- 1Anhui Province Key Laboratory of Medical Physics and Technology, Institute of Health and Medical Technology, Hefei Institutes of Physical Science, Chinese Academy of Sciences, Hefei, Anhui, China
- 2University of Science and Technology of China, Hefei, Anhui, China
- 3The 26th Research Institute of China Electronics Technology Group Corporation, Chongqing, China
A new type of lithium niobate (LiNbO3) acousto-optic Q-switched Er,Cr:YSGG laser with high peak power was designed and the thermal lensing effect compensation was studied. The values of thermal focal length in the gain medium were calculated theoretically and measured experimentally. The experimental results validate the designed plane-convex resonator validity, and the output performance of the 2.79 μm Er,Cr:YSGG laser was obviously improved. When the laser operated at free running region, the maximum values of pulse energy was 160 mJ at 60 Hz, compared with the plane-parallel resonator, the pulse energy was increased by 2 times in the plane-convex resonator. When the LiNbO3 Q-switched laser operated at 60 Hz, the maximum values of pulse energy was 8.5 mJ, and the minimum values of pulse duration was 60.8 ns, and the corresponding peak power approximately was 140 kW, respectively. Compared with the plane-parallel resonator, the pulse energy was increased by 1.6 times in the plane-convex resonator, the corresponding peak power was increased by 2.3 times.
1 Introduction
Currently, mid-infrared laser with narrow pulse duration, high peak power and good stability has wide range of scientific research and practical application value [1, 2]. The free electron laser can obtain the mid-infrared laser of peak power with adjustable wavelength, but the opto-electromechanical structure of this system is very complex, large size and high investment cost. Optical parametric oscillator (OPO) can also realize the output and wavelength tuning of mid-infrared laser by pumping nonlinear variable frequency crystal with near-infrared solid state laser. Due to its all-solid state, compact and low price, it has attracted wide attention in recent years [3, 4]. To improve the conversion efficiency to the mid-infrared wavelength (3–12-μm wavelength range) and obtain greater output power or energy, mid-infrared pumping sources with short pulse duration and high peak power is desired. The 2.79 μm Er,Cr:YSGG crystal has been proven to be a high efficiency flash-lamp pumped laser medium [5–7]. Er3+ doped YSGG laser has longer upper laser level lifetime of 1.3 ms, lower laser threshold caused by low phonon energy and higher conversion efficiency [8]. Since both Cr3+ and Er3+ ions have overlapping absorption bands with the xenon lamp, emission spectrum, there are two pumping processes working together, one is direct resonant pumping from Er3+ itself. The other is indirect Cr3+ collision Er3+ energy transfer pumping, which can improve conversion efficiency. Therefore, the sensitizing ions Cr3+ doped Er:YSGG crystal is one of the most promising candidates for realizing the output with high repetition rate and high pulse energy when the pumping source is flash-lamp. More importantly, compared with LD-pumped laser, the flash-lamp pumped laser can carry out larger energy pumping, and can get larger pulse energy output. In the Q-switched technology of realized narrow pulse duration and high peak power, acousto-optical (AO) Q-switched technology utilizes the diffraction effect of light to achieve stable laser output at high repetition rate, and has the advantages of small insertion loss and compact structure. Bulk LiNbO3 crystals have excellent AO properties and can be used as ideal AO medium. LiNbO3 crystal as AO medium has higher transmission at 3-μm wavelength range, lower acoustic attenuation coefficient (1 dB/cm @ 1 GHz) [9] and higher damage threshold (>200 MW/cm2) [10]. We innovated and developed LiNbO3-based AO Q-switch, and the effectiveness was verified in our previous study [11].
