- 1Helmholtz Zentrum Dresden Rossendorf, Dresden, Germany
- 2SLAC National Accelerator Laboratory, Menlo Park, CA, United States
A dependable and consistent electron source is a crucial requirement for the achievement of high-power free electron lasers (FELs). Over the past two decades, it has been demonstrated that photoinjectors based on SRF technology (SRF guns) are suitable for continuous wave (CW) beam generation. SRF guns possess both the high accelerating field gradients of normal conducting RF photoinjectors and the low power dissipation thanks to mature SRF cavity technology, and therefore have the potential to provide the high-brightness, high-current beams required for CW-XFELs. After the demonstration of the first SRF gun in Dresden-Rossendorf, several SRF gun programs based on different approaches have achieved promising progress and even succeeded in routine operation. SRF guns are expected to play an important role in XFEL facilities in the near future. In this paper, we give an overview of design concepts, important parameters and development status of the worldwide SRF gun projects.
1 Introduction
Superconducting radio frequency photoinjectors (SRF guns) have advantages for operating in continuous wave (CW) mode and generating the high-brightness and high-current beams required by CW X-ray free electron laser (XFEL) [1, 2] and energy recovery linac (ERL) facilities [3, 4]. Similar to the normal conducting rf guns, SRF guns are able to provide higher gradients on the cathode surface than DC guns, increasing the achievable surface current density and reducing the thermal emittance contribution for a given bunch charge; higher beam voltages help to mitigate space-charge effects as the beam travels from the gun to the next accelerator cavity. Concurrently, SRF guns have an outstanding vacuum environment for sensitive cathodes and potentially lower RF jitter compared to CW normal conducting (NC) photoinjectors [5].
From a technical perspective, an CW injector based on SRF technology is a complex device that not only presents challenges common to other types of high-brightness beam sources and superconducting accelerators, but also introduces new issues in both realms. In common with other injectors, in order to produce low emittance beams required by XFEL facilities, SRF guns must have high accelerating gradients, effective emittance compensation, precise synchronization between cathode drive laser and RF, application-appropriate photocathodes, proper laser pulse shaping, and so on. As with other SRF-based accelerators, an SRF gun utilizes superconducting RF cavities and cryostats, with requisite need for precise frequency control, field stabilization, etc. In addition to these issues, the geometry of SRF photoinjectors can introduce additional complexities regarding cleaning the SRF surface, RF power conduction along the cathode support, RF power coupling, and emittance compensation, all of which require new solutions. But the most consistently challenging aspect of SRF gun design has been cathode integration into an SRF structure.
In the last 20 years, SRF gun projects made excellent progress [6, 7]. All of them are based on the mature superconducting (SC) pure niobium cavity technology, with either high-frequency cavities based on the 1.3 GHz TESLA shape, or low frequency quarter wavelength resonator (QWR). The former is able to reach a higher field on axis and thus higher energy gain for the electrons, but the QWR cavity can produce a longitudinally homogeneous (DC-like) field profile. Two SRF guns are currently in user operation (HZDR SRF Gun-II) [8] or generate routine beams for experiments (BNL CeC SRF gun [9]). Furthermore, five gun projects have made promising R&D progress (DESY, SLAC, HZB, KEK and PKU) [10–14]. Among them, the DESY SRF gun and LCLS-II HE SRF gun are specially designed for CW-XFEL application. This overview paper focuses on the recent progress on these projects and compares their performance as potential injectors for CW-XFELs.
2 SRF guns developed for CW-FELS
2.1 DESY SRF gun (elliptical cavity with SC cathode)
A future upgrade of the European XFEL foresees High-Duty-Cycle (HDC) operation [15]. The first choice for the required CW injector is an SRF gun with high gradient [10], which is a 1.6 cell L-band cavity with a superconducting photocathode (lead-coated or nanostructured) directly installed in the cavity back wall (see Figure 1).
The elliptical L-band cavity allows the gun to operate at high accelerating gradient comparable to a NC RF L-band gun, which helps to generate the low emittance beam required by the CW-XFEL. In recent years, the DESY SRF Gun project has invested considerable effort in the treatment and modification of cavities and made significant progress. All recent prototypes achieved ∼55 MV/m peak on-axis gradients and the back wall is mechanically stable enough to withstand the high Lorenz force at this field [16]. With this gradient, the transverse emittance of 100 pC bunch change should be under 1 µm. The special feature of this design is that there is no provision for cathode transport and exchange in situ; the cathode exchange must be performed when the cavity is warmed up and in a clean room. The advantage of this design is removal of particulate contamination risk from the cathode exchange in a cold cavity. However, the integrated cathode needs to be extremely robust and exhibit a reasonable long lifetime to ensure the continuous user’s beam time. Meanwhile, after the cathode is installed into cavity, the cavity needs to be re-cleaned, which is a big challenge for the cathode robustness. If the cathode material migrates from the cathode surface onto the cavity wall, the particles might spoil the cavity and degrade drastically the achieved maximum RF field. Furthermore, another challenge of this design is the superconducting photocathode itself. Lead (Pb) coated on Nb substrate is chosen as one possibility because of its relatively high critical temperature. The treatment and photoemission of this material have been studied by the group of J. Smedley and T. Rao at BNL [17], later by R. Barday at HZB [18], and recently by J. Lorkiewicz at NCBJ [19]. After laser cleaning the QE of lead can reach 10–4 level at 258 nm. However, compared to the normal conducting photocathodes with QE values of 0.1%–10%, the low QE of lead cathode will increase the requirements for the cathode drive laser as well as the optical elements, and heating from the laser could potentially pose limitations to the maximum achievable average current.
