- 1Department of Physiology, University of Munich, Munich, Germany
- 2Medical Clinic V, University Hospital Mannheim, University of Heidelberg, Mannheim, Germany
The osmosensitive transcription factor nuclear factor of activated T-cells 5 (NFAT5), also known as tonicity enhancer element binding protein (TonEBP) plays a crucial role in protection of renal medullary cells against hyperosmotic stress, urinary concentration, the adaptive immune response, and other physiological systems. Since it is also important for development, conventional homozygous-null mutations result in perinatal death, which hinders the analysis of NFAT5 function in specific tissues in vivo. Here we describe the generation of mice with a conditional-null allele, in which loxP sites are inserted around exon 4. Mice harboring the floxed allele (NFAT5flx) were mated to a strain expressing a tamoxifen-inducible derivative of the Cre-recombinase (Cre+) under the control of the ubiqitinC promoter. The resultant homozygous conditional knockout mice (Cre+ NFAT5flx/flx) are viable, fertile, and show normal expression of NFAT5 and NFAT5 target genes, indicating that the conditional alleles retain their wild-type function. Induction of Cre-mediated recombination by administration of tamoxifen in 8-week-old mice resulted in a decrease in NFAT5 expression of about 70–90% in all tested tissues (renal cortex, renal outer medulla, renal inner medulla, heart, lung, spleen, skeletal muscle). Accordingly, the expression of the NFAT5 target genes aldose reductase and heat shock protein 70 in the renal medulla was also significantly decreased. Mice harboring this conditional knockout allele should be useful in future studies for gaining a better understanding of tissue and cell-type specific functions of NFAT5 in adult animals under physiological and pathophysiological conditions.
Introduction
The osmosensitive transcription factor tonicity-responsive enhancer binding protein (TonEBP) was first identified in renal medullary cells. Based on its homology to nuclear factors of activated T cells (NFAT) 1–4, TonEBP is also referred to as NFAT5. NFAT5 drives the expression of various genes that are important for the function of the renal medulla. On the one hand, NFAT5 regulates the expression of central components of the urinary concentrating mechanism, including the kidney-specific ClC-K1 chloride channels and their functional subunit barttin (Küper et al., 2012b), and UT-A1 urea transporters (Nakayama et al., 2000). Accordingly, NFAT5 is a prerequisite for building up the cortico-medullary osmotic gradient by the interstitial accumulation of NaCl and urea in the renal papilla. NFAT5 is further indispensible for the production of concentrated urine during antidiuresis by virtue of its regulation of the transcription of aquaporin-2 (AQP-2) water channels in collecting duct principle cells (Hasler et al., 2006; Hasler, 2011). NFAT5 is, moreover, essential for the osmoadaptation of cells in the renal medulla. In this kidney region, NFAT5 stimulates the expression of transporters and biosynthetic enzymes for cellular accumulation of organic osmolytes. These include the Na-myo-inositol cotransporter (SMIT) (Miyakawa et al., 1999), the Na-Cl-betaine cotransporter (BGT1) (Miyakawa et al., 1998), the Na-Cl-taurine cotransporter (TauT) (Ito et al., 2006), aldose reductase (AR) (Nadkarni et al., 1999), specific heat shock proteins (Woo et al., 2002), and others. The intracellular accumulation of organic osmolytes and heat shock protein 70 (HSP70) protect cells from the adverse effects of hypertonicity.
In past years, it has become increasingly apparent that, in addition to its role in the urinary concentration mechanism and in protection against hyperosmotic stress, NFAT5 is also involved in diverse other physiological and pathophysiological processes. NFAT5 drives for instance the osmotic stress-induced expression of proinflammatory cytokines LT-β, Il-6, and TNF-α in macrophages (Lopez-Rodriguez et al., 2001; Buxade et al., 2012) and of the chemokine MCP-1 (CCL2) in epithelial cells during inflammatory processes (Kojima et al., 2010; Roth et al., 2010; Küper et al., 2012a), indicating that NFAT5 enhances the proinflammatory immune response under pathophysiological conditions associated with hyperosmotic stress. In breast and colon carcinoma, Integrin-mediated activation of NFAT5 stimulates the expression of S100A4, thereby promoting metastatic activity of tumor cells (Jauliac et al., 2002; Chen and O'Connor, 2005; Chen et al., 2009, 2011). Knockdown of NFAT5 in T-lymphocytes challenged with osmotic stress results in downregulation of cyclins E1, A2, and B1, which is associated with cell cycle arrest (Drews-Elger et al., 2009). In skeletal muscle cells, NFAT5 regulates expression of Cyr61, thereby controlling migration and differentiation of myoblasts (O'Connor et al., 2007). In the placenta, NFAT5 stimulates the expression of SMIT, AR, and HSP70 (Arroyo et al., 2009, 2012; Park et al., 2014).
