertension by mechanisms that include reduced nephron morphology and function, and dysfunction on the renin-angiotensin system (Chen et al., 2010; Siddique et al., 2014). However, other hypotheses have been highlighted considering the role of sympathetic overactivity and the development of hypertension in organisms that suffered perinatal malnutrition. In different models of hypertension, it has been suggested that changes in the generation or modulation of respiratory function can contribute to the development of arterial hypertension (Simms et al., 2009, 2010; Costa-Silva et al., 2012; Moraes et al., 2014). Indeed, respiratory neurons located into the brainstem may modulate the sympathetic nervous system and the levels of arterial pressure by central pathways (Costa-Silva et al., 2009, 2010, 2012; Moraes et al., 2014). These neurons receive strong influences from peripheral respiratory chemoreceptors, located into CB at the aortic and carotid arteries. Activation of these chemoreceptors produces a powerful activation of the cardiorespiratory neuronal network and enhances the sympathetic outflow and respiratory drive, which are essential to cardiovascular and ventilatory stability and for providing a correct O2 delivery to cells (Costa-Silva et al., 2010, 2012; Moraes et al., 2014). Thus, it has been suggested that CB dysfunction induced by phenotypic plasticity at the early life can lead to autonomic and ventilatory disorders (Nanduri and Prabhakar, 2015; Prabhakar et al., 2015).
Recently, experimental studies showed that maternal protein restriction during pregnancy and lactation leads to relevant short-term effects on the CB sensitivity and respiratory control of the offspring (de Brito Alves et al., 2014, 2015). Maternal protein restriction is able to induce high phrenic burst frequency and amplitude, leading to increased baseline respiratory frequency (up to 28%) and ventilation (up to 40%) (de Brito Alves et al., 2014, 2015). Further, studies in situ also observed that these respiratory dysfunctions are associated with enhanced baseline sympathetic activity and amplified ventilatory and sympathetic responses to peripheral chemoreflex activation prior to the establishment of hypertension, and high ventilatory responses to hypoxia (de Brito Alves et al., 2015). Therefore, these data strongly support the hypothesis that protein-restricted rats have respiratory dysfunction, which was associated with sympathetic overactivity and enhanced CB sensitivity to hypoxia. Interestingly, this sympathetic-respiratory overactivity was associated with high levels of hypoxic inducible factor (HIF-1α) in CB peripheral chemoreceptor (de Brito Alves et al., 2015). Increased HIF-1α expression was previously observed in heart and brain from the protein-restricted animals (Ito et al., 2011, 2012) and support the notion that a high expression of this transcriptional factor (cellular response to hypoxia), is associated with enhanced sensory activity of the peripheral chemoreceptors, autonomic dysfunction, sympathetic overactivity, and increased risk of hypertension in the offspring subjected to maternal-protein restriction (Figure 2). However, the underlying mechanism involved in the HIF-1α up-regulation in protein-restricted rats is still unclear, but it is hypothesized that epigenetic mechanism produced by DNA methylation could be involved (Altobelli et al., 2013; Prabhakar, 2013; Nanduri and Prabhakar, 2015). Taken together, these studies reinforce the notion that the augmented afferent inputs from the CB (peripheral respiratory chemoreceptors) to brainstem and enhanced sympathetic outflow to the kidney, heart and blood vessels are highlighted as new insights on the maternal diet induced-hypertension, which may lead to increased blood pressure in the adult offspring subjected to maternal protein restriction.
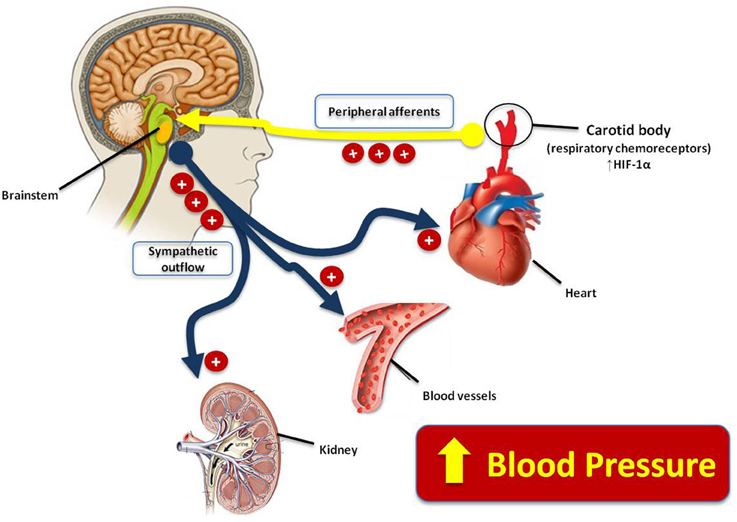
Figure 2. Schematic drawing showing the new insights on the maternal diet induced-hypertension, and the influence of the augmented afferent inputs from the carotid body (peripheral respiratory chemoreceptors) to brainstem and enhanced sympathetic outflow to the kidney, heart and blood vessels, which may lead to increased blood pressure in the adult offspring subjected to maternal protein restriction.
