- 1School of Optical and Electronic Information and Wuhan National Laboratory for Optoelectronics, Huazhong University of Science and Technology, Wuhan, China
- 2GBA Branch of Aerospace Information Research Institute, Chinese Academy of Sciences, Guangzhou, China
- 3School of Optics and Photonics, Beijing Institute of Technology, Beijing, China
We demonstrate a Kerr-lens mode-locked femtosecond Yb:YAG thin-disk oscillator and investigate the approach to increase the optical-to-optical efficiency based on the scheme of direct multiple passes of the laser beam through the thin-disk medium. With twelve passes through the thin disk, 266-fs pulses were delivered from the oscillator with an average power of 105.6 W at a repetition rate of 20 MHz. The corresponding optical-to-optical efficiency is 31.1%, which is, to the best of our knowledge, the highest efficiency of any mode-locked thin-disk oscillator with pulse duration below 300 fs. This demonstration paves the way to even more efficient mode-locked femtosecond thin-disk oscillators, and provides an excellent laser source for the applications such as non-linear frequency conversion and high-precision industrial processing.
1 Introduction
High-power high-energy femtosecond laser oscillators find growing applications in various fields such as high-harmonic generation [1, 2], high-precision micromachining [3] and biological imaging [4]. Thin-disk technology is one of the most promising approaches for the generation of high power high-energy ultrashort laser pulses due to its excellent properties. For example, the very thin gain medium attached on a water-cooled heat sink yields a highly efficient cooling capacity, which greatly reduces the thermal effects under a high pump power density compared to the traditional bulk-crystal lasers. Besides, the large ratio of the disk diameter to the thickness also enables an almost one-dimensional heat flow along the thickness direction, and results in excellent beam qualities during the laser operation. Furthermore, due to the very small thickness of the disk and the large mode size in it, the non-linear phase shift that ultrashort laser pulses experience in a thin disk is much lower than in other gain medium geometries, supporting high intracavity peak power in a mode-locked thin-disk oscillator and correspondingly high external peak power.
Since the invention of the thin-disk technology in 1994 [5], substantial progresses have been made in the power and energy scaling of mode-locked thin-disk oscillators. With semiconductor saturable absorber mirrors (SESAM) as the mode-locking devices, pulses with an average power up to 350 W [6] and energy up to 80 μJ [7] have been achieved directly from the Yb:YAG thin-disk oscillators, respectively. However, due to small modulation depth and slow response time of the SESAM, the pulse duration obtained from SESAM mode-locked Yb:YAG thin-disk oscillators are limited above 500 fs [8]. Besides, non-linear phase shifts accumulated in the air usually needs to be avoided by operating the oscillator in vacuum or inert-gas atmosphere so as to stabilize the mode locking with high-power pulses generation [9]. By contrast, Kerr-lens mode locked (KLM) thin-disk oscillators can be reliably operated in atmospheric air and offer high-power pulses with much shorter pulse duration with large modulation depth and fast response time. The highest average power up to 270 W has been achieved from a KLM thin-disk oscillator with a pulse duration of 330 fs [10]. In the meantime, short pulses with duration below 300 fs were generated with relatively lower average powers (100–200 W) [11–13], which enables the KLM thin-disk oscillators more flexible for practical use. By enhancing the Kerr-lens effect and increasing the intracavity self-phase modulation, the pulse duration from a KLM Yb:YAG thin-disk oscillator was shortened further down to ∼50 fs level [13–16], much shorter than that of the gain bandwidth limit (full width at half maximum, FWHM). These results have showed great advantage of the KLM thin-disk oscillators in generating shorter pulse duration with high average power.