Most of these findings have been derived from cell culture experiments. Conventional NFAT5 knockout mice show high perinatal lethality due to impaired renal (Lopez-Rodriguez et al., 2004) and heart (Mak et al., 2011) development. Furthermore, these animals show an impaired immune response (Trama et al., 2002; Go et al., 2004). Thus, conventional NFAT5 knockout mice are of limited use for analysis of NFAT5 function in adult animals. The goal of the present study was to create a conditional NFAT5 knockout allele to overcome the problems of conventional NFAT5 knockout mice. For this purpose, we constructed a NFAT5 allele, in which exon 4 is flanked by loxP sequences (NFAT5flx). Cre-mediated recombination of this allele leads to knockdown of NFAT5. By mating NFAT5flx mice with a mouse strain expressing a tamoxifen-inducible Cre recombinase, we generated a mouse strain in which NFAT5 can be knocked down in adult animals by tamoxifen administration.
Materials and Methods
Construction of a Conditional Targeting Vector for NFAT5
The conditional NFAT5 allele was created in collaboration with Ingenious Targeting Laboratory, Inc., (Stony Brook, NY, USA). A 8.26-Kb region from a positively identified C57BL/6 BAC clone (RP23: 187J8) was used to construct the targeting vector. The region was subcloned into a pSP72 vector (Promega, Madison, WI, USA) containing an ampicillin selection cassette. The short homology arm (SA) of the region was designed such that it extended about 2.45 kb 3′ to exon 4 of the NFAT5 gene. The long homology arm (LA) ended 5′ to exon 4, and was 5.25 kb long. A pGK-gb2 loxP/frt Neo cassette was inserted 202 bp downstream from exon 4. The Neo cassette contained the promoter of the mouse phosphoglucokinase gene, a neomycin resistance gene, and a synthetic polyadenylation sequence, and was flanked by frt sites for later excision by Flp recombinase. The cassette also contained two loxP sites, one within the frt sites, and another one at the 3′ end of the cassette, outside the frt sites. An additional single loxP site (third loxP site), containing engineered KpnI, EcoRI, MscI and StuI sites for southern blot analysis, was inserted 166 bp upstream from exon 4. The length of the target region was 561 bp and included exon 4; the total size of the targeting construct (including vector backbone and Neo cassette) was 12.4 kb. The targeting vector was confirmed by restriction analysis after each modification step. P6 and T7 primers (see Table 1) anneal to the vector sequence and read into the 5′ and 3′ ends of the BAC sub clone. N1 and N2 primers anneal to the 5′ and 3′ ends of the loxP/frt Neo cassette and sequence the SA and LA, respectively.
Targeted ES Cells and Generation of Chimeric Mice
The targeting vector (10 μg) was linearized by NotI and then transfected by electroporation of BA1(C57BL/6x129/SvEv) hybrid embryonic stem (ES) cells. After selection with G418 antibiotic, surviving clones were expanded for PCR analysis to identify recombinant ES clones. ES cell colonies were first screened by PCR, using primers A1 or A2, binding downstream from the SA, and the primer F3, binding within the Neo cassette. Size of the resulting PCR products was 2.57 or 2.73 kb, respectively. A control PCR reaction was performed using the 3′-primer AT1 and the 5′-primer AT2, which bind downstream from the Neo cassette within the SA. The size of the resulting PCR product was 2.25 kb.
PCR Confirmation of the Third loxP Site
PCR was performed on SA positive clones to detect presence of the third loxP site (5′-region of exon 4) using the LOX2 and SDL2 primers. This reaction amplifies a 334-bp wild-type product. The presence of a second PCR product 392 bp in size indicates a positive loxP PCR. Positive clones were sequenced to confirm sequence integrity and presence of the third loxP site using the SDL2 primer.
Reconfirmation of Expanded Clones by Southern Blot
Four individual clones that had passed all controls were reconfirmed by Southern blot analysis. DNA was digested with StuI, and electrophoretically separated on a 0.8% agarose gel. After transfer to a nylon membrane, the digested DNA was hybridized with a probe targeted against the 5′ external region of the LA, resulting in detection of two bands at 6.7 kb (targeted allele), and 9.5 kb (wild-type allele). Positive clones were further confirmed by Southern blot analysis using an internal probe. DNA was digested with PvuII, and electrophoretically separated. After transfer to a nylon membrane, the digested DNA was hybridized with a probe targeted against the 3′ internal region of the SA, resulting in detection of two bands at 3.5 kb (targeted allele), and 9.2 kb (wild-type allele). DNA from C57Bl/6 (B6), 129/SvEv (129), and BA1 (C57Bl/6 × 129/SvEv) (Hybrid) mouse strains were used as wild-type controls.
