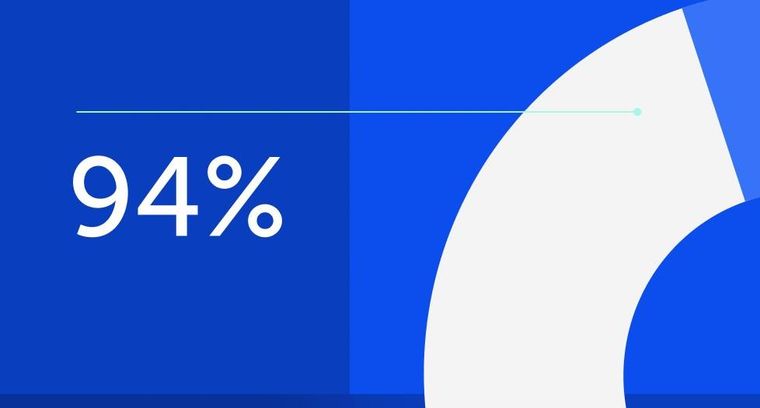
94% of researchers rate our articles as excellent or good
Learn more about the work of our research integrity team to safeguard the quality of each article we publish.
Find out more
REVIEW article
Front. Physiol., 24 June 2014
Sec. Clinical and Translational Physiology
Volume 5 - 2014 | https://doi.org/10.3389/fphys.2014.00227
Hypertension is a major risk factor for stroke, coronary events, heart and renal failure, and the renin-angiotensin system (RAS) plays a major role in its pathogenesis. Within the RAS, angiotensin converting enzyme (ACE) converts angiotensin (Ang) I into the vasoconstrictor Ang II. An “alternate” arm of the RAS now exists in which ACE2 counterbalances the effects of the classic RAS through degradation of Ang II, and generation of the vasodilator Ang 1-7. ACE2 is highly expressed in the heart, blood vessels, and kidney. The catalytically active ectodomain of ACE2 undergoes shedding, resulting in ACE2 in the circulation. The ACE2 gene maps to a quantitative trait locus on the X chromosome in three strains of genetically hypertensive rats, suggesting that ACE2 may be a candidate gene for hypertension. It is hypothesized that disruption of tissue ACE/ACE2 balance results in changes in blood pressure, with increased ACE2 expression protecting against increased blood pressure, and ACE2 deficiency contributing to hypertension. Experimental hypertension studies have measured ACE2 in either the heart or kidney and/or plasma, and have reported that deletion or inhibition of ACE2 leads to hypertension, whilst enhancing ACE2 protects against the development of hypertension, hence increasing ACE2 may be a therapeutic option for the management of high blood pressure in man. There have been relatively few studies of ACE2, either at the gene or the circulating level in patients with hypertension. Plasma ACE2 activity is low in healthy subjects, but elevated in patients with cardiovascular risk factors or cardiovascular disease. Genetic studies have investigated ACE2 gene polymorphisms with either hypertension or blood pressure, and have produced largely inconsistent findings. This review discusses the evidence regarding ACE2 in experimental hypertension models and the association between circulating ACE2 activity and ACE2 polymorphisms with blood pressure and arterial hypertension in man.
Cardiovascular disease (CVD) is the leading cause of death and disability worldwide (World Health Organization, 2011), and hypertension is the most common risk factor for CVD (Kearney et al., 2005). The renin angiotensin system (RAS) plays a major role in the pathogenesis of hypertension, which is a risk factor for coronary events, stroke, kidney failure, and heart failure (Johnston, 1994; Dzau, 2005). Within the RAS, angiotensin converting enzyme (ACE) converts angiotensin (Ang) I into the vasoconstrictor Ang II, which mediates its effects via the angiotensin type 1 receptor (AT1R). Ang II has actions to raise blood pressure through vasoconstriction and salt and water retention (Figure 1). In experimental models, stimulation of angiotensin II type 2 receptor (AT2R) may counteract AT1R mediated effects either directly or by modulating AT1R signaling (Lemarie and Schiffrin, 2010; McCarthy et al., 2013) In man, therapeutic strategies that block RAS activation using ACE inhibitors or Ang II receptor blockers are first line therapy in hypertension and have beneficial effects on morbidity and mortality.
Figure 1. Opposing arms of the renin angiotensin system. Angiotensin converting enzyme (ACE) converts angiotensin (Ang) I to Ang II, which exerts its deleterious effects via the angiotensin type 1 receptor (AT1R). Ang II can also act on the angiotensin type 2 receptor (AT2R) which can counteract AT1R mediated effects. In the opposing arm, ACE2 degrades Ang II to Ang 1-7, which is thought to exert beneficial effect via the mas receptor.
The discovery of angiotensin converting enzyme 2 (ACE2) (Donoghue et al., 2000; Tipnis et al., 2000) more than a decade ago has led to the concept of an “alternate” arm of the RAS. ACE2 has now been identified in many tissues including blood vessels (Zulli et al., 2006, 2008; Sluimer et al., 2008), heart (Donoghue et al., 2000; Burrell et al., 2005; Ohtsuki et al., 2010), kidney (Tikellis et al., 2003; Mizuiri et al., 2008; Reich et al., 2008), liver (Paizis et al., 2005), and brain (Doobay et al., 2007). The ACE2 amino acid sequence shares approximately 40% homology with the N-terminal catalytic domain of ACE, and a hydrophobic region near the C-terminus likely to serve as a membrane anchor. Like ACE, ACE2 is a type 1 membrane protein with the catalytic domain on the extracellular surface (Donoghue et al., 2000; Tipnis et al., 2000) and the ACE2 protein is encoded by the ACE2 gene located on chromosome Xp22.
ACE2 is a monocarboxypeptidase, and in vitro can catalyze cleavage of the C-terminal residue of the peptides Ang I, des-Arg-bradykinin, neurotensin 1–13, and kinetensin (Donoghue et al., 2000), as well as catalyzing the hydrolysis of the C-terminal residue of Ang II (Tipnis et al., 2000). ACE2 can hydrolyze apelin-13 and dynorphin A 1–13 with high activity (Vickers et al., 2002), but its most important biological effect is to degrade Ang II to Ang 1-7, which it does with a catalytic efficiency that is 400-fold higher with Ang II as a substrate than with Ang I (Figure 1). Thus, ACE2 may limit the vasoconstrictor action of Ang II through its degradation, as well as counteracting the actions of Ang II through the formation of Ang 1-7 which is reported to have vasodilatory and anti-fibrotic actions (Tallant and Clark, 2003) at the Ang 1-7 or mas receptor (Santos et al., 2003). Ang 1-7 has other actions of cardiovascular relevance including natriuresis, diuresis, inhibition of cell growth and anti-atherosclerotic effects; the reader is referred to a recent review for a detailed description of the actions of Ang 1-7 (Santos, 2014).
This review will discuss the evidence regarding ACE2 in experimental models of primary and secondary arterial hypertension, the association between circulating ACE2 activity and blood pressure and hypertension in man, as well as genetic association studies that have investigated ACE2 polymorphisms with blood pressure and/or arterial hypertension.
It is hypothesized that disruption of tissue ACE/ACE2 balance may result in changes in blood pressure, with increased ACE2 expression protecting against increased blood pressure, and ACE2 deficiency leading to increased blood pressure (Yagil and Yagil, 2003). Table 1 summarizes the experimental studies that have measured ACE2 gene, protein and/or activity, in either the heart, kidney and/or plasma, usually at one time point, and generally in animals with established hypertension, and compared results to a “normotensive” control.
There are several lines of evidence that support a role for ACE2 in the development of primary experimental hypertension. Firstly, the gene for ACE2 maps to a defined quantitative trait locus on the X chromosome, previously identified as a quantitative locus for blood pressure (Crackower et al., 2002), in three strains of genetically hypertensive rats the Sabra salt-sensitive rat, spontaneously hypertensive rat (SHR) and the stroke-prone SHR. ACE2 may play a role in the pathogenesis of genetic hypertension. In a study that examined ACE2 expression in the kidneys of SHR and normotensive Wistar-Kyoto (WKY) rats at birth, 6 weeks and adulthood (Tikellis et al., 2006), kidney ACE2 expression fell with the onset of hypertension in SHR compared to WKY, and remained reduced in the adult SHR kidney (Tikellis et al., 2006). Similarly, although cardiac ACE2 activity was not significantly different between 10 days old SHR and WKY rats, by 3 months cardiac ACE2 activity was reduced in SHR compared to age-matched WKY rats (Diez-Freire et al., 2006).