Alternative photocathodes for the DESY SRF gun are in planning, e.g., metallic cathode (niobium or copper) with surface nanostructuring to improve the QE by plasmonic states [20]. Such cathodes are expected to be robust to established SRF cleaning procedures [21]. However, the intrinsic emittance and its optimization of the nanostructured cathode remain to be investigated.
Presently, the DESY SRF gun project is still under R&D. Before performing the first beam tests, the next essential step is to determine the acceptable range of cathode laser power through a series of measurement.
2.2 LCLS-II HE SRF gun (QWR cavity with NC cathode)
The LCLS-II-HE project [1] will double the beam energy of the LCLS-II linac, from 4 to 8 GeV. To fully realize the benefits of this energy upgrade, including saturated lasing at 20-keV X-ray energy, a factor-of-two lower beam emittance is required than that expected from the existing LCLS-II injector. As part of addressing this gap, an SRF Gun R&D program encompassing low mean transverse energy (MTE) cathode research, and high gradient QWR cavity development, has been started. SLAC has partnered with the Facility for Rare Isotope Beams (FRIB) at Michigan State University (MSU), Argonne National Laboratory (ANL) and Helmholtz-Zentrum Dresden Rossendorf (HZDR) to build a gun prototype (see Figure 2) [14]. The aim is to demonstrate stable CW operation at a cathode gradient of 30 MV/m. The first RF tests will be performed on a “blank” cavity (no provision for inserting a cathode plug) in 2023. A second, “prototype” QWR SRF gun cavity will be fabricated and tested with an on-cathode gradient of up to 30 MV/m with a full cathode system in place, but no active photocathode deposited on the cathode plug, towards the end of 2024. Finally, the intent is to fabricate a “production” gun, incorporating lessons-learned from the fabrication and test of the “blank” and “prototype” structures.
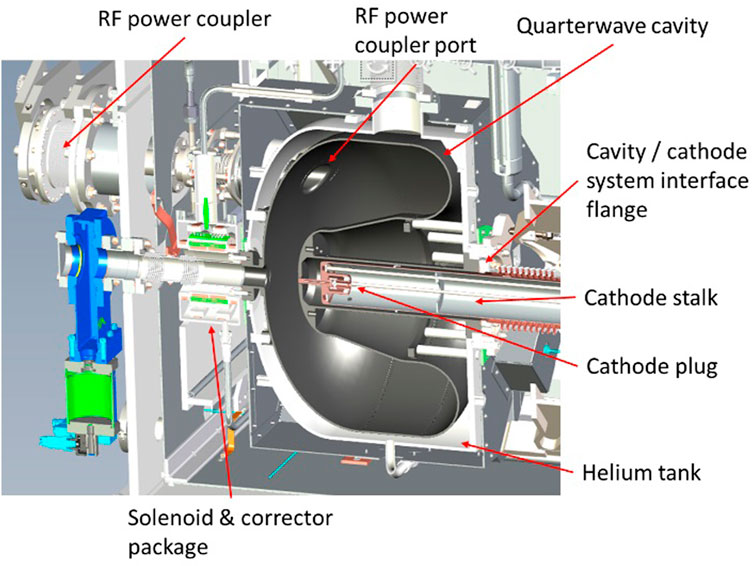
FIGURE 2. Design concept of LCLS-II HE SRF gun cavity with the cathode stalk [14].
The new gun is based around a “squat” QWR cavity profile, and a normal conducting photocathode in an rf choke, with an overall design inspired by, and drawing lessons-learned, from the NPS [22] and WiFEL designs [23]. In comparison, the SLAC/MSU QW SRF gun has a reduced cathode - anode distance and a thinner exit beam tube; this reduces the final beam energy, but retains most of the advantages of the “DC-like” beam transit through the gap, as exhibited by the VHF-type CW NCRF guns [24]. Also, the RF main coupler is placed on the downstream wall of the cavity (“anode plate”) rather than along the beamline. The RF frequency is 185.7 MHz (1/7 of 1300 MHz). The new gun should generate a beam with kinetic energy >1.6 MeV, bunch charge of 100 pC and, given a suitable photocathode, a transverse emittance <0.1 µm.
To reach the required low transverse emittance, a high-QE photocathode with a low mean transverse energy (MTE) < 0.2 eV is planned to be used. Combined with a small laser spot, the initial transverse emittance can be lower than 0.1 µm. The nominal design calls for using an “S20” photocathode (a Cs3Sb layer deposited on an underlying Na2KSb photocathode), illuminated at ∼500 nm (green). This represents both a challenge and an unknown, since there are no reported results of this cathode operating at 30 MV/m. Before more experimental experience about high-gradient performance–field emission and lifetime, as well as emittance–is available, such cathodes should not be used in the production gun. Metallic cathodes, such as copper, can be considered as the commissioning photocathode for the prototype gun.