Targeted iTL BA1 hybrid embryonic stem cells were microinjected into C57BL/6 blastocysts. Resulting chimeras with a high percentage of agouti coat color were mated to wild-type C57BL/6 homozygous Flp mice to remove the Neo cassette. Tail DNA was analyzed from pups with agouti or black coat color. Presence of the third loxP site was screened by PCR using LOX2 and SDL2 primers; presence and correct integration of the third loxP site was also confirmed by sequencing using LOX2 primer. Presence and confirmation of the SA was screened using primers A3 and F3, resulting in a 2.5-kb amplification product. Primers NDEL1 and NDEL2 were used to screen the genomic DNA for deletion of the Neo cassette. This reaction amplifies a 492-bp wild-type product, the presence of a second 675 bp PCR product indicates a targeted allele with deletion of the Neo cassette. Presence of the Neo cassette resulted in no amplification product because of its too big size. Finally, heterozygous F1 mice with confirmed loxP-sites and Neo deletion were back-crossed with C57/BL6 mice for 10 generations to obtain heterozygous mice with a >99.9% C57/BL6 background.
Knockdown of NFAT5
Mice carrying an NFAT5 allele with a loxP-flanked exon 4 (NFAT5flx) were mated to the strain B6.Cg-Tg(UBC-cre/ERT2)1Ejb/J (Jackson Laboratory, Bar Harbor, ME, USA) harboring a Cre-ERT2 transgene under control of the ubiquitinC promoter. Cre+ NFAT5flx/+ mice were interbred, and Cre+ NFAT5flx/flx mice were used for knockdown of NFAT5. To induce the knockdown, 6-week-old mice were fed a tamoxifen-containing (400 mg/kg) diet (Cre-active 400, Lasvendi, Soest, Germany) for 4 weeks. Subsequently, mice were sacrificed, and various tissues (kidney (cortex, outer medulla, inner medulla), liver, heart, spleen, lung) were snap frozen in liquid nitrogen and later analyzed for expression of NFAT5 and NFAT5 target genes. All animal experiments were in accordance with the institutional and the national guidelines and regulations. The experimental procedures were approved by the institutional animal health and care committee.
qRT-PCR Analysis
For determination of NFAT5, AR, HSP70, BGT-1, and β-actin mRNA expression levels, tissues were homogenized in TRIFAST Reagent (Peqlab, Erlangen, Germany), and RNA was isolated as recommended by the manufacturer. Oligonucleotides used in this experiment are listed in Table 1. Experiments were carried out on a Roche LightCycler 480, using the SensiMix SYBR One-Step Kit (Bioline, Luckenwalde, Germany) according to the manufacturer's recommendations. Specificity of PCR product formation was confirmed by monitoring melting point analysis and by agarose gel electrophoresis.
Immunoblot Analysis
Antibodies against NFAT5 and actin were obtained from Sigma (Deisenhofen, Germany); antibody against HSP70 was obtained from Stressgen (Victoria, Canada); antibody against rat lens aldose reductase was a kind gift from Peter F. Kador. Tissues were homogenized in 8 M urea buffer. Aliquots (5–30 μg protein) were subjected to sodium dodecylsulphate polyacrylamide gel-electrophoresis (SDS-PAGE) and blotted onto nitrocellulose membranes (Amersham Pharmacia Biotech, Little Chalfont, Bucks., UK). Non-specific binding sites were blocked with 5% non-fat dry milk in PBS containing 0.1% Tween-20 (PBS-T) at room temperature for 1 h. Samples were incubated with primary antibodies in PBS-T containing 5% non-fat dry milk over night at 4°C. Subsequently, the blots were washed 3 times with PBS-T for 5 min each. Subsequently, the membranes were incubated with appropriate secondary antibody at room temperature for 1 h in PBS-T containing 5% non-fat dry milk. After washing with PBS-T 3 times for 5 min each, immunocomplexes were visualized by enhanced chemiluminescence (Pierce, Rockford, IL, USA).
Statistical Analyses
Data are expressed as means ± SEM. The significance of differences between the means was assessed by Student's t-test. P < 0.05 was regarded as significant. All experiments were performed at least three times and representative results are shown.
Results and Discussion
The murine NFAT5 gene is located on the plus (+) strand of chromosome 8, spans about 86 kb, and is divided into 16 exons. To generate a functional NFAT5-null allele in mice, exon 4 was chosen as target for conditional deletion by insertion of flanking loxP sites. Exon 4 shares 100% sequence homology with the human NFAT5 exon 4, the perfect homology indicating a highly conserved function for the protein sequence encoded by this exon; additionally, deletion of exon 4 results in a frameshift between exons 3 and 5. To generate the conditional NFAT5-null (NFAT5flx) allele in mice, a genomic DNA fragment containing the relevant region was isolated from the murine BAC library; a loxP sequence was inserted 167 bp upstream from exon 4, and an frt-flanked Neo expression cassette containing two loxP sites was inserted 202 bp downstream from exon 4 to enable positive selection (Figure 1A). The resulting target vector was transfected into TL1 mouse embryonic stem (ES); then, the ES cell colonies were selected with G418, screened via PCR and Southern blot. Chimeric mice were generated by microinjecting NFAT5flx-neo/+ ES cells (Figure 1B) into C57BL/6 blastocysts, and germline transmission was verified via obtaining F1 NFAT5flx-neo/+.offspring from crossing chimeric and C57/Bl6 mice. The F1 NFAT5flx-neo/+ mice were crossed with Flp mice to remove the Neo cassette in the germ line and the NFAT5flx/+ offspring then backcrossed with C57/Bl6 wild-type mice over 10 generations to obtain mice with >99.9% C57/Bl6 background. Thereafter, NFAT5flx/+ mice were interbred, and the offspring were genotyped via PCR (Figure 1C). The homozygous NFAT5flx/flx mice appeared normal, were fertile, and were born in the expected Mendelian ratio. NFAT5 expression, as analyzed by qRT-PCR and immunoblotting, was comparable to C57/Bl6 wild-type mice (data not shown).