In order to further scale the average power and pulse energy of the thin-disk oscillators, one could adopt the geometric scaling concept to acquire a large beam size while increasing the pump power [11]. It will enable a constant pump power density well below the damage threshold of the disk. However, this concept also creates a challenge for the disk surface quality and the design of oscillator cavity. Another method is to increase the optical-to-optical efficiency. This can be realized in the Yb:YAG thin disk oscillators by changing the wavelength of the pump diode from 940 nm to 969 nm, whereas at the price of an increase of the cost and instability. The most effective way is based on the increased gain provided by multiple passes through the thin-disk laser medium [12], which until now has significantly improved the optical-to-optical efficiency of the mode-locked thin-disk oscillators with both KLM and SESAM techniques. However, the optical-to-optical efficiencies of high-power thin-disk oscillators in short duration region (<300 fs) are still limited below 30% (see Figure 1). Especially for the direct multi-pass scheme without using an imaging section, it is very challenging to achieve a high optical-to-optical efficiency due to the growing difference in mode size on the disk among the passes.
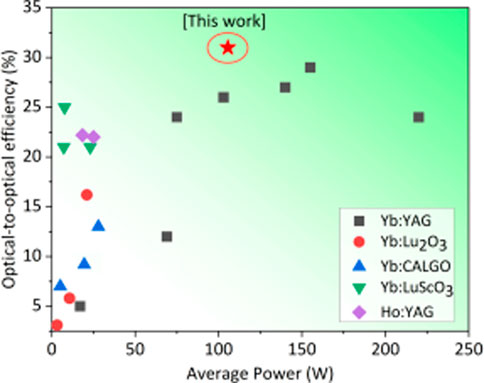
FIGURE 1. Overview of optical-to-optical efficiencies and average power for various mode-locked thin-disk oscillators with pulse the duration less than 300 fs [11–29].
In this work, we investigate the direct multiple-pass scheme in a KLM Yb:YAG thin-disk oscillator with an optimized laser cavity design. The experimental results showed an evident improvement of the optical-to-optical efficiencies when the number of laser passes through the disk was increased. Pulses as short as 266 fs were delivered from the twelve-pass cavity with an average power of 105.6 W and an optical-to-optical efficiency of 31.1%. To the best of our knowledge, this is the highest efficiency of any mode-locked thin-disk oscillator in the short pulse duration region (<300 fs) so far.
2 Experimental setup
The schematic of the mode-locked oscillator is illustrated in Figure 2. Initially we built the oscillator with four-pass configuration, as shown in Figure 2A. The 130-μm thin Yb:YAG disk with a Yb3+ doping concentration of 7% is placed inside a 48-pass pump module. The disk is antireflection coated for the pump and laser emission on the front surface and high-reflection coated for both wavelengths (R > 99.9%) on the back side. It is pumped by a fiber-coupled diode laser at 940 nm and used as a folding mirror within a Z-shaped cavity. A 2-mm-thick sapphire plate is placed in the focus of the telescope section composed of two concave mirrors to act as a Kerr medium, affording the necessary Kerr-lens effect. We also put a water cooled copper hard aperture in the beam path in order to aid the stabilization of KLM. The laser beam is reflected twice on the back side of the disk per round trip, corresponding to four propagating passes through the disk medium. The total cavity length is ∼3,750 mm, corresponding to a repetition rate of 40 MHz. After this initial arrangement, the cavity was rebuilt with the aid of a pair of flat folding mirrors to exhibit four reflections on the disk within one round trip, as shown in Figure 2B. The cavity length was increased to 7,500 mm, corresponding to a repetition rate of 20 MHz. Figure 2C shows the cavity setup containing six reflections on the disk via two pairs of flat folding mirrors with cavity length same as in Figure 2B. The number of the laser passes through the disk is eight and twelve in these two setups (Figures 2B, C), respectively.
