- 1Department of Anesthesia, Rigshospitalet, University of Copenhagen, Copenhagen, Denmark
- 2Department of Anesthesia and Perioperative Medicine, St. Boniface Hospital, University of Manitoba, Winnipeg, MB, Canada
- 3Department of Surgery and Transplantation, Rigshospitalet, University of Copenhagen, Copenhagen, Denmark
Background: As measured by near infrared spectroscopy (NIRS), cerebral oxygenation (ScO2) may be reduced by hyperventilation in the anhepatic phase of liver transplantation surgery (LTx). Conversely, the brain may be subjected to hyperperfusion during reperfusion of the grafted liver. We investigated the relationship between ScO2 and end-tidal CO2 tension (EtCO2) during the various phases of LTx.
Methods: In this retrospective study, 49 patients undergoing LTx were studied. Forehead ScO2, EtCO2, minute ventilation (VE), and hemodynamic variables were recorded from the beginning of surgery through to the anhepatic and reperfusion phases during LTx.
Results: In the anhepatic phase, ScO2 was reduced by 4.3% (95% confidence interval: 2.5–6.0%; P < 0.0001), EtCO2 by 0.3 kPa (0.2–0.4 kPa; P < 0.0001), and VE by 0.4 L/min (0.1–0.7 L/min; P = 0.0018). Conversely, during reperfusion of the donated liver, ScO2 increased by 5.5% (3.8–7.3%), EtCO2 by 0.7 kPa (0.5–0.8 kPa), and VE by 0.6 L/min (0.3–0.9 L/min; all P < 0.0001). Changes in ScO2 were correlated to those in EtCO2 (Pearson r = 0.74; P < 0.0001).
Conclusion: During LTx, changes in ScO2 are closely correlated to those of EtCO2. Thus, this retrospective analysis suggests that attention to maintain a targeted EtCO2 would result in a more stable ScO2 during the operation.
Introduction
Autoregulation ensures that cerebral blood flow (CBF) is sufficient to meet the metabolic requirements of the brain, but may be challenged by a low arterial pressure, hypoxia and/or hypocapnia (Kety and Schmidt, 1948; Lassen, 1959). Maintaining mean arterial pressure (MAP) within the cerebral autoregulatory range during surgery has been suggested to result in improved patient outcome (Ono et al., 2013). An evolving strategy for control of the circulation during surgery is to maintain cerebral oxygenation (ScO2), a real-time surrogate for CBF measured using near infrared spectroscopy (NIRS). ScO2 not only has the ability to identify whether patients demonstrate intact cerebral autoregulation, but also determines its lower limit threshold (Nissen et al., 2009).
Impaired cerebral autoregulation (Larsen et al., 1995), cerebral hyperemia, and increased intracranial pressure (Aggarwal et al., 1994) are all associated with end-stage liver disease and may predispose to either ischemic or hyperemic cerebral injury. Cerebral perfusion and thereby ScO2, is challenged by the hemodynamic events that can occur during liver transplantation (LTx) (Adams et al., 1987; Larsen et al., 1999; Pere et al., 2000; Van Mook et al., 2005; Nissen et al., 2010; Zheng et al., 2012). In the hepatic dissection phase, there is a risk for hemorrhage. In the anhepatic phase inadequate venous return to the heart and a low arterial carbon dioxide tension (PaCO2) can occur. This contrasts with the reperfusion phase where increases in PaCO2 may occur (Pere et al., 2000; Panzera et al., 2006). With clamping of the inferior vena cava (IVC), cardiac output (CO) is reduced by as much as 50%, and this can result in compromised perfusion to vital organs including the brain (Pere et al., 2000). Thus, to facilitate hemodynamic stability and to optimize organ perfusion, veno-venous bypass may be utilized (Shaw et al., 1985). Alternatively, venous return to the heart may be assisted by only partially clamping the IVC (so-called piggyback technique) (Panzera et al., 2006). However, even with the piggyback technique, ScO2 is likely to decrease by about 15% (Panzera et al., 2006) increasing the risk of cerebral ischemia (Al-Rawi and Kirkpatrick, 2006).
In the anhepatic phase of LTx, the systemic metabolic rate is reduced by ~30% and there is therefore a reduced need for minute ventilation (VE) in order to preserve CBF and ScO2. Conversely, with reperfusion of the grafted liver, metabolism is restored and the brain may be subjected to hyperperfusion due to enhanced CO2 and/or liberation of vasodilating substances (Ejlersen et al., 1994; Skak et al., 1997) that could lead to brain edema, hemorrhage and even death (Van Mook et al., 2005). ScO2 follows changes in CBF with hyper- and hypo-capnia (Rasmussen et al., 2007) and therefore to maintain ScO2 during the operation potentially minimizes incidence of post-operative neurological complications (Madsen and Secher, 2000; Pere et al., 2000; Zheng et al., 2012).