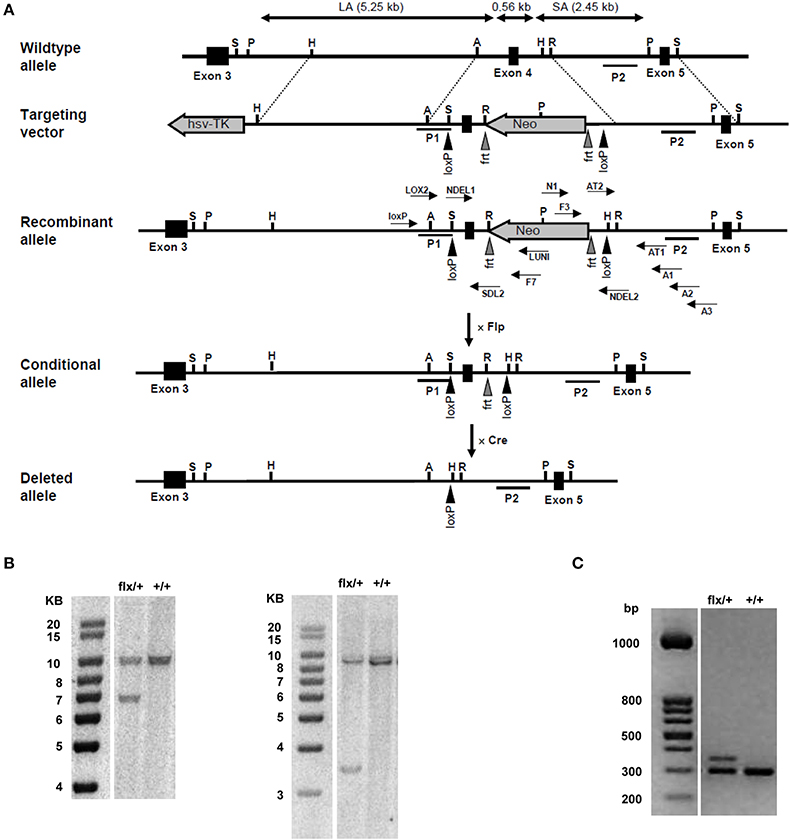
Figure 1. Generation of NFAT5 conditional null allele. (A) Schematic figures of the wild-type NFAT5 allele, the targeting vector, and the targeted NFAT5neo-flx, NFAT5flx, and NFAT5Δ alleles. Exons are represented by open boxes in which the exon number is indicated. Black triangles denote loxP sites; gray triangles denote frt sites. The 5′ homologous arm of the targeting vector is ~ 5.25 kb, the 3′ homologous arm ~ 2.45 kb. P1 and P2 denote the location of the 5′- and the 3′- external probe used for Southern blot analysis. Arrows indicate the position of the primers used for gene targeting and genotyping. NFAT5neo-flx mice were mated with Flp mice to generate NFAT5flx mice. NFAT5Δ mice were generated by crossing NFAT5flx mice with transgenic Cre mice and subsequent tamoxifen administration to targeted offspring. (B) Southern blot analysis of NFAT5neo-flx/+ genomic DNA. 5′- and 3′- external probes were used to detect the presence of the LA (5.25 kb) and the SA (2.45 kb) of the targeted NFAT5 fragment. (C) Genotypes of mice were identified using genomic DNA prepared from tail biopsies. Primers LOX2 and SDL2 were used to distinguish the targeted allele (392 bp) from the untargeted wild-type allele (334 bp).
Next, homozygous NFAT5flx/flx mice were mated to B6.Cg-Tg(UBC-cre/ERT2)1Ejb/J mice. This strain has strong tamoxifen-inducible Cre activity in all reported tissue types. Expression of the cre/ERT2 transgene is under control of the ubiquitin C promoter. The Cre-ERT2 fusion protein consists of Cre recombinase fused to a triple mutant form of the human estrogen receptor, which does not bind its natural ligand (17β-estradiol) at physiological concentrations but will bind the synthetic estrogen receptor ligand tamoxifen. Restricted to the cytoplasm, Cre-ERT2 can only gain access to the nucleus after exposure to tamoxifen, where Cre-mediated recombination will delete loxP-flanked sequences from the genome. The offspring from this mating were genotyped for the presence of the cre transgene and the NFAT5flx allele, and interbred at the age of 8 weeks. Again, the offspring from this mating were genotyped, and cre-positive, homozygous NFAT5flx/flx mice were used for knockdown of NFAT5.
