- 1ASTeC, United Kingdom Research and Innovation, Science and Technology Facilities Council (UKRI STFC) Daresbury Laboratory, Sci-Tech Daresbury, Warrington, United Kingdom
- 2Department of Physics and Astronomy, The University of Manchester, Manchester, United Kingdom
- 3The Cockcroft Institute, Sci-Tech Daresbury, Warrington, United Kingdom
The Compact Linear Accelerator for Research and Applications (CLARA) is a test facility at the STFC Daresbury Laboratory, which is capable of delivering ultra-bright electron bunches of up to 250 MeV beam energy. A new beam line has been designed to maximise the exploitation of the CLARA facility in a variety of scientific fields, including novel acceleration and new modalities of radiotherapy. In this paper, we present the specification and design of this beam line to explore the possibilities of pursuing R&D in very high-energy electron (VHEE) radiotherapy and VHEE with FLASH radiotherapy. We describe the beam line design, including the flexibility of the beam optics to focus the beam in a range of locations and present results from preliminary start-to-end simulations using Monte Carlo tracking codes. We highlight advantages of this beam line, including rapid access to the shielded experimental hutch and the possibilities for the installation of different experimental setups in two dedicated chambers. The beam line includes focussing magnets, extensive diagnostics, and allows in-air installation within the experimental beam line. The facility will allow an experimental programme towards addressing many outstanding issues related to this new radiotherapy modality. We also describe the available flexibility in beam parameters for both conventional dose rates and those entering into the FLASH regime. The possibility of both living cells and treatment planning studies is anticipated to be conducted at this world-class facility.
1 Introduction
The Compact Linear Accelerator for Research and Applications (CLARA) is an ultra-bright electron beam test facility at the STFC Daresbury Laboratory in the UK. The facility was originally created to test advanced free-electron laser (FEL) schemes that could be implemented on existing and future short-wavelength FEL facilities [1]. The facility is divided into three phases: Phase 1, front end of CLARA includes an S-band photoinjector, a 2-m-long S-band linac, a collimator, focussing and spectrometer magnets, and diagnostics. The front end, which produced 50-MeV, 250-pC electron bunches from a 10-Hz S-band photoinjector gun and linac, was successfully commissioned in 2018 [2]. Phase 2 includes additional accelerator modules that increase the beam energy to 250 MeV. The front end photoinjector gun has also been replaced with a novel 100-Hz high-repetition rate gun (HRRG) [3], which was previously commissioned on an adjacent beam line. The Phase 2 accelerator consists of a front end with the new high-repetition rate injector, three 4-m-long S-band (2,998.5 MHz) linacs, an X-band (11.994 GHz) fourth-harmonic cavity (4HC) phase-space lineariser, a variable magnetic bunch compressor (VBC), a dielectric dechirper, and a dedicated diagnostics line including a transverse deflecting cavity (TDC) for 6D phase-space characterisation. Focussing magnets and spectrometer beam lines are incorporated into the beam line for beam optics control and energy and energy spread measurements. Although Phase 3, which includes the installation of the FEL, has not yet been funded, the space has been reserved for possible future applications.