In this retrospective observational study, we reviewed ScO2, end-tidal CO2 tension (EtCO2), and VE for LTx patients and hypothesized that ScO2 would decrease in the anhepatic phase of the operation and increase again with reperfusion of the grafted liver. We considered that the data would provide an indication as to what extent VE should be adjusted to maintain ScO2 and potentially contribute to brain protection during LTx.
Materials and Methods
Data were collected retrospectively for patients undergoing LTx at Rigshospitalet (Copenhagen) from 1997 to 2001. The study was performed in accordance with guidelines provided by The National Committee on Health Research and approved by the Local Ethical Committee (H-2-2014-FSP27) who waived the need for patient consent.
The liver transplantation technique involved clamping of the IVC with lower body venous return supported by a veno-venous bypass from the left femoral vein to one or two arm veins (Rasmussen et al., 1994). Reperfusion of the grafted liver was established by opening the IVC above the hepatic vein, followed by the IVC below the hepatic vein, and lastly the hepatic artery. Reported hemodynamic variables include heart rate (HR) and femoral MAP measured via an arterial catheter (Becton Dickinson and Company, New Jersey, NY, USA) cardiac output (CO) by thermodilution (7.5F; Baxter, Uden, Holland), thoracic electrical impedance index (THI) (n = 30) (TI; Caspersen and Nielsen, Copenhagen, Denmark) as an indication of the central blood volume (Cai et al., 2000), and ScO2 (Invos 3100 Cerebral Oximeter, Somanetics, Troy, MI, USA) along with VE and EtCO2. PaCO2 was not continuously monitored, however, it was assumed that EtCO2 reflects changes in PaCO2 as expressed by the ratio between CO2 and the alveolar ventilation. All values were noted every 10 min as recorded in the anesthetic chart. Hematocrit was monitored (ABL 700 Radiometer, Copenhagen) and any administration of packed red blood cells and plasma was performed through a rapid infusion system (Haemonetics, Braintree, MA, USA) to maintain a hematocrit of 30%.
Data from the last 60 min of the dissection phase, first and last 30 min of the anhepatic phase, and the first 40 min of the reperfusion phase of the operation were included in the analysis. Hemodynamic changes from dissection to early anhepatic phase were calculated as the difference between an average over 60 min in the dissection phase and 30 min in the early anhepatic phase. Changes from late anhepatic to reperfusion phase were identified as the difference in average from the last 30 min of the anhepatic phase, and the first 20 min of the reperfusion phase.
Distribution of data including variance and probability plots were assessed independently for each patient and the whole population using Proc Univariate in SAS 9.2 (SAS Institute, Cary NC, USA). All variables exhibited normal distribution, however, CO and THI were skewed to the right. Thus, we performed a logarithmic transformation (log10) on CO and THI-data and relative changes are reported as log(x) − log(y) = log(x/y) (Bland and Altman, 1996a). In Figure 1, CO and THI are presented as geometric means ±95% confidence interval (Bland and Altman, 1996b). We applied an analysis of variance followed by a Tukey–Kramer post-hoc test to evaluate changes between conditions and a P-value < 0.05 was considered as statistically significant. Association between ScO2, VE, and EtCO2 was evaluated by Pearson's correlation. Since ScO2 has been reported to decrease with increasing plasma bilirubin (Madsen et al., 2000; Song et al., 2011) that relation was also evaluated with Spearman rank order correlation.