In adult rats with established hypertension, there was an association between reduced kidney ACE2 and blood pressure in SHR and in stroke-prone SHR, compared to levels in normotensive rats (Crackower et al., 2002). Reduced cardiac ACE2 expression (Zhong et al., 2004; Ferreira et al., 2011; Tan et al., 2011; Yang et al., 2013) and activity (Diez-Freire et al., 2006) has been reported in SHR compared to WKY rats, in Dahl salt-sensitive rats compared to salt-resistant rats (Takeda et al., 2007), and in salt-loaded SHR compared to SHR fed a low salt diet (Varagic et al., 2010). A subsequent study showed no change in cardiac ACE2 gene and activity in salt-loaded SHR (Varagic et al., 2012), and similarly in Sabra salt-sensitive rats, salt loading did not change cardiac ACE2 protein compared to salt-resistant Sabra rats on a normal diet (Landau et al., 2008).
The results with regard to regulation of tissue ACE2 in transgenic models of hypertension are mixed. Transgenic overexpression of renin in Sprague Dawley (SD) rats did not change kidney ACE2 expression and activity (Kamilic et al., 2010), but in transgenic Lewis rats, kidney cortical ACE2 was reduced with no change in cardiac ACE2 (Pendergrass et al., 2008). Similarly, in transgenic mice overexpressing human renin and angiotensinogen, no change in ACE2 activity was observed in the kidney, however this study reported elevated ACE2 activity in the brain of transgenic mice compared to non-transgenic mice (Xia et al., 2009). The disparity in observed changes highlights the importance of the strain and model used when examining the role of ACE2 in hypertension.
Although a potentially reversible cause of hypertension can be identified in less than 10% of patients with hypertension, the overall high prevalence of hypertension means that secondary forms can affect millions of patients worldwide. Dysregulation of ACE2 has been reported in experimental models of secondary hypertension, such as endocrine hypertension [deoxycorticosterone (DOCA)-salt, Ang II infusion], renal failure (subtotal nephrectomy), and renal artery stenosis (Goldblatt hypertension).
In DOCA-salt hypertension in the rat, plasma ACE2 activity (Ocaranza et al., 2011) and left ventricular ACE2 gene expression (Santiago et al., 2010) were unchanged compared to uninephrectomised rats with no DOCA or high salt intake. Hypertension secondary to short term Ang II infusion (2 weeks) in SD rats resulted in decreased kidney cortical and medullary ACE2 protein (Prieto et al., 2011), although chronic Ang II infusion (4 weeks) in SD rats increased cardiac ACE2 expression with no change in kidney ACE2 (Meng et al., 2014). In mice, a 2 week Ang II infusion increased plasma ACE2 activity and cardiac ACE2 gene expression (Patel et al., 2013a), but reduced cardiac ACE2 protein and activity (Patel et al., 2013a) and kidney protein (Zhong et al., 2011).
In the Goldblatt (2-kidney, 1-clip) hypertensive SD rat model with kidney impairment, kidney ACE2 gene and protein expression were reduced in both clipped and non-clipped kidneys (Prieto et al., 2011; Bai et al., 2013), while renal ACE was significantly elevated, resulting in increased Ang II and reduced Ang 1-7 levels (Prieto et al., 2011). However, others have shown that kidney ACE2 and Ang 1-7 levels are increased in Goldblatt hypertension in Hannover SD rats without kidney impairment (Burgelova et al., 2009). In a short-term kidney failure model of hypertension induced by subtotal nephrectomy (STNx), we reported organ specific regulation of ACE2 with increased plasma and cardiac ACE2 (Burchill et al., 2008; Velkoska et al., 2011), and decreased kidney ACE2 (Velkoska et al., 2010). These data suggest that during the early stages of kidney impairment, activation of cardiac ACE2 may protect the heart against the adverse effects of an activated RAS, whilst depletion of kidney ACE2 contributes to kidney injury. Furthermore, in a long-term model of chronic kidney failure induced hypertension, we reported a persistent reduction in renal ACE2, but the early elevation in cardiac ACE2 was no longer evident, suggesting that this may contribute to ongoing cardiac dysfunction with renal disease progression (Burrell et al., 2012). In both short-term and chronic models of kidney failure induced hypertension, reduced kidney ACE2 was associated with increased blood pressure (Dilauro et al., 2010; Velkoska et al., 2010; Burrell et al., 2012).
ACE2 has also been identified in regions of the mouse brain involved in control of cardiovascular function (Doobay et al., 2007), with ACE2 gene deletion resulting in impaired baroreflex sensitivity and autonomic function (Xu et al., 2011), thus supporting a potential role of ACE2 in central blood pressure regulation. ACE2 protein expression was reduced in the rostral ventrolateral medulla (RVLM) of SHR compared to WKY rats, and overexpression of ACE2 in the RVLM decreased blood pressure in SHR but not WKY rats (Yamazato et al., 2007). Similarly reduced ACE2 activity in the brain stem was observed in genetically hypertensive mice (Xia et al., 2009), while specific ACE2 overexpression in the brain restored baroreflex function and contributed to the reduction of blood pressure in these mice (Xia et al., 2009). In Ang II induced hypertension, reduced ACE2 gene and protein expression in the periventricular nucleus (PVN) was reported, and adenoviral injection directly in the PVN reduced blood pressure (Sriramula et al., 2011). A recent study in DOCA-salt hypertensive mice, demonstrated that low-renin hypertension was associated with reduced ACE2 expression and activity in the hypothalamus and increased ACE2 activity in the cerebrospinal fluid, as a result of increased shedding of ACE2 from the tissue membrane by ADAM17 (a disintegrin and metalloproteinase) (Xia et al., 2013). Furthermore, knockdown of ADAM17 prevented the reduction of ACE2 in the brain, which was associated with a blunting of DOCA-salt hypertension (Xia et al., 2013). This data suggests that central ACE2 shedding by ADAM17 can also contribute to the development of hypertension.
The development of ACE2 inhibitors (Dales et al., 2002) and approaches to enhance ACE2 including viral transfection, recombinant ACE2 and ACE2 activators (Hernandez Prada et al., 2008; Kulemina and Ostrov, 2011), have helped to clarify whether ACE2 deficiency has a pathophysiological role in the onset of hypertension or is simply a consequence of elevated blood pressure. Treatment with the ACE2 inhibitor MLN-4760 had no effect on blood pressure in hypertensive mRen2 transgenic rats (Trask et al., 2010), nor in wild type mice (Tikellis et al., 2008). In mice with genetic deletion of ACE2 on the C57BL/6 background, ACE2 deficiency was associated with only a modest increase in blood pressure (Gurley et al., 2006; Tikellis et al., 2012), but enhanced susceptibility to Ang II-induced hypertension (Gurley et al., 2006). Other studies have shown no change in blood pressure in ACE2 knock-out (KO) mice compared to control C57BL/6 (Tikellis et al., 2008; Shiota et al., 2010), but once diabetes was induced in ACE2 KO mice, hypertension did develop (Tikellis et al., 2008). This suggests that ACE2 plays a compensatory role in the setting of disease, but not in normal physiology. This notion is supported by results from ACE2 overexpression studies. For example, cardiac ACE2 gene transfer reduced blood pressure and attenuated cardiac hypertrophy and fibrosis in SHR, but had no effect in normotensive WKY rats (Diez-Freire et al., 2006), and intra-cardiac ACE2 overexpression protected from angiotensin II-induced cardiac hypertrophy and fibrosis, but had no effect in vehicle infused rats (Huentelman et al., 2005). In addition, transgenic ACE2 overexpression in vessels of stroke-prone SHR reduced blood pressure and improved endothelial function (Rentzsch et al., 2008).
