- Beijing Institute of Space Mechanics and Electricity, Beijing, China
We describe an efficient long-wave infrared optical parametric oscillator based on ZnGeP2 crystal pumped by a diode-pumped AO Q-switched Ho:YAG laser in this paper. The wavelength of the laser pulse generated by Ho:YAG laser is 2.09-μm, and the maximum output average power is 25.3 W at repetition rate of 10 kHz. When pumped by such Ho:YAG laser, the maximum average output power of 0.66 W at 9.8 μm is achieved, corresponding to slope efficiency of 5.0% and optical efficiency of 2.6%. Furthermore, the beam quality factor of the long-wave infrared beam is measured to be approximately 1.3. To the authors’ best knowledge, this is the best performance reported for a long-wave infrared ZGP-OPO pumped by a diode-pumped AO Q-switched Ho:YAG laser.
1 Introduction
The solid-state laser sources working in the long-wave infrared spectral region (8–12 μm) have important applications in many technical fields, such as environmental monitoring, chemical remote sensing, and national security etc. [1–3]. At present, the optical parametric oscillator (OPO) provides a good way for generating long-wave infrared laser radiation. In recent years, long-wave OPOs have been widely demonstrated with the crystals of AgGaSe2 (AGSe), BaGa4Se7 (BGSe), CdSe, OP-GaAs and ZnGeP2 (ZGP). A continuous-wave (CW) tunable OPO based on a whispering gallery resonator of AGSe was reported [4]. Under the pump wavelength of 1.57-μm, the output spectrum tunable range of the OPO from 2 μm to 8 µm was achieved. A widely tunable BGSe OPO was demonstrated by utilizing a Q-switched Nd:YLF laser as the pump source, enabling tuning capabilities ranging from 2.6 μm to 10.4 μm [5]. In a CdSe OPO pumped by a Tm:YLF-pumped Ho:YAG MOPA system at repletion rate of 1 kHz, the average output power of 1.03 W and 1.18 W were obtained at 10.15 μm and 11 μm respectively [6]. When pumped by a 1.95-μm pulsed thulium fiber laser, the long-wave infrared OP-GaAs OPO achieved an average output power of 0.8 W at 10.6 μm [7]. ZGP is an excellent nonlinear crystal for producing long-wave infrared laser radiation, as it possesses a unique combination of advantages, including a large nonlinear coefficient, high damage threshold, and excellent thermal conductivity. Due to its exceptional properties, the ZGP crystal has found extensive use in nonlinear optical frequency conversions for long-wave infrared generation. With optical parametric amplifier (OPA) method, average output power of 11.4 W at 8.3 μm was achieved by using ZGP crystal, corresponding to an optical efficiency of 9.8% under the pump power of 116.0 W [8]. The output power of 3.5 W at 9.8 μm was also reported in a ZGP OPO pumped by a 90-W Tm:YLF-pumped Ho:YAG laser [9]. It can be seen that, the Tm:YLF-pumped Ho:YAG laser is a good pump source for long-wave ZGP OPO. However, this system is complex due to the conversion way of diode-Tm-Ho. The high-power Tm laser also resulted in significant thermal loading, which affected the overall stability of the long-wave laser system. Diode -pumped Ho lasers exhibit notable characteristics of high efficiency, high power and simple structure [10–16], which are promising pump source for compact long-wave ZGP OPO systems. In this paper, we demonstrated a high power long-wave infrared ZGP OPO system at 9.8 μm based on type-I phase matching ZGP crystal pumped by a diode-pumped AO Q-switched Ho:YAG laser. To the best of our knowledge, such a long-wave infrared laser is reported for the first time. The maximum average output power of the laser pulse is 0.66 W, and the central wavelength is 9.8 μm with repetition rate of 10 kHz. The beam quality factor M2 is measured to be 1.3, with a corresponding optical efficiency of 2.6%.