Contrary to the DESY SRF gun with SC cathode, the SLAC/MSU gun is intended to use high-QE normal conducting (NC) photocathode, thus the photocathode drive laser can be simpler. However, the SLAC/MSU gun needs a more complex cathode system. A specially designed cathode stalk is under development at MSU. In addition to acting as an RF choke to reduce the RF loss in the cathode system, the cathode stalk is electrically and mechanically isolated from the SRF cavity. This allows to suppress multipacting between the cathode stalk and the gun with a DC bias. Mechanically, the stalk provides for fine adjustment of the cathode position to reach the best working point, and allows for the cathode to be maintained at either room or cryogenic (∼50 K) temperature [25]. The photocathode structure and the load-lock system adopt the HZDR design, which should ensure the particle-free and warm exchange ability.
This gun is a very promising injector for the SLAC XFEL project. The challenge for both the cavity and cathode is the high gradient of 30 MV/m on cathode and the low dark current requirement <10 nA during operation. For the future operation, reliable cathode exchange is another key point, as is the identification of a photocathode capable of meeting strict requirements for MTE, quantum efficiency, lifetime and field emission/dark current.
3 Other SRF guns with potential as CW-XFEL injectors
3.1 Elliptical cavities with RF chokes (HZDR, KEK, HZB)
3.1.1 HZDR SRF gun
Although the HZDR SRF gun was not developed as an injector for CW-XFEL, it represents a practical and successful CW SRF gun design. HZDR SRF gun-I was the first SRF gun worldwide that injected beams into an accelerator for CW IR-FEL production [26], and the Gun-II is the first one in user operation for CW super radiation THz source [8].
As shown in Figure 3, the cryostat of Gun-II includes the 3½ cell 1.3 GHz cavity and choke cell in the He vessel, the RF coupler, the SC solenoid and the cathode holding system. From 2017 to 2020 this gun was operated with a Mg cathode and since 2020 with Cs2Te cathode driven at 262 nm. The bunch charge injected into the ELBE linac can be up to 250 pC with repetition rates of 50–250 kHz. The drive laser is adjusted to a short rms pulse length of 2.3 ps. The accelerating gradient Eacc is 8 MV/m, with a corresponding field on the cathode of 14 MV/m, limited by the field emission and liquid He power consummation in the SRF cavity. The beam kinetic energy after an acceleration gap of 0.5 m can be as high as 4 MeV, which simplifies the beam transport to linac.
The successful operation of HZDR SRF gun benefits from its special cathode structure. The cavity gradient has not obviously degraded even though the cathode has been exchanged dozens of times. The cavity was recovered even after a Cs2Te cathode overheated and evaporated in the cavity in 2017.
Up to now, the main task of HZDR SRF gun has been to provide high bunch charge with sub-ps pulse lengths for the THz beamline at ELBE [27]. Thus, there are no systematic theoretical studies or demonstration experiments performed, which can prove the capability of this design to generate low emittance beam with moderate bunch charge, for example, 100 pC. One possible disadvantage as an XFEL injector might be its solenoid design. Both the large distance of 70 cm from the SC solenoid to the cathode and the coil design itself are not optimized for low emittance applications.
3.1.2 HZB SRF gun
The HZB-SRF gun is designed for the bERLinPro ERL project, which originally requires a very high CW beam current of 100 mA. The latest version is a 1.4-cell cavity with a choke cell, normal conducting bi-antimonite cathode and a SC solenoid at the cavity exit (see Figure 4) [11].
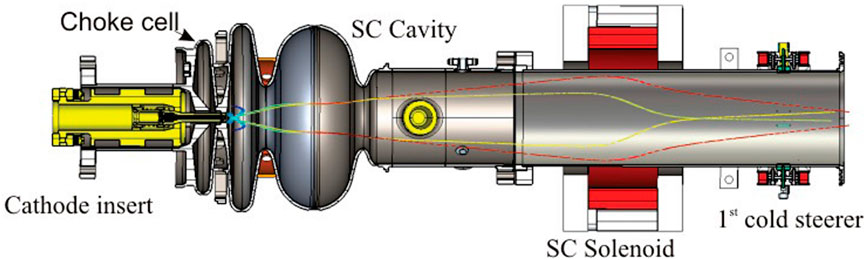
FIGURE 4. The cross section of the bERLinPro SRF gun cavity with an inserted cathode [11].
After the first successful beam test with a copper cathode in 2017, the cavity performance was found severely degraded due to the cathode unexpectedly overheating. Moreover, careful inspection of the cavity revealed surface damage close to the cathode opening to be the source of the measured dark current. A repair program was developed and a second gun cavity was built. Unfortunately, during the final high-pressure rinsing at the manufacturer, the cavity received unexpected damage again. By applying a combination of mechanical grinding, buffered chemical polishing, HF rinsing and an optimized high-pressure water rinsing both cavities could be brought back to nominal performance, without any field emission up to 30 MV/m peak on-axis field. Since end of 2022, the SRF gun has been reassembled in the cleanroom, and the cryo-module setup and integration has started in the bERLinPro accelerator hall during early 2023. It is planned to start commissioning of bERLinPro’s injector line only with the SRF gun operating, and to create the first beam with the 50 MHz photocathode laser system to measure the possible parameter space of this setup. Afterwards the injector will be completed by adding the booster, so that the full injector performance can be studied from short pulse low charge to high current and high charge.
