- 1College of Physics and Electronics Engineering, Shanxi University, Taiyuan, Shanxi, China
- 2Collaborative Innovation Center of Extreme Optics, Shanxi University, Taiyuan, Shanxi, China
Properties of the particle distribution in high energy heavy-ion collisions are important for understanding the particle production. In Tsallis statistics with a multisource production, we study the transverse momentum spectra of
1 Introduction
The transverse momentum
Particle distribution is a significant value observed in the LHC experiment. Many phenomenological models were proposed to discuss the abundant experimental data. However, it is very difficult to uniformly describe the whole properties of particle distribution and to analyze the whole process of matter evolution in relativistic heavy-ion collisions by using only one method. In recent years, some different methods were combined with each other in order to figure out the multiparticle production in heavy-ion collisions at high energies. Recently, different statistic-based models were proposed to understand the transverse momentum
Heavy-flavor quarks are mostly produced in the initial stage of collisions in hard scattering processes between nucleus partons. Heavy-flavor quarks can undergo the whole evolution process of QGP created in ultra-relativistic heavy-ion collisions. Therefore, heavy-flavor mesons carry the substantial information of the hot-dense QCD medium and hadron production and are recognized as important probes of the QGP. It is crucial to study the interaction between heavy-flavor quarks and the strongly interacting medium by the differential production yield, the nuclear modification factor, and the anisotropic collective flow of heavy-flavor mesons. Thermodynamic properties were obtained via the comparison of theoretical models with transverse momentum spectra pT of heavy-flavor mesons measured in collisions. The nuclear modification factor RAA is a key observable, allowing us to discuss the mechanisms of the particle production in proton–proton collisions and heavy-ion collisions at high energies and understand the effects of energy loss and the dynamics of the heavy-quark hadronization. In the investigation of the transverse momentum pT spectra of heavy-flavor mesons, some parameters required in the model calculation of the nuclear modification factor RAA may be extracted synchronously.
The transverse momentum spectra of final-state particles can give the significant information of the produced matter in high-energy collisions. In our previous work [29], the temperature parameters of particle-emission sources were determined qualitatively in the geometrical manner of the multisource thermal model, and thermodynamic properties of these emission sources were determined from the central axis to the side-surface of the source cylinder. In this paper, we will investigate the transverse momentum distributions of prompt
2 Particle distribution in Tsallis statistics
In the multisource thermal model [29], the projectile and target cylinders were supposed to be formed in nucleus–nucleus collisions at high energy. In the rapidity space, the projectile cylinder and the target cylinder lie in the rapidity range [-Y, Y]. The final-state particles are produced from different emission sources in the cylinders. Final-state particles emit anisotropically from these emission sources in different longitudinal locations. On the other hand, the projectile and target cylinder are thought to be composed of a series of emission sources with different rapidity shifts. The model is commonly known as a multisource thermal model. The simple model can only describe transverse momentum spectra of particles and can only identify the qualitative temperature parameters of emission sources by transverse momentum spectra. The limitation of the model is very difficult to avoid. In this work, the multisource production will be considered in Tsallis statistics, which is a thermodynamic formalism of describing the fractal structure of Yang–Mills fields [31, 32]. In addition, the relaxation time approximation of the collision term in the Boltzmann transport equation will be introduced into the model in order to describe the
In the calculation, we consider a thermodynamically consistent form of the Tsallis distribution, which was described in detail in Refs [5, 33]. From the Tsallis distribution, the correlative thermodynamic quantities can be extracted. According to Tsallis statistics, the particle number is given by
where
When the nonextensivity parameter q tends to 1, the distribution function is the Boltzmann distribution, given by
Considering the multisource emission [29, 30], the transverse momentum distribution of initial-state particles can be written as
In the calculation, Eq. 4 is regarded as an initial distribution of the Boltzmann transport equation. By the relaxation time approximation of the collision term, the Boltzmann transport equation is solved in order to obtain a distribution of final-state particles.