To induce the knockdown, Cre+ NFAT5flx/flx mice (or Cre+ NFAT5+/+ mice as control) were fed a tamoxifen-containing diet for 4 weeks. Subsequently, the animals were sacrificed and tissue samples prepared from liver, spleen, heart, skeletal muscle, and kidney Kidney tissue samples were subdivided into cortical, outer medullary, and inner medullary samples. Renal expression of NFAT5 and the designated NFAT5 target genes AR, HSP70, and BGT-1 was tested by semiquantitative RT-PCR (Figure 2A) and immunoblot (Figure 2B). Highest expression of NFAT5 and NFAT5 target genes in control animals was found in inner medullary tissue samples, as expected. In knockout animals, NFAT5 expression was almost completely abolished in all renal tissue sections whilst expression of NFAT5 target genes was reduced significantly in the inner medulla. Expression in cortical and outer medullary sections was largely unaffected. These results indicate that Cre-mediated recombination of the NFAT5flx allele indeed leads to a knockdown of NFAT5 and under the tested conditions (H2O ad libitum), regulation of osmoprotective target genes by NFAT5 occurs predominantly in the inner medulla, which is in accordance with previous studies (Lopez-Rodriguez et al., 2004). NFAT5 expression in tissue samples of heart, liver, spleen, and skeletal muscle was tested by qRT-PCR (Figure 3), and expression levels were significantly decreased to 15–25% in NFAT5 knockout animals (compared with 100% in control animals).
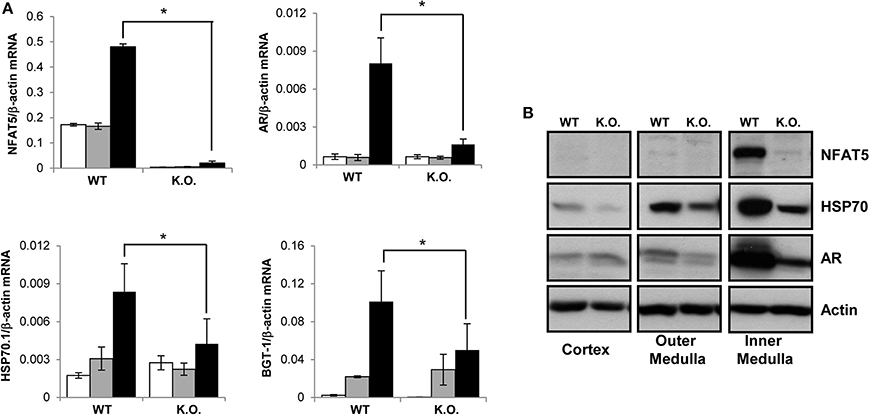
Figure 2. Expression of NFAT5 and NFAT5 target genes in the kidney. Cre+ NFAT5flx/flx mice (or Cre+ NFAT5+/+ mice, as control) were fed a tamoxifen-containing diet for 4 weeks to induce Cre-mediated excision of exon 4 of the NFAT5 gene. Subsequently, animals were sacrificed and tissue samples obtained from the renal cortex, outer medulla and inner medulla. (A) mRNA from renal cortex (☐), outer medulla () and inner medulla (■) was tested for transcription of NFAT5 and NFAT5 target genes AR, BGT-1 and HSP70 by semiquantitative RT-PCR. Relative mRNA abundance was normalized to that of β-actin to correct for differences in RNA input. Data are means ± SEM for n = 4; *P < 0.05. (B) Protein samples from renal cortex, outer medulla and inner medulla were tested for protein expression of NFAT5 and NFAT5 target genes AR and HSP70 by Western blot. To demonstrate comparable protein loading, the blots were also probed for actin. Representative blots from at least 4 independent experiments are shown.
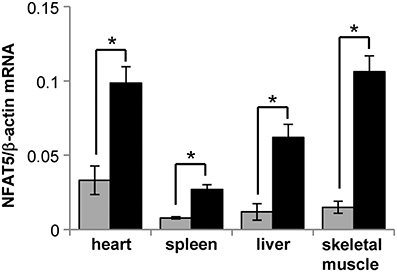
Figure 3. Expression of NFAT5 in various tissues of conditional knockout mice. Cre+ NFAT5flx/flx mice () or Cre+ NFAT5+/+ mice (■; as control) were fed a tamoxifen-containing diet for 4 weeks to induce Cre-mediated excision of exon 4 of the NFAT5 gene. Subsequently, tissue samples from heart, liver, spleen, and skeletal muscle, were taken and expression of NFAT5 was determined by semiquantitative qRT-PCR. Relative mRNA abundance was normalized to that of β-actin to correct for differences in RNA input. Data are means ± SEM for n = 4; *P < 0.05.