Conclusion
The etiology of the SAH is multifactorial involving genetic influences and the physiological integration of cardiovascular, renal, neural, and endocrine systems. Environmental stimuli are also strongly related to the high prevalence of SAH. Recently, it was recognize that perinatal malnutrition is related with the risk of developing metabolic syndrome and hypertension in adult life. The underlying mechanism can be explained in the context of phenotypic plasticity during development that includes adaptive change on the renal morphology and physiology with subsequent arterial hypertension. Moreover, maternal protein restriction may alter the central control of SAH by a mechanism that include enhanced sympathetic-respiratory activities and respiratory dysfunction at early life, which may contribute to adult hypertension. There are experimental evidences that respiratory dysfunction may be associated with both sympathetic overactivity and the high levels of HIF-1α in CB peripheral chemoreceptor.
Author Contributions
JC and CL drafted the work and revised critically for important intellectual content; wrote the paper; Final review of the manuscript. JB, VO, MA, KP, AO, IN, JF contributions to the conception of the work; Final review of the manuscript.
Conflict of Interest Statement
The authors declare that the research was conducted in the absence of any commercial or financial relationships that could be construed as a potential conflict of interest.
Acknowledgments
This study was supported by National Council for Scientific and Technological Development (CNPq, GRANT n° 477915/2012-4; 459341/2014-6), Coordination for the Improvement of Higher Level—or Education—Personnel (CAPES, GRANT n° 2317/2008), and State of Pernambuco Science and Technology Support Foundation (FACEPE, GRANT n° APQ-0877-4.09/12).
Abbreviations
SAH, systemic arterial hypertension; BP, Blood pressure; CB, carotid bodies; HF, High frequency; LF, low frequency; VLF, Very low frequency; AP, Arterial pressure; SAP, systemic arterial pressure.
References
Altobelli, G., Bogdarina, I. G., Stupka, E., Clark, A. J., and Langley-Evans, S. (2013). Genome-wide methylation and gene expression changes in newborn rats following maternal protein restriction and reversal by folic acid. PLoS ONE 8:e82989. doi: 10.1371/journal.pone.0082989
Andersen, L. G., Angquist, L., Gamborg, M., Byberg, L., Bengtsson, C., Canoy, D., et al. (2009). Birth weight in relation to leisure time physical activity in adolescence and adulthood: meta-analysis of results from 13 nordic cohorts. PLoS One 4:e8192. doi: 10.1371/journal.pone.0008192
Antony, G. M., and Laxmaiah, A. (2008). Human development, poverty, health & nutrition situation in India. Indian J. Med. Res. 128, 198–205.