Access to the electron beam from the low-energy Phase 1 CLARA front end was made available from the beginning of 2018 to users from both academia and industry. This allowed for experiments to be carried out in a wide range of disciplines to test new concepts and ideas, such as the development of advanced accelerator technology [4], medicinal applications [5], and novel particle beam acceleration concepts [6–8]. For most experiments, a beam energy of 35 MeV with 100 pC charge was delivered, with beam energy of
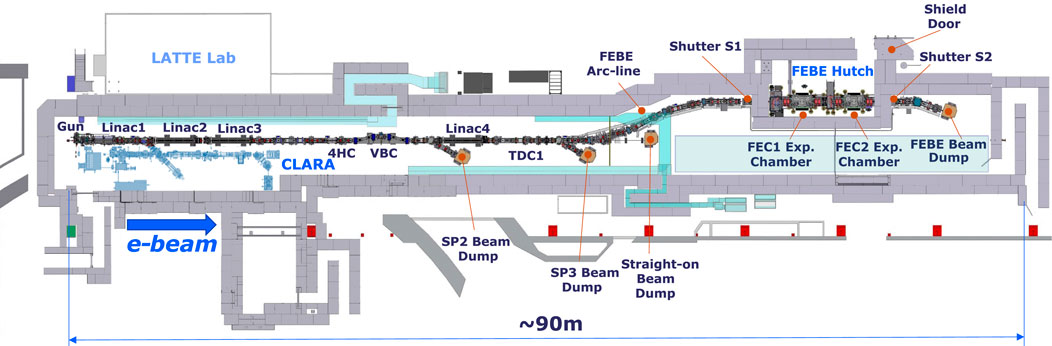
Figure 1. Schematic representation of the CLARA linear accelerator test facility, including the FEBE beam line, shielded FEBE hutch, and space reserved for potential future applications (shaded light blue area). The major components of the accelerator are highlighted, including the two shutters and the shield door used to allow rapid access to the hutch. The 100-TW laser system (not shown) is situated above the FEBE Hutch.
CLARA is one of only a few of test facilities worldwide that can provide user access to mid-energy range (less than 300 MeV), high-brightness electron beams to test proof-of-principle novel applications. A survey of beam dynamics challenges of such mid-energy high-brightness facilities in Europe was recently carried out and presented at IPAC’23 [9]. In addition to CLARA, there are three other comparable facilities in Europe: CLEAR@CERN [10, 11], ARES@DESY [12, 13], and SPARC_LAB@INFN [14].
In contrast to most other medium-energy facilities, the FEBE beam line is relatively unique in providing a dedicated shielded experimental hutch. This arrangement allows on-demand user access to the experimental area without fully switching off the accelerator, reducing disruption, improving machine stability, and allowing experiments to resume promptly after access periods. This is achieved by interlocking the FEBE dipoles to the accelerator personal safety system, allowing the continued operation of the RF systems and electron beam in the main CLARA accelerator while users have free access to the hutch. Upon completion of user access, the hutch is searched and locked, and the FEBE dipoles are re-energised to provide beam into the FEC chambers with minimal downtime and with minimal change to the electron beam properties from the CLARA accelerator. Such rapid experimental access is currently not possible at similar facilities in Europe, although CLEAR has developed robotic systems to reduce user access requirements during some types of experiments.
The detailed design of the FEBE beam line for the delivery of high-brightness beams for novel acceleration and other applications was recently published [15]. In this article, our focus is on the specification and design of the FEBE beam line and its suitability to explore VHEE and VHEE with FLASH in detail. The article is structured as follows: the layout of the machine and beam specification is presented in Section 2. Section 3 discusses previous studies on VHEE utilising the CLARA front end. Section 4 presents the results of beam dynamics simulations conducted from the CLARA photoinjector through the FEBE experiment chamber, where VHEE water phantom and other irradiation samples (such as plasmids and cells) will be installed. Section 5 details the dosage calculations performed using BDSIM/GEANT4 for different beam parameters at the predicted location of the experiment that will support future user exploitation. Section 6 describes future work. The article concludes with the current status of the facility in Section 7 and summary in Section 8.