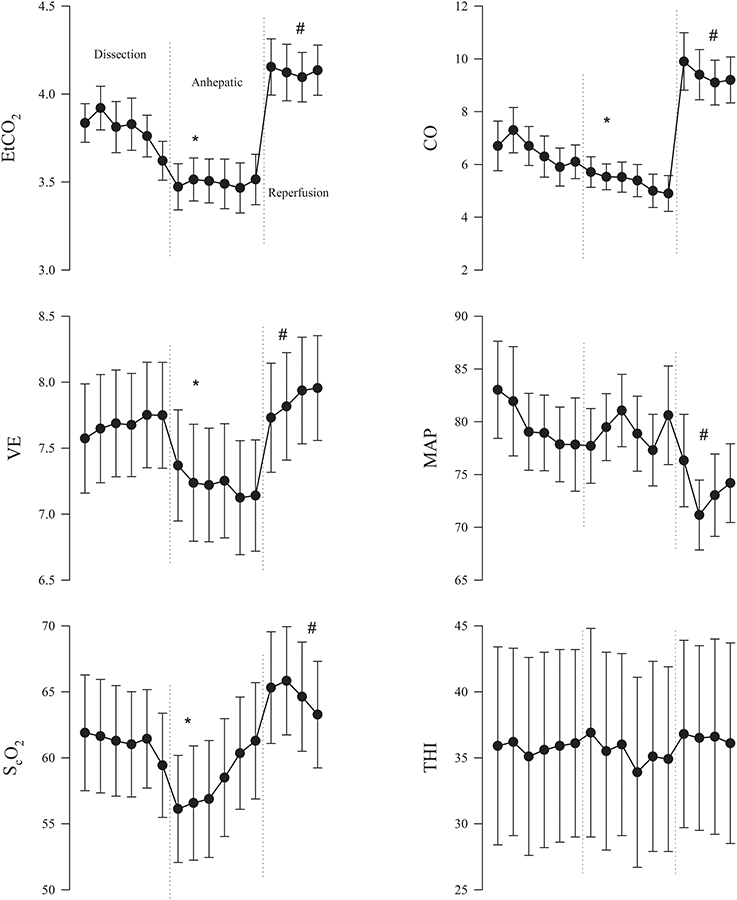
Figure 1. Mean (±95% confidence interval) for 10th min in the dissection, anhepatic and reperfusion phases of liver transplantation surgery for end-tidal CO2 tension (EtCO2); ventilation (VE); near infrared spectroscopy-determined frontal lobe oxygenation (ScO2); cardiac output (CO); mean arterial pressure (MAP); and thoracic electrical impedance index (THI). CO and THI reported as geometric means. *P < 0.05 compared to the dissection phase of the operation. #P < 0.05 compared to the anhepatic phase.
Results
Forty nine patients, [21 women, 28 men, 53±10 (mean ± SD) years] were admitted for LTx. Twenty six patients had cirrhosis, 5 primary biliary cirrhosis, 4 primary sclerosing cholangitis, 3 acute liver failure, 3 hepatocellular carcinoma, and the remaining 8 patients had other liver diseases. The duration of surgery was 368 min (range; 240–675), representing 141 min (60–465) for the dissection phase of the operation, 83 min (50–250) for the anhepatic phase, and 145 min (70–230) for completion of the operation.
Anhepatic Phase
From the initial dissection to the anhepatic phase of the operation, ScO2 and EtCO2 decreased by 4.3% [(95% confidence intervals: 2.5–6.0%) and by 0.3 kPa (0.2–0.4 kPa; both P < 0.0001)] as VE was reduced by 0.4 L/min (0.1–0.7 L/min; P = 0.0018). HR, MAP, and THI remained stable (Figure 1). CO was reduced by 15% (6–24%; P = 0.0003).
Changes in ScO2 was correlated to those in EtCO2 (Pearson r = 0.74; P < 0.0001), however, no correlation between ScO2 and VE was observed (Pearson r = 0.06; P = 0.7) (Figure 2). In 11 patients, ScO2 was reduced by more than 15%. We observed an inverse relationship between ScO2 with plasma bilirubin (Spearman r = −0.49; P = 0.008) ranging from 9 to 565 μmol/L (n = 28).
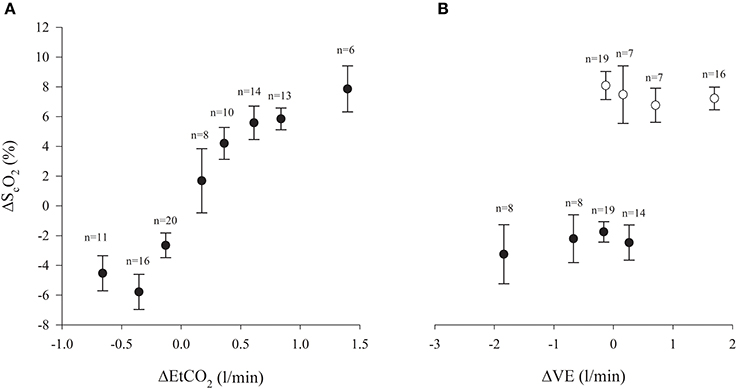
Figure 2. (A) Frontal lobe oxygenation (ScO2) and end-tidal CO2 tension (EtCO2) in the anhepatic and reperfusion phases of liver transplantation surgery (% changes from baseline; ± s.e.m.) (Pearson r = 0.74; P < 0.0001). Number of subjects indicated. (B) Changes from baseline (± s.e.m.) for ScO2 and ventilation (VE). Black symbols: anhepatic phase (Pearson r = 0.06; P = 0.7). Open symbols: reperfusion phase (Pearson r = −0.18; P = 0.21). Number of subjects is indicated.