Ashton, N. (2000). Perinatal development and adult blood pressure. Braz. J. Med. Biol. Res. 33, 731–740. doi: 10.1590/S0100-879X2000000700002
Barker, D. J., Osmond, C., Forsen, T. J., Kajantie, E., and Eriksson, J. G. (2005). Trajectories of growth among children who have coronary events as adults. N. Engl. J. Med. 353, 1802–1809. doi: 10.1056/NEJMoa044160
Barros, M. A., de Brito Alves, J. L., Nogueira, V. O., Wanderley, A. G., and Costa-Silva, J. H. (2015). Maternal low-protein diet induces changes in the cardiovascular autonomic modulation in male rat offspring. Nutr. Metab. Cardiovasc. Dis. 25, 123–130. doi: 10.1016/j.numecd.2014.07.011
Biosca, M., Rodriguez, G., Ventura, P., Samper, M. P., Labayen, I., Collado, M. P., et al. (2011). Central adiposity in children born small and large for gestational age. Nutr. Hosp. 26, 971–976. doi: 10.1590/S0212-16112011000500008
Chen, J. H., Tarry-Adkins, J. L., Matharu, K., Yeo, G. S., and Ozanne, S. E. (2010). Maternal protein restriction affects gene expression profiles in the kidney at weaning with implications for the regulation of renal function and lifespan. Clin. Sci. (Lond). 119, 373–384. doi: 10.1042/CS20100230
Conde, W. L., and Monteiro, C. A. (2014). Nutrition transition and double burden of undernutrition and excess of weight in Brazil. Am. J. Clin. Nutr. 100, 1617S–1622S. doi: 10.3945/ajcn.114.084764
Costa-Silva, J. H., Silva, P. A., Pedi, N., Luzardo, R., Einicker-Lamas, M., Lara, L. S., et al. (2009). Chronic undernutrition alters renal active Na+ transport in young rats: potential hidden basis for pathophysiological alterations in adulthood? Eur. J. Nutr. 48, 437–445. doi: 10.1007/s00394-009-0032-z
Costa-Silva, J. H., Zoccal, D. B., and Machado, B. H. (2010). Glutamatergic antagonism in the NTS decreases post-inspiratory drive and changes phrenic and sympathetic coupling during chemoreflex activation. J. Neurophysiol. 103, 2095–2106. doi: 10.1152/jn.00802.2009
Costa-Silva, J. H., Zoccal, D. B., and Machado, B. H. (2012). Chronic intermittent hypoxia alters glutamatergic control of sympathetic and respiratory activities in the commissural NTS of rats. Am. J. Physiol. Regul. Integr. Comp. Physiol. 302, R785–R793. doi: 10.1152/ajpregu.00363.2011
de Brito Alves, J. L., Nogueira, V. O., Cavalcanti Neto, M. P., Leopoldino, A. M., Curti, C., Colombari, D. S., et al. (2015). Maternal protein restriction increases respiratory and sympathetic activities and sensitizes peripheral chemoreflex in male rat offspring. J. Nutr. 145, 907–914. doi: 10.3945/jn.114.202804
de Brito Alves, J. L., Nogueira, V. O., de Oliveira, G. B., da Silva, G. S., Wanderley, A. G., Leandro, C. G., et al. (2014). Short- and long-term effects of a maternal low-protein diet on ventilation, O(2)/CO(2) chemoreception and arterial blood pressure in male rat offspring. Br. J. Nutr. 111, 606–615. doi: 10.1017/S0007114513002833
Falcão-Tebas, F., Bento-Santos, A., Fidalgo, M. A., de Almeida, M. B., dos Santos, J. A., Lopes de Souza, S., et al. (2012). Maternal low-protein diet-induced delayed reflex ontogeny is attenuated by moderate physical training during gestation in rats. Br. J. Nutr. 107, 372–377. doi: 10.1017/S0007114511002947
Fidalgo, M., Falcão-Tebas, F., Bento-Santos, A., de Oliveira, E., Nogueira-Neto, J. F., de Moura, E. G., et al. (2013). Programmed changes in the adult rat offspring caused by maternal protein restriction during gestation and lactation are attenuated by maternal moderate-low physical training. Br. J. Nutr. 109, 449–456. doi: 10.1017/S0007114512001316
Franco, M. C., Casarini, D. E., Carneiro-Ramos, M. S., Sawaya, A. L., Barreto-Chaves, M. L., and Sesso, R. (2008). Circulating renin-angiotensin system and catecholamines in childhood: is there a role for birthweight? Clin. Sci. (Lond). 114, 375–380. doi: 10.1042/CS20070284
Hales, C. N., and Barker, D. J. (1992). Type 2 (non-insulin-dependent) diabetes mellitus: the thrifty phenotype hypothesis. Diabetologia 35, 595–601. doi: 10.1007/BF00400248
