- 1 University Camilo Castelo Branco, São Paulo, Brazil
- 2 Gr. T. PopaCenter for Biomedical Research University of Medicine and Pharmacy, Iasi, Romania
- 3 Department of Research, Experimental Cardiology Research Group, University of Basel, Basel, Switzerland
- 4 Department of Physiology and Biophysics, University of Mississippi Medical Center, Jackson, MS, USA
- 5 Critical Care Development, Novartis Pharma AG, Basel, Switzerland
Several clinical studies have investigated the potential benefits of endothelin receptor antagonism in chronic pathologies such as diabetic kidney disease. However, fluid retention and edema have been identified as major side effects of endothelin receptor antagonists. In the present study we hypothesized that avosentan which was described as a predominant ETA receptor antagonist would produce fluid retention at high concentrations where non-specific blockade of ETB receptors may occur. Incremental doses of the predominant ETA receptor antagonist SPP301 (0.003; 0.03; 3 mg/kg) were administered intravenously to anesthetized Sprague-Dawley rats undergoing saline diuresis. Diuresis, glomerular filtration rate, and blood pressure (BP) were monitored. SPP301 decreased urine output (5.6; 34.8; 58.8% decrease from vehicle) and fractional excretion of water (5.7; 31.7; 56.4% decrease from vehicle) in a concentration-dependent manner. Glomerular filtration rate was unchanged while BP was reduced by 10 mmHg only by the highest dose of SPP301. Administration of the ETB selective receptor antagonist BQ-788 (3 mg/kg) following SPP301 3 mg/kg did not further decrease urine output or water excretion and was without effect on glomerular filtration rate. These data indicate that increasing concentrations of SPP301 may also block ETB receptors and cause antidiuresis. This effect could explain why fluid retention and edema occur during treatment with predominant ETA receptor blockers.
Introduction
Endothelin-1 (ET-1) is a 21 amino acid peptide derived from the vascular endothelium that has potent vasoactive properties (Yanagisawa et al., 1988). ET-1 induces its effect by acting on endothelin A and B receptors (ETA and ETB). Chronically elevated ET-1 is involved in the pathophysiology of pulmonary arterial hypertension, heart failure, systemic hypertension, renal dysfunction, and atherosclerosis (Haynes and Webb, 1998; Schneider et al., 2007). Elevated plasma levels of ET-1 can be found in several pathological states, including diabetes mellitus (De Mattia et al., 1998; Bruno et al., 2002). The discovery of several compounds acting as endothelin receptor antagonists has prompted research toward their use in clinical practice (Anand et al., 2004; Packer et al., 2005; McMurray et al., 2007).
Avosentan (SPP301) is a predominant ETA receptor antagonist which was in development for the treatment of diabetic nephropathy (Mann et al., 2010). Administration of SPP301 on top of standard care [including angiotensin-converting enzyme inhibitors (ACEIs) or high dose angiotensin receptor blockers (ARBs)] has been shown to result in a clinically relevant decrease in proteinuria in patients with diabetic nephropathy. In the Randomized, Double Blind, Placebo Controlled, Parallel Group Study to Assess the Effect of the Endothelin Receptor Antagonist Avosentan on Time to Doubling of Serum Creatinine, End Stage Renal Disease or Death in Patients With Type 2 Diabetes Mellitus, and Diabetic Nephropathy (ASCEND) study, 50% of patients with diabetic nephropathy and well-controlled blood pressure (BP) showed a 40–50% reduction in proteinuria with the addition of SPP301 to standard care (Mann et al., 2010). However, the ASCEND trial was stopped due to the adverse effects of SPP301. The most commonly reported adverse effects were consistent with those previously reported for the endothelin receptor antagonist class; namely edema, headache, and anemia (Mann et al., 2010). Edema appears to be dose-related and occurs more frequently with SPP301 than standard care alone. In patients with renal failure, SPP301 can aggravate existing edema.
ETA receptors are mainly localized in vascular smooth muscle, vasa recta, and arcuate arteries and glomerulus (mesangial cells and podocytes) and their stimulation or activation induce vasoconstriction, salt and water retention, vascular proliferation, inflammation, and fibrosis (Jandeleit-Dahm and Watson, 2012). There is now large evidence that ETA endothelin receptor blockade confers renoprotection in several progressive renal disorders (Davenport and Maguire, 2011; Gagliardini et al., 2011; Jandeleit-Dahm and Watson, 2012). Preclinical and clinical data demonstrates that of ETA receptor blockade is protective in chronic kidney disease through several effects including vasodilation, attenuation of proteinuria, increase of diuresis and natriuresis, inhibition of inflammation, oxidative stress, and fibrosis (Dhaun et al., 2011; Jandeleit-Dahm and Watson, 2012). ETB receptors are expressed in vascular and glomerular endothelial cells, mesangial cells, convoluted tubules and collecting duct epithelial cells, and their stimulation or activation cause vasodilation, increase sodium excretion, and inhibit vascular proliferation, inflammation, and fibrosis (Schneider et al., 2007). Recent data indicate that renal ETB receptor antagonism or knockout may cause sodium retention (Ge et al., 2006; Guo and Yang, 2006). Furthermore, ETB receptor deficiency is associated with renal injury and an impaired ability to excrete a sodium load (Ohkita et al., 2005). Thus, compounds with predominant selectivity against ETA receptors may produce fluid retention at concentrations where non-specific blockade of ETB receptors may occur.