The thermal lensing effect always is an important factor affecting the output performance of all solid-state laser at high repetition rate. Er,Cr:YSGG crystal has high quantum defect, accordingly, strong thermal lensing effect at high repetition rate operation has an adverse effect on the output performance of the laser. Compensating or reducing the strong thermal lensing effect in the laser crystal to achieve higher output energy and better beam quality is one of the main problems in the field of developed mid-infrared laser. The main means of thermal lensing effect compensation are grinding negative curvatures on the crystal ends [12, 13], thermal bonding [13, 14], and thermal stable resonator structure [15–17]. Among them, thermal bonding technology is mainly used in the LD pumped laser system [13, 14, 18]. The center of curvature of two concave surfaces is required to be on the same optical axis in the grinding negative curvatures on the crystal ends technology, which puts forward higher requirements for machining accuracy. A few years ago, we used the grinding negative curvatures on the crystal ends to compensate for the strong thermal lensing effect in the LD-side pumped Er:YSGG crystal, and obtained a higher pulse energy 2.79 μm laser output at lower repetition rate [12]. The designing of resonator does not require any optical element insertion, nor does it require technical processing of laser crystal, and under certain pumping conditions can better compensate the thermal focal lensing effect, improve the output energy of the laser and improve the beam quality [3, 15, 19]. AO Q-switched technology can realize non-polarized laser oscillation, which is especially suitable for the design of thermal compensation resonator, and is conducive to obtaining larger laser pulse energy output. In addition, the damage threshold of 3-μm wavelength range Q-switch is generally low. The thermal lensing effect is compensated by the resonator design to improve the output performance of the laser, which can reduce the risk of Q-switch damage at high repetition rate, and improve the reliability and stability of the 2.79 μm Er,Cr:YSGG laser.
The goal of this study is to obtain laser output with high pulse energy and narrow pulse width at high repetition rate, so as to satisfy the application of ∼ 3 μm laser in dental and orthopedic ablation surgery. For this purpose, the focus of this study is not to pursue a higher beam quality index, but a higher pulse energy and beam quality suitable for application. In order to obtain high energy pulse laser output, the pump source used in this manuscript is market-oriented cheap xenon flash rather than expensive LD pump source, in order to meet the needs of the majority of clinical users. As we all know, the xenon flash-lamp pumped laser will cause strong thermal lensing effect at high repetition rate pumping, and the thermal conductivity of YSGG crystal is about 1/3 of that of YAG crystal, resulting in unsatisfactory M2 factor value of Er,Cr:YSGG crystal laser. In this manuscript, the plane-convex resonator was used to compensate the thermal lensing effect to optimize the beam quality, so as to obtain the appropriate beam quality.
In this letter, the thermal focal length of Er,Cr:YSGG laser crystal was calculated theoretically and measured experimentally. The thermal lensing effect compensation resonator structure was designed. The plane-convex resonator (PCR) was used to compensate the thermal lensing effect of Er,Cr:YSGG laser crystal. The output characteristics of AO Q-switched Er,Cr:YSGG laser pumped by flash-lamp were studied at 60 Hz. The results showed that the designed thermal compensated plane-convex resonator significantly improved the beam quality and the output capacity of the laser at high repetition rate. The new LiNbO3 AO Q-switched Er,Cr:YSGG laser achieved stable laser output, narrow laser pulse duration and high pulse energy.
2 Laser experimental setup
The schematic experimental setup of the 2.79-μm emission Er,Cr:YSGG laser system with a LiNbO3-based AO Q-switch is shown in Figure 1. The gain medium has a diameter of 3 mm and a length of 100 mm. The dopant concentrations of Er3+ and Cr3+ of this laser crystal are 30 at.% and 1.5 at.%, respectively. The two facets of the laser gain medium were antireflection coated at 2.79 μm. The Er,Cr:YSGG crystal was pumped by xenon flash-lamp inside a pumping resonator, and the value of pumping duration was approximately 100 µs. To ensure high cooling efficiency, the laser rod and the flash-lamp were cooled by circulating deionized water at a temperature of 283 ± 0.3 K with a large flow rate of 33 L/min. The generation of 2.79-μm wavelength laser was between the high reflective (HR) mirror and the output coupler (OC) mirror, and the geometric length of the resonator was 275 mm.