Hales, C. N., Barker, D. J., Clark, P. M., Cox, L. J., Fall, C., Osmond, C., et al. (1991). Fetal and infant growth and impaired glucose tolerance at age 64. BMJ 303, 1019–1022. doi: 10.1136/bmj.303.6809.1019
Hemachandra, A. H., Klebanoff, M. A., Duggan, A. K., Hardy, J. B., and Furth, S. L. (2006). The association between intrauterine growth restriction in the full-term infant and high blood pressure at age 7 years: results from the Collaborative Perinatal Project. Int. J. Epidemiol. 35, 871–877. doi: 10.1093/ije/dyl080
Heusser, K., Tank, J., Engeli, S., Diedrich, A., Menne, J., Eckert, S. Jordan, J., et al. (2010). Carotid baroreceptor stimulation, sympathetic activity, baroreflex function, and blood pressure in hypertensive patients. Hypertension 55, 619–626. doi: 10.1161/HYPERTENSIONAHA.109.140665
Ito, T., Funamoto, K., Sato, N., Nakamura, A., Tanabe, K., Hoshiai, T., et al. (2012). Maternal undernutrition induces the expression of hypoxia-related genes in the fetal brain. Tohoku J. Exp. Med. 226, 37–44. doi: 10.1620/tjem.226.37
Ito, T., Tanabe, K., Nakamura, A., Funamoto, K., Aoyagi, A., Sato, K., et al. (2011). Aberrant expression of hypoxia-inducible factor 1alpha in the fetal heart is associated with maternal undernutrition. Tohoku J. Exp. Med. 224, 163–171. doi: 10.1620/tjem.224.163
Japundzic-Zigon, N. (1998). Physiological mechanisms in regulation of blood pressure fast frequency variations. Clin. Exp. Hypertens. 20, 359–388. doi: 10.3109/10641969809053219
Johansson, S., Norman, M., Legnevall, L., Dalmaz, Y., Lagercrantz, H., and Vanpée, M. (2007). Increased catecholamines and heart rate in children with low birth weight: perinatal contributions to sympathoadrenal overactivity. J. Intern. Med. 261, 480–487. doi: 10.1111/j.1365-2796.2007.01776.x
Julien, C. (2006). The enigma of Mayer waves: facts and models. Cardiovasc. Res. 70, 12–21. doi: 10.1016/j.cardiores.2005.11.008
Labayen, I., Moreno, L. A., Blay, M. G., Blay, V. A., Mesana, M. I., González-Gross, M., et al. (2006). Early programming of body composition and fat distribution in adolescents. J. Nutr. 136, 147–152.
Landsberg, L., Aronne, L. J., Beilin, L. J., Burke, V., Igel, L. I., Lloyd-Jones, D., et al. (2013). Obesity-related hypertension: pathogenesis, cardiovascular risk, and treatment–a position paper of the The Obesity Society and The American Society of Hypertension. Obesity 21, 8–24. doi: 10.1002/oby.20181
Lucas, A. (1998). Programming by early nutrition: an experimental approach. J. Nutr. 128(2 Suppl.), 401S–406S.
Moraes, D. J., Bonagamba, L. G., Costa, K. M., Costa-Silva, J. H., Zoccal, D. B., and Machado, B. H. (2014). Short-term sustained hypoxia induces changes in the coupling of sympathetic and respiratory activities in rats. J. Physiol. 592(Pt 9), 2013–2033. doi: 10.1113/jphysiol.2013.262212
Nanduri, J., and Prabhakar, N. R. (2015). Epigenetic regulation of carotid body oxygen sensing: clinical implications. Adv. Exp. Med. Biol. 860, 1–8. doi: 10.1007/978-3-319-18440-1_1
Nuyt, A. M. (2008). Mechanisms underlying developmental programming of elevated blood pressure and vascular dysfunction: evidence from human studies and experimental animal models. Clin. Sci. (Lond). 114, 1–17. doi: 10.1042/CS20070113
Nuyt, A. M., and Alexander, B. T. (2009). Developmental programming and hypertension. Curr. Opin. Nephrol. Hypertens. 18, 144–152. doi: 10.1097/MNH.0b013e328326092c
Ozanne, S. E., and Hales, C. N. (2004). Lifespan: catch-up growth and obesity in male mice. Nature 427, 411–412. doi: 10.1038/427411b
Parra, D. C., Iannotti, L., Gomez, L. F., Pachón, H., Haire-Joshu, D., Sarmiento, O. L., et al. (2015). The nutrition transition in Colombia over a decade: a novel household classification system of anthropometric measures. Arch. Public Health 73, 12. doi: 10.1186/s13690-014-0057-5
Prabhakar, N. R. (2013). Sensing hypoxia: physiology, genetics and epigenetics. J. Physiol. 591(Pt 9), 2245–2257. doi: 10.1113/jphysiol.2012.247759
Prabhakar, N. R., Peng, Y. J., Kumar, G. K., and Nanduri, J. (2015). Peripheral chemoreception and arterial pressure responses to intermittent hypoxia. Compr. Physiol. 5, 561–577. doi: 10.1002/cphy.c140039