2 Layout and beam specification
FEBE is designed to support a variety of experiments in the fields of accelerator applications and accelerator technology. A user survey performed in 2018 established a particular interest in R&D related to the new modality of VHEE and FLASH radiotherapy, as well as novel acceleration R&D. As a result, we separate the beam parameter specification provided for general FEBE operation from that for novel acceleration experiments, which frequently prioritises longitudinal peak-current and ultra-short bunch lengths. On the contrary, requirements for VHEE with bunch lengths in the multiple
A schematic representation of the beam line is shown in Figure 2. The FEBE layout is divided into three sections, namely, an arc and matching section connected to the main CLARA beam line; the FEBE experimental hutch, which brings the electron beam to a focus at two possible interaction points (IPs); and the post-hutch transport line and beam dump. The FEBE experimental hutch is a
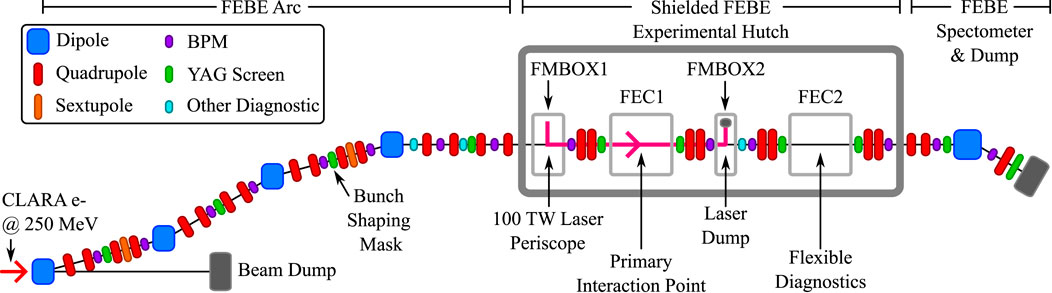
Figure 2. Schematic representation of the full energy beam exploitation (FEBE) beam line, including arc (connecting to the upstream CLARA main beam line, Figure 1), experiment hutch, and post-hutch beam dump with energy and emittance diagnostics.
The hutch is transversely offset from the main CLARA beam line, with a quadrupole FODO structure providing a horizontal
The beam transport within the hutch is notionally designed to deliver a strong focus to two possible IPs (IP1/2), each of which is located within a large-volume (
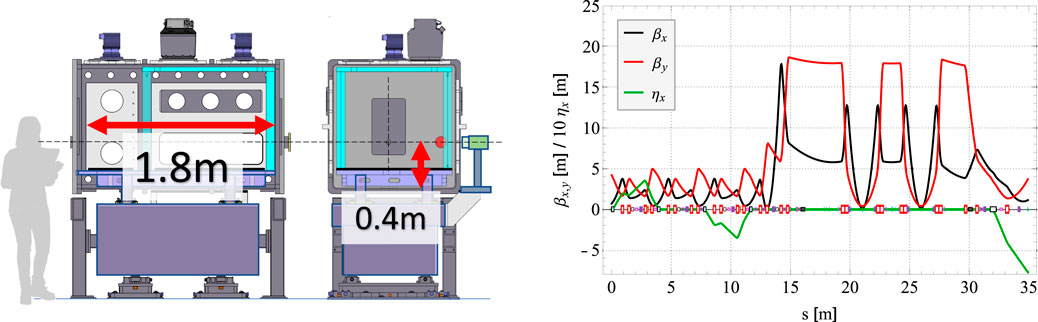
Figure 3. Left: schematic representation of the FEBE experimental chambers FEC [1/2]. The dotted line shows the electron beam height. Right: Twiss parameters for the FEBE beam line shown in Figure 2. The dotted lines indicate the locations of IP1/2 in FEC1/2, respectively.
VHEE experiments will be conducted in air. To enable this, a beryllium window will be installed at the entrance and exit of the relevant experimental chamber (FEC1 or FEC2). In previous CLARA front end VHEE experiments, a 500-
Access to the hutch with the accelerator running is made possible via an interlock of the FEBE arc dipoles to the machine personal safety system. Radiation shutters on either side of the enclosure (in the CLARA accelerator hall) are used to shield the hutch from the radiation generated from the main CLARA accelerator.
3 Previous studies on the CLARA front end
As noted, access to the 35-MeV, 100-pC electron beam from the CLARA front end was used for major experimental runs. Experimental setups were custom built at CLARA, and experiments at higher energies were carried out at CLEAR. During each of these experimental runs, VHEE irradiation experiments were performed in collaboration with the Manchester group, including studies on dosimetric inhomogeneities on beam dose profiles in water phantoms at various energies [17, 18], plasmid irradiation studies quantifying characteristics of VHEE relative biological effectiveness (RBE) [5, 19], and comparisons between radiotherapy modalities using DNA plasmids [20].