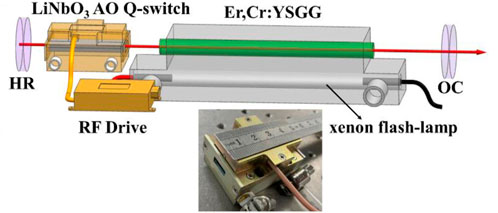
FIGURE 1. Schematic diagram of the 2.79-μm emission Er,Cr:YSGG laser system with LiNbO3-based AO Q-switch. The photograph of the LiNbO3 AQ-switch is shown in inset.
The LiNbO3-based AO Q-switch was placed between the rear mirror HR and the Er,Cr:YSGG active medium inside an optical resonator, and the length × width ×height of the LiNbO3 acousto-optic medium are 48 × 18 × 5.5 mm3, respectively. The two ends of LiNbO3 AO crystal were coated with 2.79 μm anti-reflection film respectively, and the transmission of the LiNbO3 crystal was about 90% at 2.79-μm wavelength, which can reduce insertion loss in the resonator. When the RF frequency was 40.68 MHz and the RF drive power was adjusted to the maximum 30 W, the maximum diffraction efficiency of the Q switch was 57%.
3 The design of the laser resonator and thermal effect compensation
At high pumping power, thermal deposition in laser crystal results in uneven temperature distribution, thermal stress and thermal deformation within the crystals, resulting in thermal focal lensing effect (as shown in Figure 2A). The thermal length can be expressed as [20].
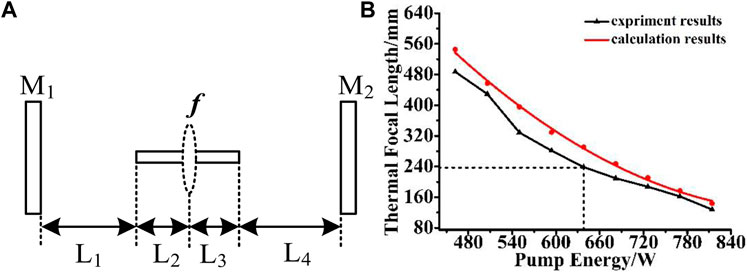
FIGURE 2. Thermal focal length in various pump power (A); the equivalent schematic diagram of the resonant cavity (B).
Where, K is the thermal conductivity, A is the cross section area of the laser rod, dn/dT is the temperature gradient of the refractive index, α is the coefficient of thermal expansion, Pa is the heat absorbed by the laser crystal. To compensate the thermal lensing effect effectively, the thermal focal length of Er,Cr:YSGG laser crystals was calculated theoretically according to formula (1). Because the thermal focal length of laser crystal is related to many factors, the theoretical calculation can not be completely accurate to get the values of the thermal focal length. Hence, the thermal focal length of Er,Cr:YSGG laser crystal was measured by the critical resonator stabilization method in plane-parallel resonator (PPR) at 60 Hz. The changing relationship between thermal focal length and pump power was shown in Figure 2B.
In the free oscillation region (without LiNbO3 AO Q-switch), we carried out the measurement of the laser maximum pulse output energy of three output coupling mirrors with the reflectance of 60%, 70% and 80%. The laser output pulse energy of the output coupling mirror with the reflectance of 70% was the largest, so we chose this as the experimental output coupling mirror. Because of the serious thermal focal lensing effect under high repetition rate pumping, the laser output capacity in the plane-parallel resonator was limited. The values of laser output energy were measured by an energy meter (COHERENT J-50MB-IR). Figure 3 shows the input-output characteristics at 60 Hz. As shown in Figure 3, the maximum laser pulse energy was 78.7 mJ in the plane-parallel resonator, and the corresponding pump power was 638 W. Then the thermal lensing effect became serious as the pump energy increases, and the laser output pulse energy decreased rapidly.