It has been suggested that enhancing ACE2 activity may be a useful therapeutic approach in the management of high blood pressure. Current approaches to increase ACE2 activity include compounds that can activate ACE2 such as xanthenone (XNT) (Hernandez Prada et al., 2008) and all-trans retinoic acid (atRA) (Zhong et al., 2004). The compound atRA increased cardiac and kidney levels of ACE2 in SHR, reduced blood pressure and attenuated myocardial damage (Zhong et al., 2004). In vitro studies show that the ACE2 activator, XNT shifts the conformational equilibrium of ACE2 to increase its activity (Hernandez Prada et al., 2008), and acute administration in SHR reduced blood pressure, while chronic infusion reversed cardiac and renal fibrosis (Hernandez Prada et al., 2008). XNT infusion increased cardiac gene and protein ACE2 levels in SHR, which was associated with increased Ang 1-7 production and inhibition of ERK signaling (Ferreira et al., 2011). Central administration of XNT reduced acute air jet stress-induced increases in blood pressure (Martins Lima et al., 2013). By contrast, a recent study reported that XNT mediates its effects without activation of ACE2 (Haber et al., 2014). In a model of Ang II–induced acute hypertension, reduction in blood pressure was markedly enhanced by XNT with no effect on plasma nor kidney ACE2 activity (Haber et al., 2014). As the blood pressure-lowering effects of XNT were seen in both wild-type and ACE2 KO mice, this study concludes that the effects of XNT cannot be due to the activation of ACE2 (Haber et al., 2014). Further studies are needed to determine the precise mechanism of the antihypertensive and antifibrotic benefits of XNT.
A more direct approach to increase ACE2 is through recombinant ACE2. Recombinant human ACE2 (rhACE2) treatment partially reduced the pressor effect of an Ang II infusion without affecting basal systolic blood pressure in vehicle-treated mice (Zhong et al., 2010). Administration of rhACE2 also improved Ang II induced cardiac remodeling and dysfunction with attenuation of signaling pathways relevant for hypertrophy and fibrosis (Zhong et al., 2010). Both rhACE2 (Wysocki et al., 2010) and mouse recombinant ACE2 (Ye et al., 2012) attenuated Ang II induced hypertension in mice, with no effect on blood pressure in normotensive mice (Wysocki et al., 2010). However the initial increase in serum ACE2 observed in mice infused with rhACE2 was not sustained long-term due to the development of antibodies (Wysocki et al., 2010). The Ang II induced pressor effect in WKY rats was blunted with co-administration of rhACE2 (Lo et al., 2013), while in SHR 2-weeks of rhACE2 partially attenuated the blood pressure in association with reduced plasma Ang II levels and increased Ang 1-7 (Lo et al., 2013). Furthermore, lowering plasma and renal cortex levels of Ang II with rhACE2 over 4 weeks was associated with reduced blood pressure and slower progression of diabetic nephropathy in diabetic Akita mice (Oudit et al., 2010). A recent study examined the effect of intravenous administration of rhACE2 in healthy human subjects and found that a single rhACE2 dose of 100–1200 μg/kg was well tolerated and resulted in a dose-dependent increase in plasma ACE2, with no change in blood pressure or heart rate (Haschke et al., 2013). No antibodies to rhACE2 were detected 7, 14, and 28 days after the last dose of rhACE2 (Haschke et al., 2013). Studies evaluating the efficacy of this approach to lower blood pressure are currently lacking in patients with hypertension.
ACE2 undergoes “shedding” from endothelial cells to release the catalytically active ectodomain into the circulation (Lambert et al., 2005; Patel et al., 2013b). This process involves the proteinase ADAM17 (Lambert et al., 2005, 2008), and results in soluble ACE2, that can be measured in human plasma (Rice et al., 2006; Lew et al., 2008; Freeman et al., 2011; Chong et al., 2012; Soro-Paavonen et al., 2012; Ortiz-Perez et al., 2013; Roberts et al., 2013; Uri et al., 2014) using an ACE2-specific quenched fluorescent substrate assay described by Vickers et al. (2002). There are few studies that have specifically investigated whether circulating ACE2 activity is a biomarker of CVD in man, or if it is associated with blood pressure or hypertension. The results from the available studies suggest that plasma ACE2 activity is low in normal healthy volunteers (Lew et al., 2008), but increased in patients with cardiovascular risk factors or CVD (Freeman et al., 2011; Chong et al., 2012).
The Leeds Family study measured plasma ACE2 activity levels in healthy subjects and family members (Rice et al., 2006). Plasma ACE2 activity was detectable in only 40/537 subjects, and these subjects had significantly higher systolic and diastolic blood pressure compared to those without detectable plasma ACE2 (Rice et al., 2006). This study also provided evidence of genetic influences on circulating ACE2 activity and estimated that up to 67% of the variability in circulating ACE2 levels was explained by hereditable factors (Rice et al., 2006). This conclusion was based on the observation that among the 40 subjects with detectable ACE2 activity, half had at least one other family member with detectable ACE2 activity (Rice et al., 2006).
A number of other studies suggest an association between ACE2 activity and blood pressure. In patients with type 1 diabetes (n = 859), serum ACE2 activity was significantly higher in male compared to female patients, and was positively correlated with systolic blood pressure in both males and females (Soro-Paavonen et al., 2012). In patients with chronic kidney disease (n = 59), hemodialysis patients (n = 100) and kidney transplant recipients (n = 80) (Roberts et al., 2013), male patients had significantly higher plasma ACE2 activity compared to female patients; in females, plasma ACE2 activity was significantly associated with post-hemodialysis systolic blood pressure with a 4% increase in ACE2 for every 1 mmHg increase in systolic blood pressure. A recent study (Uri et al., 2014) has also reported that serum ACE2 activity was higher in hypertensive patients (n = 239) compared to healthy individuals (n = 45).
Others have reported no association between circulating ACE2 activity and hypertension. For example, monocyte-derived macrophage ACE2 activity in untreated hypertensive patients was not different to levels in normotensive individuals (Keidar et al., 2007), and in patients with ST-elevation myocardial infarction (n = 95), there was no association between ACE2 activity and hypertension (Ortiz-Perez et al., 2013). Whilst the studies by Soro-Paavonen et al. (2012) and Roberts et al. (2013) show that plasma ACE2 activity varies according to gender, most published ACE2 activity studies have not taken gender into account in their analysis.
There have been 17 studies since 2004 (summarized in Table 2) investigating ACE2 single nucleotide polymorphisms (SNPs) with blood pressure and/or hypertension (Burrell et al., 2013). These studies have been mainly conducted in Chinese populations (Huang et al., 2006; Yi et al., 2006; Zhong et al., 2006; Fan et al., 2007, 2009; Niu et al., 2007; Zhao et al., 2010a,b) with only four in Caucasians (Benjafield et al., 2004; Lieb et al., 2006; Patel et al., 2012; Malard et al., 2013), one in Koreans (Song et al., 2011) and one in an Indian population (Patnaik et al., 2014). Two ACE2 SNPs, rs2285666 and rs1978124 are among those most frequently investigated in association studies. In three Chinese studies, significant associations in women with the rs2285666 SNP with either hypertension or systolic or diastolic blood pressure were reported (Yi et al., 2006; Zhong et al., 2006; Niu et al., 2007). In two of these studies, higher blood pressure was associated with the A allele (Yi et al., 2006; Niu et al., 2007) but in the third study it was the G allele (Zhong et al., 2006). Another study investigated orthostatic blood pressure responses in 3630 Chinese Han subjects and found no association with the rs2285666 SNP (Fan et al., 2009).
Two meta-analyses of the rs2285666 SNP have been reported with conflicting results (Zhou and Yang, 2009; Lu et al., 2012). One study had a combined total of 2528 Chinese Han subjects with hypertension and 2024 ethnicity matched normotensive controls and reported no association with hypertension (Zhou and Yang, 2009). The other study, a meta-analysis of 7251 hypertensive patients, reported a significant association between the rs2285666 AA genotype with hypertension in females only (Lu et al., 2012). However, this meta-analysis included mixed ethnicities (Chinese Han, Chinese Dongxiang, Anglo-Celtic) and reported significant heterogeneity in males, suggesting that the A allele was associated with hypertension in the Chinese Han subjects only. In one Korean study, the ACE2 SNPs rs1514282 and rs1514283 were found to be significantly associated with diastolic pressure but not with hypertension (Song et al., 2011). A recent study investigated the ACE2 gene in 246 hypertensive subjects from Odisha, India, and reported that the SNP rs2106809 TT genotype in females and the T allele in males was associated with hypertension compared to normotensive controls (Patnaik et al., 2014). Further studies with ACE2 SNPs in other Indian populations will need to be conducted.