These experimental runs were vital for developing experience and the technical knowledge in running high-throughput irradiation experiments for both wet and dry VHEE biological experiments. A simple, yet robust and repeatable, system of stages and sample holders was developed to allow multiple samples to be irradiated sequentially with the whole system automated from the CLARA control room. Although relatively simple in comparison to other facilities’ advanced commercial robotic sample preparation systems, the rapid experimental access available at the CLARA front end and designed into the FEBE beam line mitigate some of these disadvantages. The system is also intrinsically flexible due to its simplicity and can accommodate a variety of irradiation targets.
4 Beam dynamics simulations
4.1 Start-to-end simulations on CLARA/FEBE
Particle tracking simulations were carried out using ASTRA [21] and ELEGANT [22], accounting for the non-linear effects (both longitudinal and transverse) of space charge and CSR. A Python-based framework (Simframe) developed at the STFC Daresbury Laboratory, which allows a single human-readable lattice file to be deployed consistently across several codes, was used throughout. ASTRA was used to simulate the CLARA injector at low energy (below 35 MeV), where transverse and longitudinal space charge forces are the dominant emittance-diluting processes. ELEGANT was used above 35 MeV due to its processing speed and the inclusion of CSR effects in the bunch compressor and FEBE arc.
The main optimisation actuators for the FEBE beam are found in the preceding CLARA beam line, as shown in Figure 1. The first 2-m S-band injector linac (Linac 1) can act as either a standard low-energy accelerating structure or a longitudinal bunching structure for a short single-spike operation. The remaining three 4-m-long S-band linacs (Linacs 2–4) provide acceleration up to a nominal beam energy of 250 MeV. A chicane-type VBC is located between Linac3 and Linac4, with X-band 4HC immediately before the VBC to compensate for longitudinal phase-space curvatures. The VBC is located at a nominal energy of
4.2 Start-to-end simulations for VHEE
Start-to-end simulations were performed to evaluate and optimise the electron beam properties at the FEC1 IP, although operation at FEC2 IP is similar. Simulations targeted expected requirements for VHEE operation as shown in Table 1: high charge (250 pC), high energy (250 MeV), long pulse length (
The machine settings for the optimised VHEE mode are set to provide a mild amount of compression, following the variable bunch compressor (∼2 ps RMS) with Linacs 1, 2, and 3 at moderate off-crest phases (+5°) and the 4HC cavity at the nominal phase of −180°. Linac4 is set to reduce the longitudinal chirp at −45°. These settings, including the required photoinjector laser pulse length for ∼2-ps bunch lengths, are compatible with machine requirements for other expected operation modes on CLARA. For ultra-compressed modes, we only need to modify the linac off-crest phases to increase the longitudinal compression in the VBC and FEBE arcs and vice versa for the VHEE operation.
The relaxed longitudinal emittance required for VHEE studies (as compared to other experiments planned on FEBE) reduces the impact of collective effects (such as CSR) in the bunch compressor and FEBE arc sections, allowing for significantly lower nominal transverse emittance at the FEBE IP (<1
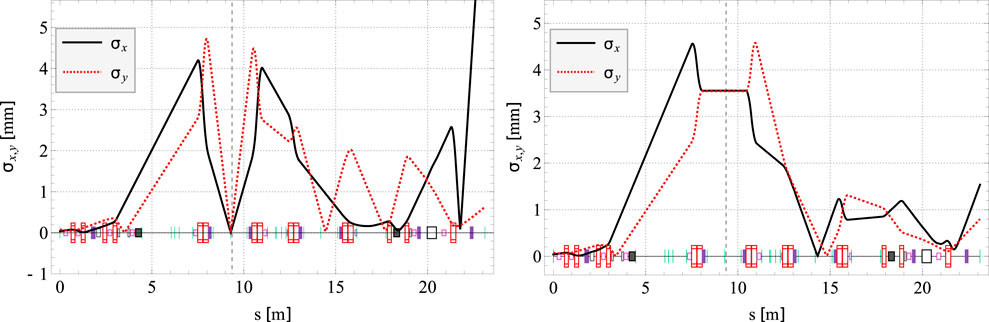
Figure 4. Left: transverse beam sizes for a focused beam at IP1 in FEC1. Right: transverse beam sizes for a pencil beam at IP1 in FEC1, both in vacuo.