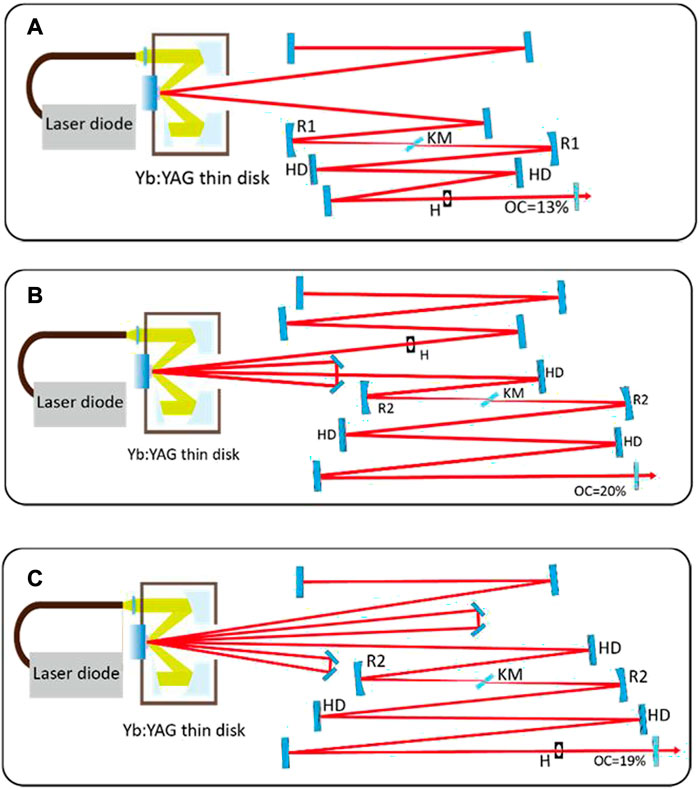
FIGURE 2. Schematics of the KLM thin-disk Yb:YAG oscillator realized with passes of (A) four, (B) eight, and (C) twelve. HR: All flat mirrors are highly reflective; HD: High-dispersion mirrors; OC: Output coupler; KM: Kerr medium; H: Hard aperture; R1 and R2: Concave mirrors with radius of curvature (ROC) of −250 mm and −500 mm, respectively.
3 Results and discussion
In the four-pass configuration (Figure 2A), the pump spot diameter was set to be 2.7 mm. The round-trip anomalous group delay dispersion (GDD) was −12000 fs2, provided by a pair of high-dispersion chirped mirrors. The mode locking was triggered by perturbing the OC mounted on a translation stage. Under an output coupling rate of 13%, an average power of 32 W was reached at a pump power of 220 W, corresponding to an optical-to-optical efficiency of 14.5%. The mode-locked spectrum was measured using a spectrometer (DEVISER, AE8600) with a width of 4.1 nm at FWHM, as shown in Figure 3A. Figure 3B shows the pulse trace measured with an intensity autocorrelator. The pulse duration was 271 fs assuming a sech2 fit, indicating an almost Fourier-transform limited pulse with a time-bandwidth product of 0.32. The pulse energy was limited as 0.8 μJ at the repetition rate of 40 MHz. Optimizations were implemented based on the four-pass configuration by increasing the pump power and output coupling rate, and the average power and pulse energy could be increased to 54 W and 1.35 μJ, respectively. However, the mode locking showed pronounced instability, accompanied with multi-pulsing effect or continuous wave spike appearance in the spectrum.
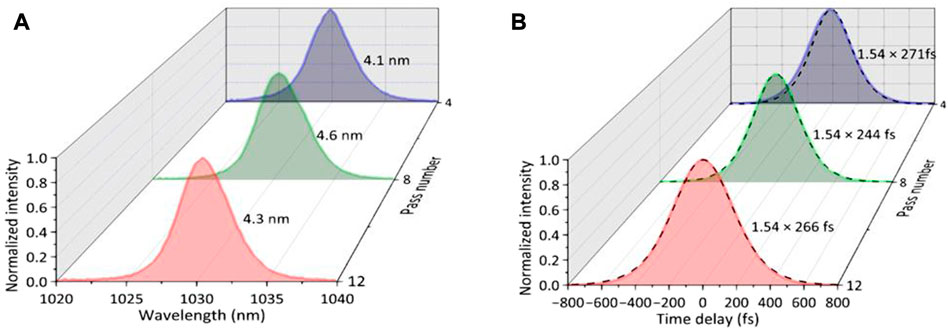
FIGURE 3. Spectra (A) and pulse durations (B) of the KLM Yb:YAG oscillator depicted in Figure 2 with passes of four, eight, and twelve.