The evidence of ACE2 gene associations in Caucasians is also inconsistent. In a small case-control study conducted in Anglo-Celtic Australian subjects with hypertension (n = 152) or normotension (n = 193), four ACE2 SNPs were not associated with hypertension (Benjafield et al., 2004). In another study of healthy subjects participating in the MONICA Augsburg echocardiographic substudy, four ACE2 SNPs (rs4646156, rs879922, rs4240157, and rs233575) were investigated but none were associated with systolic blood pressure variation (Lieb et al., 2006). However, in our own study of 503 Australian Caucasians with type 2 diabetes (Patel et al., 2012), the prevalence of hypertension was significantly higher in both men and women and was associated with the G allele of the ACE2 SNP rs4240157 (Patel et al., 2012).
In another analysis, three ACE2 SNPs (rs1514283, rs4646176, and rs879922) were reported to be significantly associated with the diastolic blood pressure response to a cold pressor test in female Chinese subjects participating in the GenSalt study (Huang et al., 2012). One study in the Chinese population reported no association of ACE2 SNPs rs2106809 and rs2285666 with orthostatic blood pressure dysregulation in 3630 untreated hypertensive patients compared to 826 normotensive subjects (Fan et al., 2009).
The association of ACE2 SNPs with baseline blood pressure and blood pressure changes over 5 years was examined in 555 adolescents mainly of French Canadian or European descent (Malard et al., 2013). This study restricted the analyses to homozygous females. In European males the ACE2 SNP rs2074192 minor A allele was associated with a lower baseline diastolic and systolic blood pressure after weight and height adjustment. The ACE2 SNP rs233575 C allele was associated with higher baseline systolic and diastolic blood pressure in European males. In contrast, French Canadian females with the rs233575 T allele had a 4 mmHg higher baseline diastolic blood pressure compared to the SNPs C allele. With regards to ACE2 SNPs associated with changes in blood pressure from the baseline visit, there were no significant associations with the four ACE2 SNPs investigated in European or French Canadian males. However in females of European descent, the rs2074192 A allele was associated with a change in systolic blood pressure that was lower and the C allele of SNPs rs2333575 and rs2158083 were associated with a change in systolic blood pressure that was higher compared to the alternative allele (Malard et al., 2013). This study demonstrates that the association of the ACE2 SNPs with blood pressure was influenced by both gender and ethnicity.
A small number of dietary and pharmacological interventions in Chinese populations have also been conducted in relation to ACE2 polymorphisms. The GenSalt study in Chinese Han subjects examined responses to variation in sodium and potassium intake (Zhao et al., 2010a). This study reported ACE2 SNPs to be associated with the reduction in blood pressure in response to potassium while on a high salt intake in males only (Zhao et al., 2010a) and also with variations in sodium intake in males and females combined (Zhao et al., 2010b). One study investigated the blood pressure response to RAS blockade in Chinese Han subjects (Fan et al., 2007), and reported that the diastolic pressure response to ACE inhibition was 3.3 mmHg greater in women with the rs2106809 T allele after adjustments for age, pre-treatment blood pressure, body mass index and fasting blood glucose. There are no studies of ACE2 gene associations with blood pressure treatment responses in Caucasian populations.
The experimental studies clearly support a physiological and pathophysiological role for ACE2 in arterial hypertension, and data is also available that increasing/activating ACE2 has beneficial effects to lower blood pressure. As therapeutic strategies that block the RAS using ACE inhibitors or Ang II receptor blockers are first line therapy in hypertension, experimental studies are needed that combine ACE2 activators/recombinant ACE2 with RAS blockers to determine if this approach offers incremental benefits.
There are very few clinical studies that have specifically investigated whether circulating ACE2 activity is associated with blood pressure or hypertension. Plasma ACE2 activity levels are low in healthy individuals, and may or may not be increased in patients with hypertension. Males have higher circulating ACE2 activity than females, but few reports have analyzed data according to gender. There are 17 studies that have examined ACE2 genetic associations with blood pressure and hypertension; the majority are in Chinese populations, but the results have been inconsistent. There are not yet any studies that combine genetic approaches with measurement of plasma ACE2 activity. Large carefully conducted clinical studies, designed with sufficient numbers and power to allow separate analyses of males and females are urgently needed in patients with hypertension to more precisely clarify the potential role of ACE2 in blood pressure variation and hypertension, and to uncover mechanisms that might offer novel treatment targets.
The authors declare that the research was conducted in the absence of any commercial or financial relationships that could be construed as a potential conflict of interest.
ACE, angiotensin converting enzyme; ACE2, angiotensin converting enzyme 2; ADAM17, a disintegrin and metalloproteinase; Ang, angiotensin; AT1R, angiotensin type 1 receptor; AT2R, angiotensin type 2 receptor; atRA, all-trans retinoic acid; CV, cardiovascular; CVD, cardiovascular disease; DOCA, deoxycorticosterone; KO, knockout; PVN, periventricular nucleus; RAS, renin angiotensin system; rhACE2, recombinant human ACE2; RVLM, rostral ventrolateral medulla; SD, Sprague Dawley; SHR, spontaneously hypertensive rat; SNP, single nucleotide polymorphism; STNx, subtotal nephrectomy; WKY, Wistar-Kyoto; XNT, xanthenone.
Bai, S., Huang, Z. G., Chen, L., Wang, J. T., and Ding, B. P. (2013). Effects of felodipine combined with puerarin on ACE2-Ang (1-7)-Mas axis in renovascular hypertensive rat. Regul. Pept. 184, 54–61. doi: 10.1016/j.regpep.2013.03.005
Benjafield, A. V., Wang, W. Y., and Morris, B. J. (2004). No association of angiotensin-converting enzyme 2 gene (ACE2) polymorphisms with essential hypertension. Am. J. Hypertens. 17, 624–628. doi: 10.1016/j.amjhyper.2004.02.022
Burchill, L., Velkoska, E., Dean, R. G., Lew, R. A., Smith, A. I., Levidiotis, V., et al. (2008). Acute kidney injury in the rat causes cardiac remodelling and increases angiotensin-converting enzyme 2 expression. Exp. Physiol. 93, 622–630. doi: 10.1113/expphysiol.2007.040386
Burgelova, M., Vanourkova, Z., Thumova, M., Dvorak, P., Opocensky, M., Kramer, H. J., et al. (2009). Impairment of the angiotensin-converting enzyme 2-angiotensin-(1-7)-Mas axis contributes to the acceleration of two-kidney, one-clip Goldblatt hypertension. J. Hypertens. 27, 1988–2000. doi: 10.1097/HJH.0b013e32832f0d06