Variation in the focus position is also possible within the chamber, providing a variable dose-depth profile and a spread-out-electron-peak (SOEP). The results for such a variation are shown in Figure 5 in vacuum, ignoring scattering from the beryllium window or for air or water. Focussing close to the chamber entrance is difficult, but variation in the focus position between 0.5 m and 1.5 m of the chamber shows a very flat distribution. The beam transverse angular divergence achievable for a flat SOEP, 250
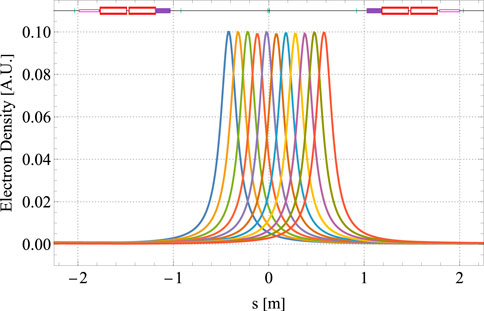
Figure 5. Variation in the electron focus position inside the FEC1 chamber shown using electron density plots. All results are in vacuo and do not include the effects of the beryllium window or scattering in air/water.
5 BDSIM/Geant4 dose calculations
The dose delivered to IP1/2 in FEBE was calculated using Beam Delivery Simulation (BDSIM) [23]. BDSIM is a Geant4-based code, which simulates the passage of particles through accelerator components. BDSIM has already extensively been used to simulate a wide range of facilities from proton beam therapy systems to high-energy particle physics colliders. Inside the vacuum of common accelerator components, such as quadrupoles, accelerator style tracking is used. As particles pass through matter and/or complex field regions Geant4 style tracking is employed. A BDSIM model of the FEBE beam line was created by converting the optical configurations, as shown in Figure 4. The physical (mass) geometry of the accelerator uses generic BDSIM accelerator components but with magnetic fields consistent with the accelerator optics. Electrons with the same phase space characteristics were tracked through the BDSIM magnetic fields, and beam sizes were computed after each element. The BDSIM-computed beam size agrees with that presented in Figure 4, confirming that the BDSIM model accurately represented the FEBE magnetic lattice.
The drift space around the FEC1 focus is replaced in the BDSIM model with a 500-
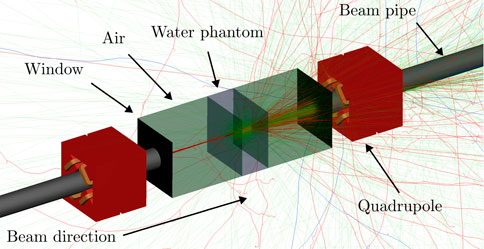
Figure 6. BDSIM/Geant4 visualisation region around the water phantom, including the quadrupoles before and after the experimental FEC1 chamber. Overlaid includes 100 primary electrons and the interaction products.
The Geant4 computed doses are shown in Figures 7, 8 for the focused and pencil electron optics, respectively. In both optics configurations, FLASH dose rates (>40 Gy/s) are achievable at FEBE. For a focused beam with a realistic material budget (window, air, and target), the maximum dose rate is 238.5 Gy/s and transversely Gaussian-distributed with a full width at half maximum of 3.9 mm. For the pencil beam with a realistic material budget (window, air, and target), the maximum dose rate is 102.6 Gy/s and transversely Gaussian-distributed with a full width at half maximum of 6.1 mm. The target-only dose curves shown in Figures 7, 8 represent the absolute maximum dose deliverable at FEBE. Reducing the air length by a factor of two and using a thinner 250-

Figure 7. Left: axial dose distribution for the focused electron beam distribution as a function of depth in the water phantom. The horizontal dashed line at 40 Gy/s indicates the nominal FLASH dose rate. Right: full width at half maximum of the focused electron beam dose distribution as a function of depth in the water phantom. The solid line is just for the water phantom target, the dashed line is for air and target, and the dot-dash line is for the window, air, and target.