The objectives of our study were to (1) test the hypothesis that the ETA receptor antagonist SPP301 at high concentrations may cause fluid retention, and (2) identify the concentration of SPP301 that inhibits ETB receptor-mediated effects and consequently leads to acute fluid retention in anesthetized rats. It is thought that SPP301 at high doses may antagonize the ETB receptors and induce fluid retention.
Materials and Methods
All procedures complied with guidelines from the American Physiological Society, and the study was approved by a local review board.
Rat Strains
Male Sprague-Dawley (SD) rats were obtained from Harlan, Indianapolis (12–14 weeks of age). Following acclimatization and a health examination, rats were housed in groups of three in standard cages with wire mesh tops and standardized softwood bedding, synchronized to a 12-h light–dark cycle, at ambient temperature 23 ± 2°C. A standard rat diet and tap water were supplied ad libitum.
Study Design: Effect of SPP301 on Renal Excretory Function
The purpose of the experimental protocols was to examine the acute effect of SPP301 on renal excretory function in a model of volume expansion/saline diuresis (Guo and Yang, 2006).
After induction of anesthesia with Inactin (80 mg/kg i.p. thiobutabarbital sodium, Sigma Chemical, St. Louis, MO, USA), SD rats were instrumented with catheters in: (1) the femoral artery for measurement of BP by a pressure transducer connected to a computerized data-acquisition system (PowerLab, ADInstruments, Colorado Springs, CO, USA; Campos et al., 2004); (2) the femoral vein for a constant infusion of artificial rat plasma and to administer study drugs via bolus infusion; and (3) the jugular vein for a constant infusion of 3H-inulin solution. A catheter was also inserted in the left ureter for urine collection. Artificial rat plasma [bovine serum albumin (BSA 2.5 g/100 mL, Sigma Chemical, St. Louis, MO, USA) + bovine immunoglobulins (2.5 g/100 mL, Sigma Chemical, St. Louis, MO, USA) in 0.9% NaCl] was infused (12.5 mL/kg/h for 45 min, thereafter 1.5 mL/kg/h) to maintain euvolemia throughout the experiment (Reckelhoff et al., 1992).
Rats were divided into two groups of six animals each (Group A and Group B). In both groups, Na2CO3 0.1 M 1 mL/kg was administered as a vehicle during the baseline period.
In Group A, the selective ETB receptor antagonist BQ-788 (Sigma Chemical, St. Louis, MO, USA) was administered alone as a 3-mg/kg dose by intravenous (i.v.) bolus in order to demonstrate its effects on ETB receptors compared with the vehicle, as described by Okada and Nishikibe(2002; Figure 1). Urine and blood samples were collected over 20-min clearance periods during the baseline period and 10 min after the i.v. bolus administration. The protocol was to administer vehicle, followed by BQ-788, then two more administrations of vehicle. The concentration of BQ-788 used was the highest concentration that could be obtained by dissolution in the same vehicle as SPP301. Since this concentration had the expected physiological effects during single administration, it was used as final positive control at the end of SPP301 administration experiments (Group B of study).
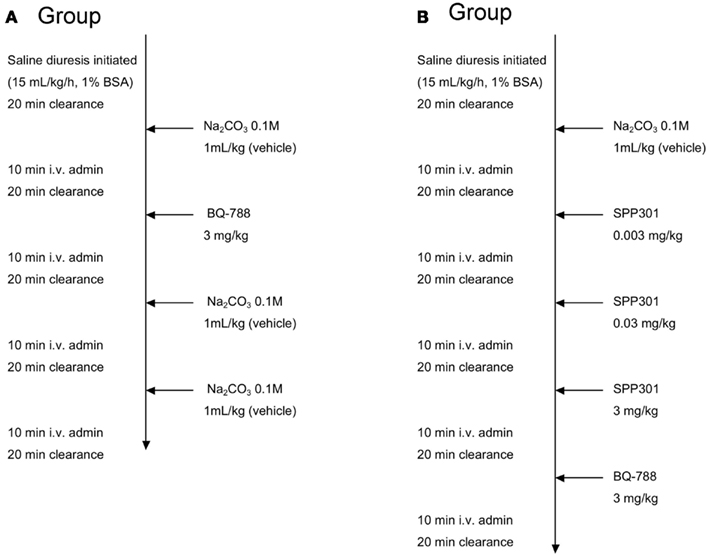
Figure 1. Study design. Group A study design: i.v. administration of BQ-788 (3 mg/kg) to anesthetized male SD rats undergoing saline diuresis. Group B study design: i.v. administration of incremental doses of SPP301 (0.003; 0.03; 3 mg/kg) followed by BQ-788 (3 mg/kg) to anesthetized male SD rats undergoing saline diuresis.