Further improvement of the oscillator was realized by increasing the number of the propagating passes through the disk up to eight. In the meantime, the pump spot size was increased to 3.3 mm, and the cavity length was extended to reduce the repetition rate down to 20 MHz. Compared to the first oscillator, there was a request for the eight-pass configuration to design the cavity and avoid the difference in mode size between the first and second reflections on the thin disk. This would ensure efficient power extracting from the disk medium and increase the round-trip gain. With this configuration, the mode locking was realized with a round-trip anomalous dispersion of −18000 fs2 and a raised output coupling rate of 20%. 247-fs pulses with an average power of 60 W were generated under a pump power of 350 W, corresponding to an increased optical-to-optical efficiency of 17.1% and a pulse energy of 3 μJ. The measured spectrum and pulse duration are shown in Figure 3.
After this successful improvement, the number of the propagating passes through the disk was increased to twelve. In order to keep the mode size similar among the passes and extract the power from the disk more efficiently, a symmetric cavity configuration was used with the lengths of the two cavity arms (One arm spans the distance from the KM to the output coupler, and the second spans the remainder of the cavity) comparable with each other. With round-trip dispersion of −18000 fs2 and an output coupling rate of 19%, the oscillator was mode locked and delivered 266-fs pulses with an average power of 105.6 W under a pump power of 340 W. The optical-to-optical efficiency was increased up to 31.1%, and the pulse energy reached 5.3 μJ at a repetition rate of 20 MHz. Figure 3 shows the measured spectrum and pulse duration trace. In this case, the radio frequency (RF) spectrum was characterized using a RF spectrum analyzer (Agilent E4440A) at a resolution bandwidth of 100 Hz (see Figure 4). The fundamental beat note has a signal-to-noise ratio of 73 dB, indicating a stable mode-locked operation of the thin-disk oscillator. With a simple monolithic housing, the KLM operation was able to be maintained for several hours on a day-to-day basis under ambient air conditions. The pulses have an excellent beam profile with the beam quality factor M2 around 1.1.
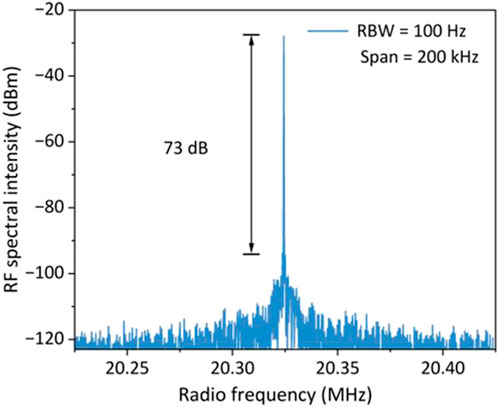
FIGURE 4. Fundamental RF spectrum measured for twelve-pass configuration at a resolution bandwidth of 100 Hz showing a signal-to-noise ratio of 73 dB.
For the next step, a larger number of passes can be employed and should enable even higher output power and efficiency. One might suffer from the spatial constraints while adding further passes by flat folding mirrors due to the limited window size of the multi-pass pump module. This can be solved by guiding the laser beam on both horizontal and vertical plane. Another challenge is the growing difference in mode size among the passes, which can be avoided via an optimized cavity design. Besides, an imaging concept called active multipass cell (AMC) has been demonstrated in both KLM and SESAM mode-locked thin-disk oscillators [6, 12], which adopts concave mirrors for the pass folding and can increase the number of thin-disk passes without affecting the main cavity mode size. Based on this concept, further increase of the output power and efficiency from a KLM thin-disk oscillator can be expected. Such a high-power and high-energy KLM thin-disk oscillator can be used as the seed source for the laser amplifiers, which makes it possible to obtain pulses with high energy up to 1 J [30, 31].