Reperfusion Phase
During reperfusion of the grafted liver, ScO2 and EtCO2 increased 5.5% (3.8–7.3%) and 0.7 kPa (0.5–0.8 kPa; P < 0.0001) as VE was increased by 0.6 L/min (−0.5–3.1 L/min; all P < 0.0001) (Figure 1). No changes were observed in HR and THI, but CO increased by 90% (71–110%; P < 0.0001). Conversely, MAP decreased by 5 mmHg (1–9 mmHg; P = 0.007). No significant correlation between ScO2 and VE was identify (Pearson r = −0.18; P = 0.21) (Figure 2), but 13 patients ScO2 increased >15% compared to the late anhepatic phase.
Discussion
In this retrospective study of measurements during LTx in 49 patients, cerebral oxygenation (ScO2), as determined by NIRS, was shown to decrease in the anhepatic phase of the operation and to increase during reperfusion of the grafted liver. Changes in ScO2 were directly related to the end-tidal CO2 tension. Therefore, a ventilatory strategy directed to a maintain EtCO2 could ensure stability of ScO2 during the operation and may, at least potentially, minimize the incidence of post-operative seizures, confusion, and stroke (Madsen and Secher, 2000; Pere et al., 2000; Zheng et al., 2012). Despite bilirubin absorption of infrared light resulting in a low ScO2, NIRS detected changes in cerebral oxygenation even in patients who were significantly jaundiced (Madsen et al., 2000).
Patients with liver disease are susceptible to alterations in MAP that can frequently result in pressure below the limits of cerebral autoregulation and then may lead to cerebral ischemia. Cerebral oxygenation might further be aggravated by increases in intracranial pressure that reduce cerebral perfusion pressure according to the Monro–Kellie doctrine (Larsen and Wendon, 2008). Thus, it seems to be an advantage if handling of the circulation during LTx involves continuous monitoring of the brain circulation to reduce adverse neurological outcome. NIRS represents a real-time, though indirect, monitor of CBF and indicates its autoregulatory capacity (Nissen et al., 2009; Zheng et al., 2012). In this cohort of LTx patients, ScO2 was reduced by 4.3% (2.5–6.0%) with IVC clamping (Figure 1), which is likely induced by hyperventilation as indicated by a reduction in EtCO2 by 0.3 kPa, albeit VE was diminished by 0.4 l/min. Thus, with the central blood volume maintained as indicated by THI (Cai et al., 2000), a ventilatory strategy guided by EtCO2 may avoid cerebral ischemia in the anhepatic phase (Pott et al., 1995), e.g., by keeping EtCO2 between 4.7 and 6.0 kPa, arterial CO2, CBF, and ScO2 were maintained (Pott et al., 1995; Zheng et al., 2012). In contrast, no ventilatory adjustment in the anhepathic phase of the operation has been reported to lead to pronounced reductions in PaCO2, and yet maintained CBF as indicated by transcranial Doppler (Pere et al., 2000). In that study (Pere et al., 2000), cardiac preload was not supported by a venous-venous bypass, while we registered an 15% reduction in CO when the shunt was established. Although we cannot rule out that this reduction in CO may affect CBF and ScO2, we find it more likely that changes in ScO2 relate to alterations in EtCO2 than to the reduction in CO with the hierarchy of blood flow in the anhepatic phase (Figure 2) (Rhee et al., 2012; Ono et al., 2013; Mahal et al., 2014). In 22% of the patients, ScO2 was reduced by >15% (relative to the value in the dissection phase) thus lowering the threshold for cerebral ischemia (Al-Rawi and Kirkpatrick, 2006). Similar significant cerebral deoxygenation is reported in up to 50% of patients undergoing LTx (Plachky et al., 2004), and also seen with the use of the piggy-back technique (Panzera et al., 2006).