Compared to the HZDR design, the HZB SRF gun has a bigger exit tube to let higher order modes (HOM) propagate to the HOM damper, thus its solenoid must also have a larger bore, which is reducing spherical aberration of the magnet. In addition, because of its 1.4 cell cavity, its SC solenoid sits closer to the cathode to provide early focusing. And the special solenoid holder based on a hexapod enables fine axis adjustment to minimize dipole- and quadrupole-field errors from misalignment of the solenoid magnet.
3.1.3 KEK SRF gun
The KEK SRF gun, still in the R&D phase, was developed as a source for the compact energy recovery linac (cERL) at KEK [3]. It consists of a 1.5 cell SC gun cavity with a choke cell (see Figure 5). The gun incorporates several unique design features. The cathode is cooled with liquid He. The cathode laser can illuminate the photocathode (bi-alkali antimonite) from the backside through a transparent superconductor substratum [28]. The vertical tests have been successfully performed at KEK, receiving a maximum surface peak electric field of ∼75 MV/m without cathode and over 60 MV/m with a niobium cathode plug [12]. Following the successful vertical test, the next step will be to install the gun cavity with the cathode rod in the horizontal cryostat and commence beam commissioning. This process was initiated at KEK in the last few years and will be continued at MSU in 2023.
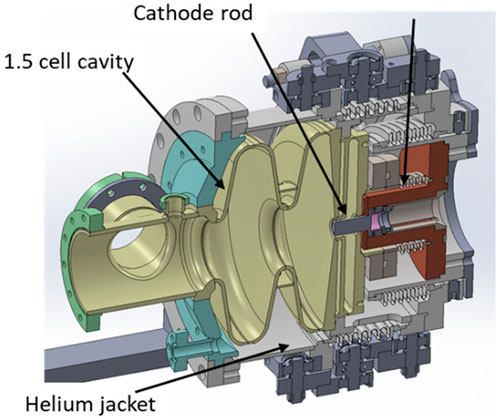
FIGURE 5. Cross section of the KEK SRF gun cavity with a cathode plug [12].
The obtained high gradient can help to obtain low emittance. Nevertheless, one concern is the cathode performance at such high gradient. Furthermore, the design of a transparent superconducting cathode plug coated with bi-alkali antimonite photo-emitter is very challenging, and the low operation temperature is not ideal for bi-alkali antimonite photocathode.
3.2 Elliptical cavities with NC cathodes in DC gun (PKU)
PKU’s SRF gun has a unique hybrid design, combining a compact DC gun with a 1.3 GHz cavity. This simplifies the cavity design and minimizes contamination risk from photocathode operation and exchange. However, a main concern is the limited electric field gradient on the cathode surface (∼6 MV/M) lower than that of the other SRF gun designs (>10 MV/m).
The last version of DC-SRF gun with 3.5 cell reached mA-level current in the pulsed mode operation in 2014 [29]. In 2021 the installation of the updated version DC-SRF-II has been finished, which aimed especially to improve the transverse emittance by increasing the electric field gradient on the cathode surface. The DC voltage is increased to 100 kV, with a corresponding field on cathode surface of 6 MV/m. A 1.5-cell SRF cavity with Eacc of 13–14 MV/m is adopted in this new version (see Figure 6) [30]. A K2CsSb photocathode is driven by a temporally- and spatially-shaped drive laser. The beam optics are also carefully designed for optimized emittance compensation [31]. The gun is intended to produce bunch charge of 100 pC with rms emittance as low as 0.33 µm, and repetition rate of 1 MHz. Commissioning of the new gun was successful. The gun was operated with the expected RF field gradient of 13 MV/m CW, with dark current < 1 pA; the normalized emittance of the delivered electron beam was measured as 0.85 µm @ 100 pC [13].
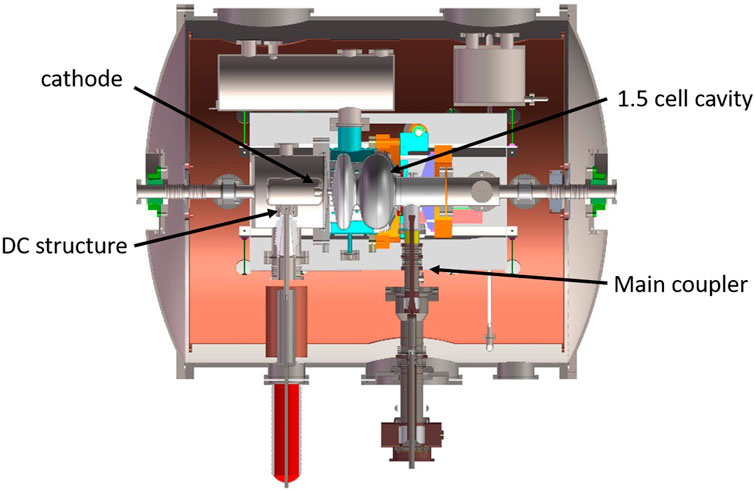
FIGURE 6. Design concept of the new PKU DC-SRF gun with 1.5 cell cavity and a cathode in the DC gap.