The nuclear modification factor
where
An evolution of the particle distribution
where
By considering the relaxation time approximation, the collision term
where
where
By using Eq. 5, the nuclear modification factor [6] is given by
3 Comparison and discussion
Figure 1 shows the transverse momentum spectra of prompt
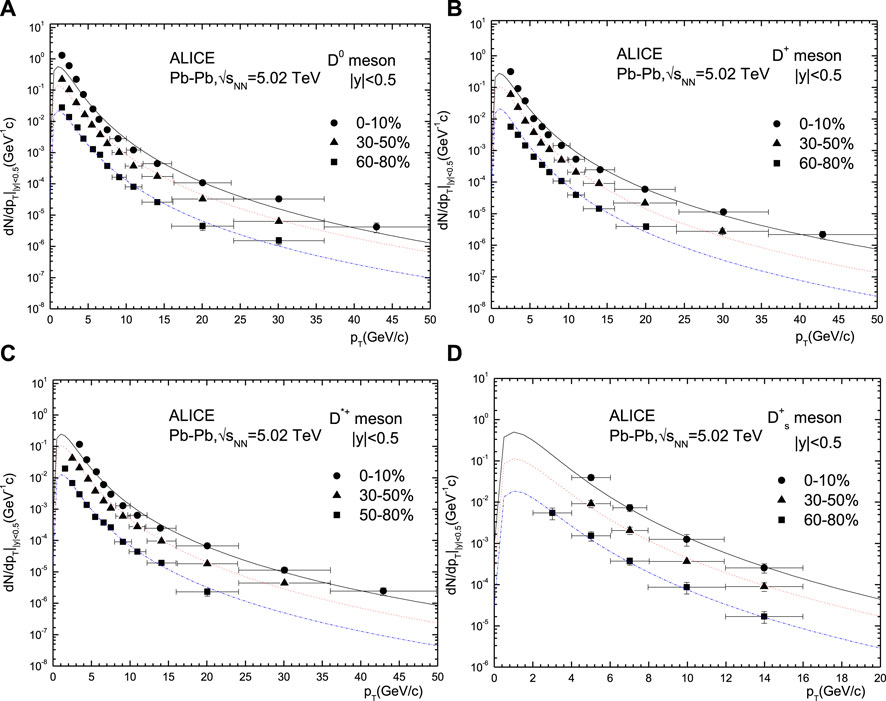
FIGURE 1. Transverse momentum distributions of prompt
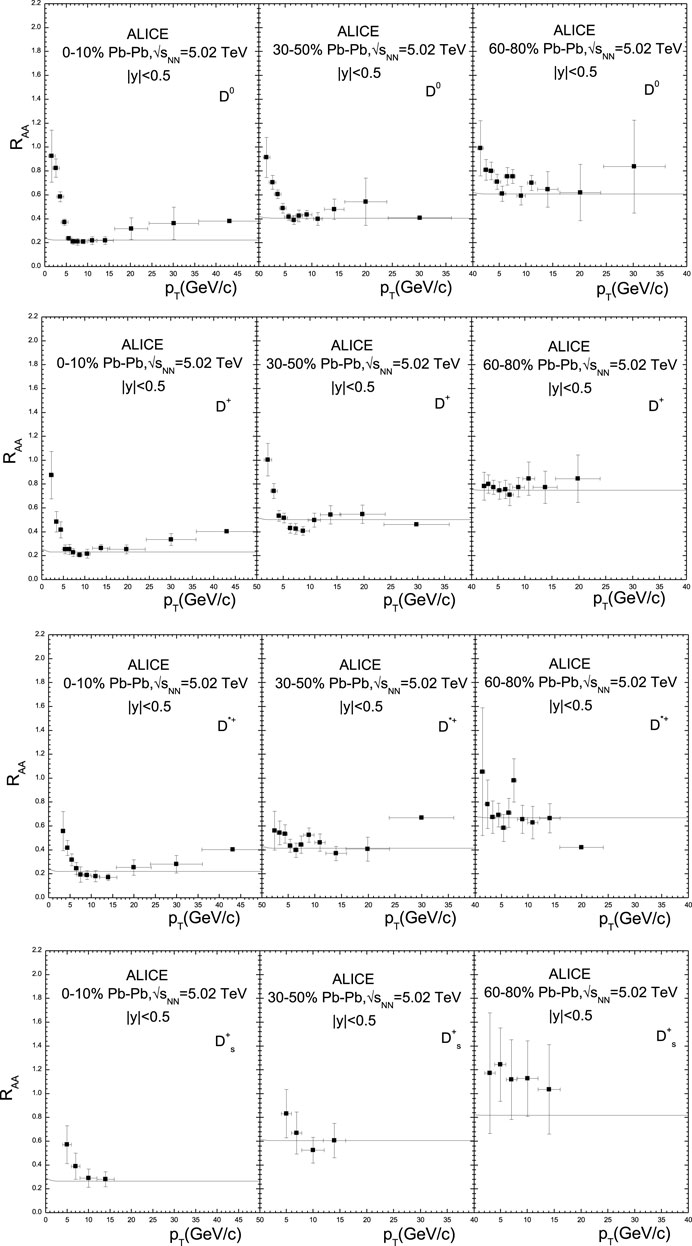
FIGURE 2. Nuclear modification factor
Figure 3 shows the transverse momentum spectra of the prompt
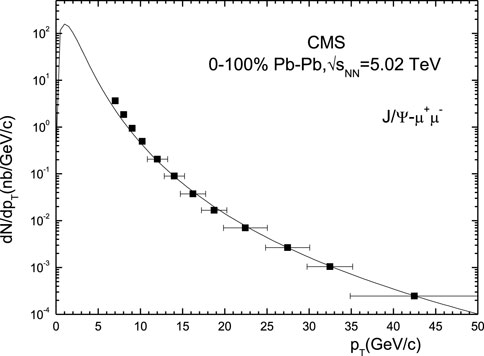
FIGURE 3. Differential cross section of the prompt
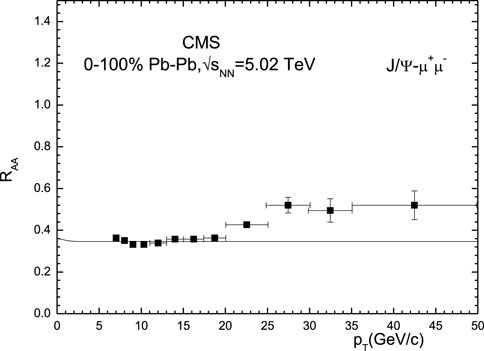
FIGURE 4. Nuclear modification factor
To further test the capacity of the model, we analyze other particles at a higher energy. Figure 5 shows the transverse momentum spectra of charged particles for 0%–5%, 5%–10%, 10%–30%, 30%–50%, 50%–70%, and 70%–90% centrality classes in Pb–Pb collisions at
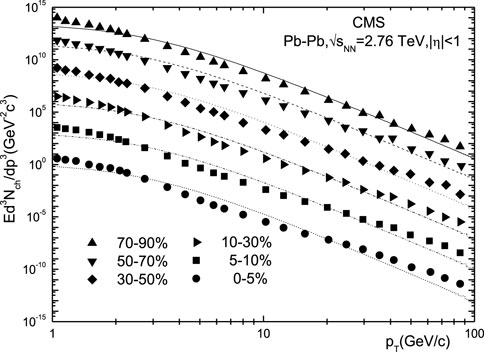
FIGURE 5. Transverse momentum distributions of charged particles in the 70%–90%, 50%–70%, 30%–50%, 10%–30%, 5%–10%, and 0%–5% centrality classes in Pb–Pb collisions at
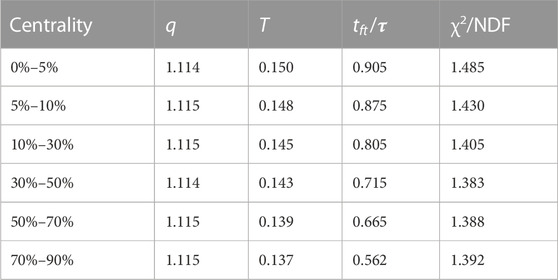
TABLE 2. Fitted values of
By analyzing the results, it is revealed that the improved model can explain the transverse momentum
4 Conclusion