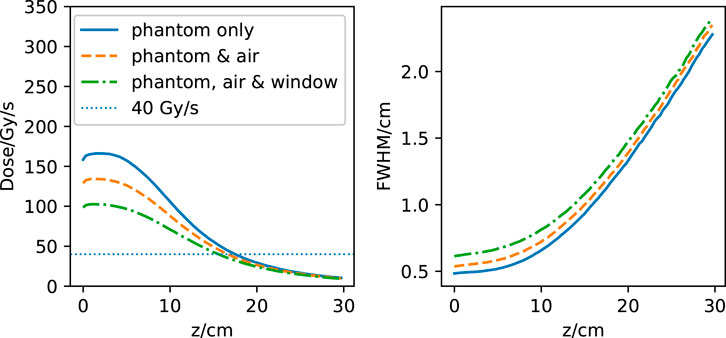
Figure 8. Left: axial dose distribution for the pencil electron beam distribution as a function of depth in the water phantom. The horizontal dashed line at 40 Gy/s, indicating the nominal FLASH dose rate. Right: full width at half maximum of the pencil electron beam dose distribution as a function of depth in the water phantom. The solid line is just for the water phantom target, the dashed line is for air and target, and the dot-dash line is for the window, air, and target.
Achieving a large dose uniformly over a large volume will either require a focus spot scanning system or a scattering system. Given the dose rates presented in this paper, a single or multiple scattering system at CLARA/FEBE is likely to reduce dose rates below those that are required for FLASH. Although the scanning system is technically possible, questions about the spatio-temporal dose rate distribution remain unexplored. From the perspective of accelerator beam delivery, both these questions can be studied using ELEGANT and BDSIM/Geant4 models of FEBE developed for this paper. The utility of VHEE FLASH via spot scanning also requires radiobiological experiments, which are likely to be performed at CLARA/FEBE.
6 Further work
The VHEE working group within the Cockcroft Institute at STFC Daresbury Laboratory is bringing together interested groups from universities and STFC to develop a long-term plan for VHEE studies on CLARA, as well as other relevant facilities. The primary focus for the near term is VHEE focussing on studies and their applicability for FLASH radiotherapy. Simulation work will prioritise improved focussing models and enhancements to enable more rapid optimisation of other potential operating modes for VHEE and FLASH operations. Experimental work on FEBE will prioritise electron focussing and spread-out electron peak (SOEP) research in water phantoms alongside tissue and cell biological studies, particularly those investigating previously unavailable FLASH modalities at CLARA. Exact details of conducting in-air VHEE experiments in FEBE experimental chambers will be worked out with the experimental groups in the near future.
7 Current status of the facility
As of this writing, CLARA installation in the accelerator hall is almost complete, and installation of the FEBE beam line into the hutch is expected to be completed by the end of 2024. RF conditioning of the gun and linacs is currently ongoing. It is expected that the RF conditioning of gun, linacs, 4HC, and TDC will be progressed sufficiently in the next few months for first beam threading, allowing for the completion of technical systems commissioning with the beam. This is followed by beam commissioning, characterisation, and setup to experimentally confirm beam parameters in the hutch; the installation and commissioning of the 100-TW laser transport in the hutch will then take place, with completion scheduled for mid-2025. An open call to the community is expected to be issued in early 2025 for beam time in Autumn 2025, depending on the results of beam commissioning.
8 Summary
A new beam line for full energy beam exploitation (FEBE) has been designed and is currently undergoing installation on the CLARA test facility at the STFC Daresbury Laboratory. The goal of this beam line is to support a wide variety of user-driven experiments utilising 250-MeV ultra-bright electron bunches of 250-pC bunch charge delivered at repetition rates of up to 100 Hz. The beam line incorporates two large-volume experiment chambers with a shielded user hutch for easy user access and flexibility when setting up of in-air VHEE and VHEE with FLASH experiments. Initial simulations of the viability of the beam line for VHEE studies have demonstrated the potential for FLASH dose rates, alongside the use of beam focussing and spread-out electron peak studies.
The planned studies at the CLARA/FEBE facility will further extend the work done on the CLARA front end at lower energy levels, pushing the facility into a new era of high-energy, high-charge FLASH modalities.