One of the design tasks of this DC-SRF-II is a potential injector for CW-XFEL. The advantages of this design include separation of cathode and SRF cavity, reducing the potential for contamination of cathode to cavity, cathode overheating, and multipacting due to migration of cathode material. No complex cathode choke structure is required, but a complicated high voltage part must be operated in the cryostat. Meanwhile, although the RF leakage through the hole on the cavity back wall has been excluded, the free drift space between the DC gap and the cavity may increase the problem of space charge effects at low beam energies. Additionally, no published reports exist on the analysis of the solenoid field utilized in this project, nor on the dipole and quadrupole correctors for the solenoid field. These factors are crucial for achieving a low emittance injector, highlighting the need for further investigation and documentation in this topic.
3.3 QWR cavity with NC cathode in choke filer (BNL)
The BNL SRF gun was built for the Coherent electron Cooling (CeC) proof of principle experiment at the Relativistic Heavy Ion Collider (RHIC), and has been in routine operation since 2016 [32]. The gun consists of a 112 MHz quarter wavelength resonator (QWR) and a room temperature K2CsSb cathode cooled with water (see Figure 7). The typical cathode lifetime for the high bunch charge (∼nC) operation is one to 2 months [33]. Intensive multipacting studies have been performed for this gun, resulting in a procedure for crossing the multipacting barriers from zero to the operational voltage 1.2 MV [34].
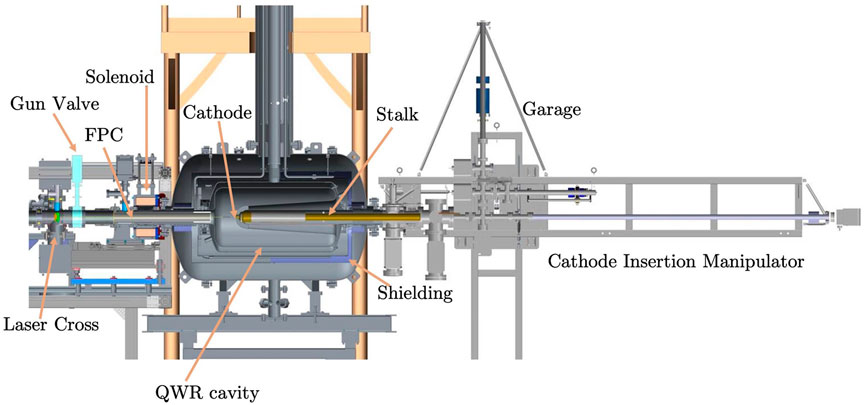
FIGURE 7. Assembly overview of the BNL QWR SRF gun and cathode insertion system [35].
The BNL 112 MHz SRF gun has shown its outstanding performance as a high current CW operation injector [9]. The gun demonstrated the ability to deliver low emittance electron bunches, e.g., 0.15 mm-mrad slice emittance for 100 pC, albeit the bunch length of 400 ps (required by CeC application) before compression is about twenty times longer than the pulse length required by the XFEL. The low electron energy at the gun exit (1.25 MeV) also mandates a careful beam transport design.
One concern for long-term user facility operation is cavity performance degradation over time, especially due to the cathode exchange. Special care is needed when manipulating the insertion mechanism to correctly install the cathode, as incorrect manipulation could lead to unexpected field emission and new multipacting barriers [35]. Though the He conditioning technique has proven to be an effective method for cavity restoration, the cathode lock and exchange system still poses a potential risk to the facility’s operating lifetime. Therefore, it is essential to implement additional measures to minimize the risks associated with cathode exchange and ensure the smooth operation of the facility in the long run.
4 Photocathode integration into SRF guns
Providing a stable beam with high current and low emittance for CW-XFEL facility, requires high accelerating gradients on the photocathode, high QE, low mean transverse energy (MTE), and long lifetime. A big challenge for SRF guns is how to best place the selected cathode in the fields in the SRF cavity. In NC RF guns, direct electrical and mechanical contact between the cathode and cavity is typically done via an RF spring, but for SRF guns this approach is problematic due to particle generation and, potentially, the need to keep the cathode at a substantially higher temperature than the gun cavity. Several concepts of cathode assembly and insertion have been successfully applied in the SRF guns [36], but this remains one of the major challenges to SRF gun development and operation.
4.1 SC cathodes on the cavity back wall
Intuitively a superconducting cathode is well-matched to the SC RF cavity. The superconducting cathode material (for example, Pb or Nb) can be directly coated or sealed onto the cavity back wall [37, 38], or the well cleaned cavity back wall can be used as photocathode [39]. The advantage of this method is that the cavity and cryostat design can be simplified because no special cathode assembly or RF choke structure is required. However, for applications demanding high average beam current, such as most CW-XFELs, the low quantum efficiency presents the risk of overheating the cathode and cavity from the required laser power, and the high work functionrequires challenging high-power UV drive lasers.
Using this method has a significant drawback - the SRF gun needs to be moved back to the cleanroom if the cathode needs to be replaced. This can result in increased machine downtime, high maintenance costs, and a new risk of cavity contamination when either the entire SRF cavity needs to be replaced or the cavity needs to be cleaned again.