In the past, there have been attempts to analyze NFAT5 function in vivo by generation of conventional NFAT5 knockout mice. These experiments clearly demonstrated the importance of NFAT5 for embryonic development, however, there are severe limitations on the use of these animals for defining the functions of NFAT5 in adult animals. Conventional NFAT5−/− animals show high embryonic and perinatal lethality. Until E14.5 no significant deviation from the expected Mendelian ratios can be observed in the number of NFAT5−/− embryos, while only 50% of the expected number is obtained at E17.5, and only 3% at P21 (Lopez-Rodriguez et al., 2004). NFAT5−/− embryos show peripheral edema, probably due to abnormal heart development as indicated by reduced cell density of the myocardium and thinner ventricular wall (Mak et al., 2011). The few animals that reach P21 show congenital growth retardation and suffer from renal atrophy, probably due to decreased expression of NFAT5 target genes like AR, HSP70, BGT-1, and SMIT during kidney development (Lopez-Rodriguez et al., 2004). Hence, the kidney is already severely damaged when the animals reach adulthood, and thus research on NFAT5 function in the adult kidney, e.g., its role in the urinary concentration mechanism, is severely restricted in conventional NFAT5−/− mice. Moreover, these animals develop an immune defect due to lymphoid hypocellularity and impaired antigen-specific antibody responses (Go et al., 2004). Mice that express a dominant-negative derivative of NFAT5 exhibit a 25% reduction of CD4+ T-cells and a 50% reduction of CD8+ T-cells (Trama et al., 2002).
Generally, the fact that NFAT5 has multiple physiological and pathophysiological functions indicates that a conventional knockdown is an inappropriate model for investigations on NFAT5 function in a specific cell type or tissue in vivo, since systemic NFAT5 knockdown may generate unspecific side effects with unpredictable impact on the tissue of interest. Modern Cre-Lox recombination technology would appear to be an appropriate means of overcoming this problem. Today, multiple mouse strains are available, which express constitutively active or inducible Cre recombinase with high specificity in individual cell types and tissues, or at various developmental stages. Thus, combination of such restricted Cre expression with the NFAT5flx allele described in the present study will provide a useful tool for characterizing the tissue-type and cell type-specific functions of NFAT5 in many developmental processes and diseases, as well as physiological functions in adult animals, without the bias produced by unspecific side effects of systemic knockdown.
Conclusions
Taken together, the results indicate that we have been able to generate a conditional NFAT5-null mutant mouse line. The NFAT5flx allele retains the function of the wild-type gene, and inactive NFAT5-null allele can be induced via Cre-mediated recombination. This will allow the study of NFAT5 function in specific cell types and tissues in vivo.
Conflict of Interest Statement
The authors declare that the research was conducted in the absence of any commercial or financial relationships that could be construed as a potential conflict of interest.
Acknowledgments
Work in the authors' laboratory was supported by grants from the Deutsche Forschungsgemeinschaft, the Deutsche Nierenstiftung, the Münchener Medizinische Wochenschrift, and by the Friedrich Baur Stiftung, Munich. The help of Maria-Luisa Fraek is gratefully acknowledged. We thank Dr. John Davies for critical reading of the manuscript.
References
Arroyo, J. A., Garcia-Jones, P., Graham, A., Teng, C. C., Battaglia, F. C., and Galan, H. L. (2012). Placental TonEBP/NFAT5 osmolyte regulation in an ovine model of intrauterine growth restriction. Biol. Reprod. 86, 94. doi: 10.1095/biolreprod.111.094797
Pubmed Abstract | Pubmed Full Text | CrossRef Full Text | Google Scholar
Arroyo, J. A., Teng, C., Battaglia, F. C., and Galan, H. L. (2009). Determination of the NFAT5/TonEBP transcription factor in the human and ovine placenta. Syst. Biol. Reprod. Med. 55, 164–170. doi: 10.3109/19396360902846401
Pubmed Abstract | Pubmed Full Text | CrossRef Full Text | Google Scholar
Buxade, M., Lunazzi, G., Minguillon, J., Iborra, S., Berga-Bolanos, R., Del Val, M., et al. (2012). Gene expression induced by Toll-like receptors in macrophages requires the transcription factor NFAT5. J. Exp. Med. 209, 379–393. doi: 10.1084/jem.20111569
Pubmed Abstract | Pubmed Full Text | CrossRef Full Text | Google Scholar
Chen, M., and O'Connor, K. L. (2005). Integrin alpha6beta4 promotes expression of autotaxin/ENPP2 autocrine motility factor in breast carcinoma cells. Oncogene 24, 5125–5130. doi: 10.1038/sj.onc.1208729
Pubmed Abstract | Pubmed Full Text | CrossRef Full Text | Google Scholar