In our previous works [29, 30], the multisource production of final-state particles in high-energy nuclear collisions was proposed in several versions, which can be applied to study the transverse momentum distributions, elliptic flows, and so on. Final-state particles emit from different emission sources in the model, which can only identify the qualitative temperature parameters of emission sources. In recent years, Tsallis statistics is widely used in the investigation of particle distribution in high-energy collisions. In this paper, we combine Tsallis statistics with the multisource model. Moreover, the relaxation time approximation of the collision term in the Boltzmann transport equation is applied in the improved model. We study the transverse momentum spectra for different centrality classes in Pb–Pb collisions at
The temperature
In the multisource thermal model, final-state particles emit from different emission sources, which are expected to be formed in collisions. The model is still in development. The present work will further be improved in the framework of multisource production. The interaction of emission sources is related to the hot dense matter in the sources and also results in the azimuthally anisotropic expansion in the momentum space. The momentum asymmetry will be used to describe the elliptic flows of particles produced in ultra-relativistic heavy-ion collisions. Considering different rapidity shifts of anisotropic emission sources, the particle distribution in the rapidity space can be discussed. In the future, more properties of the multiparticle system will be found in the model and some thermodynamic quantities (such as the heat capacity, speed of sound, and conformal symmetry breaking measure) can be calculated.