4.2 RF choke for utilizing normal conducting photocathodes
To operate high-QE normal conducting photocathode in a SC RF gun, a thermal isolation between the NC cathode and SC cavity, for example, a vacuum gap, must be induced. Even if the cathode material can be operated effectively at 2–4 K, the cathode substrate itself presents the risk of dissipating too much RF power and quenching the cavity. Therefore, the design of the SC cavity and cryostat becomes more complex. Cathode holders (stalks) are usually designed as RF chokes in order to minimize the RF loss in this vacuum gap. Various RF choke designs suitable for the 1.3 GHz elliptical cavity guns [3, 8, 11] and for the QWR cavity guns [9, 14, 23]have been reported.
Because of the isolation from the SRF gun body, the photocathode can operate at different temperatures, cooled with liquid helium (KEK SRF gun), liquid nitrogen (HZDR, HZB SRF gun), low- or room-temperature He gas (SLAC SRF gun) or with water (BNL SRF gun). This unique variable provides scientists a possibility to obtain the lowest mean transverse energy (MTE) as well as a good QE of the photocathode by controlling the working temperature.
The multipacting phenomena is an inevitable problem, especially given the sensitivity of high-QE photocathodes to the vacuum environment. In the area of cathode, if the cathode is electrically isolated from the SC cavity, a DC bias can be applied on the cathode, which helps to suppress the possible MP resonance [25, 40] between the cathode stalk and body of the gun. Multipacting within the gun itself is still a concern, but this can be addressed both by the initial cavity design and by conditioning the cavity prior to photocathode insertion.
4.3 DC gap for normal conducting cathodes
As introduced in Section 3.2, a NC cathode can be integrated to the SRF cavity through a DC gap. Compared to the other RF choke designs, the main advantages of this design are greatly reduced chance of contamination from cathode to the SC cavity, and no RF heating of cathode. But this design sacrifices the electrical field strength on cathode surface. Meanwhile, the free drift space between the DC gap and the cavity back wall enhances the difficulty of space charge control and thus the following emittance compensation. Nevertheless, this approach continues to be intriguing for the low-medium bunch charge CW photoinjector.
5 Summary and outlook
Benefitting from the fast development of both SC RF cavity and photocathode technology, SRF photoinjectors are becoming rather promising as potential electron sources for CW-XFEL facilities. Over the last several years SRF guns made excellent progress, even realizing routine operation (see parameters overview in Table 1). The transverse emittance of 100 pC bunch charge can reach sub-µm level in simulation and even in experiments. Different from the NC RF guns, the solenoids for the SRF guns have to be placed outside the cavity area, but a suitable solenoid design can still efficiently improve the emittance. The idea to compensate the transverse emittance with an additional TE mode exited in the cavity might be an applicable solution to the long-distance limitation [41].
Besides the first-rate niobium cavity quality and delicate cryostat design, proper cathode system design is a key for successful gun operation. Various solutions for cathode integration have shown to be reliable, and new design concepts continue to emerge. CsK2Sb has shown its good lifetime in SRF guns, and it is believed the best candidate as low emittance photocathode required by the low emittance beam for XFELs. Present efforts to improve gun performance include increasing the cavity gradient/cathode field in operation while maintaining low dark current, as well as to further improve the robustness and safety of selected photocathodes.
Author contributions
RX wrote the first draft of the manuscript. JL und AA modified the manuscript. All authors contributed to manuscript revision, read, and approved the submitted version.
Acknowledgments
Many thanks to the colleagues, who provided materials on their projects for this review: I. Petrushina (BNL, SBU), E. Vogel (DESY), A. Neumann (HZB), T. Konomi (FRIB), and S. Huang (PKU).
Conflict of interest
The authors declare that the research was conducted in the absence of any commercial or financial relationships that could be construed as a potential conflict of interest.
Publisher’s note
All claims expressed in this article are solely those of the authors and do not necessarily represent those of their affiliated organizations, or those of the publisher, the editors and the reviewers. Any product that may be evaluated in this article, or claim that may be made by its manufacturer, is not guaranteed or endorsed by the publisher.
References
1. Raubenheimer TO. The LCLS-II-HE, A high energy up-grade of the LCLS-II. In: Proc. 60th ICFA Advanced Beam Dynamics Workshop on Future Light Sources (FLS'18); 5-9 March 2018; Shanghai, China (2018). p. 6–11.
2. Decking W. A MHz-repetition-rate hard X-ray free-electron laser driven by a superconducting linear accelerator. Nat Photon (2020) 14(6):391–7. doi:10.1038/s41566-020-0607-z
3. Konomi T, Honda Y, Kako E, Kobayashi Y, Michizono S, Miyajima T, et al. Development of high intensity, high brightness CW SRF gun in KEK. In: Oral talk for 19th International Conference on RF Superconductivity (SRF 2019); 30 June-5 July 2019; Dresden, Germany (2019).
4. Abo-Bakr M. Status report of the berlin energy recovery linac project BERLinPro. In: Proc. 9th Int. Particle Accelerator Conf. (IPAC'18); 29 Apr - 4 May 2018; Vancouver, Canada (2018).
5. Qian H, Vogel E. Overview of CW RF guns for short wavelength FELs. In: 39th International Free-Electron Laser Conference (FEL2019); August 26-30, 2019; Hamburg, Germany (2019).