Burrell, L. M., Burchill, L., Dean, R. G., Griggs, K., Patel, S. K., and Velkoska, E. (2012). Chronic kidney disease: cardiac and renal angiotensin converting enzyme (ACE) 2 expression in rats after subtotal nephrectomy and the effect of ACE inhibition. Exp. Physiol. 97, 477–485. doi: 10.1113/expphysiol.2011.063156
Burrell, L. M., Harrap, S. B., Velkoska, E., and Patel, S. K. (2013). The ACE2 gene: its potential as a functional candidate for cardiovascular disease. Clin. Sci. (Lond.) 124, 65–76. doi: 10.1042/CS20120269
Burrell, L. M., Risvanis, J., Kubota, E., Dean, R. G., MacDonald, P. S., Lu, S., et al. (2005). Myocardial infarction increases ACE2 expression in rat and humans. Eur. Heart J. 26, 369–375. doi: 10.1093/eurheartj/ehi114
Chong, C. P., Lim, W. K., Velkoska, E., van Gaal, W. J., Ryan, J. E., Savige, J., et al. (2012). N-terminal pro-brain natriuretic peptide and angiotension converting enzyme 2 levels and their association with post-operative cardiac complications after emergency orthopaedic surgery. Am. J. Cardiol. 109, 1365–1373. doi: 10.1016/j.amjcard.2011.12.032
Crackower, M. A., Sarao, R., Oudit, G. Y., Yagil, C., Kozieradzki, I., Scanga, S. E., et al. (2002). Angiotensin-converting enzyme 2 is an essential regulator of heart function. Nature 417, 822–828. doi: 10.1038/nature00786
Dales, N. A., Gould, A. E., Brown, J. A., Calderwood, E. F., Guan, B., Minor, C. A., et al. (2002). Substrate-based design of the first class of angiotensin-converting enzyme-related carboxypeptidase (ACE2) inhibitors. J. Am. Chem. Soc. 124, 11852–11853. doi: 10.1021/ja0277226
Diez-Freire, C., Vazquez, J., Correa de Adjounian, M. F., Ferrari, M. F., Yuan, L., Silver, X., et al. (2006). ACE2 gene transfer attenuates hypertension-linked pathophysiological changes in the SHR. Physiol. Genomics 27, 12–19. doi: 10.1152/physiolgenomics.00312.2005
Dilauro, M., Zimpelmann, J., Robertson, S. J., Genest, D., and Burns, K. D. (2010). The effect of ACE2 and angiotensin-(1-7) in a mouse model of early chronic kidney disease. Am. J. Physiol. Renal Physiol. 298, F1523–F1532. doi: 10.1152/ajprenal.00426.2009
Donoghue, M., Hsieh, F., Baronas, E., Godbout, K., Gosselin, M., Stagliano, N., et al. (2000). A novel angiotensin-converting enzyme-related carboxypeptidase (ACE2) converts angiotensin I to angiotensin 1-9. Circ. Res. 87, E1–E9. doi: 10.1161/01.RES.87.5.e1
Doobay, M. F., Talman, L. S., Obr, T. D., Tian, X., Davisson, R. L., and Lazartigues, E. (2007). Differential expression of neuronal ACE2 in transgenic mice with overexpression of the brain renin-angiotensin system. Am. J. Physiol. Regul. Integr. Comp. Physiol. 292, R373–R381. doi: 10.1152/ajpregu.00292.2006
Dzau, V. (2005). The cardiovascular continuum and renin-angiotensin-aldosterone system blockade. J. Hypertens. Suppl. 23, S9–S17. doi: 10.1097/01.hjh.0000165623.72310.dd
Fan, X., Wang, Y., Sun, K., Zhang, W., Yang, X., Wang, S., et al. (2007). Polymorphisms of ACE2 gene are associated with essential hypertension and antihypertensive effects of Captopril in women. Clin. Pharmacol. Ther. 82, 187–196. doi: 10.1038/sj.clpt.6100214
Fan, X. H., Wang, Y. B., Wang, H., Sun, K., Zhang, W. L., Song, X. D., et al. (2009). Polymorphisms of angiotensin-converting enzyme (ACE) and ACE2 are not associated with orthostatic blood pressure dysregulation in hypertensive patients. Acta Pharmacol. Sin. 30, 1237–1244. doi: 10.1038/aps.2009.110
Ferreira, A. J., Shenoy, V., Qi, Y., Fraga-Silva, R. A., Santos, R. A., Katovich, M. J., et al. (2011). Angiotensin-converting enzyme 2 activation protects against hypertension-induced cardiac fibrosis involving extracellular signal-regulated kinases. Exp. Physiol. 96, 287–294. doi: 10.1113/expphysiol.2010.055277
Freeman, M., Patel, S. K., Lancefield, T., Velkoska, E., Griggs, K., Dean, R. G., et al. (2011). Angiotensin converting enzyme 2: a novel marker of coronary artery disease. Eur. Heart J. 31, 515.
Gurley, S. B., Allred, A., Le, T. H., Griffiths, R., Mao, L., Philip, N., et al. (2006). Altered blood pressure responses and normal cardiac phenotype in ACE2-null mice. J. Clin. Invest. 116, 2218–2225. doi: 10.1172/JCI16980
Haber, P. K., Ye, M., Wysocki, J., Maier, C., Haque, S. K., and Batlle, D. (2014). Angiotensin-converting enzyme 2-independent action of presumed angiotensin-converting enzyme 2 activators: studies in vivo, ex vivo, and in vitro. Hypertension 63, 774–782. doi: 10.1161/HYPERTENSIONAHA.113.02856
Haschke, M., Schuster, M., Poglitsch, M., Loibner, H., Salzberg, M., Bruggisser, M., et al. (2013). Pharmacokinetics and pharmacodynamics of recombinant human angiotensin-converting enzyme 2 in healthy human subjects. Clin. Pharmacokinet. 52, 783–792. doi: 10.1007/s40262-013-0072-7
Hernandez Prada, J. A., Ferreira, A. J., Katovich, M. J., Shenoy, V., Qi, Y., Santos, R. A., et al. (2008). Structure-based identification of small-molecule angiotensin-converting enzyme 2 activators as novel antihypertensive agents. Hypertension 51, 1312–1317. doi: 10.1161/HYPERTENSIONAHA.107.108944
Huang, J., Chen, S., Lu, X., Zhao, Q., Rao, D. C., Jaquish, C. E., et al. (2012). Polymorphisms of ACE2 are associated with blood pressure response to cold pressor test: the GenSalt study. Am. J. Hypertens. 25, 937–942. doi: 10.1038/ajh.2012.61
Huang, W., Yang, W., Wang, Y., Zhao, Q., Gu, D., and Chen, R. (2006). Association study of angiotensin-converting enzyme 2 gene (ACE2) polymorphisms and essential hypertension in northern Han Chinese. J. Hum. Hypertens. 20, 968–971. doi: 10.1038/sj.jhh.1002090
Huentelman, M. J., Grobe, J. L., Vazquez, J., Stewart, J. M., Mecca, A. P., Katovich, M. J., et al. (2005). Protection from angiotensin II-induced cardiac hypertrophy and fibrosis by systemic lentiviral delivery of ACE2 in rats. Exp. Physiol. 90, 783–790. doi: 10.1113/expphysiol.2005.031096
Johnston, C. I. (1994). Tissue angiotensin converting enzyme in cardiac and vascular hypertrophy, repair, and remodeling. Hypertension 23, 258–268. doi: 10.1161/01.HYP.23.2.258
Kamilic, J., Hamming, I., Kreutz, R., Bolbrinker, J., Siems, W. E., Nassar, I., et al. (2010). Renal ACE2 expression and activity is unaltered during established hypertension in adult SHRSP and TGR(mREN2)27. Hypertens. Res. 33, 123–128. doi: 10.1038/hr.2009.191
Kearney, P. M., Whelton, M., Reynolds, K., Muntner, P., Whelton, P. K., and He, J. (2005). Global burden of hypertension: analysis of worldwide data. Lancet 365, 217–223. doi: 10.1016/S0140-6736(05)17741-1
Keidar, S., Strizevsky, A., Raz, A., and Gamliel-Lazarovich, A. (2007). ACE2 activity is increased in monocyte-derived macrophages from prehypertensive subjects. Nephrol. Dial. Transplant 22, 597–601. doi: 10.1093/ndt/gfl632
Kulemina, L. V., and Ostrov, D. A. (2011). Prediction of off-target effects on angiotensin-converting enzyme 2. J. Biomol. Screen. 16, 878–885. doi: 10.1177/1087057111413919