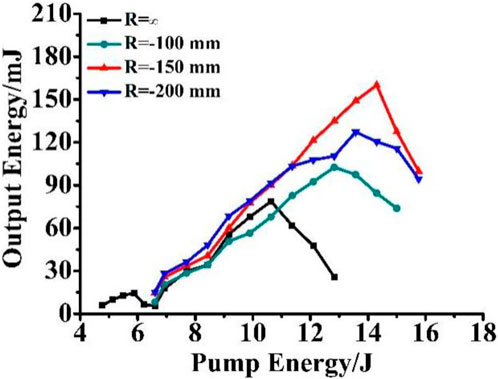
FIGURE 3. Characteristic curves of output energy changed with pump energy in the free oscillation region at 60 Hz.
In a certain pump power range, the plane-convex resonator can effectively compensate the thermal lensing effect, so as to optimize the beam quality and improve the output performance of the laser. The pump power corresponding to the maximum laser pulse energy (78.7 mJ) was 638 W in plane-parallel resonator, and the thermal focal length was 254.9 mm as shown by the dotted line in Figure 2B. To higher laser pulse energy and appropriate beam quality to meet the application requirements, so it is our purpose to find a resonator mirror curvature that can obtain larger laser pulse energy. According to the design theory of the resonator with the embedded thermal lens, when
Where,
The experimental results shown that the designed plane-convex resonator can effectively compensate the thermal lensing effect in Er,Cr:YSGG crystals, improve the output energy of laser, and realize the stable output of static laser. It lays a foundation for realizing the laser pulse with high peak power and short pulse duration by inserting LiNbO3 AO Q-switch into the plane-convex resonator.
4 Experimental results and discussion
4.1 Influence of thermal lensing effect on pulse performances of AO Q-switched
In order to explore the influence of thermal lensing effect on the output performances of LiNbO3 AO Q-switch, a comparison experiment of Q-switched between the plane-parallel resonator and the plane-convex resonator was designed. In the experiment, the high reflective mirror was a convex mirror with a curvature radius of −150 mm, the reflectivity of the OC mirror was 70%, and the RF driving power was 30 W. As shown in Figure 4A, when the repetition rate was 60 Hz, the values of the maximum pulse energy of LiNbO3 AO Q-switch were 5.3 mJ and 8.5 mJ in the plane parallel resonator and the plane-convex resonator, and the corresponding pulse duration was 88.5 ns and 60.8 ns, respectively. The output pulse energy of LiNbO3 AO Q-switch in the plane-convex resonator was 1.6 times higher than that in the plane-parallel resonator. When the repetition rates was 60 Hz, the Q-switched pulse profile and corresponding pulse train were measured (see Figures 4B, C) with a detector (VIGO PVI-ZTE-10.6) in the plane-convex resonator, the pulse was a standard Q-switched pulse waveform, similar to Gaussian type, with smooth rising and falling edges and very weak bottom background. Therefore, the structure of plane-convex resonator can improve the output performance of LiNbO3 AO Q-switched Er,Cr:YSGG laser in a certain pump energy range.
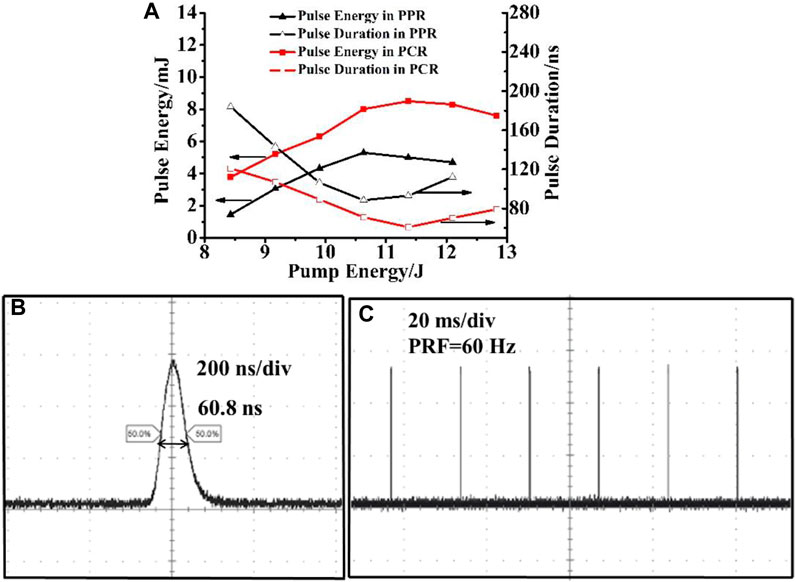
FIGURE 4. Characteristic curves of pulse energy and duration changed with pump energy (A); pulse profile at 8.5 mJ (B); corresponding pulse train (C).