6. Arnold A, Teichert J. Overview on superconducting photoinjectors. Phys Rev Spec Top - Acc Beams (2011) 14(2):024801. doi:10.1103/physrevstab.14.024801
7. Xiang R. Review of superconducting radio frequency gun. In: 12th Int. Particle Accelerator Conf.(IPAC’21); 24 - 28 May 2021; Campinas, Brazil (2021).
8. Teichert J, Arnold A, Ciovati G, Deinert JC, Evtushenko P, Justus M, et al. Successful user operation of a superconducting radio-frequency photoelectron gun with Mg cathodes. Phys Rev Acc Beams (2021) 24(3):033401. doi:10.1103/physrevaccelbeams.24.033401
9. PetrushinaI I, Litvinenko1 VN, Jing Y, Ma J, Pinayev I, Shih K, et al. High-brightness continuous-wave electron beams from superconducting radio-frequency photoemission gun. Phys Rev Lett (2020) 124(24):244801. doi:10.1103/physrevlett.124.244801
10. Vogel E, Barbanotti S, Brinkmann A, Buettner T, Iversen J, Kay J, et al. Status of the all superconducting gun cavity at DESY. In: 19th Int. Conf. RF Superconductivity (SRF'19); 30 Jun - 05 Jul 2019; Dresden, Germany (2019).
11. Neumann A, Böhlick D, Bürger M, Echevarria P, Frahm A, Glock H-W, et al. The berlinpro srf photoinjector system - from first Rf commissioning to first beam. In: 9th International Particle Accelerator Conference ( IPAC2018); 29 Apr - 4 May 2018; Vancouver, BC, Canada (2018).
12. Konomi T, Honda Y, Kako E, Kobayashi Y, Michizono S, Miyajima T, et al. Development of high intensity, high brightness, CW SRF gun with Bi-alkali photocathode. In: 19th Int. Conf. RF Superconductivity (SRF'19); 30 Jun - 05 Jul 2019; Dresden, Germany (2019).
13. Huang S, Ouyang D, Wang F, Jiao F, Zhu F, Wang G, et al. Continuous-wave operation of a low-emittance DC-SRF photocathode gun (WEAO3). In: 40th International Free Electron Laser Conference (FEL2022); August 22-26 2022; Trieste, Italy (2022).
14. Lewellen J, Adolphsen C, Coy R, Ge L, Ji F, Murphy M, et al. Status of the SLAC/MSU SRF gun development project. In: North American Particle Accelerator Conference 2022; 7-12 August 2022; Albuquerque, New Mexico, USA (2022).
15.DESY. TS4I | SRF photoinjector test stand Ts4i (2023). Available at: https://ts4i.desy.de/motivation/index_eng.html.
16. van der Horst B, Klinke D, Muhs A, Schmökel M, Sekutowicz J, Sievers S, et al. Development and adjustment of tools for supercondcuting rf gun cavities. In: 20th Int. Conf. on RF Superconductivity SRF2021; 28 June-2 July 2021; East Lansing, MI, USA (2021).
17. Smedley J, Rao T, Sekutowicz J. Lead photocathodes. Phys Rev ST Accel Beams (2008) 11:013502. doi:10.1103/physrevstab.11.013502
18. Barday R, Burrill A, Jankowiak A, Kamps T, Knobloch J, Kugeler O, et al. Characterization of a superconducting Pb photocathode in a superconducting rf photoinjector cavity. Phys Rev Spec Topics-Accelerators Beams (2013) 16(12):123402. doi:10.1103/physrevstab.16.123402
19. Lorkiewicz J, Nietubyć R, Diduszko R, Sekutowicz J, Kosińska A, Mirowski R, et al. Coating in ultra-high vacuum cathodic-arc and processing of Pb films on Nb substrate as steps in preparation of Nb-Pb photocathodes for radio-frequency, superconducting e-guns. Vacuum (2020) 179:109524. doi:10.1016/j.vacuum.2020.109524
20. Li RK, To H, Andonian G, Feng J, Polyakov A, Scoby CM, et al. Surface-plasmon resonance-enhanced multiphoton emission of high-brightness electron beams from a nanostructured copper cathode. Phys Rev Lett (2013) 110:074801. doi:10.1103/physrevlett.110.074801
22. Harris JR, Ferguson KL, Lewellen JW, Niles SP, Rusnak B, Swent RL, et al. Design and operation of a superconducting quarter-wave electron gun. Phys Rev Spec Topics-Accelerators Beams (2011) 14(5):053501. doi:10.1103/physrevstab.14.053501
23. Legg R, Bisognano J, Bissen M, Bosch R, Eisert D, Fisher M, et al. Status of the Wisconsin srf gun. In: Proceedings of IPAC2012; May 2-25, 2012; New Orleans, Louisiana, USA (2012).
24. Schmerge JF, Brachmann A, Dowell D, Fry A, Li RK, Li Z, et al. The LCLS-II injector design. In: Proceedings of the 2014 International Free-Electron Laser Conference; 25-29 August 2014; Basel, Switzerland (2014).
25. Konomi T, Hartung W, Kim S, Miller S, Morris D, Popielarski J, et al. Design of the cathode stalk for the LCLS-II-HE low emittance injector. In: 5th North American Particle Accel. Conf. (NAPAC2022); August 6-12, 2022; Albuquerque, NM, USA (2022).