Postoperative biomarkers of brain damage include neuron-specific enolase and S-100β and they may increase three-fold in patients who demonstrate cerebral deoxygenation (Plachky et al., 2004). S-100β levels are high in patients who develope post-operative cognitive dysfunction (POCD) (Linstedt et al., 2002) and cerebral deoxygenation (>15% relative to baseline) is related to POCD and longer hospital stay (Casati et al., 2005; Ballard et al., 2012; Colak et al., 2014). Moreover, inherent to prolonged cerebral deoxygenation, confusion, somnolence and transient hemiparesis manifest post-operatively (Madsen and Secher, 2000) or permanent neurological damage develops (Philips et al., 1998). Also in patients with acute liver failure, cerebral infarction after LTx can led to long-term hospital care, however, perioperative cerebral oxygenation was not reported for that patient (Pere et al., 2000). In general, patients with encephalopathy have been reported with a 15% higher ScO2 (Panzera et al., 2006), may be as a result of cerebral hyperemia because of lack of cerebral autoregulation (Ejlersen et al., 1994). However, similar reductions of ~30% relative to the pre-operative ScO2 were seen with IVC clamping in patients with and without encephalopathy (Panzera et al., 2006).
When the transplanted liver is reperfused, the brain can be subjected to hyperemia due to enhanced CO2 reactivity and/or liberation of vasodilating substances (Ejlersen et al., 1994) as we demonstrated by the 0.7 kPa increase in EtCO2 and if untreated can have adverse effects and affect even mortality (Skak et al., 1997). With impaired cerebral autoregulation, the risk of hyperperfusion is even larger due to missing cerebral vasoconstriction in response a 90% increase in CO and be aggravated by the vasodilatory effect of CO2 (Figure 1). Accordingly, ScO2 may guide to what extent VE should be increased in order to protect the brain. We observed an increase in ScO2 by 5.5% (3.8–7.3%) during reperfusion although VE was increased by 0.6 l/min. We, therefore, suggest a more meticulous control of VE is in need, as guided by EtCO2, until the end of LTx (Nissen et al., 2010). Although EtCO2 was kept within 4.6–6.0 kPa (Pott et al., 1995; Zheng et al., 2012) or VE increased by 15% (Pere et al., 2000), CBF becomes elevated (by more than 80% in some patients) with reperfusion of the liver, which emphasizes that attempts to maintain EtCO2 toward the end of the operation could attenuate cerebral hyperperfusion (Pott et al., 1995; Philips et al., 1998; Zheng et al., 2012).
The PaCO2 relates to hydrogen ion concentration and is a potent modulator of cerebrovascular resistance and, thus, CBF (Lassen, 1959). Hypercapnia leads to cerebral vasodilation while the opposite occurs with hypocapnia through a serial of endogenous mediators (Eriksson et al., 1983). In healthy humans, CBF increases 2–8% per mmHg CO2 as determined by Fick's principle (Kety and Schmidt, 1946) or transcranial Doppler (Madsen and Secher, 1999), however, CO2-reactivity has not yet been describe for NIRS despite ScO2 does follow CBF induced by hypercapnia and hypocapnia (Rasmussen et al., 2007). As evaluated by 133Xenon clearance in patients undergoing LTx, CBF increases by 25% and may be more than can be explained by the increase in PaCO2 (Larsen et al., 1999). Increasing PaCO2 may mitigate the CO2-reactivity because of near-maximal cerebral vasodilatation or may be attributable to other vasodilating substances interfering with the effect of CO2 on the cerebral vasculature (Philips et al., 1998).
As this was a retrospective study, we did not evaluate neurological outcome. In related studies, neurological complications range from mild seizures to hemorrhage and stroke after LTx (Adams et al., 1987; Stein et al., 1992; Madsen and Secher, 2000; Pere et al., 2000; Zheng et al., 2012) and cerebral hemorrhage and anoxic-ischemic lesions are common at brain autopsy after LTx (Ferreiro et al., 1992). However, the evidence for improved neurological outcome by maintaining ScO2 during LTx remains sparse, although improved outcome is seen in cardiac (Slater et al., 2009; Ono et al., 2013; Colak et al., 2014; Harilall et al., 2014), abdominal (Casati et al., 2005), and orthopedic surgery (Ballard et al., 2012). An observational cohort study is underway investigating the relationship between perioperative desaturation during hepatic surgery or LTx and adverse postoperative events and length of ICU stay, but optimization of ScO2 in the anhepatic and reperfusion phase is not included (clinicaltrials.gov: NCT01458262). Although the adequacy of cerebral autoregulation and oxygenation can be monitored in the operating room, impaired CBF regulation may persist into the early postoperative phase (Larsen et al., 1999), but no study describes the efficacy of maintaining cerebral monitoring in the ICU after LTx (Ejlersen et al., 1994; Van Mook et al., 2005).
From this retrospective study, we conclude that despite adjustments of VE in the anhepatic and reperfusion phases of LTx, ScO2 changes occur that have the potential to expose patients to cerebral ischemia and/or hyperemia. We suggest that a ventilatory strategy guided by EtCO2 would keep ScO2 more stable during LTx.