4 Conclusion
In conclusion, we have realized the generation of high-power femtosecond pulses from a KLM Yb:YAG thin-disk oscillator based on the direct multiple-pass concept (see Table 1). We have shown that an increased number of the thin-disk passes is beneficial to the improvement of the optical-to-optical efficiency. In addition, efficient power extracting can be enabled by an optimized cavity configuration with similar mode size among different passes. The twelve-pass configuration enables 266-fs pulses generation with an average power up to 105.6 W and an optical-to-optical efficiency up to 31.1%. Further increase of the efficiency up to 40% will be feasible with a larger number of passes through the gain medium. The current oscillator provides an excellent laser source for non-linear frequency conversion and high-precision industrial processing.

TABLE 1. Parameters of the realized oscillators with different thin-disk passes. frep, repetition rate; GDD, intra-cavity dispersion; Pavg, average power; EP, pulse energy; τ, pulse duration; ηO−O, optical-to-optical efficiency.
Data availability statement
The original contributions presented in the study are included in the article/supplementary material, further inquiries can be directed to the corresponding authors.
Author contributions
The main setup was designed and built by HC. The data was measured, analyzed and interpreted by HC, LY, HL, JH, TY, HL, JZ, QL, HW, GZ, XZ, HX, QW, and JZ. The experiment was conceived by JZ (Jinwei Zhang). All authors reviewed and contributed to the final manuscript.
Funding
This work is supported by the National Natural Science Foundation of China (No. 62075068), the International Science and Technology Cooperation Programme of Hubei Province (No. 2021EHB004) and the Research Project of Aerospace Information Research Institute, Chinese Academy of Sciences (E1Z1D101, E2Z2D101).
Conflict of interest
The authors declare that the research was conducted in the absence of any commercial or financial relationships that could be construed as a potential conflict of interest.
Publisher’s note
All claims expressed in this article are solely those of the authors and do not necessarily represent those of their affiliated organizations, or those of the publisher, the editors, and the reviewers. Any product that may be evaluated in this article, or claim that may be made by its manufacturer, is not guaranteed or endorsed by the publisher.
References
1. Emaury F, Diebold A, Saraceno CJ, Keller U. Compact extreme ultraviolet source at megahertz pulse repetition rate with a low-noise ultrafast thin-disk laser oscillator. Optica (2015) 2:980–4. doi:10.1364/OPTICA.2.000980
2. Galletti M, Pires H, Hariton V, João CP, Künzel S, Galimberti M, et al. High efficiency second harmonic generation of nanojoule-level femtosecond pulses in the visible based on BiBO. High Power Laser Sci Eng (2019) 7:11. doi:10.1017/hpl.2018.72
3. Jiang G, Narushima T, Okamoto H. Nonlinear optical effects in trapping nanoparticles with femtosecond pulses. Nat Phys (2010) 6:1005–9. doi:10.1038/nphys1776
4. Liu F, Yoo KM, Alfano RR. Ultrafast laser-pulse transmission and imaging through biological tissues. Appl Opt (1993) 32:554–8. doi:10.1364/AO.32.000554
5. Giesen A, Hügel H, Voss A, Wittig K, Brauch U, Opower H. Scalable concept for diode-pumped high-power solid-state lasers. Appl Phys B (1994) 58:365–72. doi:10.1007/BF01081875
6. Saltarelli F, Graumann IJ, Lang L, Bauer D, Phillips CR, Keller U. Power scaling of ultrafast oscillators: 350-W average-power sub-picosecond thin-disk laser. Opt Express (2019) 27:31465–74. doi:10.1364/OE.27.031465