Group B received increasing concentrations of SPP301 (0.003; 0.03; 3 mg/kg, determined following completion of a dose-ranging study; Speedel, Basel, Switzerland) administrated as an i.v. bolus at 30-min intervals (Figure 1). The choice of bolus vs. infusion was justified by the relatively long half-life of the compound (4 h) compared to the length of our acute experimental protocol. It was also preferred in order to minimize the length of time required for loading and maintenance infusions. A 3-mg/kg dose of the selective ETB receptor antagonist BQ-788 was administered 30 min after the highest dose of SPP301 in order to test whether any additional effects of blocking ETB receptors would be observed (Okada and Nishikibe, 2002). Urine and blood samples were collected over a total of five 20-min clearance periods during the baseline period and 10 min after i.v. bolus administration of each study drug (Figure 1).
During each clearance period, urine was collected throughout the 20-min clearance period and an arterial blood sample (two hematocrit tubes – approximately 60 μL) was collected from the femoral artery catheter at the middle of the clearance period. Blood samples were centrifuged and the resulting plasma used for further analysis.
Plasma and Urine Electrolyte Measurement
At the end of the experiment, sodium and potassium concentration in the urine was determined for each clearance period. The rate of sodium and potassium excretion was calculated from these values and the volume of urine per 20 min as mEq/min. In addition, 3H-Inulin concentration in the plasma and urine samples was measured (using a scintillation counter) and used to calculate GFR. GFR for each clearance period was calculated as [Urine volume (mL)/min] × [Urine concentration (cpm/mL)]/[Plasma concentration (cpm/mL)] and expressed as mL/min.
Statistical Analysis
Statistical differences were determined with a one-way analysis of variance (ANOVA), followed by Tukey’s range test for dose-ascending studies using GraphPad software. p < 0.05 was considered to be statistically significant. Data are expressed as means ± SE.
Results
Selective Blockade of ETB Receptors with BQ-788 at Concentrations that Do Not Affect Glomerular Hemodynamics (GFR) Leads to Antidiuresis
In this study, BQ-788 decreased urine output (115.4 ± 15 vs. 150.1 ± 21 μL/min, p < 0.05) and fractional excretion of water (5 ± 0.3 vs. 7.7 ± 1%, p < 0.05) compared with the vehicle, while urinary excretion rates and plasma concentrations of sodium and potassium were not altered. BQ-788 increased BP by 6 ± 1 mmHg (p < 0.05), but did not affect GFR (2.2 ± 0.1 vs. 1.9 ± 0.1 mL/min, p = 0.2) compared with the vehicle. These alterations returned rapidly to normal values when vehicle was further administered, demonstrating that the effects of BQ-788 are not only reversible after acute administration but also provide a time-course effect of vehicle.
SPP301 Induces Antidiuresis at High Concentration
In this study, SPP301 decreased urine output and fractional excretion of water in a concentration-dependent manner (Figures 2A,B). Sodium excretion was initially increased but returned to control values after the highest dose of SPP301 (Figures 2C,D). GFR was unchanged (Figure 3) while mean arterial pressure (MAP) was reduced only by the highest dose (3 mg/kg) of SPP301 (Figure 4). Administration of BQ-788 (3 mg/kg) after SPP301 3 mg/kg did not further decrease urine output or water excretion (Figures 2A,B) and was without effect on GFR (Figure 3). The data indicates that after the highest concentration of SPP301, the effects persist beyond the 20-min clearance period, when BQ-788 does not have an additional effect. This indicates a full occupancy of ETB receptors by SPP301 before the BQ-788 administration. Hematocrit was significantly (p < 0.05) decreased by the higher doses of SPP301 (0.03; 3 mg/kg) and with BQ-788 (Figure 5). Repetitive administration of the vehicle did not cause the effects seen with SPP301 or BQ-788.