4.2 The M2 and stability of the LiNbO3 AO Q-switched laser
The far-field laser beam profiles of the LiNbO3 AO Q-switched Er,Cr:YSGG laser were recorded with a pyroelectric array camera (Ophir-Spiricon PY-III-HR), and the M2 factor was determined to analyze the laser beam quality. As shown in Figure 5A, when the pulse energy was 8.5 mJ, the MX2/MY2 factors of the laser beam were 6.2 and 6.6 at 60 Hz. In our scheme, the MX2/MY2 factor values of the laser beam were increased from 9.82/10.9 in the plane-parallel resonator to 6.2/6.6 in the plane-convex resonator.
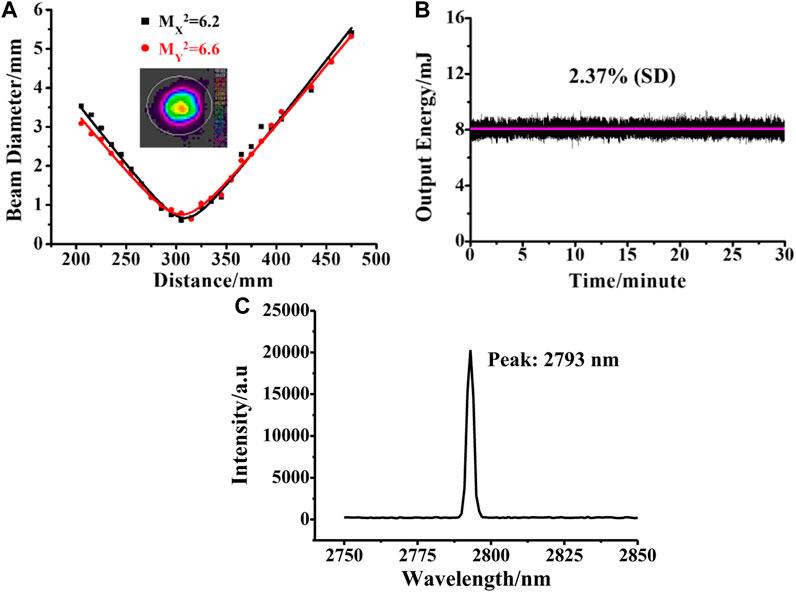
FIGURE 5. The factor of M2 and the far-field beam profile (A); pulse energy stability over 30 min (B); spectrogram of the LiNbO3 AO Q-switched Er,Cr:YSGG laser (C).
A variety of methods can be used to improve the beam quality, such as a small hole can be inserted in the resonator to sacrifice the laser pulse energy to obtain a higher beam quality, low thermal lensing effect and high beam quality of low energy laser oscillation + amplification scheme can be adopted or through LD end pump + multisegmented crystals scheme, or growth gradient doping concentration of Er,Cr:YSGG laser crystal [22]. The best way is to adopt the population-dynamic-coupled combined guiding effect method [23].