Altogether, this work is a new attempt to study the properties of particle distribution using the improved method.
Data availability statement
The original contributions presented in the study are included in the article/Supplementary Material; further inquiries can be directed to the corresponding author.
Author contributions
Y-FG: formal analysis, investigation, and writing–original draft. B-CL: methodology, project administration, writing–original draft, and writing–review and editing.
Funding
The authors declare that the financial support was received for the research, authorship, and/or publication of this article. This work was supported by the National Natural Science Foundation of China under Grant Nos 12147215, 12047571, and 11575103, the Shanxi Provincial Natural Science Foundation under Grant No. 202103021224036, the Scientific and Technological Innovation Programs of Higher Education Institutions in Shanxi (STIP) under Grant No. 201802017, and the Fund for Shanxi “1331 Project” Key Subjects Construction
Conflict of interest
The authors declare that the research was conducted in the absence of any commercial or financial relationships that could be construed as a potential conflict of interest.
Publisher’s note
All claims expressed in this article are solely those of the authors and do not necessarily represent those of their affiliated organizations, or those of the publisher, the editors, and the reviewers. Any product that may be evaluated in this article, or claim that may be made by its manufacturer, is not guaranteed or endorsed by the publisher.
References
1. Adams J, Adler C, Aggarwal MM, Ahammed Z, Amonett J, Anderson BD, et al. Transverse momentum and collision energy dependence of high pT hadron suppression in Au+Au collisions at ultrarelativistic energies. Phys Rev Lett (2003) 91:172302. doi:10.1103/PhysRevLett.91.172302
2. Adam J, Adamczyk L, Adams JR, Adkins JK, Agakishiev G, Aggarwal MM, et al. Strange hadron production in Au+Au collisions at sNN = 7.7, 11.5, 19.6, 27, and 39 GeV. Phys Rev C (2020) 102:034909. doi:10.1103/PhysRevC.102.034909
3. Abelev B, Adam J, Adamova D, Adare AM, Aggarwal MM, Rinella GA, et al. Pion, kaon, and proton production in central Pb-Pb collisions at sNN =2.76 TeV. Phys Rev Lett (2012) 109:252301. doi:10.1103/PhysRevLett.109.252301
4. Acharya S, Adamova D, Adler A, Adolfsson J, Aggarwal MM, Rinella GA, et al. Centrality and transverse momentum dependence of inclusive J/ψ production at midrapidity in Pb-Pb collisions at sNN =5.02 TeV. Phys Lett B (2020) 805:135434. doi:10.1016/j.physletb.2020.135434
5. Cleymans J, Worku D. The Tsallis distribution in proton–proton collisions at s = 0.9 TeV at the LHC. J Phys G (2012) 39:025006. doi:10.1088/0954-3899/39/2/025006
6. Tripathy S, Bhattacharyya T, Garg P, Kumar P, Sahoo R, Cleymans J. Nuclear modification factor using Tsallis non-extensive statistics. The European Physical Journal A (2016) 52:289. doi:10.1140/epja/i2016-16289-4
7. Braun-Munzinger P, Magestro D, Redlich K, Stachel J. Hadron production in Au - Au collisions at RHIC. Phys Lett B (2001) 518:41–6. doi:10.1016/s0370-2693(01)01069-3
8. Wolschin G. Beyond the thermal model in relativistic heavy-ion collisions. Phys Rev C (2016) 94:024911. doi:10.1103/physrevc.94.024911