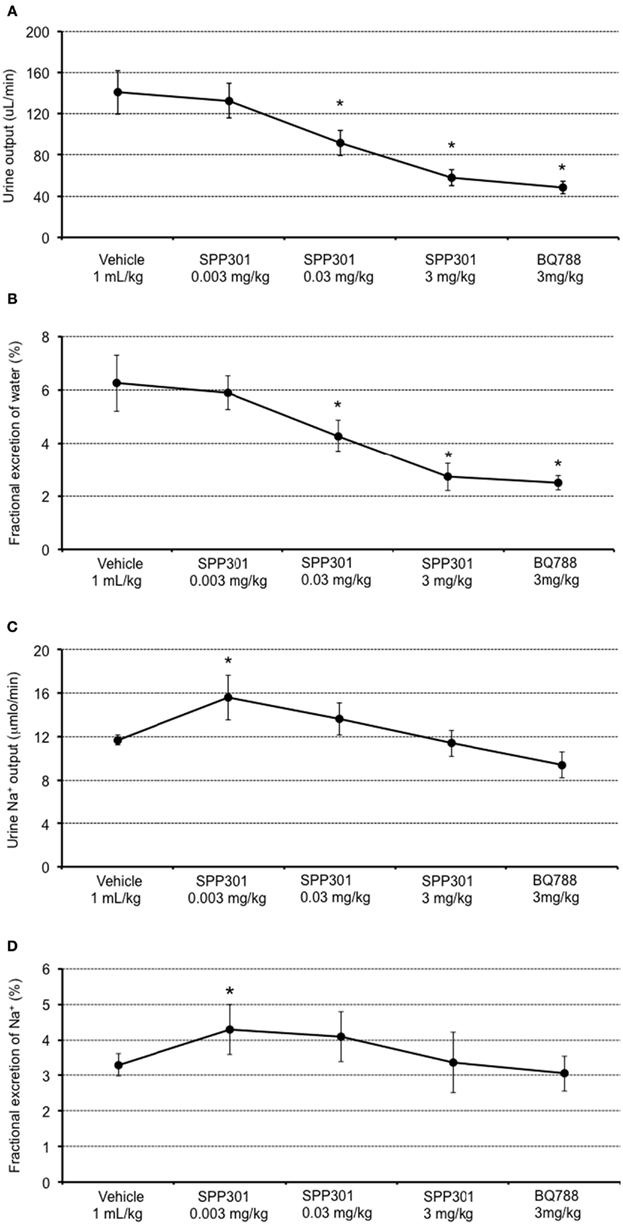
Figure 2. Urine and sodium excretion measured by: (A) urine output (μL/min); (B) fractional excretion of water (%); (C) urine sodium output (μmol/min); and (D) fractional excretion of sodium (%); in male SD rats treated with incremental doses of SPP301 (0.003; 0.03; 3 mg/kg) followed by BQ-788 (3 mg/kg). Fractional excretion of water (percentage of water excreted by the kidney from the total amount filtered) = urine output/GFR. Fractional excretion of sodium (percentage of Na+ excreted by the kidney from the total amount filtered) = urine Na+ output/[Plasma (Na+) × GFR]. *p < 0.05 compared with control (vehicle).
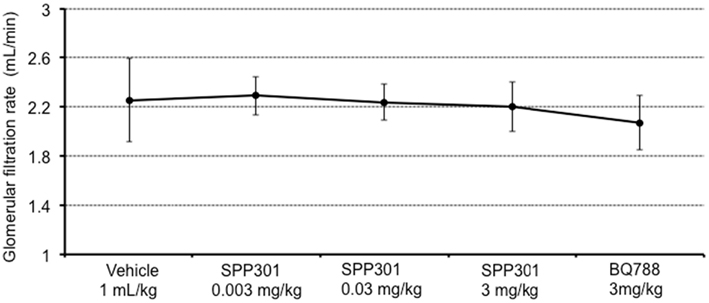
Figure 3. Glomerular filtration rate (mL/min) in male SD rats treated with incremental doses of SPP301 (0.003; 0.03; 3 mg/kg) followed by BQ-788 (3 mg/kg).
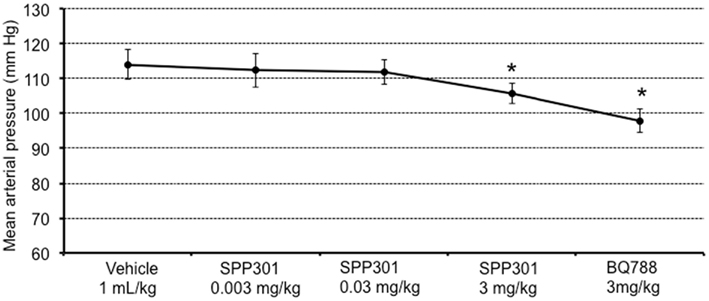
Figure 4. Mean arterial pressure (mm Hg) in male SD rats treated with incremental doses of SPP301 (0.003; 0.03; 3 mg/kg) followed by BQ-788 (3 mg/kg). *p < 0.05 compared with control (vehicle).