Chen, M., Sastry, S. K., and O'Connor, K. L. (2011). Src kinase pathway is involved in NFAT5-mediated S100A4 induction by hyperosmotic stress in colon cancer cells. Am. J. Physiol. Cell Physiol. 300, C1155–C1163. doi: 10.1152/ajpcell.00407.2010
Pubmed Abstract | Pubmed Full Text | CrossRef Full Text | Google Scholar
Chen, M., Sinha, M., Luxon, B. A., Bresnick, A. R., and O'Connor, K. L. (2009). Integrin alpha6beta4 controls the expression of genes associated with cell motility, invasion, and metastasis, including S100A4/metastasin. J. Biol. Chem. 284, 1484–1494. doi: 10.1074/jbc.M803997200
Pubmed Abstract | Pubmed Full Text | CrossRef Full Text | Google Scholar
Drews-Elger, K., Ortells, M. C., Rao, A., Lopez-Rodriguez, C., and Aramburu, J. (2009). The transcription factor NFAT5 is required for cyclin expression and cell cycle progression in cells exposed to hypertonic stress. PLoS ONE 4:e5245. doi: 10.1371/journal.pone.0005245
Pubmed Abstract | Pubmed Full Text | CrossRef Full Text | Google Scholar
Go, W. Y., Liu, X., Roti, M. A., Liu, F., and Ho, S. N. (2004). NFAT5/TonEBP mutant mice define osmotic stress as a critical feature of the lymphoid microenvironment. Proc. Natl. Acad. Sci. U.S.A. 101, 10673–10678. doi: 10.1073/pnas.0403139101
Pubmed Abstract | Pubmed Full Text | CrossRef Full Text | Google Scholar
Hasler, U. (2011). An example of functional interaction between NFAT5/TonEBP and nuclear factor-kappaB by hypertonic stress: aquaporin-2 transcription. Cell Cycle 10, 364–365. doi: 10.4161/cc.10.3.14520
Pubmed Abstract | Pubmed Full Text | CrossRef Full Text | Google Scholar
Hasler, U., Jeon, U. S., Kim, J. A., Mordasini, D., Kwon, H. M., Feraille, E., et al. (2006). Tonicity-responsive enhancer binding protein is an essential regulator of aquaporin-2 expression in renal collecting duct principal cells. J. Am. Soc. Nephrol. 17, 1521–1531. doi: 10.1681/ASN.2005121317
Pubmed Abstract | Pubmed Full Text | CrossRef Full Text | Google Scholar
Ito, T., Fujio, Y., Uozumi, Y., Matsuda, T., Maeda, M., Takahashi, K., et al. (2006). TauT gene expression is regulated by TonEBP and plays a role in cell survival. Adv. Exp. Med. Biol. 583, 91–98. doi: 10.1007/978-0-387-33504-9_9
Pubmed Abstract | Pubmed Full Text | CrossRef Full Text | Google Scholar
Jauliac, S., Lopez-Rodriguez, C., Shaw, L. M., Brown, L. F., Rao, A., and Toker, A. (2002). The role of NFAT transcription factors in integrin-mediated carcinoma invasion. Nat. Cell Biol. 4, 540–544. doi: 10.1038/ncb816
Pubmed Abstract | Pubmed Full Text | CrossRef Full Text | Google Scholar
Kojima, R., Taniguchi, H., Tsuzuki, A., Nakamura, K., Sakakura, Y., and Ito, M. (2010). Hypertonicity-induced expression of monocyte chemoattractant protein-1 through a novel cis-acting element and MAPK signaling pathways. J. Immunol. 184, 5253–5262. doi: 10.4049/jimmunol.0901298
Pubmed Abstract | Pubmed Full Text | CrossRef Full Text | Google Scholar
Küper, C., Beck, F. X., and Neuhofer, W. (2012a). NFAT5 contributes to osmolality-induced MCP-1 expression in mesothelial cells. Mediators Inflamm. 2012, 513015. doi: 10.1155/2012/513015
Pubmed Abstract | Pubmed Full Text | CrossRef Full Text | Google Scholar
Küper, C., Fraek, M. L., Muller, H. H., Beck, F. X., and Neuhofer, W. (2012b). Sepsis-induced urinary concentration defect is related to nitric oxide-dependent inactivation of TonEBP/NFAT5, which downregulates renal medullary solute transport proteins and aquaporin-2. Crit. Care Med. 40, 1887–1895. doi: 10.1097/CCM.0b013e31824e1186
Pubmed Abstract | Pubmed Full Text | CrossRef Full Text | Google Scholar
Lopez-Rodriguez, C., Antos, C. L., Shelton, J. M., Richardson, J. A., Lin, F., Novobrantseva, T. I., et al. (2004). Loss of NFAT5 results in renal atrophy and lack of tonicity-responsive gene expression. Proc. Natl. Acad. Sci. U.S.A. 101, 2392–2397. doi: 10.1073/pnas.0308703100
Pubmed Abstract | Pubmed Full Text | CrossRef Full Text | Google Scholar
Lopez-Rodriguez, C., Aramburu, J., Jin, L., Rakeman, A. S., Michino, M., and Rao, A. (2001). Bridging the NFAT and NF-kappaB families: NFAT5 dimerization regulates cytokine gene transcription in response to osmotic stress. Immunity 15, 47–58. doi: 10.1016/S1074-7613(01)00165-0
Pubmed Abstract | Pubmed Full Text | CrossRef Full Text | Google Scholar
Mak, M. C., Lam, K. M., Chan, P. K., Lau, Y. B., Tang, W. H., Yeung, P. K., et al. (2011). Embryonic lethality in mice lacking the nuclear factor of activated T cells 5 protein due to impaired cardiac development and function. PLoS ONE 6:e19186. doi: 10.1371/journal.pone.0019186
Pubmed Abstract | Pubmed Full Text | CrossRef Full Text | Google Scholar
Miyakawa, H., Woo, S. K., Chen, C. P., Dahl, S. C., Handler, J. S., and Kwon, H. M. (1998). Cis- and trans-acting factors regulating transcription of the BGT1 gene in response to hypertonicity. Am. J. Physiol. 274, F753–F761.