7. Saraceno CJ, Emaury F, Schriber C, Hoffmann M, Golling M, Südmeyer T, et al. Ultrafast thin-disk laser with 80 μJ pulse energy and 242 W of average power. Opt Lett (2014) 39:9–12. doi:10.1364/OL.39.000009
8. Saraceno CJ, Schriber C, Mangold M, Hoffmann M, Heckl OH, Baer CRE, et al. SESAMs for high-power oscillators: Design guidelines and damage thresholds. IEEE J Selected Top Quan Electron (2012) 18:29–41. doi:10.1109/JSTQE.2010.2092753
9. Saraceno CJ, Emaury F, Heckl OH, Baer CRE, Hoffmann M, Schriber C, et al. 275 W average output power from a femtosecond thin disk oscillator operated in a vacuum environment. Opt Express (2012) 20:23535–41. doi:10.1364/OE.20.023535
10. Brons J, Pervak V, Fedulova E, Bauer D, Sutter D, Kalashnikov V, et al. Energy scaling of Kerr-lens mode-locked thin-disk oscillators. Opt Lett (2014) 39:6442–5. doi:10.1364/OL.39.006442
11. Brons J, Pervak V, Bauer D, Sutter D, Pronin O, Krausz F. Powerful 100-fs-scale Kerr-lens mode-locked thin-disk oscillator. Opt Lett (2016) 41:3567–70. doi:10.1364/OL.41.003567
12. Poetzlberger M, Zhang J, Gröbmeyer S, Bauer D, Sutter D, Brons J, et al. Kerr-lens mode-locked thin-disk oscillator with 50% output coupling rate. Opt Lett (2019) 44:4227–30. doi:10.1364/OL.44.004227
13. Fischer J, Drs J, Modsching N, Labaye F, Wittwer VJ, Südmeyer T. Efficient 100-MW, 100-W, 50-fs-class Yb:YAG thin-disk laser oscillator. Opt Express (2021) 29:42075–81. doi:10.1364/OE.442833
14. Drs J, Fischer J, Modsching N, Labaye F, Wittwer VJ, Südmeyer T. Sub-30-fs Yb:YAG thin-disk laser oscillator operating in the strongly self-phase modulation broadened regime. Opt Express (2021) 29:35929–37. doi:10.1364/OE.440196
15. Liu H, Yang T, Xu J, Wang Q, Xuan H, Zhang J. High-power 55-fs Yb:YAG thin-disk oscillator at 200 MHz repetition rate. Front Phys (2022) 4:1–6. doi:10.3389/fphy.2022.1070826
16. Zhang J, Pӧtzlberger M, Wang Q, Seidel M, Bauer D, Sutter D, et al. Distributed kerr lens mode-Locked Yb:YAG thin-disk oscillator. Ultrafast Sci 2022(2022):9837592. doi:10.34133/2022/9837892
17. Baer CRE, Kränkel C, Heckl OH, Engqvist AG, Golling M, Südmeyer T, et al. 275-fs pulses from the first mode-locked Yb:LuScO3 thin disk laser. In: Advanced solid-state Photonics, OSA technical digest series (CD). Optica Publishing Group (2009). p. TuB17.
18. Baer CRE, Kränkel C, Heckl OH, Golling M, Südmeyer T, Peters R, et al. 227-fs pulses from a mode-locked Yb:LuScO3 thin disk laser. Opt Express (2009) 17:10725–30. doi:10.1364/OE.17.010725
19. Saraceno CJ, Heckl OH, Baer CRE, Golling M, Südmeyer T, Beil K, et al. SESAMs for high-power femtosecond mode-locking: Power scaling of an Yb:LuScO3 thin disk laser to 23 W and 235 fs. Opt Express (2011) 19:20288–300. doi:10.1364/OE.19.020288
20. Ricaud S, Jaffres A, Wentsch K, Suganuma A, Viana B, Loiseau P, et al. Femtosecond Yb:CaGdAlO4 thin-disk oscillator. Opt Lett (2012) 37:3984–6. doi:10.1364/OL.37.003984
21. Diebold A, Emaury F, Schriber C, Golling M, Saraceno CJ, Südmeyer T, et al. SESAM mode-locked Yb:CaGdAlO4 thin disk laser with 62 fs pulse generation. Opt Lett (2013) 38:3842–5. doi:10.1364/OL.38.003842
22. Paradis C, Modsching N, Wittwer VJ, Deppe B, Kränkel C, Südmeyer T. Generation of 35-fs pulses from a Kerr lens mode-locked Yb:Lu2O3 thin-disk laser. Opt Express (2017) 25:14918–25. doi:10.1364/OE.25.014918
23. Kitajima S, Shirakawa A, Yagi H, Yanagitani T. Kerr-lens mode-locked Yb:Lu2O3 ceramic thin-disk laser. In: Conference on lasers and electro-optics, OSA technical digest (online). Optica Publishing Group (2018). SF2N.5.