2 Experimental setup
The experimental setup is schematically shown in Figure 1. A dual end-pumping AO Q-switched Ho:YAG laser was used as the pump source in this work in order to obtain high efficiency and high power of long-wave ZGP OPO. The maximum output power of the fiber-coupled laser diode (LD) (QPC, Ultra-500) is 40 W with wavelength of 1910 nm, and the core diameter and NA of the fiber are 0.6 mm and 0.22, respectively. The electrical-to-optical power efficiency of LD was about 10%. A 1:1 telescope was employed to focus the pump beam into laser crystal. Ho:YAG crystal with doped concentration of 1.0 at.% was cut to dimensions of 25 mm in length and 4 mm in diameter. The end-faces of the crystal were polished and coated with spectral range of 1.9–2.1 µm. The single-pass absorption of Ho:YAG was measured to be about 87%. In order to obtain better heat dissipation effect, the laser crystal was set in a copper heat-sink cooled by circulating water. The M1 mirror served as the input mirror, featuring high transmission for 1.9 µm and high reflectivity for 2.1 µm. The M2 was a dichroic mirror with high transmission for 1.9 µm, designed to operate as a polarizer with high transmission for p-polarized oscillating light and high reflective s-polarized oscillating light. The M3 mirror, acting as the output coupler, had a curvature of 500 mm and a transmittance of 50%. An air-cooled acousto-optic Q-switch (CETC, SGQ41-2000-1QC) with 20 W RF power at 41 MHz was placed between M2 and M3, and the loss modulation is more than 70%. The whole cavity length of Ho:YAG laser was about 90 mm.
After passing through a lens F2 with a focal length of 150 mm, the Ho laser beam was focused into the ZGP crystal, resulting in a pump diameter of 2.0 mm. The ring cavity, composed of three 30° plane mirrors, was used to achieve singly signal beam resonant. Two M4 mirrors were coated with high reflection for signal beam (∼2.6 μm) and high transmission for pump wavelength and idler beam (∼10.0 μm). Mirror M5 had high transmission for pump wavelength, high reflective for idler beam and a transmittance of approximately 30% for the signal beam. The physical length of OPO cavity was about 110 mm. The ZGP crystal, with dimensions of 5 mm × 5 mm in cross-section and 25 mm in length, was cut at an angle of θ = 51.5° for type-I phase matching. Both end faces of the ZGP crystal were coated with high transmission for the pump, signal, and idler wavelengths. Additionally, the ZGP crystal was set in a copper heat-sink cooled by circulating water.
3 Experimental results
For the purpose of measuring the output powers, a power meter (Thorlabs, S425C-L) was utilized in this work. The output powers curve of diode-pumped Ho:YAG laser was shown in Figure 3A. When the RF power was off, the maximum CW output power of 27.8 W was obtained with incident LD power of 80 W, corresponding to the slope efficiency of 37.9% and the optical-to-optical conversion efficiency of 34.8%. Within a period of 1 h, a power stability of Ho:YAG laser was measured to be about 2.3%. Compared with Ho:YAG lasers pumped by Tm-lasers, the efficiency of diode-pumped Ho:YAG laser is not high. The main reason of this phenomenon is overlap efficiency between pump and oscillating beams. Tm lasers have good beam quality (M2<3), while fiber-coupled LD has poor beam quality (∼100). This results in a relatively low overlap efficiency under fiber-coupled LD pumping. Smaller core diameter of pigtail fiber could be improve the efficiency of diode-pumped Ho:YAG laser.
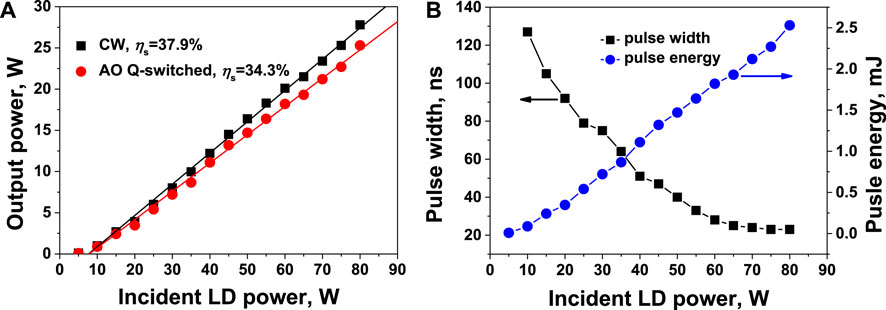
Figure 3. The output characteristics of diode-pumped Ho:YAG laser, (A) output powers (B) pulse width and pulse energy.
By turn on the RF power, the AO Q-switched Ho:YAG laser reached an average output power of 25.3 W, operating at repetition rate of 10 kHz under the incident LD power of 80 W. The slope efficiency and optical-to-optical conversion efficiency were 34.3% and 31.6%, respectively. A fast photodiode (EOT, ET-5000) and an oscilloscope (Tektronix, DPO 4000) were employed to measure the pulse width, as shown in Figure 3B. When the pump power was set as the maximum value, the shortest pulse width of 23 ns was achieved, leading to a calculating peak power of 110 kW.