Lambert, D. W., Clarke, N. E., Hooper, N. M., and Turner, A. J. (2008). Calmodulin interacts with angiotensin-converting enzyme-2 (ACE2) and inhibits shedding of its ectodomain. FEBS Lett. 582, 385–390. doi: 10.1016/j.febslet.2007.11.085
Lambert, D. W., Yarski, M., Warner, F. J., Thornhill, P., Parkin, E. T., Smith, A. I., et al. (2005). Tumor necrosis factor-alpha convertase (ADAM17) mediates regulated ectodomain shedding of the severe-acute respiratory syndrome-coronavirus (SARS-CoV) receptor, angiotensin-converting enzyme-2 (ACE2). J. Biol. Chem. 280, 30113–30119. doi: 10.1074/jbc.M505111200
Landau, D., Chayat, C., Zucker, N., Golomb, E., Yagil, C., Yagil, Y., et al. (2008). Early blood pressure-independent cardiac changes in diabetic rats. J. Endocrinol. 197, 75–83. doi: 10.1677/JOE-08-0040
Lemarie, C. A., and Schiffrin, E. L. (2010). The angiotensin II type 2 receptor in cardiovascular disease. J. Renin Angiotensin Aldosterone Syst. 11, 19–31. doi: 10.1177/1470320309347785
Lew, R. A., Warner, F. J., Hanchapola, I., Yarski, M. A., Manohar, J., Burrell, L. M., et al. (2008). Angiotensin-converting enzyme 2 catalytic activity in human plasma is masked by an endogenous inhibitor. Exp. Physiol. 93, 685–693. doi: 10.1113/expphysiol.2007.040352
Lieb, W., Graf, J., Gotz, A., Konig, I. R., Mayer, B., Fischer, M., et al. (2006). Association of angiotensin-converting enzyme 2 (ACE2) gene polymorphisms with parameters of left ventricular hypertrophy in men. Results of the MONICA Augsburg echocardiographic substudy. J. Mol. Med. 84, 88–96. doi: 10.1007/s00109-005-0718-5
Lo, J., Patel, V. B., Wang, Z., Levasseur, J., Kaufman, S., Penninger, J. M., et al. (2013). Angiotensin-converting enzyme 2 antagonizes angiotensin II-induced pressor response and NADPH oxidase activation in Wistar-Kyoto rats and spontaneously hypertensive rats. Exp. Physiol. 98, 109–122. doi: 10.1113/expphysiol.2012.067165
Lu, N., Yang, Y., Wang, Y., Liu, Y., Fu, G., Chen, D., et al. (2012). ACE2 gene polymorphism and essential hypertension: an updated meta-analysis involving 11,051 subjects. Mol. Biol. Rep. 39, 6581–6589. doi: 10.1007/s11033-012-1487-1
Malard, L., Kakinami, L., O'Loughlin, J., Roy-Gagnon, M. H., Labbe, A., Pilote, L., et al. (2013). The association between the Angiotensin-Converting Enzyme-2 gene and blood pressure in a cohort study of adolescents. BMC Med. Genet. 14:117. doi: 10.1186/1471-2350-14-117
Martins Lima, A., Xavier, C. H., Ferreira, A. J., Raizada, M. K., Wallukat, G., Velloso, E. P., et al. (2013). Activation of angiotensin-converting enzyme 2/angiotensin-(1-7)/Mas axis attenuates the cardiac reactivity to acute emotional stress. Am. J. Physiol. Heart Circ. Physiol. 305, H1057–H1067. doi: 10.1152/ajpheart.00433.2013
McCarthy, C. A., Widdop, R. E., Denton, K. M., and Jones, E. S. (2013). Update on the angiotensin AT(2) receptor. Curr. Hypertens. Rep. 15, 25–30. doi: 10.1007/s11906-012-0321-4
Meng, W., Zhao, W., Zhao, T., Liu, C., Chen, Y., Liu, H., et al. (2014). Autocrine and paracrine function of angiotensin 1-7 in tissue repair during hypertension. Am. J. Hypertens. 27, 775–782. doi: 10.1093/ajh/hpt270
Mizuiri, S., Hemmi, H., Arita, M., Ohashi, Y., Tanaka, Y., Miyagi, M., et al. (2008). Expression of ACE and ACE2 in individuals with diabetic kidney disease and healthy controls. Am. J. Kidney Dis. 51, 613–623. doi: 10.1053/j.ajkd.2007.11.022
Niu, W., Qi, Y., Hou, S., Zhou, W., and Qiu, C. (2007). Correlation of angiotensin-converting enzyme 2 gene polymorphisms with stage 2 hypertension in Han Chinese. Transl. Res. 150, 374–380. doi: 10.1016/j.trsl.2007.06.002
Ocaranza, M. P., Rivera, P., Novoa, U., Pinto, M., Gonzalez, L., Chiong, M., et al. (2011). Rho kinase inhibition activates the homologous angiotensin-converting enzyme-angiotensin-(1-9) axis in experimental hypertension. J. Hypertens. 29, 706–715. doi: 10.1097/HJH.0b013e3283440665
Ohtsuki, M., Morimoto, S., Izawa, H., Ismail, T. F., Ishibashi-Ueda, H., Kato, Y., et al. (2010). Angiotensin converting enzyme 2 gene expression increased compensatory for left ventricular remodeling in patients with end-stage heart failure. Int. J. Cardiol. 145, 333–334. doi: 10.1016/j.ijcard.2009.11.057
Ortiz-Perez, J. T., Riera, M., Bosch, X., De Caralt, T. M., Perea, R. J., Pascual, J., et al. (2013). Role of circulating angiotensin converting enzyme 2 in left ventricular remodeling following myocardial infarction: a prospective controlled study. PLoS ONE 8:e61695. doi: 10.1371/journal.pone.0061695
Oudit, G. Y., Liu, G. C., Zhong, J., Basu, R., Chow, F. L., Zhou, J., et al. (2010). Human recombinant ACE2 reduces the progression of diabetic nephropathy. Diabetes 59, 529–538. doi: 10.2337/db09-1218
Paizis, G., Tikellis, C., Cooper, M. E., Schembri, J. M., Lew, R. A., Smith, A. I., et al. (2005). Chronic liver injury in rats and humans upregulates the novel enzyme angiotensin converting enzyme 2. Gut 54, 1790–1796. doi: 10.1136/gut.2004.062398
Patel, S. K., Velkoska, E., and Burrell, L. M. (2013b). Emerging markers in cardiovascular disease: where does angiotensin-converting enzyme 2 fit in? Clin. Exp. Pharmacol. Physiol. 40, 551–559. doi: 10.1111/1440-1681.12069
Patel, S. K., Wai, B., Ord, M., Macisaac, R. J., Grant, S., Velkoska, E., et al. (2012). Association of ACE2 genetic variants with blood pressure, left ventricular mass, and cardiac function in Caucasians with type 2 diabetes. Am. J. Hypertens. 25, 216–222. doi: 10.1038/ajh.2011.188
Patel, V. B., Clarke, N., Wang, Z., Fan, D., Parajuli, N., Basu, R., et al. (2013a). Angiotensin II induced proteolytic cleavage of myocardial ACE2 is mediated by TACE/ADAM-17: a positive feedback mechanism in the RAS. J. Mol. Cell. Cardiol. 66, 167–176. doi: 10.1016/j.yjmcc.2013.11.017
Patnaik, M., Pati, P., Swain, S. N., Mohapatra, M. K., Dwibedi, B., Kar, S. K., et al. (2014). Association of angiotensin-converting enzyme and angiotensin-converting enzyme-2 gene polymorphisms with essential hypertension in the population of Odisha, India. Ann. Hum. Biol. 41, 143–150. doi: 10.3109/03014460.2013.837195
Pendergrass, K. D., Pirro, N. T., Westwood, B. M., Ferrario, C. M., Brosnihan, K. B., and Chappell, M. C. (2008). Sex differences in circulating and renal angiotensins of hypertensive mRen(2). Lewis but not normotensive Lewis rats. Am. J. Physiol. Heart Circ. Physiol. 295, H10–H20. doi: 10.1152/ajpheart.01277.2007
Prieto, M. C., Gonzalez-Villalobos, R. A., Botros, F. T., Martin, V. L., Pagan, J., Sato, R., et al. (2011). Reciprocal changes in renal ACE/Ang II and ACE2/Ang 1-7 are associated with enhanced collecting duct renin in Goldblatt hypertensive rats. Am. J. Physiol. Renal Physiol. 300, F749–F755. doi: 10.1152/ajprenal.00383.2009
Reich, H. N., Oudit, G. Y., Penninger, J. M., Scholey, J. W., and Herzenberg, A. M. (2008). Decreased glomerular and tubular expression of ACE2 in patients with type 2 diabetes and kidney disease. Kidney Int. 74, 1610–1616. doi: 10.1038/ki.2008.497