Also, to study the stability of the LiNbO3 AO Q-switched Er,Cr:YSGG laser, the laser output energy was tested for 30 min. As shown in Figure 5B, the energy fluctuation standard deviation (SD) of the laser was less than 2.37%, which was very stable. Therefore, the developed new LiNbO3 AO Q-switched Er,Cr:YSGG laser can achieve stable laser output with high pulse energy and narrow pulse duration at high repetition rate, the spectrogram of the LiNbO3 AO Q-switched Er,Cr:YSGG laser was shown in Figure 5C. In order to obtain pulse output with higher repetition rate, LD-pumped source can be used to reduce the influence of laser thermal lensing effect on LiNbO3 acousto-optic Q-switch.
At present, the high performance solid-state laser in the 3-μm wavelength range is not mature, so that the mid-infrared OPO system often uses the 1-μm short pulse laser as the pumping source. However, when it is converted to the mid-infrared above 5 μm, the quantum loss is large, the group velocity mismatch (GVM) is large, and the gain bandwidth is small because it is far from the degeneracy point, and other defects are more prominent. The conversion efficiency and amplifier bandwidth are greatly reduced, which greatly limits the improvement of mid-infrared OPO conversion efficiency and output performance. Because the 3-μm is closer to the mid-infrared wavelength range, the above problems can be solved by using the 3-μm wavelength range laser as pumping source of OPO system, which has become the forefront of mid-infrared OPO technology [3, 24, 25]. To this end, scientists are even tedious by indirectly pumping another nonlinear crystal to produce 2–3 μm parameter laser instead of 1-μm pumping source. Compared with the 1-μm pumping source, the 3-μm wavelength range of erbium laser has lower quantum deficit, smaller GVM and larger bandwidth, and the theoretical conversion efficiency is higher. Moreover, the signal light and idle light of the parametric conversion are in the interest mid-infrared wavelength range of (3–12 μm).
5 Conclusion
In this letter, a new 2.79-μm LiNbO3 acousto-optical Q-switched Er,Cr:YSGG laser was studied. The output performance of Er,Cr:YSGG laser was obviously improved by effectively compensating the thermal lensing effect in the gain medium with the plane-convex resonator structure. Hence, the LiNbO3 acousto-optic Q-switched Er,Cr:YSGG laser can achieve stable laser output with high pulse energy and narrow pulse duration at high repetition rate. The LiNbO3 acousto-optic Q-switch achieved stable Q-switching characteristics at 60 Hz in the designed plane-convex resonator. The maximum pulse energy was 8.5 mJ, the shortest pulse duration was 60.8 ns, the pulse duration was narrowed by 31%, and the corresponding peak power was increased by 2.3 times to 140 kW. However, the beam quality of the xeon-lamp pumped laser is not perfect, and needs further optimization and improvement. This study lays a foundation for enhancing the effective pumping spectrum in the absorption wavelength of the gain medium through LD pump source, reducing the waste heat generated by the ineffective spectrum, and improving the heat dissipation by using the slatted gain medium structure to further improve the beam quality.
Data availability statement
The raw data supporting the conclusion of this article will be made available by the authors, without undue reservation.
Author contributions
All authors listed have made a substantial, direct, and intellectual contribution to the work and approved it for publication.
Funding
This work is supported by National Key Research and Development Plan (2018YFB0407204).
Conflict of interest
Author ZW was employed by The 26th Research Institute of China Electronics Technology Group Corporation.
The remaining authors declare that the research was conducted in the absence of any commercial or financial relationships that could be construed as a potential conflict of interest.
The handling editor (HM) declared a shared affiliation with author (LJ) (SM) (YZ) (TC) (HJ) at the time of the review.
Publisher’s note
All claims expressed in this article are solely those of the authors and do not necessarily represent those of their affiliated organizations, or those of the publisher, the editors and the reviewers. Any product that may be evaluated in this article, or claim that may be made by its manufacturer, is not guaranteed or endorsed by the publisher.
Abbreviations
RF, radio frequency; OPO, optical parametric oscillator; OPG, optical parametric generation; mid-IR, medium infrared; EO, electro-optical; AO, acousto-optical; HR, high reflective; OC, output coupler; PCR, plane-convex resonator; PPR, plane-parallel resonator; RFDP, RF driving power; SD, stability degree.