The type-I ZGP crystal was employed to generate an idler beam at 9.8 μm, with a pump diameter of approximately 1.0 mm. The measured output power curves of long-wave IR ZGP OPO were shown in Figure 4A. At the maximum Ho pump power, an impressive signal power of 5.12 W was achieved, accompanied by a slope efficiency of 33.4%. Keep the pump power constant, an idler power of 0.66 W was also obtained, which corresponded to a slope efficiency of about 5.0%. Within a period of 1 h, a power stability of long-wave IR ZGP OPO was measured to be about 1.8%. The efficiency of long-wave ZGP OPO was Relatively low. This is mainly caused by the absorption at 9.8 μm of ZGP crystal.
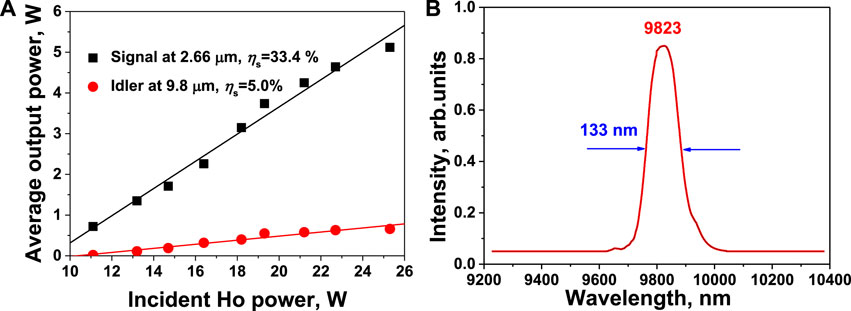
Figure 4. The output characteristics of long-wave IR ZGP OPO, (A) output powers (B) output spectrum.
The idler spectrum was recorded using a monochromator (WDG30A) and an HgCdTe detector (Vigo, PVM-10.6), which were connected to an oscilloscope. The recorded spectrum is depicted in Figure 4B. The central wavelength of the generated idler beam was approximately 9,823 nm, while the full width at half maximum was measured to be approximately 133 nm. In addition, the shortest pulse width of idler was measured to be about 21.3 ns The beam quality factor (M2) of idler beam at maximum output level was obtained by 90/10 knife-edge method. Figure 5 illustrates the spot radii at various positions along the beam propagation direction. By fitting the beam propagation equation to these data, the M2 factor of approximately 1.3 was estimated.
4 Conclusion
In summary, we have demonstrated an efficient long-wave infrared ZGP optical parametric oscillator. By using a dual end-pumping AO Q-switched Ho:YAG laser as the pump source, the maximum average output power of 0.66 W at 9.8 μm was obtained under the pump power of 25.3 W(in the Q-switching regimes). In addition, the Ho:LuAG laser also can operate in CW mode, corresponding to the output power of 27.8 W. The obtained slope efficiency and optical efficiency of the ZGP OPO were 5.0% and 2.6%, respectively. The M2 factor was measured to be approximately 1.3. These findings suggest that solid-state Ho lasers serve as a promising pump source for long-wave infrared OPO.
Data availability statement
The raw data supporting the conclusion of this article will be made available by the authors, without undue reservation.
Author contributions
HZ: Methodology, Writing–original draft, Writing–review and editing. YS: Data curation, Formal Analysis, Writing–original draft, Writing–review and editing. ZG: Investigation, Conceptualization, Writing–original draft, Writing–review and editing.
Funding
The author(s) declare financial support was received for the research, authorship, and/or publication of this article. This research was supported by the National Natural Science Foundation of China (NFSC, Grant No. 62175072), and Excellent youth foundation of CAST (2019).
Conflict of interest
The author declares that the research was conducted in the absence of any commercial or financial relationships that could be construed as a potential conflict of interest.
Publisher’s note
All claims expressed in this article are solely those of the authors and do not necessarily represent those of their affiliated organizations, or those of the publisher, the editors and the reviewers. Any product that may be evaluated in this article, or claim that may be made by its manufacturer, is not guaranteed or endorsed by the publisher.