Data availability statement
The original contributions presented in the study are publicly available. This data can be found here: https://edata.stfc.ac.uk/handle/edata/962
Author contributions
DA-K: conceptualisation, investigation, methodology, writing–original draft, and writing–review and editing. SB: formal analysis, investigation, methodology, software, validation, and writing–review and editing. JJ: data curation, formal analysis, investigation, methodology, software, validation, and writing–review and editing.
Funding
The author(s) declare that financial support was received for the research, authorship, and/or publication of this article. This work was supported by the Science and Technology Facilities Council under the Cockcroft Institute core grant ST/V001612/1.
Acknowledgments
The authors would like to thank members of the CLARA team at Daresbury Laboratory for help and support with various aspects of the design, simulation, and experimental studies on CLARA front end. In particular, they would also like to thank Barry Fell and George Marshall from Technology Division for useful discussions and advice on in-air experiments in FEBE chambers and Roger Jones and Kristina Small from the University of Manchester and the Cockcroft Institute for useful discussions.
Conflict of interest
The authors declare that the research was conducted in the absence of any commercial or financial relationships that could be construed as a potential conflict of interest.
Publisher’s note
All claims expressed in this article are solely those of the authors and do not necessarily represent those of their affiliated organizations, or those of the publisher, the editors, and the reviewers. Any product that may be evaluated in this article, or claim that may be made by its manufacturer, is not guaranteed or endorsed by the publisher.
Footnotes
1A range cut is the minimum distance to consider physical processes in a Geant4 simulation. The distance range cut is converted to an energy minimum for each material used in the simulation. Particles which then fall below the energy are not then simulated further. Typically range cuts need to be smaller than the distances in a Geant4 simulation.
References
1. Clarke J, Angal-Kalinin D, Bliss N, Buckley R, Buckley S, Cash R, et al. Clara conceptual design report. J Instrumentation. (2014) 9:T05001. doi:10.1088/1748-0221/9/05/t05001
2. Angal-Kalinin D, Bainbridge A, Brynes A, Buckley R, Buckley S, Burt G, et al. Design, specifications, and first beam measurements of the compact linear accelerator for research and applications front end. Phys Rev Acc Beams (2020) 23:044801. doi:10.1103/physrevaccelbeams.23.044801
3. McKenzie J, Goudket P, Jones T, Militsyn B, Cowie L, Burt G, et al. High repetition rate s-band photoinjector design for the clara fel. In: Proceedings of FEL’14. Basel, Switzerland (2014).014801.
4. Pacey T, Saveliev Y, Healy A, Huggard P, Alderman B, Karataev P, et al. Continuously tunable narrow-band terahertz generation with a dielectric lined waveguide driven by short electron bunches. Phys Rev Acc Beams (2019) 22:091302. doi:10.1103/physrevaccelbeams.22.091302
5. Small K, Henthorn N, Angal-Kalinin D, Chadwick A, Santina E, Aitkenhead A, et al. Evaluating very high energy electron rbe from nanodosimetric pbr322 plasmid dna damage. Scientific Rep (2021) 11:3341. doi:10.1038/s41598-021-82772-6
6. Hibberd M, Healy AL, Lake DS, Georgiadis V, Smith EJH, Finlay OJ, et al. Acceleration of relativistic beams using laser-generated terahertz pulses. Nat Photon (2020) 14:755–9. doi:10.1038/s41566-020-0674-1
7. Saveliev YM, Pacey TH, Jones JK, Xia G. First dielectric wakefield experiments at daresbury laboratory. J Phys Conf Ser (2020) 1596:012015. doi:10.1088/1742-6596/1596/1/012015
8. Saveliev Y, Overton TJ, Pacey TH, Joshi N, Mathisen S, Muratori BD, et al. Experimental study of transverse effects in planar dielectric wakefield accelerating structures with elliptical beams. Phys Rev Accel Beams (2022) 25:081302. doi:10.1103/PhysRevAccelBeams.25.081302
9. Angal-Kalinin D, Jones JK, Pacey T, Corsini R, Burkart F, Pompili R, et al. Electron beam test facilities for novel applications. In: Proceedings of IPAC’23. Venice, Italy (2023).