Author Contributions
All authors contributed equally to the design, data analysis and interpretation, drafting the manuscript and critical revision. All authors approved the final version before submission.
Conflict of Interest Statement
The authors declare that the research was conducted in the absence of any commercial or financial relationships that could be construed as a potential conflict of interest.
Acknowledgments
Assistance with the article: We thank Carol A. Cooke, MLIS (Neil John Maclean Health Sciences Library) for developing the literature search strategies and John McVagh, Study Coordinator, Department of Anesthesia, University of Manitoba, for invaluable help and encouragement. Financial support and sponsorship: The author (Henrik Sørensen) received a grant from Dansk Medicinsk Selskab i København to visit Department of Anesthesia & Perioperative Medicine, St. Boniface Hospital, University of Manitoba, Winnipeg, Canada.
References
Adams, D. H., Ponsford, S., Gunson, B., Boon, A., Honigsberger, L., Williams, A., et al. (1987). Neurological complications following liver transplantation. Lancet 1, 949–951. doi: 10.1016/S0140-6736(87)90294-7
Aggarwal, S., Kramer, D., Yonas, H., Obrist, W., Kang, Y., Martin, M., et al. (1994). Cerebral hemodynamic and metabolic changes in fulminant hepatic failure: a retrospective study. Hepatology 19, 80–87. doi: 10.1002/hep.1840190114
Al-Rawi, P. G., and Kirkpatrick, P. J. (2006). Tissue oxygen index: thresholds for cerebral ischemia using near-infrared spectroscopy. Stroke 37, 2720–2725. doi: 10.1161/01.STR.0000244807.99073.ae
Ballard, C., Jones, E., Gauge, N., Aarsland, D., Nilsen, O. B., Saxby, B. K., et al. (2012). Optimised anaesthesia to reduce post operative cognitive decline (POCD) in older patients undergoing elective surgery, a randomised controlled trial. PLoS ONE 7:e37410. doi: 10.1371/journal.pone.0037410
Bland, J. M., and Altman, D. G. (1996a). Statistics notes. Logarithms. BMJ 312, 700. doi: 10.1136/bmj.312.7032.700
Bland, J. M., and Altman, D. G. (1996b). Transformations, means, and confidence intervals. BMJ 312, 1079. doi: 10.1136/bmj.312.7038.1079
Cai, Y., Holm, S., Jenstrup, M., Stromstad, M., Eigtved, A., Warberg, J., et al. (2000). Electrical admittance for filling of the heart during lower body negative pressure in humans. J. Appl. Physiol. (1985) 89, 1569–1576.
Casati, A., Fanelli, G., Pietropaoli, P., Proietti, R., Tufano, R., Danelli, G., et al. (2005). Continuous monitoring of cerebral oxygen saturation in elderly patients undergoing major abdominal surgery minimizes brain exposure to potential hypoxia. Anesth. Analg. 101, 740–747. doi: 10.1213/01.ane.0000166974.96219.cd
Colak, Z., Borojevic, M., Bogovic, A., Ivancan, V., Biocina, B., and Majeric-Kogler, V. (2014). Influence of intraoperative cerebral oximetry monitoring on neurocognitive function after coronary artery bypass surgery: a randomized, prospective study. Eur. J. Cardiothorac. Surg. doi: 10.1093/ejcts/ezu193. [Epub ahead of print].
Ejlersen, E., Larsen, F. S., Pott, F., Gyrtrup, H. J., Kirkegaard, P., and Secher, N. H. (1994). Hepatectomy corrects cerebral hyperperfusion in fulminant hepatic failure. Transplant. Proc. 26, 1794–1795.