9. Rafelski J, Letessier J. Testing limits of statistical hadronization. Nucl Phys A (2003) 715:98–107c. doi:10.1016/s0375-9474(02)01418-5
10. Kapusta JI. Perspective on Tsallis statistics for nuclear and particle physics. Int J Mod Phys E (2021) 30:2130006. doi:10.1142/s021830132130006x
11. Srivastava PK, Singh A, Chaturvedi OSK, Raina PK, Singh BK. Transverse momentum distribution of charged hadrons based on wounded quark model. Eur Phys J A (2019) 55:69. doi:10.1140/epja/i2019-12741-3
12. Gupta R, Jena S. Model comparison of the transverse momentum spectra of charged hadrons produced in PbPb collision at sNN =5.02 TeV. Adv High Energ Phys. (2022) 2022:5482034. doi:10.1155/2022/5482034
13. Liu FH, Abd Allah NN, Singh BK. Dependence of black fragment azimuthal and projected angular distributions on polar angle in silicon-emulsion collisions at 4.5A-GeV/C. Phys Rev C (2004) 69:057601. doi:10.1103/physrevc.69.057601
14. Shao GC, Li HL. Rayleigh-like distribution of particle transverse momenta in collisions at high energies. Chin Phys. C (2004) 34:964. doi:10.1088/1674-1137/34/7/007
15. He XW, Wu FM, Wei HR, Hong BH. Energy dependent chemical potentials of light hadrons and quarks based on transverse momentum spectra and yield ratios of negative to positive particles. Adv High Energ Phys. (2020) 2020:1–19. doi:10.1155/2020/1265090
16. Tsallis C. Possible generalization of Boltzmann-Gibbs statistics. J Stat Phys (1988) 52:479–87. doi:10.1007/bf01016429
17. Bediaga I, Curado EMF, de Miranda JM. A Nonextensive thermodynamical equilibrium approach in e+ e- ---> hadrons. Physica A (2000) 286:156–63. doi:10.1016/s0378-4371(00)00368-x
18. Abelev BI, Adams J, Aggarwal MM, Ahammed Z, Amonett J, Anderson BD, et al. Strange particle production in p+p collisions sNN = 200 GeV. Phys Rev C (2007) 75(064901). doi:10.1103/PhysRevC.75.064901
19. Adare A, Afanasiev S, Aidala C, Ajitanand NN, Akiba Y, AlBataineh H, et al. Measurement of neutral mesons in p+p collisions at sNN = 200 GeV and scaling properties of hadron production. Phys Rev D (2011) 83:052004. doi:10.1103/PhysRevD.83.052004
20. Aamodt K, Abel N, Abeysekara U, Quintana AA, Abramyan A, Adamova D, et al. Transverse momentum spectra of charged particles in proton-proton collisions at s =900 GeV with ALICE at the LHC. Phys Lett B (2010) 693:53. doi:10.1016/j.physletb.2010.08.026
21. Aamodt K, Abel N, Abeysekara U, Abrahantes Quintana A, Abramyan A, Adamová D, et al. Production of pions, kaons and protons in pp collisions at s =900 GeV with ALICE at the LHC. Eur Phys J C (2011) 71:1655. doi:10.1140/epjc/s10052-011-1655-9
22. ALICE Collaboration. Measurement of (anti) nuclei production in p-Pb collisions at sNN = 8.16 TeV (2022). Available at: https://arxiv.org/abs/2212.04777.
23. Acharya S, Adamova D, Adhya SP, Adler A, Adolfsson J, Aggarwal MM, et al. Measurement of Λ (1520) production in pp collisions at s = 7 TeV and p-Pb collisions at sNN = 5.02 TeV. Eur Phys J C (2020) 80:160. doi:10.1140/epjc/s10052-020-7687-2
24. Abelev BB, Adam J, Adamová D, Aggarwal MM, Agnello M, Agostinelli A, et al. Neutral pion production at midrapidity in pp and Pb-Pb collisions at sNN = 2.76 TeV. Eur Phys J C (2014) 74:3108. doi:10.1140/epjc/s10052-014-3108-8
25. Tasevsky M. [ALICE, ATLAS, CMS, LHCb, LHCf and TOTEM], Soft QCD measurements at LHC (2018). Available at: https://arxiv.org/abs/1802.02818.