10. CERN. CERN linear electron accelerator for research (2024). Available from: http://clear.cern/ (Accessed October 22, 2024).
11. Corsini R, Farabolini W, Malyzhenkov A, Dyks A, Korysko P, Sjobak K, et al. Status of the clear user facility at cern and its experiments. In: Proceedings of LINAC’22, liverpool, United Kingdom (JaCoW, Geneva, Switzerland) (2022).
12. DESY. Accelerator research experiment at SINBAD (2024). Available from: https://ares.desy.de/ (Accessed October 22, 2024).
13. Burkart F, Assmann R, Dinter H, Jaster-Merz S, Kuropka W, Mayet F, et al. The ares linac at desy. In: Proceedings of LINAC’22. Liverpool, United Kingdom (2022).
14. SPARC_LAB. Sources for plasma accelerators and radiation compton with laser and beam (2024). Available from: https://sparclab.lnf.infn.it/ (Accessed October 22, 2024).
15. Snedden EW, Angal-Kalinin D, Bainbridge AR, Brynes AD, Buckley SR, Dunning DJ, et al. Specification and design for full energy beam exploitation of the compact linear accelerator for research and applications. Phys Rev Accel Beams (2024) 27:041602. doi:10.1103/PhysRevAccelBeams.27.041602
16. Di Mitri S, Cornacchia M, Spampinati S. Cancellation of coherent synchrotron radiation kicks with optics balance. Phys Rev Lett (2013) 110:014801. doi:10.1103/PhysRevLett.110.014801
17. Lagzda A. VHEE radiatherapy studies at CLARA and CLEAR facilities. Manchester, UK: University of Manchetser (2019) PhD dissertation.
18. Lagzda A, Angal-Kalinin D, Jones J, Aitkenhead A, Kirkby KJ, MacKay R, et al. Influence of heterogeneous media on very high energy electron (vhee)dose penetration and a Monte Carlo-based comparison with existing radiotherapy modalities. Nucl Instr Methods Phys Res B (2020) 482:70–81. doi:10.1016/j.nimb.2020.09.008
19. Small K. Nanodosimetric properties of very high energy electrons through pBR322 plasmid DNA studies. Manchester, UK: University of Manchester (2020) PhD dissertation.
20. Wanstall HC, Henthorn NT, Jones J, Santina E, Chadwick AL, Angal-Kalinin D, et al. Quantification of damage to plasmid dna from 35 MeV electrons, 228 MeV protons and 300 kVp x-rays in varying hydroxyl radical scavenging environments. J Radiat Res (2023) 64:547–57. doi:10.1093/jrr/rrad032
22. Borland M. Elegant: a flexible sdds-compliant code for accelerator simulation. In: Proceedings of ICAP’00. Darmstadt, Germany: Darmstadt University of Technology (2000).
Keywords: ultra-bright electron source, beam energy, bunch charge, repetition rate, shielded hutch, very high-energy radiotherapy, flash dose rate
Citation: Angal-Kalinin D, Boogert S and Jones JK (2024) Potential of the CLARA test facility for VHEE radiotherapy research. Front. Phys. 12:1496850. doi: 10.3389/fphy.2024.1496850
Received: 15 September 2024; Accepted: 12 November 2024;
Published: 11 December 2024.
Edited by:
Luigi Palumbo, Sapienza University of Rome, ItalyReviewed by:
Jiaru Shi, Tsinghua University, ChinaMauro Migliorati, Sapienza University of Rome, Italy
Copyright © 2024 Angal-Kalinin, Boogert and Jones. This is an open-access article distributed under the terms of the Creative Commons Attribution License (CC BY). The use, distribution or reproduction in other forums is permitted, provided the original author(s) and the copyright owner(s) are credited and that the original publication in this journal is cited, in accordance with accepted academic practice. No use, distribution or reproduction is permitted which does not comply with these terms.
*Correspondence: Deepa Angal-Kalinin, ZGVlcGEuYW5nYWwta2FsaW5pbkBzdGZjLmFjLnVr