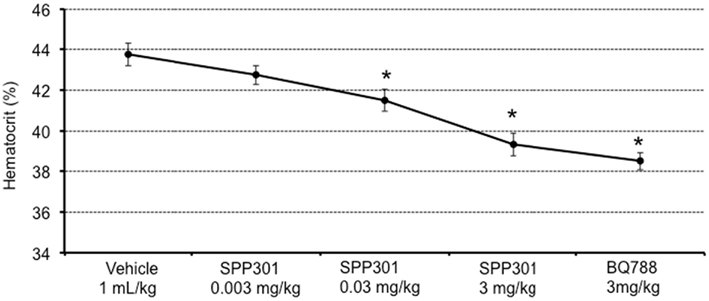
Figure 5. Hematocrit (%) in male SD rats treated with incremental doses of SPP301 (0.003; 0.03; 3 mg/kg) followed by BQ-788 (3 mg/kg). *p < 0.05 compared with control (vehicle).
Discussion
The present study demonstrates that SPP301 has antidiuretic effects at high doses and causes subsequent hemodilution (indicated by a decrease in hematocrit). These effects of SPP301 appear independent of changes in GFR or BP. Following administration of high dose (3 mg/kg) SPP301, ETB receptor blockade with BQ-788 did not cause further reductions in fractional water excretion in the presence of SPP301.
Endothelin is known to have an important role in the regulation of salt and water excretion by the kidney (Kohan et al., 2011). For example, endothelin inhibits the action of vasopressin in the isolated inner medullary collecting ducts (IMCD) of the kidneys, an effect mediated by ETB receptors (Nadler et al., 1992; Edwards et al., 1993). Medullary ETB receptor activation induces diuretic and natriuretic responses through a nitric oxide synthase 1 (NOS1) and cyclic guanylate monophosphate (cGMP) pathway (Nakano et al., 2008). Intramedullary infusion of an ETB antagonist has been shown to reduce urine flow rate to 68% at 30 min. Urinary sodium excretion starts to decline only after 20–30 min (Guo and Yang, 2006). Collecting duct-specific knockout of ET-1 increases urine concentrating effects of arginine vasopressin (AVP), while collecting duct-specific ETA receptor knockout decreases renal sensitivity to AVP (Ge et al., 2005). These findings suggest that ETB receptor activation in the collecting duct has diuretic effects and ETA receptor activation has antidiuretic effects. These effects are mediated through altered renal sensitivity to the urinary concentrating effects of AVP.
ETB receptor activation is thought to play an important role in the renal actions of some naturally occurring hormones. For example, relaxin has been localized in close proximity to the endothelin receptors in the systemic and renal vasculature as well as in the tubules of the kidney (Teichman et al., 2010). Relaxin binding to its receptor, RXFP1, results in activation of the ETB receptor on endothelial cells. Activation of the ETB receptor in the tubules of the kidney by relaxin may inhibit sodium/potassium ATPase, facilitating sodium and water secretion (Danielson et al., 1999; Dschietzig et al., 2003; Bogzil and Ashton, 2009). Accordingly, relaxin has been shown to increase clearance and urinary excretion of sodium in preclinical rat studies (Bogzil et al., 2005). Moreover, in the Pre-RELAX acute heart failure study, dyspnea relief correlated with BP reductions and increased weight loss in the relaxin treatment groups, supporting the hypothesis that relaxin may mediate natriuretic/diuretic effects (Teerlink et al., 2009).
Our results show that bolus i.v. administration of an ETB receptor antagonist (BQ-788) reduces urine output and fractional excretion of water within 30 min of administration, but does not significantly alter urine sodium output or fractional excretion of sodium. These data indicate that endogenous ET-1 has a tonic diuretic effect mediated via renal ETB receptors and may explain why fluid retention occurs during treatment with non-selective endothelin receptor blockers. Similarly, administration of a predominant ETA receptor antagonist (SPP301) dose-dependently reduced urine output, fractional excretion of water and hematocrit. Since this effect does not appear to be mediated through blockade of ETA receptors (which should promote diuresis), SPP301 may produce dose-dependent antidiuresis through non-specific blockade of ETB receptors. Moreover, since one of the actions ascribed to the ETB receptor is the clearance of ET-1 from circulation, its blockade may mediate an increased accessibility of ET-1 to ETA receptors.