References
1. Li BW, Yin GD, Wang JT, Yang JB, Yan PG. 2.76–3.98 μm picosecond mid-infrared optical parametric generation in a muti-grating MgO: Ppln. Front Phys (2022) 10:1082520. doi:10.3389/fphy.2022.1082520
2. Deng Y, Kang M, Wang Z, Li J, Jiang X, Xiao K, et al. A multi-wavelength mid-IR laser based onBaGa4Se7 optical parametric oscillators. Front Phys (2023) 11:1126773. doi:10.3389/fphy.2023.1126773
3. Hu SW, Wang L, Guo YW, Cheng TQ, Wu XY, Wang ZY, et al. High-conversion-efficiency tunable mid-infrared Baga4Se7 optical parametric oscillator pumped by a 2.79-μm laser. Opt Lett (2019) 44:2201–3. doi:10.1364/OL.44.002201
4. Xi C, Wang P, Li X, Liu ZJ. Highly efficient continuous-wave mid-infrared generation based on intracavity difference frequency mixing. High Power Laser Sci Eng (2019) 7:e67. doi:10.1017/hpl.2019.45
5. Zhang H, Li YL, Wu QT. 2.79 μm LGS electro-optical Q-switched Er,Cr:YSGG laser. Opt Commun (2022) 503:127448. doi:10.1016/j.optcom.2021.127448
6. Liu JS, Liu JJ, Tang Y. Performance of a diode end-pumped Cr,Er:YSGG laser at 2.79 μm. Laser Phys (2008) 18:1124–7. doi:10.1134/S1054660X08100022
7. Könz F, Frenz M, Romano V, Forrer M, Weber HP, Kharkovskiy AV, et al. Active and passive Q-switching of a 2.79 μm Er:Cr:YSGG laser. Opt Commun (1993) 103:398–404. doi:10.1016/0030-4018(93)90164-Z
8. Fang ZQ, Sun DL, Luo JQ, Zhang HL, Zhao XY, Quan C, et al. A modified formula of thermal focal length for lamp pumping Cr, Er:YSGG crystal with high performance 2.79 μm laser. Opt Laser Technol (2019) 115:398–403. doi:10.1016/j.optlastec.2019.02.051
9. Mys O, Kostyrko M, Krupych O, Vlokh R. Anisotropy of the acousto-optic figure of merit for LiNbO3 crystals: Isotropic diffraction. Appl Opt (2015) 54:8176–86. doi:10.1364/AO.54.008176
10. Yang JW, Wang L, Wu XY, Cheng TQ, Jiang HH. High peak power Q-switched Er:YAG laser with two polarizers and its ablation performance for hard dental tissues. Opt Express (2014) 22:15686–96. doi:10.1364/OE.22.015686
11. Jiang LL, Wu ZC, Cheng TQ, Jiang HH. 2.79-μm efficient acousto-optic Q-switched Er,Cr:YSGG laser with an LiNbO3 crystal modulator. Opt Lett (2022) 47:6193–6. doi:10.1364/OL.477264
12. Wang JT, Cheng TQ, Wang L, Yang JW, Sun DL, Yin ST, et al. Compensation of strong thermal lensing in an LD side-pumped high-power Er:YSGG laser. Laser Phys Lett (2015) 12:105004. doi:10.1088/1612-2011/12/10/105004
13. Chen YW, Sun DL, Zhang HL, Luo JQ, Quan C, Han ZY, et al. 2.79 μm Cr,Er:YSGG laser with a high energy realized by thermal bonding and concave end-face. Opt Laser Technol (2023) 162:109255. doi:10.1016/j.optlastec.2023.109255
14. Shen BJ, Kang HX, Sun DL, Zhang QL, Yin ST, Chen P, et al. Investigation of laser-diode end-pumped Er:YSGG/YSGG composite crystal lasers at 2.79 µm. Laser Phys Lett (2014) 11:015002. doi:10.1088/1612-2011/11/1/015002
15. Cui QZ, Wei ME, Xiong ZD, Hu SW, Jiang JT, Wang L, et al. 100-300 Hz repetition-rate acousto-optic Q-switched 2.79 μm Er:YSGG laser side-pumped by laser-diode. Infrared Phys Technol (2019) 98:256–9. doi:10.1016/j.infrared.2019.03.029