Rentzsch, B., Todiras, M., Iliescu, R., Popova, E., Campos, L. A., Oliveira, M. L., et al. (2008). Transgenic angiotensin-converting enzyme 2 overexpression in vessels of SHRSP rats reduces blood pressure and improves endothelial function. Hypertension 52, 967–973. doi: 10.1161/HYPERTENSIONAHA.108.114322
Rice, G. I., Jones, A. L., Grant, P. J., Carter, A. M., Turner, A. J., and Hooper, N. M. (2006). Circulating activities of angiotensin-converting enzyme, its homolog, angiotensin-converting enzyme 2, and neprilysin in a family study. Hypertension 48, 914–920. doi: 10.1161/01.HYP.0000244543.91937.79
Roberts, M. A., Velkoska, E., Ierino, F. L., and Burrell, L. M. (2013). Angiotensin-converting enzyme 2 activity in patients with chronic kidney disease. Nephrol. Dial. Transplant 28, 2287–2294. doi: 10.1093/ndt/gft038
Santiago, N. M., Guimaraes, P. S., Sirvente, R. A., Oliveira, L. A., Irigoyen, M. C., and Santos, R. A. (2010). Campagnole-Santos, MJ. Lifetime overproduction of circulating angiotensin-(1-7) attenuates deoxycorticosterone acetate-salt hypertension-induced cardiac dysfunction and remodeling. Hypertension 55, 889-96. doi: 10.1161/HYPERTENSIONAHA.110.149815
Santos, R. A. (2014). Angiotensin-(1-7). Hypertension 63, 1138–1147. doi: 10.1161/HYPERTENSIONAHA.113.01274
Santos, R. A., Simoes e Silva, A. C., Maric, C., Silva, D. M., Machado, R. P., de Buhr, I., et al. (2003). Angiotensin-(1-7) is an endogenous ligand for the G protein-coupled receptor Mas. Proc. Natl. Acad. Sci. U.S.A. 100, 8258–8263. doi: 10.1073/pnas.1432869100
Shiota, A., Yamamoto, K., Ohishi, M., Tatara, Y., Ohnishi, M., Maekawa, Y., et al. (2010). Loss of ACE2 accelerates time-dependent glomerular and tubulointerstitial damage in streptozotocin-induced diabetic mice. Hypertens. Res. 33, 298–307. doi: 10.1038/hr.2009.231
Sluimer, J. C., Gasc, J. M., Hamming, I., van Goor, H., Michaud, A., van den Akker, L. H., et al. (2008). Angiotensin-converting enzyme 2 (ACE2) expression and activity in human carotid atherosclerotic lesions. J. Pathol. 215, 273–279. doi: 10.1002/path.2357
Song, S. B., Jin, H. S., Hong, K. W., Lim, J. E., Moon, J. Y., Jeong, K. H., et al. (2011). Association between renin-angiotensin-aldosterone system-related genes and blood pressure in a Korean population. Blood Press. 20, 204–210. doi: 10.3109/08037051.2011.555074
Soro-Paavonen, A., Gordin, D., Forsblom, C., Rosengard-Barlund, M., Waden, J., Thorn, L., et al. (2012). Circulating ACE2 activity is increased in patients with type 1 diabetes and vascular complications. J. Hypertens. 30, 375–383. doi: 10.1097/HJH.0b013e32834f04b6
Sriramula, S., Cardinale, J. P., Lazartigues, E., and Francis, J. (2011). ACE2 overexpression in the paraventricular nucleus attenuates angiotensin II-induced hypertension. Cardiovasc. Res. 92, 401–408. doi: 10.1093/cvr/cvr242
Takeda, Y., Zhu, A., Yoneda, T., Usukura, M., Takata, H., and Yamagishi, M. (2007). Effects of aldosterone and angiotensin II receptor blockade on cardiac angiotensinogen and angiotensin-converting enzyme 2 expression in Dahl salt-sensitive hypertensive rats. Am. J. Hypertens. 20, 1119–1124. doi: 10.1016/j.amjhyper.2007.05.008
Tallant, E. A., and Clark, M. A. (2003). Molecular mechanisms of inhibition of vascular growth by angiotensin-(1-7). Hypertension 42, 574–579. doi: 10.1161/01.HYP.0000090322.55782.30
Tan, Z., Wu, J., and Ma, H. (2011). Regulation of angiotensin-converting enzyme 2 and Mas receptor by Ang-(1-7) in heart and kidney of spontaneously hypertensive rats. J. Renin Angiotensin Aldosterone Syst. 12, 413–419. doi: 10.1177/1470320311402109
Tikellis, C., Bialkowski, K., Pete, J., Sheehy, K., Su, Q., Johnston, C., et al. (2008). ACE2 deficiency modifies renoprotection afforded by ACE inhibition in experimental diabetes. Diabetes 57, 1018–1025. doi: 10.2337/db07-1212
Tikellis, C., Cooper, M. E., Bialkowski, K., Johnston, C. I., Burns, W. C., Lew, R. A., et al. (2006). Developmental expression of ACE2 in the SHR kidney: a role in hypertension? Kidney Int. 70, 34–41. doi: 10.1038/sj.ki.5000428
Tikellis, C., Johnston, C. I., Forbes, J. M., Burns, W. C., Burrell, L. M., Risvanis, J., et al. (2003). Characterization of renal angiotensin-converting enzyme 2 in diabetic nephropathy. Hypertension 41, 392–397. doi: 10.1161/01.HYP.0000060689.38912.CB
Tikellis, C., Pickering, R., Tsorotes, D., Du, X. J., Kiriazis, H., Nguyen-Huu, T. P., et al. (2012). Interaction of diabetes and ACE2 in the pathogenesis of cardiovascular disease in experimental diabetes. Clin. Sci. (Lond.) 123, 519–529. doi: 10.1042/CS20110668
Tipnis, S. R., Hooper, N. M., Hyde, R., Karran, E., Christie, G., and Turner, A. J. (2000). A human homolog of angiotensin-converting enzyme. Cloning and functional expression as a captopril-insensitive carboxypeptidase. J. Biol. Chem. 275, 33238–33243. doi: 10.1074/jbc.M002615200
Trask, A. J., Groban, L., Westwood, B. M., Varagic, J., Ganten, D., Gallagher, P. E., et al. (2010). Inhibition of angiotensin-converting enzyme 2 exacerbates cardiac hypertrophy and fibrosis in Ren-2 hypertensive rats. Am. J. Hypertens. 23, 687–693. doi: 10.1038/ajh.2010.51
Uri, K., Fagyas, M., Manyine Siket, I., Kertesz, A., Csanadi, Z., Sandorfi, G., et al. (2014). New perspectives in the renin-angiotensin-aldosterone system (RAAS) IV: circulating ACE2 as a biomarker of systolic dysfunction in human hypertension and heart failure. PLoS ONE 9:e87845. doi: 10.1371/journal.pone.0087845
Varagic, J., Ahmad, S., Brosnihan, K. B., Groban, L., Chappell, M. C., Tallant, E. A., et al. (2010). Decreased cardiac Ang-(1-7) is associated with salt-induced cardiac remodeling and dysfunction. Ther. Adv. Cardiovasc. Dis. 4, 17–25. doi: 10.1177/1753944709353337
Varagic, J., Ahmad, S., Voncannon, J. L., Moniwa, N., Simington, S. W. Jr., Brosnihan, B. K., et al. (2012). Nebivolol reduces cardiac angiotensin II, associated oxidative stress and fibrosis but not arterial pressure in salt-loaded spontaneously hypertensive rats. J. Hypertens. 30, 1766–1774. doi: 10.1097/HJH.0b013e328356766f
Velkoska, E., Dean, R. G., Burchill, L., Levidiotis, V., and Burrell, L. M. (2010). Reduction in renal ACE2 expression in subtotal nephrectomy in rats is ameliorated with ACE inhibition. Clin. Sci. (Lond.) 118, 269–279. doi: 10.1042/CS20090318
Velkoska, E., Dean, R. G., Griggs, K., Burchill, L., and Burrell, L. M. (2011). Angiotensin-(1-7) infusion is associated with increased blood pressure and adverse cardiac remodelling in rats with subtotal nephrectomy. Clin. Sci. (Lond.) 120, 335–345. doi: 10.1042/CS20100280
Vickers, C., Hales, P., Kaushik, V., Dick, L., Gavin, J., Tang, J., et al. (2002). Hydrolysis of biological peptides by human angiotensin-converting enzyme-related carboxypeptidase. J. Biol. Chem. 277, 14838–14843. doi: 10.1074/jbc.M200581200
World Health Organization. (2011). Global Atlas on Cardiovascular Disease Prevention and Control. eds S. Mendis, P. Puska, and B. Norrving (Geneva: World Health Organization).