In addition to the induction of fluid retention by renal mechanisms discussed here, it has been hypothesized that endothelin receptor antagonists induce edema through increased capillary permeability and consequent extravasation of fluids. Results of a related study in anesthetized bi-nephrectomized male Wistar rats show that SPP301 blunted a decrease in hematocrit in a concentration-dependent manner compared with a control, with a plateau at 1 μM (p < 0.05 for the trend). These observations suggest that SPP301 induces a concentration-dependent vascular leakage of fluid in this model (Maillard et al., 2008). Together, these findings help to elucidate the mechanisms by which the predominant ETA receptor antagonist SPP301 induces fluid retention.
High doses of SPP301 (25, 50 mg/day) have previously been reported to result in fluid overload and congestive heart failure in patients with diabetic nephropathy (Mann et al., 2010). In a follow-up explanatory mechanistic study in healthy subjects, a dose-dependent median reduction in the fractional renal excretion of sodium was found (up to 8.7% withSPP301 50 mg); this reduction was paralleled by a dose-related increase in proximal sodium reabsorption (Smolander et al., 2009). These clinical data together with our preclinical results suggest thatSPP301 may cause non-specific blockade of renal ETB receptors at high concentrations, thereby causing fluid retention.
The results of this study indicate that increasing concentrations of SPP301 may block ETB receptors in addition to ETA receptors and cause antidiuresis, which could explain the occurrence of fluid retention and the abolishment of benefits mediated by ETA receptor blockade observed in clinical trials (Smolander et al., 2009; Mann et al., 2010). Further studies are needed to clarify whether lower doses of ETA receptor blockers may be associated with fewer renal adverse effects in clinical practice. In addition, further studies may help to clarify the potential therapeutic value of ETB receptor activation.
ETA receptor antagonists show promise in trials for the treatment of chronic pathologies associated with tissue remodeling, including diabetic kidney disease (Wenzel et al., 2009; Saleh et al., 2011). However whilst demonstrating benefits, these drugs also have adverse effects on fluid retention, leading to an altered risk–benefit ratio and limiting their clinical value (Kohan et al., 2011). Indeed, efficacy and safety are the main issues responsible for the high attrition rate in phase III clinical drug development (van Gool et al., 2010). Studying the complex mechanisms of drug actions and corroborating them in an integrative physiological context are necessary for a valid translation of medical research into clinical practice. Our study represents a concrete example of translational research, demonstrating that drug development is a two-way street requiring efficient interactions between preclinical and clinical research, and between pharmaceutical and academic scientists. These data, corroborated with our colleagues’ data (Smolander et al., 2009), support further investigation of the antiproteinuric effect of SPP301 at adjusted doses.
Conflict of Interest Statement
Christoph Schumacher and Pat Louie are employed by Novartis Pharma AG, Basel, Switzerland. Radu Iliescu has received significant honoraria and Jane Reckelhoff has received a significant research grant from Speedel Holding AG, Basel, Switzerland. The remaining authors have no conflicts of interests.
Acknowledgments
We would like to thank Jennifer Pollard of ACUMED® for providing writing assistance. Source of Funding: this work was supported by Speedel Holding AG and the São Paulo Research Foundation [Grant 2011/50078-0].
References
Anand, I., McMurray, J., Cohn, J. N., Konstam, M. A., Notter, T., Quitzau, K., Ruschitzka, F., and Luscher, T. F. (2004). Long-term effects of darusentan on left-ventricular remodelling and clinical outcomes in the endothelin A Receptor Antagonist Trial in Heart Failure (EARTH): randomised, double-blind, placebo-controlled trial. Lancet 364, 347–354.
Bogzil, A. H., and Ashton, N. (2009). Relaxin-induced changes in renal function and RXFP1 receptor expression in the female rat. Ann. N. Y. Acad. Sci. 1160, 313–316.
Bogzil, A. H., Eardley, R., and Ashton, N. (2005). Relaxin-induced changes in renal sodium excretion in the anesthetized male rat. Am. J. Physiol. Regul. Integr. Comp. Physiol. 288, R322–R328.
Bruno, C. M., Meli, S., Marcinno, M., Ierna, D., Sciacca, C., and Neri, S. (2002). Plasma endothelin-1 levels and albumin excretion rate in normotensive, microalbuminuric type 2 diabetic patients. J. Biol. Regul. Homeost. Agents 16, 114–117.
Campos, L. A., Couto, A. S., Iliescu, R., Santos, J. A., Santos, R. A., Ganten, D., Campagnole-Santos, M. J., Bader, M., and Baltatu, O. (2004). Differential regulation of central vasopressin receptors in transgenic rats with low brain angiotensinogen. Regul. Pept. 119, 177–182.