26. Aad G, Abbott B, Abdallah J, Abdelalim AA, Abdesselam A, Abdinov O, et al. Charged-particle multiplicities inppinteractions measured with the ATLAS detector at the LHC. New J Phys (2011) 13:053033. doi:10.1088/1367-2630/13/5/053033
27. Chatrchyan S, Khachatryan V, Sirunyan AM, Tumasyan A, Adam W, Bergauer T, et al. Study of the Production of Charged Pions, Kaons, and Protons in pPb Collisions at sNN = 5.02 TeV. Eur Phys J C (2014) 74:2847. doi:10.1140/epjc/s10052-014-2847-x
28. Khachatryan V, Sirunyan AM, Tumasyan A, Adam W, Bergauer T, Dragicevic M, et al. [CMS]. Transverse momentum and pseudorapidity distributions of charged hadrons in pp collisions at s =0.9 and 2.36 TeV. JHEP (2010) 02:041. doi:10.1007/JHEP02(2010)041
29. Li BC, Fu YY, Wang LL, Wang EQ, Liu FH. Transverse momentum distributions of strange hadrons produced in nucleus-nucleus collisions at sNN = 62.4 GeV and 200 GeV. J Phys G (2012) 39:025009. doi:10.1088/0954-3899/39/2/025009
30. Li BC, Fu YY, Wang LL, Liu FH. Dependence of elliptic flows on transverse momentum and number of participants in Au + Au collisions at sNN = 200 GeV. J Phys G (2013) 40:025104. doi:10.1088/0954-3899/40/2/025104
31. Deppman A, Megias E, Menezes DP. Fractals, nonextensive statistics, and QCD. Phys Rev D (2020) 101:034019. doi:10.1103/physrevd.101.034019
32. Deppman A, Megias E, Menezes DP. Fractal structures of yang–mills Fields and non-extensive statistics: Applications to high energy physics. MDPI Phys (2020) 2:455–80. doi:10.3390/physics2030026
33. Cleymans J, Worku D. Relativistic thermodynamics: Transverse momentum distributions in high-energy physics. Eur Phys J A (2012) 48:160. doi:10.1140/epja/i2012-12160-0
34. Acharya S, Acosta FT, Adamova D, Adolfsson J, Aggarwal MM, Rinella GA, et al. Measurement of D0D+, D*+ and Ds+ production in Pb-Pb collisions at sNN =5.02 TeV. JHEP (2018) 10:174. doi:10.1007/JHEP10(2018)174
35. Sirunyan AM, Tumasyan A, Adam W, Ambrogi F, Asilar E, Bergauer T, et al. Measurement of prompt and nonprompt charmonium suppression in PbPb collisions at 5.02 TeV. Eur Phys J C (2018) 78:509. doi:10.1140/epjc/s10052-018-5950-6
36. Chatrchyan S, Khachatryan V, Sirunyan AM, Tumasyan A, Adam W, Bergauer T, et al. Study of high-pT charged particle suppression in PbPb compared to pp collisions at sNN =2.76 TeV. Eur Phys J C (2012) 72:1945. doi:10.1140/epjc/s10052-012-1945-x
Keywords: Tsallis statistics, multisource production, transverse momentum spectra, nuclear modification factor, high-energy heavy-ion collisions
Citation: Geng Y-F and Li B-C (2023) Properties of the particle distribution in Pb–Pb collisions at
Received: 13 July 2023; Accepted: 24 August 2023;
Published: 13 September 2023.
Edited by:
Airton Deppman, University of São Paulo, BrazilReviewed by:
Ying Yuan, Guangxi University of Chinese Medicine, ChinaWaqas Muhammad, Hubei University of Automotive Technology, China
Junsheng Li, Shanxi Normal University, China
Copyright © 2023 Geng and Li. This is an open-access article distributed under the terms of the Creative Commons Attribution License (CC BY). The use, distribution or reproduction in other forums is permitted, provided the original author(s) and the copyright owner(s) are credited and that the original publication in this journal is cited, in accordance with accepted academic practice. No use, distribution or reproduction is permitted which does not comply with these terms.
*Correspondence: Bao-Chun Li, czYxMDlAc3h1LmVkdS5jbg==