16. Arbabzadah EA, Phillips CC, Damzen MJ. Free-running and Q-switched operation of a diode pumped Er:YSGG laser at the 3µm transition. Appl Phys B (2013) 111:333–9. doi:10.1007/s00340-013-5337-1
17. Wang J, He QY, Gao JY, Kang ZH, Jiang Y, Sun H. Comparison of electrooptically Q-switched Er:Cr:YSGG lasers by two polarizers: Glan-Taylor prism and Brewster angle structure. Laser Phys Let (2006) 3:349–52. doi:10.1002/lapl.200610020
18. Tsunekane M, Taguchi N, Inaba H. Improvement of thermal effects in a diode-end-pumped, composite Tm:YAG rod with undoped ends. Appl Opt (1999) 38:1788–91. doi:10.1364/ao.38.001788
19. Wei ME, Cheng TQ, Dou RQ, Zhang QL, Jiang HH. High-peak-power electro-optically Q-switched laser with a gradient-doped Nd:YAG crystal. Opt Lett (2021) 46:5016–8. doi:10.1364/OL.442131
21. Xiong ZD. Study on high peak power, high repetition rate Fe:ZnSe passively Q-switched laser at 2.794 μm and dentin ablation and bonding strength. PhD. Thesis. (University of Science and Technology of China (2022).
22. Wei ME, Cheng TQ, Dou RQ, Zhang QL, Jiang HH. Superior performance of a 2 kHz pulse Nd:YAG laser based on a gradient-doped crystal. Photon Res (2021) 9:1191–6. doi:10.1364/PRJ.424989
23. Shen YJ, Gong ML, Fu X. Beam quality improvement by population-dynamic-coupled combined guiding effect in end-pumped Nd:YVO4 laser oscillator. Appl Phys B (2018) 124:85–9. doi:10.1007/s00340-018-6954-5
24. Dherbecourt JB, Godard A, Raybaut M, Melkonian JM, Lefebvre M. Picosecond synchronously pumped ZnGeP2 optical parametric oscillator. Opt Lett (2010) 35:2197–9. doi:10.1364/OL.35.002197
Keywords: nanosecond pulsed 3-μm lasers, Er,Cr:YSGG laser, thermal lensing compensation, high peak power, acousto-optics Q-switch
Citation: Jiang L, Wu Z, Ma S, Zhu Y, Cheng T and Jiang H (2023) 2.79-μm high peak power LiNbO3 acousto-optic Q-switched Er,Cr:YSGG laser with thermal lensing effect compensation. Front. Phys. 11:1201822. doi: 10.3389/fphy.2023.1201822
Received: 07 April 2023; Accepted: 21 June 2023;
Published: 15 August 2023.
Edited by:
Haotong Ma, Chinese Academy of Sciences (CAS), ChinaReviewed by:
Yijie Shen, University of Southampton, United KingdomChujun Zhao, Hunan University, China
Zhiyi Wei, Chinese Academy of Sciences (CAS), China
Xuechun Lin, Chinese Academy of Sciences (CAS), China
Copyright © 2023 Jiang, Wu, Ma, Zhu, Cheng and Jiang. This is an open-access article distributed under the terms of the Creative Commons Attribution License (CC BY). The use, distribution or reproduction in other forums is permitted, provided the original author(s) and the copyright owner(s) are credited and that the original publication in this journal is cited, in accordance with accepted academic practice. No use, distribution or reproduction is permitted which does not comply with these terms.
*Correspondence: Haihe Jiang, aGppYW5nQGFpb2ZtLmFjLmNu