Danielson, L. A., Sherwood, O. D., and Conrad, K. P. (1999). Relaxin is a potent renal vasodilator in conscious rats. J. Clin. Invest. 103, 525–533.
Davenport, A. P., and Maguire, J. J. (2011). Pharmacology of renal endothelin receptors. Contrib. Nephrol. 172, 1–17.
De Mattia, G., Cassone-Faldetta, M., Bellini, C., Bravi, M. C., Laurenti, O., Baldoncini, R., Santucci, A., and Ferri, C. (1998). Role of plasma and urinary endothelin-1 in early diabetic and hypertensive nephropathy. Am. J. Hypertens. 11, 983–988.
Dhaun, N., MacIntyre, I. M., Kerr, D., Melville, V., Johnston, N. R., Haughie, S., Goddard, J., and Webb, D. J. (2011). Selective endothelin-A receptor antagonism reduces proteinuria, blood pressure, and arterial stiffness in chronic proteinuric kidney disease. Hypertension 57, 772–779.
Dschietzig, T., Bartsch, C., Richter, C., Laule, M., Baumann, G., and Stangl, K. (2003). Relaxin, a pregnancy hormone, is a functional endothelin-1 antagonist: attenuation of endothelin-1-mediated vasoconstriction by stimulation of endothelin type-B receptor expression via ERK-1/2 and nuclear factor-kappaB. Circ. Res. 92, 32–40.
Edwards, R. M., Stack, E. J., Pullen, M., and Nambi, P. (1993). Endothelin inhibits vasopressin action in rat inner medullary collecting duct via the ETB receptor. J. Pharmacol. Exp. Ther. 267, 1028–1033.
Gagliardini, E., Buelli, S., and Benigni, A. (2011). Endothelin in chronic proteinuric kidney disease. Contrib. Nephrol. 172, 171–184.
Ge, Y., Bagnall, A., Stricklett, P. K., Strait, K., Webb, D. J., Kotelevtsev, Y., and Kohan, D. E. (2006). Collecting duct-specific knockout of the endothelin B receptor causes hypertension and sodium retention. Am. J. Physiol. Renal Physiol. 291, F1274–F1280.
Ge, Y., Stricklett, P. K., Hughes, A. K., Yanagisawa, M., and Kohan, D. E. (2005). Collecting duct-specific knockout of the endothelin A receptor alters renal vasopressin responsiveness, but not sodium excretion or blood pressure. Am. J. Physiol. Renal Physiol. 289, F692–F698.
Guo, X., and Yang, T. (2006). Endothelin B receptor antagonism in the rat renal medulla reduces urine flow rate and sodium excretion. Exp. Biol. Med. (Maywood) 231, 1001–1005.
Haynes, W. G., and Webb, D. J. (1998). Endothelin as a regulator of cardiovascular function in health and disease. J. Hypertens. 16, 1081–1098.
Jandeleit-Dahm, K. A., and Watson, A. M. (2012). The endothelin system and endothelin receptor antagonists. Curr. Opin. Nephrol. Hypertens. 21, 66–71.
Kohan, D. E., Rossi, N. F., Inscho, E. W., and Pollock, D. M. (2011). Regulation of blood pressure and salt homeostasis by endothelin. Physiol. Rev. 91, 1–77.
Maillard, M. P., Wang, Q., Baltatu, O. C., and Burnier, M. (2008). Do endothelin receptor antagonists induce edema through an extravasation of fluids? Evidence from an experiment in bi-nephrectomized rats. J. Hypertens. 26, S371.
Mann, J. F., Green, D., Jamerson, K., Ruilope, L. M., Kuranoff, S. J., Littke, T., and Viberti, G. (2010). Avosentan for overt diabetic nephropathy. J. Am. Soc. Nephrol. 21, 527–535.
McMurray, J. J., Teerlink, J. R., Cotter, G., Bourge, R. C., Cleland, J. G., Jondeau, G., Krum, H., Metra, M., O’Connor, C. M., Parker, J. D., Torre-Amione, G., van Veldhuisen, D. J., Lewsey, J., Frey, A., Rainisio, M., and Kobrin, I. (2007). Effects of tezosentan on symptoms and clinical outcomes in patients with acute heart failure: the VERITAS randomized controlled trials. JAMA 298, 2009–2019.
Nadler, S. P., Zimpelmann, J. A., and Hebert, R. L. (1992). Endothelin inhibits vasopressin-stimulated water permeability in rat terminal inner medullary collecting duct. J. Clin. Invest. 90, 1458–1466.