26. Teichert J, Arnold A, Büttig H, Justus M, Kamps T, Lehnert U, et al. Free-electron laser operation with a superconducting radio-frequency photoinjector at ELBE. Nucl Instrum Methods Phys Res Sect A (2014) 743:114–20. doi:10.1016/j.nima.2014.01.006
27. Lu P, Arnold A, Teichert J, Vennekate H, Xiang R. Simulation of ELBE SRF gun II for high-bunch-charge applications. Nucl Instr Methods Phys Res A (2016) 830:536–44. doi:10.1016/j.nima.2016.05.087
28. Konomi T, Honda Y, Kako E, Kobayashi Y, Michizono S, Miyajma T, et al. Development of SRF gun applying new cathode idea using a transparent superconducting layer. In: 59th ICFA Advanced Beam Dynamics Workshop on Energy Recovery Linacs (ERL17); 18-23 June 2017; Geneva, Switzerland (2017).
29. Quan S, Hao J, Lin L, Zhu F, Wang F, Feng L, et al. Stable operation of the DC-SRF photoinjector. Nucl Instr Methods Phys Res Section A: Acc Spectrometers, Detectors Associated Equipment (2015) 798:117–20. doi:10.1016/j.nima.2015.07.025
30. Liu YQ, Chen M, Huang S, Lin L, Liu KX, Quan SW, et al. Engineering design of low-emittance DC-SRF photocathode injector. In: 39th Free Electron Laser Conference (FEL2019); August 26-30, 2019; Hamburg, Germany (2019).
31. Tan T, Jia H, Zhao S, Li T, Wang T, Liu Z, et al. Sub-micron normalized emittance measurement for a MeV continuous-wave electron gun. Nucl Instr Methods Phys Res Section A: Acc Spectrometers, Detectors Associated Equipment (2023) 1045:167552. doi:10.1016/j.nima.2022.167552
32. Litvinenko V, Altinbas Z, Anderson R, Belomestnykh S, Boulware C, Brown K, et al. Commissioning of FEL-based coherent electron cooling system. In: 38th Int. Free Electron Laser Conf. (FEL'17); 20-25 August 2017; Santa Fe, NM, USA (2017).
33. Wang E, Litvinenko VN, Pinayev I, Gaowei M, Skaritka J, Belomestnykh S, et al. Long lifetime of bialkali photocathodes operating in high gradient superconducting radio frequency gun. Scientific Rep (2021) 11(1):4477–9. doi:10.1038/s41598-021-83997-1
34. Petrushina I, Litvinenko VN, Pinayev I, Smith K, Narayan G, Severino F. Mitigation of multipacting in 113 MHz superconducting rf photoinjector. Phys Rev Acc Beams (2018) 21(8):082001. doi:10.1103/physrevaccelbeams.21.082001
35. Petrushina I, Jing Y, Litvinenko VN, Ma J, Severino F, Narayan G, et al. First experience with He conditioning of a superconducting rf photoinjector. Phys Rev Acc Beams (2022) 25(9):092001. doi:10.1103/physrevaccelbeams.25.092001
36. Xiang R, Jan S. Review of recent progress on advanced photocathodes for superconducting RF guns. Micromachines (2022) 13(8):1241. doi:10.3390/mi13081241
37. Vogel E, Barbanotti S, Brinkmann A, Buettner T, Iversen J. Status of the all superconducting gun cavity at desy. In: 19th Int. Conf. on RF Superconductivity; 30 Jun - 05 Jul 2019; Dresden, Germany (2019).
38. Hannon F, Andonian G, Harris L. A plasmonic niobium photocathode for srf gun applications. In: 10th Int. Particle Accelerator Conf. IPAC2019; 19 - 24 May 2019; Melbourne, Australia (2019).
39. Liu A, Kostin R, Jing C, Avrakhov P. Optimization of an srf gun design for uem applications. In: North American Particle Acc. Conf (NAPAC2019); 1—6 September 2019; Lansing, MI, USA (2019).
40. Tulu ET, van Rienen U, Arnold A. Systematic study of multipactor suppression techniques for a superconducting rf gun. Phys Rev Acc Beams (2018) 21(11):113402. doi:10.1103/physrevaccelbeams.21.113402
Keywords: superconducting RF, photoinjector, continues wave, FEL, low emittance, SRF gun
Citation: Xiang R, Arnold A and Lewellen JW (2023) Superconducting radio frequency photoinjectors for CW-XFEL. Front. Phys. 11:1166179. doi: 10.3389/fphy.2023.1166179
Received: 14 February 2023; Accepted: 27 March 2023;
Published: 12 April 2023.
Edited by:
Nicholas Walker, Helmholtz Association of German Research Centres (HZ), GermanyReviewed by:
Jacek Sekutowicz, Helmholtz Association of German Research Centres (HZ), GermanyMathieu Omet, High Energy Accelerator Research Organization, Japan
Copyright © 2023 Xiang, Arnold and Lewellen. This is an open-access article distributed under the terms of the Creative Commons Attribution License (CC BY). The use, distribution or reproduction in other forums is permitted, provided the original author(s) and the copyright owner(s) are credited and that the original publication in this journal is cited, in accordance with accepted academic practice. No use, distribution or reproduction is permitted which does not comply with these terms.
*Correspondence: R. Xiang, ci54aWFuZ0BoemRyLmRl