Wysocki, J., Ye, M., Rodriguez, E., Gonzalez-Pacheco, F. R., Barrios, C., Evora, K., et al. (2010). Targeting the degradation of angiotensin II with recombinant angiotensin-converting enzyme 2: prevention of angiotensin II-dependent hypertension. Hypertension 55, 90–98. doi: 10.1161/HYPERTENSIONAHA.109.138420
Xia, H., Feng, Y., Obr, T. D., Hickman, P. J., and Lazartigues, E. (2009). Angiotensin II type 1 receptor-mediated reduction of angiotensin-converting enzyme 2 activity in the brain impairs baroreflex function in hypertensive mice. Hypertension 53, 210–216. doi: 10.1161/HYPERTENSIONAHA.108.123844
Xia, H., Sriramula, S., Chhabra, K. H., and Lazartigues, E. (2013). Brain ACE2 shedding contributes to the development of neurogenic hypertension. Circ. Res. 113, 1087–1096. doi: 10.1161/CIRCRESAHA.113.301811
Xu, P., Sriramula, S., and Lazartigues, E. (2011). ACE2/ANG-(1-7)/Mas pathway in the brain: the axis of good. Am. J. Physiol. Regul. Integr. Comp. Physiol. 300, R804–R817. doi: 10.1152/ajpregu.00222.2010
Yagil, Y., and Yagil, C. (2003). Hypothesis: ACE2 modulates blood pressure in the mammalian organism. Hypertension 41, 871–873. doi: 10.1161/01.HYP.0000063886.71596.C8
Yamazato, M., Yamazato, Y., Sun, C., Diez-Freire, C., and Raizada, M. K. (2007). Overexpression of angiotensin-converting enzyme 2 in the rostral ventrolateral medulla causes long-term decrease in blood pressure in the spontaneously hypertensive rats. Hypertension 49, 926–931. doi: 10.1161/01.HYP.0000259942.38108.20
Yang, Z., Yu, X., Cheng, L., Miao, L.-Y., Li, H.-X., Han, L.-H., et al. (2013). Effects of enalapril on the expression of cardiac angiotensin-converting enzyme and angiotensin-converting enzyme 2 in spontaneously hypertensive rats. Arch. Cardiovasc. Dis. 106, 196–201. doi: 10.1016/j.acvd.2013.01.004
Ye, M., Wysocki, J., Gonzalez-Pacheco, F. R., Salem, M., Evora, K., Garcia-Halpin, L., et al. (2012). Murine recombinant angiotensin-converting enzyme 2: effect on angiotensin II-dependent hypertension and distinctive angiotensin-converting enzyme 2 inhibitor characteristics on rodent and human angiotensin-converting enzyme 2. Hypertension 60, 730–740. doi: 10.1161/HYPERTENSIONAHA.112.198622
Yi, L., Gu, Y. H., Wang, X. L., An, L. Z., Xie, X. D., Shao, W., et al. (2006). Association of ACE, ACE2 and UTS2 polymorphisms with essential hypertension in Han and Dongxiang populations from north-western China. J. Int. Med. Res. 34, 272–283. doi: 10.1177/147323000603400306
Zhao, Q., Gu, D., Kelly, T. N., Hixson, J. E., Rao, D. C., Jaquish, C. E., et al. (2010a). Association of genetic variants in the apelin-APJ system and ACE2 with blood pressure responses to potassium supplementation: the GenSalt study. Am. J. Hypertens. 23, 606–613. doi: 10.1038/ajh.2010.36
Zhao, Q., Hixson, J. E., Rao, D. C., Gu, D., Jaquish, C. E., Rice, T., et al. (2010b). Genetic variants in the apelin system and blood pressure responses to dietary sodium interventions: a family-based association study. J. Hypertens. 28, 756–763. doi: 10.1097/HJH.0b013e3283370d32
Zhong, J., Basu, R., Guo, D., Chow, F. L., Byrns, S., Schuster, M., et al. (2010). Angiotensin-converting enzyme 2 suppresses pathological hypertrophy, myocardial fibrosis, and cardiac dysfunction. Circulation 122, 717–728. doi: 10.1161/CIRCULATIONAHA.110.955369
Zhong, J., Guo, D., Chen, C. B., Wang, W., Schuster, M., Loibner, H., et al. (2011). Prevention of angiotensin II-mediated renal oxidative stress, inflammation, and fibrosis by angiotensin-converting enzyme 2. Hypertension 57, 314–322. doi: 10.1161/HYPERTENSIONAHA.110.164244
Zhong, J., Yan, Z., Liu, D., Ni, Y., Zhao, Z., Zhu, S., et al. (2006). Association of angiotensin-converting enzyme 2 gene A/G polymorphism and elevated blood pressure in Chinese patients with metabolic syndrome. J. Lab. Clin. Med. 147, 91–95. doi: 10.1016/j.lab.2005.10.001
Zhong, J. C., Huang, D. Y., Yang, Y. M., Li, Y. F., Liu, G. F., Song, X. H., et al. (2004). Upregulation of angiotensin-converting enzyme 2 by all-trans retinoic acid in spontaneously hypertensive rats. Hypertension 44, 907–912. doi: 10.1161/01.HYP.0000146400.57221.74
Zhou, J. B., and Yang, J. K. (2009). Meta-analysis of association of ACE2 G8790A polymorphism with Chinese Han essential hypertension. J. Renin Angiotensin Aldosterone Syst. 10, 31–34. doi: 10.1177/1470320309103047
Zulli, A., Burrell, L. M., Buxton, B. F., and Hare, D. L. (2008). ACE2 and AT4R are present in diseased human blood vessels. Eur. J. Histochem. 52, 39–44.
Keywords: renin angiotensin system, angiotensin converting enzyme, angiotensin converting enzyme 2, blood pressure, hypertension
Citation: Patel SK, Velkoska E, Freeman M, Wai B, Lancefield TF and Burrell LM (2014) From gene to protein—experimental and clinical studies of ACE2 in blood pressure control and arterial hypertension. Front. Physiol. 5:227. doi: 10.3389/fphys.2014.00227
Received: 15 April 2014; Paper pending published: 05 May 2014;
Accepted: 02 June 2014; Published online: 24 June 2014.
Edited by:
Marcelo Roberto Choi, National Scientific and Technical Research Council (CONICET), ArgentinaReviewed by:
Geoffrey A. Head, Baker IDI Heart and Diabetes Institute, AustraliaCopyright © 2014 Patel, Velkoska, Freeman, Wai, Lancefield and Burrell. This is an open-access article distributed under the terms of the Creative Commons Attribution License (CC BY). The use, distribution or reproduction in other forums is permitted, provided the original author(s) or licensor are credited and that the original publication in this journal is cited, in accordance with accepted academic practice. No use, distribution or reproduction is permitted which does not comply with these terms.
*Correspondence: Louise M. Burrell, Department of Medicine, Austin Health, University of Melbourne, Level 7, Lance Townsend Building, 145 Studley Road, Heidelberg, VIC 3084, Australia e-mail:bC5idXJyZWxsQHVuaW1lbGIuZWR1LmF1
Disclaimer: All claims expressed in this article are solely those of the authors and do not necessarily represent those of their affiliated organizations, or those of the publisher, the editors and the reviewers. Any product that may be evaluated in this article or claim that may be made by its manufacturer is not guaranteed or endorsed by the publisher.
Research integrity at Frontiers
Learn more about the work of our research integrity team to safeguard the quality of each article we publish.