Nakano, D., Pollock, J. S., and Pollock, D. M. (2008). Renal medullary ETB receptors produce diuresis and natriuresis via NOS1. Am. J. Physiol. Renal Physiol. 294, F1205–F1211.
Ohkita, M., Wang, Y., Nguyen, N. D., Tsai, Y. H., Williams, S. C., Wiseman, R. C., Killen, P. D., Li, S., Yanagisawa, M., and Gariepy, C. E. (2005). Extrarenal ETB plays a significant role in controlling cardiovascular responses to high dietary sodium in rats. Hypertension 45, 940–946.
Okada, M., and Nishikibe, M. (2002). BQ-788, a selective endothelin ET(B) receptor antagonist. Cardiovasc. Drug Rev. 20, 53–66.
Packer, M., McMurray, J., Massie, B. M., Caspi, A., Charlon, V., Cohen-Solal, A., Kiowski, W., Kostuk, W., Krum, H., Levine, B., Rizzon, P., Soler, J., Swedberg, K., Anderson, S., and Demets, D. L. (2005). Clinical effects of endothelin receptor antagonism with bosentan in patients with severe chronic heart failure: results of a pilot study. J. Card. Fail. 11, 12–20.
Reckelhoff, J. F., Yokota, S. D., and Baylis, C. (1992). Renal autoregulation in midterm and late-pregnant rats. Am. J. Obstet. Gynecol. 166, 1546–1550.
Saleh, M. A., Pollock, J. S., and Pollock, D. M. (2011). Distinct actions of endothelin A-selective versus combined endothelin A/B receptor antagonists in early diabetic kidney disease. J. Pharmacol. Exp. Ther. 338, 263–270.
Schneider, M. P., Boesen, E. I., and Pollock, D. M. (2007). Contrasting actions of endothelin ET(A) and ET(B) receptors in cardiovascular disease. Annu. Rev. Pharmacol. Toxicol. 47, 731–759.
Smolander, J., Vogt, B., Maillard, M., Zweiacker, C., Littke, T., Hengelage, T., and Burnier, M. (2009). Dose-dependent acute and sustained renal effects of the endothelin receptor antagonist avosentan in healthy subjects. Clin. Pharmacol. Ther. 85, 628–634.
Teerlink, J. R., Metra, M., Felker, G. M., Ponikowski, P., Voors, A. A., Weatherley, B. D., Marmor, A., Katz, A., Grzybowski, J., Unemori, E., Teichman, S. L., and Cotter, G. (2009). Relaxin for the treatment of patients with acute heart failure (Pre-RELAX-AHF): a multicentre, randomised, placebo-controlled, parallel-group, dose-finding phase IIb study. Lancet 373, 1429–1439.
Teichman, S. L., Unemori, E., Teerlink, J. R., Cotter, G., and Metra, M. (2010). Relaxin: review of biology and potential role in treating heart failure. Curr. Heart Fail. Rep. 7, 75–82.
van Gool, A. J., Henry, B., and Sprengers, E. D. (2010). From biomarker strategies to biomarker activities and back. Drug Discov. Today 15, 121–126.
Wenzel, R. R., Littke, T., Kuranoff, S., Jurgens, C., Bruck, H., Ritz, E., Philipp, T., and Mitchell, A. (2009). Avosentan reduces albumin excretion in diabetics with macroalbuminuria. J. Am. Soc. Nephrol. 20, 655–664.
Keywords: endothelin receptor antagonist, fluid retention, diuresis, renal
Citation: Baltatu OC, Iliescu R, Zaugg CE, Reckelhoff JF, Louie P, Schumacher C and Campos LA (2012) Antidiuretic effects of the endothelin receptor antagonist avosentan. Front. Physio. 3:103. doi: 10.3389/fphys.2012.00103
Received: 06 March 2012; Paper pending published: 27 March 2012;
Accepted: 02 April 2012; Published online: 18 April 2012.
Edited by:
Teresa Malecka-Massalska, Medical University, PolandReviewed by:
Azeez Aileru, Winston-Salem State University, USASulayma Albarwani, Sultan Qaboos University, Oman
Abdu Adem, United Arab Emirates University, United Arab Emirates
Copyright: © 2012 Baltatu, Iliescu, Zaugg, Reckelhoff, Louie, Schumacher and Campos. This is an open-access article distributed under the terms of the Creative Commons Attribution Non Commercial License, which permits non-commercial use, distribution, and reproduction in other forums, provided the original authors and source are credited.
*Correspondence: Ovidiu Constantin Baltatu, University Camilo Castelo Branco (UNICASTELO), São José dos Campos Technology Park, Rod. Presidente Dutra Km 138, São José dos Campos, São Paulo 12247-004, Brazil. e-mail: ocbaltatu@yahoo.com