Eriksson, S., Hagenfeldt, L., Law, D., Patrono, C., Pinca, E., and Wennmalm, A. (1983). Effect of prostaglandin synthesis inhibitors on basal and carbon dioxide stimulated cerebral blood flow in man. Acta Physiol. Scand. 117, 203–211. doi: 10.1111/j.1748-1716.1983.tb07198.x
Ferreiro, J. A., Robert, M. A., Townsend, J., and Vinters, H. V. (1992). Neuropathologic findings after liver transplantation. Acta Neuropathol. 84, 1–14. doi: 10.1007/BF00427209
Harilall, Y., Adam, J. K., Biccard, B. M., and Reddi, A. (2014). The effect of optimising cerebral tissue oxygen saturation on markers of neurological injury during coronary artery bypass graft surgery. Heart Lung Circ. 23, 68–74. doi: 10.1016/j.hlc.2013.07.002
Kety, S. S., and Schmidt, C. F. (1946). The effects of active and passive hyperventilation on cerebral blood flow, cerebral oxygen consumption, cardiac output, and blood pressure of normal young men. J. Clin. Invest. 25, 107–119. doi: 10.1172/JCI101680
Kety, S. S., and Schmidt, C. F. (1948). The effects of altered arterial tensions of carbon dioxide and oxygen on cerebral blood floow and cerebral oxygen consumption of normal young men. J. Clin. Invest. 27, 484–492. doi: 10.1172/JCI101995
Larsen, F. S., Ejlersen, E., Hansen, B. A., Knudsen, G. M., Tygstrup, N., and Secher, N. H. (1995). Functional loss of cerebral blood flow autoregulation in patients with fulminant hepatic failure. J. Hepatol. 23, 212–217. doi: 10.1016/0168-8278(95)80338-6
Larsen, F. S., Ejlersen, E., Strauss, G., Rasmussen, A., Kirkegaard, P., Hansen, B. A., et al. (1999). Cerebrovascular metabolic autoregulation is impaired during liver transplantation. Transplantation 68, 1472–1476. doi: 10.1097/00007890-199911270-00007
Larsen, F. S., and Wendon, J. (2008). Prevention and management of brain edema in patients with acute liver failure. Liver Transpl. 14(Suppl. 2), S90–S96. doi: 10.1002/lt.21643
Linstedt, U., Meyer, O., Kropp, P., Berkau, A., Tapp, E., and Zenz, M. (2002). Serum concentration of S-100 protein in assessment of cognitive dysfunction after general anesthesia in different types of surgery. Acta Anaesthesiol. Scand. 46, 384–389. doi: 10.1034/j.1399-6576.2002.460409.x
Madsen, P. L., and Secher, N. H. (1999). Near-infrared oximetry of the brain. Prog. Neurobiol. 58, 541–560. doi: 10.1016/S0301-0082(98)00093-8
Madsen, P. L., and Secher, N. H. (2000). Postoperative confusion preceded by decreased frontal lobe haemoglobin oxygen saturation. Anaesth. Intensive Care 28, 308–310.
Madsen, P. L., Skak, C., Rasmussen, A., and Secher, N. H. (2000). Interference of cerebral near-infrared oximetry in patients with icterus. Anesth. Analg. 90, 489–493. doi: 10.1213/00000539-200002000-00046
Mahal, I., Davie, S. N., and Grocott, H. P. (2014). Cerebral oximetry and thoracic surgery. Curr. Opin. Anaesthesiol. 27, 21–27. doi: 10.1097/ACO.0000000000000027
Nissen, P., Frederiksen, H. J., and Secher, N. H. (2010). Intraoperative hemodynamic monitoring during liver transplantation: goals and devices. Minerva Gastroenterol. Dietol. 56, 261–277.
Nissen, P., Pacino, H., Frederiksen, H. J., Novovic, S., and Secher, N. H. (2009). Near-infrared spectroscopy for evaluation of cerebral autoregulation during orthotopic liver transplantation. Neurocrit. Care 11, 235–241. doi: 10.1007/s12028-009-9226-8
Ono, M., Arnaoutakis, G. J., Fine, D. M., Brady, K., Easley, R. B., Zheng, Y., et al. (2013). Blood pressure excursions below the cerebral autoregulation threshold during cardiac surgery are associated with acute kidney injury. Crit. Care Med. 41, 464–471. doi: 10.1097/CCM.0b013e31826ab3a1
Panzera, P., Greco, L., Carravetta, G., Gentile, A., Catalano, G., Cicco, G., et al. (2006). Alteration of brain oxygenation during “piggy back” liver transplantation. Adv. Exp. Med. Biol. 578, 269–275. doi: 10.1007/0-387-29540-2_43
Pere, P., Hockerstedt, K., Isoniemi, H., and Lindgren, L. (2000). Cerebral blood flow and oxygenation in liver transplantation for acute or chronic hepatic disease without venovenous bypass. Liver Transpl. 6, 471–479. doi: 10.1053/jlts.2000.8186
Philips, B. J., Armstrong, I. R., Pollock, A., and Lee, A. (1998). Cerebral blood flow and metabolism in patients with chronic liver disease undergoing orthotopic liver transplantation. Hepatology 27, 369–376. doi: 10.1002/hep.510270209
Plachky, J., Hofer, S., Volkmann, M., Martin, E., Bardenheuer, H. J., and Weigand, M. A. (2004). Regional cerebral oxygen saturation is a sensitive marker of cerebral hypoperfusion during orthotopic liver transplantation. Anesth. Analg. 99, 344–349, doi: 10.1213/01.ANE.0000124032.31843.61
Pott, F., Larsen, F. S., Ejlersen, E., Linkis, P., Jorgensen, L. G., and Secher, N. H. (1995). Cerebral perfusion during human liver transplantation. Clin. Physiol. 15, 119–130. doi: 10.1111/j.1475-097X.1995.tb00436.x
Rasmussen, L. S., Ejlersen, E., Hjortrup, A., Kirkegaard, P., and Secher, N. H. (1994). Veno-venous bypass during human liver transplantation. Transplant. Proc. 26, 1791.