Ravelli, G. P., Stein, Z. A., and Susser, M. W. (1976). Obesity in young men after famine exposure in utero and early infancy. N. Engl. J. Med. 295, 349–353. doi: 10.1056/NEJM197608122950701
Sawaya, A. L., Martins, P. A., Grillo, L. P., and Florêncio, T. T. (2004). Long-term effects of early malnutrition on body weight regulation. Nutr. Rev. 62(7 Pt 2), S127–S133. doi: 10.1301/nr.2004.jul.S127-S133
Sawaya, A. L., and Roberts, S. (2003). Stunting and future risk of obesity: principal physiological mechanisms. Cad. Saude. Publica. 19(Suppl. 1), S21–S28. doi: 10.1590/S0102-311X2003000700003
Siddique, K., Guzman, G. L., Gattineni, J., and Baum, M. (2014). Effect of postnatal maternal protein intake on prenatal programming of hypertension. Reprod. Sci. 21, 1499–1507. doi: 10.1177/1933719114530186
Simms, A. E., Paton, J. F., Allen, A. M., and Pickering, A. E. (2010). Is augmented central respiratory-sympathetic coupling involved in the generation of hypertension? Respir. Physiol. Neurobiol. 174, 89–97. doi: 10.1016/j.resp.2010.07.010
Simms, A. E., Paton, J. F., Pickering, A. E., and Allen, A. M. (2009). Amplified respiratory-sympathetic coupling in the spontaneously hypertensive rat: does it contribute to hypertension? J. Physiol. 587(Pt 3), 597–610. doi: 10.1113/jphysiol.2008.165902
Souza, H. C., Ballejo, G., Salgado, M. C., Da Silva, V. J., and Salgado, H. C. (2001). Cardiac sympathetic overactivity and decreased baroreflex sensitivity in L-NAME hypertensive rats. Am. J. Physiol. Heart Circ. Physiol. 280, H844–H850.
Tseng, W. T., Chen, R. F., Tsai, M. L., and Yen, C. T. (2009). Correlation of discharges of rostral ventrolateral medullary neurons with the low-frequency sympathetic rhythm in rats. Neurosci. Lett. 454, 22–27. doi: 10.1016/j.neulet.2009.02.057
Tsyrlin, V. A., Galagudza, M. M., Kuzmenko, N. V., Pliss, M. G., Rubanova, N. S., and Shcherbin, Y. I. (2013). Arterial baroreceptor reflex counteracts long-term blood pressure increase in the rat model of renovascular hypertension. PLoS ONE 8:e64788. doi: 10.1371/journal.pone.0064788
Victora, C. G., Adair, L., Fall, C., Hallal, P. C., Martorell, R., Richter, L., et al. (2008). Maternal and child undernutrition: consequences for adult health and human capital. Lancet 371, 340–357. doi: 10.1016/S0140-6736(07)61692-4
Wells, J. C. (2011). The thrifty phenotype: an adaptation in growth or metabolism? Am. J. Hum. Biol. 23, 65–75. doi: 10.1002/ajhb.21100
Wells, J. C. (2012). Obesity as malnutrition: the role of capitalism in the obesity global epidemic. Am. J. Hum. Biol. 24, 261–276. doi: 10.1002/ajhb.22253
Keywords: hypertension, developmental plasticity, perinatal nutrition, respiratory control, protein restriction
Citation: Costa-Silva JH, de Brito-Alves JL, Barros MAV, Nogueira VO, Paulino-Silva KM, de Oliveira-Lira A, Nobre IG, Fragoso J and Leandro CG (2015) New Insights on the Maternal Diet Induced-Hypertension: Potential Role of the Phenotypic Plasticity and Sympathetic-Respiratory Overactivity. Front. Physiol. 6:345. doi: 10.3389/fphys.2015.00345
Received: 02 August 2015; Accepted: 06 November 2015;
Published: 24 November 2015.
Edited by:
Eugene Nalivaiko, University of Newcastle, AustraliaReviewed by:
Marco Antônio Peliky Fontes, Universidade Federal de Minas Gerais, BrazilValdir Andrade Braga, Federal University of Paraiba, Brazil
Copyright © 2015 Costa-Silva, de Brito-Alves, Barros, Nogueira, Paulino-Silva, de Oliveira-Lira, Nobre, Fragoso and Leandro. This is an open-access article distributed under the terms of the Creative Commons Attribution License (CC BY). The use, distribution or reproduction in other forums is permitted, provided the original author(s) or licensor are credited and that the original publication in this journal is cited, in accordance with accepted academic practice. No use, distribution or reproduction is permitted which does not comply with these terms.
*Correspondence: João H. Costa-Silva, am9hby5oY3NpbHZhQHVmcGUuYnI=