References
1. Liu W, Ma Y, Liu X, Zhou J, Xu C, Dong B, et al. Larger-than-unity external optical field confinement enabled by metamaterial-assisted comb waveguide for ultrasensitive long-wave infrared gas spectroscopy. Nano Lett (2022) 22(15):6112–20. doi:10.1021/acs.nanolett.2c01198
2. Kulp TJ, Bisson SE, Bambha RP, Reichardt TA, Goers U-B, Aniolek KW, et al. The application of quasi-phase-matched parametric light sources to practical infrared chemical sensing systems. Appl Phys B (2002) 75:317–27. doi:10.1007/s00340-002-0978-5
3. Tidrow MZ, Dyer WR. Infrared sensors for ballistic missile defense. Infrared Phys Tech (2001) 42(3-5):333–6. doi:10.1016/s1350-4495(01)00092-5
4. Meisenheimer S-K, Urban Fürst J, Buse K, Breunig I. Continuous-wave optical parametric oscillation tunable up to an 8 μm wavelength. Optica (2017) 4(2):189–92. doi:10.1364/optica.4.000189
5. Kolker DB, Kostyukova NY, Boyko AA, Badikov VV, Badikov DV, Shadrintseva AG, et al. Widely tunable (2.6–10.4 μm) Baga4Se7 optical parametric oscillator pumped by a Q-switched Nd:YLiF4 laser. J Phys Commun (2018) 2(3):035039. doi:10.1088/2399-6528/aab007
6. Yang K, Li J, Gao Y, Wei D, Yao B, Wang J, et al. Watt-level long-wave infrared CdSe pulsed-nanosecond optical parametric oscillator. Opt Laser Tech (2022) 145:107491. doi:10.1016/j.optlastec.2021.107491
7. Wueppen J, Nyga S, Jungbluth B, Hoffmann D. 1.95 μm-pumped OP-GaAs optical parametric oscillator with 10.6 μm idler wavelength. Opt Lett (2016) 41(18):4225–8. doi:10.1364/ol.41.004225
8. Qian C, Duan X, Yao B, Shen Y, Zhang Y, Zhao B, et al. 11.4 W long-wave infrared source based on ZnGeP2 optical parametric amplifier. Opt Express (2018) 26(23):30195–201. doi:10.1364/oe.26.030195
9. Liu G-Y, Chen Y, Yao B-Q, Wang R-X, Yang K, Yang C, et al. 3.5 W long-wave infrared ZnGeP2 optical parametric oscillator at 9.8 µm. Opt Lett (2020) 45(8):2347–50. doi:10.1364/ol.389603
10. Jackson SD, Bugge F, Erbert G. Directly diode-pumped holmium fiber lasers. Opt Lett (2007) 32(17):2496–8. doi:10.1364/ol.32.002496
11. Venkatesan J, Xavier M, Cinta Pujol M, Carvajal JJ, Aguiló M, Díaz F, et al. Diode-pumped Ho-doped KLu(WO4)2Laser at 2.08 µm. Appl Phys Express (2011) 4(7):072601. doi:10.1143/apex.4.072601
12. Newburgh GA, Word-Daniels A, Michael A, Merkle LD, Ikesue A, Dubinskii M. Resonantly diode-pumped Ho^3+:Y_2O_3 ceramic 21 µm laser. Opt Express (2011) 19(4):3604–11. doi:10.1364/oe.19.003604
13. Lamrini S, Koopmann P, Schäfer M, Scholle K, Peter F. Directly diode-pumped high-energy Ho:YAG oscillator. Opt Lett (2012) 37(4):515–7. doi:10.1364/ol.37.000515
14. Zhang W, Gao Q, Zhou S, Li L, Ma X. Diode-pumped acousto-optical Q-switched Ho:GdTaO4 laser at 2.07 μm. Opt Laser Tech (2021) 144:107368. doi:10.1016/j.optlastec.2021.107368
15. Duan X, Wu J, Ding Y, Yang X, Dai T. Electro-optically Q-switching of dual-diode-pumped Ho-doped lutetium vanadate laser. Opt Laser Tech (2023) 158:108929. doi:10.1016/j.optlastec.2022.108929
Keywords: long-wave infrare, optical parametric oscillator, Ho:YAG, diode-pumped lasers, AO Q-switched
Citation: Hou Z, Yang S and Zheng G (2024) Efficient long-wave infrared ZGP optical parametric oscillator pumped by a diode-pumped AO Q-switched Ho:YAG laser. Front. Phys. 12:1374090. doi: 10.3389/fphy.2024.1374090
Received: 21 January 2024; Accepted: 22 February 2024;
Published: 21 March 2024.
Edited by:
Xiaoming Duan, Harbin Institute of Technology, ChinaReviewed by:
Preeti Gupta, Leibniz Institute for Solid State and Materials Research Dresden (IFW Dresden), GermanyChuanpeng Qian, Chinese Academy of Sciences (CAS), China
Quan Sheng, Tianjin University, China
Copyright © 2024 Hou, Yang and Zheng. This is an open-access article distributed under the terms of the Creative Commons Attribution License (CC BY). The use, distribution or reproduction in other forums is permitted, provided the original author(s) and the copyright owner(s) are credited and that the original publication in this journal is cited, in accordance with accepted academic practice. No use, distribution or reproduction is permitted which does not comply with these terms.
*Correspondence: Zuoxun Hou, aHp4XzAwN3hqdHVAMTYzLmNvbQ==