Rasmussen, P., Dawson, E. A., Nybo, L., Van Lieshout, J. J., Secher, N. H., and Gjedde, A. (2007). Capillary-oxygenation-level-dependent near-infrared spectrometry in frontal lobe of humans. J. Cereb. Blood Flow Metab. 27, 1082–1093. doi: 10.1038/sj.jcbfm.9600416
Rhee, C. J., Kibler, K. K., Easley, R. B., Andropoulos, D. B., Czosnyka, M., Smielewski, P., et al. (2012). Renovascular reactivity measured by near-infrared spectroscopy. J. Appl. Physiol. 113, 307–314. doi: 10.1152/japplphysiol.00024.2012
Shaw, B. W. Jr., Martin, D. J., Marquez, J. M., Kang, Y. G., Bugbee, A. C. Jr., Iwatsuki, S., et al. (1985). Advantages of venous bypass during orthotopic transplantation of the liver. Semin. Liver Dis. 5, 344–348. doi: 10.1055/s-2008-1040631
Skak, C., Rasmussen, A., Kirkegaard, P., and Secher, N. H. (1997). Cerebral oxygen saturation and blood flow during liver transplantation. Anesth. Analg. 84, 730–733.
Slater, J. P., Guarino, T., Stack, J., Vinod, K., Bustami, R. T., Brown, J. M. 3rd., et al. (2009). Cerebral oxygen desaturation predicts cognitive decline and longer hospital stay after cardiac surgery. Ann. Thorac. Surg. 87, 36–44. discussion: 44–45. doi: 10.1016/j.athoracsur.2008.08.070
Song, J. G., Jeong, S. M., Shin, W. J., Jun, I. G., Shin, K., Huh, I. Y., et al. (2011). Laboratory variables associated with low near-infrared cerebral oxygen saturation in icteric patients before liver transplantation surgery. Anesth. Analg. 112, 1347–1352. doi: 10.1213/ANE.0b013e318214b2b0
Stein, D. P., Lederman, R. J., Vogt, D. P., Carey, W. D., and Broughan, T. A. (1992). Neurological complications following liver transplantation. Ann. Neurol. 31, 644–649. doi: 10.1002/ana.410310612
Van Mook, W. N., Rennenberg, R. J., Schurink, G. W., Van Oostenbrugge, R. J., Mess, W. H., Hofman, P. A., et al. (2005). Cerebral hyperperfusion syndrome. Lancet Neurol. 4, 877–888. doi: 10.1016/S1474-4422(05)70251-9
Keywords: cerebral oxygenation, cerebral oximetry, end-tidal carbon dioxide, liver transplantation, monitoring, ventilation
Citation: Sørensen H, Grocott HP, Niemann M, Rasmussen A, Hillingsø JG, Frederiksen HJ and Secher NH (2014) Ventilatory strategy during liver transplantation: implications for near-infrared spectroscopy-determined frontal lobe oxygenation. Front. Physiol. 5:321. doi: 10.3389/fphys.2014.00321
Received: 31 May 2014; Accepted: 04 August 2014;
Published online: 25 August 2014.
Edited by:
Patrice Brassard, Laval University, CanadaReviewed by:
Stephane Perrey, Montpellier I University, FrancePhil Neil Ainslie, University of British Columbia, Canada
Copyright © 2014 Sørensen, Grocott, Niemann, Rasmussen, Hillingsø, Frederiksen and Secher. This is an open-access article distributed under the terms of the Creative Commons Attribution License (CC BY). The use, distribution or reproduction in other forums is permitted, provided the original author(s) or licensor are credited and that the original publication in this journal is cited, in accordance with accepted academic practice. No use, distribution or reproduction is permitted which does not comply with these terms.
*Correspondence: Henrik Sørensen, Department of Anesthesia, Rigshospitalet 2041, Blegdamsvej 9, DK-2100 Copenhagen, Denmark e-mail: hs770@hotmail.com