Miyakawa, H., Woo, S. K., Dahl, S. C., Handler, J. S., and Kwon, H. M. (1999). Tonicity-responsive enhancer binding protein, a rel-like protein that stimulates transcription in response to hypertonicity. Proc. Natl. Acad. Sci. U.S.A. 96, 2538–2542. doi: 10.1073/pnas.96.5.2538
Pubmed Abstract | Pubmed Full Text | CrossRef Full Text | Google Scholar
Nadkarni, V., Gabbay, K. H., Bohren, K. M., and Sheikh-Hamad, D. (1999). Osmotic response element enhancer activity. Regulation through p38 kinase and mitogen-activated extracellular signal-regulated kinase kinase. J. Biol. Chem. 274, 20185–20190. doi: 10.1074/jbc.274.29.20185
Pubmed Abstract | Pubmed Full Text | CrossRef Full Text | Google Scholar
Nakayama, Y., Peng, T., Sands, J. M., and Bagnasco, S. M. (2000). The TonE/TonEBP pathway mediates tonicity-responsive regulation of UT-A urea transporter expression. J. Biol. Chem. 275, 38275–38280. doi: 10.1074/jbc.M004678200
Pubmed Abstract | Pubmed Full Text | CrossRef Full Text | Google Scholar
O'Connor, R. S., Mills, S. T., Jones, K. A., Ho, S. N., and Pavlath, G. K. (2007). A combinatorial role for NFAT5 in both myoblast migration and differentiation during skeletal muscle myogenesis. J. Cell Sci. 120, 149–159. doi: 10.1242/jcs.03307
Pubmed Abstract | Pubmed Full Text | CrossRef Full Text | Google Scholar
Park, J. K., Kang, T. G., Kang, M. Y., Park, J. E., Cho, I. A., Shin, J. K., et al. (2014). Increased NFAT5 expression stimulates transcription of Hsp70 in preeclamptic placentas. Placenta 35, 109–116. doi: 10.1016/j.placenta.2013.12.005
Pubmed Abstract | Pubmed Full Text | CrossRef Full Text | Google Scholar
Roth, I., Leroy, V., Kwon, H. M., Martin, P. Y., Feraille, E., and Hasler, U. (2010). Osmoprotective transcription factor NFAT5/TonEBP modulates nuclear factor-kappaB activity. Mol. Biol. Cell 21, 3459–3474. doi: 10.1091/mbc.E10-02-0133
Pubmed Abstract | Pubmed Full Text | CrossRef Full Text | Google Scholar
Trama, J., Go, W. Y., and Ho, S. N. (2002). The osmoprotective function of the NFAT5 transcription factor in T cell development and activation. J. Immunol. 169, 5477–5488. doi: 10.4049/jimmunol.169.10.5477
Pubmed Abstract | Pubmed Full Text | CrossRef Full Text | Google Scholar
Woo, S. K., Lee, S. D., Na, K. Y., Park, W. K., and Kwon, H. M. (2002). TonEBP/NFAT5 stimulates transcription of HSP70 in response to hypertonicity. Mol. Cell. Biol. 22, 5753–5760. doi: 10.1128/MCB.22.16.5753-5760.2002
Pubmed Abstract | Pubmed Full Text | CrossRef Full Text | Google Scholar
Keywords: NFAT5, knockout mouse model, TonEBP, osmosensing, kidney physiology
Citation: Küper C, Beck F-X and Neuhofer W (2015) Generation of a conditional knockout allele for the NFAT5 gene in mice. Front. Physiol. 5:507. doi: 10.3389/fphys.2014.00507
Received: 12 September 2014; Accepted: 06 December 2014;
Published online: 05 January 2015.
Edited by:
John W. Osborn, University of Minnesota, USAReviewed by:
Laura Veronica Gonzalez Bosc, University of New Mexico-Health Sciences Center, USAMichael J. Flister, Medical College of Wisconsin, USA
Copyright © 2015 Küper, Beck and Neuhofer. This is an open-access article distributed under the terms of the Creative Commons Attribution License (CC BY). The use, distribution or reproduction in other forums is permitted, provided the original author(s) or licensor are credited and that the original publication in this journal is cited, in accordance with accepted academic practice. No use, distribution or reproduction is permitted which does not comply with these terms.
*Correspondence: Christoph Küper, Department of Physiology, University of Munich, Pettenkoferstrasse 12, 80336 Munich, Germany e-mail: christoph.kueper@lrz.uni-muenchen.de