24. Zhang J, Mak KF, Nagl N, Seidel M, Bauer D, Pervak V, et al. Multi-mW, few-cycle mid-infrared continuum spanning from 500 to 2250 cm-1. Light: Sci Appl (2018) 7:17180. doi:10.1038/lsa.2017.180
25. Zhang J, Mak KF, Pronin O. Kerr-lens mode-locked 2-μm thin-disk lasers. IEEE J Selected Top Quan Electron (2018) 24:1–11. doi:10.1109/JSTQE.2018.2814780
26. Modsching N, Drs J, Fischer J, Paradis C, Labaye F, Gaponenko M, et al. Sub-100-fs Kerr lens mode-locked Yb:Lu2O3 thin-disk laser oscillator operating at 21 W average power. Opt Express (2019) 27:16111–20. doi:10.1364/OE.27.016111
27. Goncharov S, Fritsch K, Pronin O. 100 MW thin-disk oscillator. In: 2021 conference on lasers and electro-optics europe and European quantum electronics conference, OSA technical digest. Optica Publishing Group (2021). cf_4_1.
28. Wang H, Pan J, Meng Y, Liu Q, Shen Y. Advances of Yb:CALGO laser crystals. Crystals (2021) 11:1131. doi:10.3390/cryst11091131
29. Ma Y, Shen Y, Xu L, Zhou T, Lin W, Gao Q, et al. High-power continuous-wave dual-wavelength diode-pumped Yb:YAG slab amplifier with temperature-dependent effects. Appl Physicis B (2017) 123:258. doi:10.1007/s00340-017-6834-4
30. Kuznetsov I, Mukhin I, Palashov O, Ueda KI. Thin-rod Yb:YAG amplifiers for high average and peak power lasers. Opt Lett (2018) 43:3941–4. doi:10.1364/OL.43.003941
Keywords: high efficiency, high power, ultrashort pulses, thin disk, multi-pass
Citation: Chen H, Yan L, Liu H, Hao J, Yang T, Liu H, Zhang J, Li Q, Wang H, Zhu G, Zhu X, Xuan H, Wang Q and Zhang J (2023) High-efficiency 100-W Kerr-lens mode-locked Yb:YAG thin-disk oscillator. Front. Phys. 11:1191010. doi: 10.3389/fphy.2023.1191010
Received: 21 March 2023; Accepted: 11 April 2023;
Published: 24 April 2023.
Edited by:
Karol Krzempek, Wrocław University of Science and Technology, PolandReviewed by:
Haiyong Zhu, Wenzhou University, ChinaYijie Shen, University of Southampton, United Kingdom
Copyright © 2023 Chen, Yan, Liu, Hao, Yang, Liu, Zhang, Li, Wang, Zhu, Zhu, Xuan, Wang and Zhang. This is an open-access article distributed under the terms of the Creative Commons Attribution License (CC BY). The use, distribution or reproduction in other forums is permitted, provided the original author(s) and the copyright owner(s) are credited and that the original publication in this journal is cited, in accordance with accepted academic practice. No use, distribution or reproduction is permitted which does not comply with these terms.
*Correspondence: Hongwen Xuan, eHVhbmh3QGFpcmNhcy5hYy5jbg==; Jinwei Zhang, amlud2VpemhhbmdAaHVzdC5lZHUuY24=
†These authors have contributed equally to this work