- 1Key Laboratory of Micro and Nano Heat Fluid Flow Technology and Energy Application, School of Physical Science and Technology, Suzhou University of Science and Technology, Suzhou, China
- 2College of Science, University of Shanghai for Science and Technology, Shanghai, China
- 3Key Laboratory of Advanced Micro-structure Materials, Ministry of Education, School of Physics Science and Engineering, Tongji University, Shanghai, China
In this article, we present a miniaturized electromagnetic modulator based on electrically controllable spontaneous-emission-cancellation-like (SEC-like) effect in meta-molecule. The SEC-like meta-molecule with in-unit destructive interference interaction is constructed by two detuned side-coupled resonators based on zero-index-metamaterial The subwavelength ZIM-based resonators, regarding as meta-atoms, are arranged symmetrically in a cut microstrip. A diode serving as an adjustable resistor is embedded in the gap of microstrip to inductively tune the interference of two ZIM-based meta-atoms. Numerical simulations indicate that the remarkable modulation on the SEC-like spectrum can be realized by changing the resistance from 9,000 Ω (unconnected) to 10 Ω (connected). Microwave experiments validate the electromagnetic modulation in three narrow bands on the SEC-like spectrum, and a peak modulation contrast of 52.1 dB on the transmission at 2.59 GHz is achieved through electric biasing. The results in this work may pave the way for our design to be applied in new integrated active devices and applications.
1 Introduction
Electromagnetic (EM) modulator is a device typically used to control the characteristic properties of electromagnetic (EM) waves [1–8]. EM modulator has a great potential for applications in on-chip optical interconnects and communication systems, including but not limited to the polarization converters [1–3], speed regulation [4, 5], intensity modulated absorber [6, 7], and band-pass/stop filters [8]. The three instrumental factors for EM modulator performance are multi-band modulation, large modulation-depth, and miniaturized device volume. To this end, considerable efforts have been devoted and various types of functional materials have been employed [9–14]. Some representative schemes, like exploiting two-dimensional graphene sheets [9–11], using metal strips as electrical resonators with power feeds at their ends [12, 13], and integrating photo-active silicon islands into functional unit cells [14], are proposed to make EM modulator for practical applications in functional optical devices. However, on one hand, the existing designs mentioned above often come at the expense of bandwidth or structure, while others suffer from severe limitations in material properties, complicated manufacturing processes, and insignificant modulation effects. On the other hand, although active modulators in the optical realm are initially established, the low-frequency region is still in great demand for highly efficient active EM modulator, especially in the GHz frequency regime where the wavelength is large enough. Therefore, to circumvent these problems, some auxiliary subwavelength designs, like metamaterials or the related meta-molecule, should be introduced.
Metamaterials are artificial micro-structured materials with unique properties not attainable in nature [15, 16]. These artificial materials are usually composed of subwavelength-sized metallic resonant units as meta-atoms and can be designed to exhibit required values of permittivity and permeability in the desired frequency range. Among them, zero-index metamaterials (ZIMs) characterized by zero effective permittivity and permeability simultaneously, have come into sight and been actively explored. The exotic physical characteristics of ZIMs yield a variety of special EM effects, such as squeezing light wave energy [17–19], realizing high-gain and high-directivity antennas [20, 21], attaining directive radiation patterns [22], and EM cloaking [23–25]. In particular, based on effective zero-index media, the size of EM resonator is no longer restricted by the half-wavelength limit [26]. And hence, metamaterials, including ZIM-based resonator, can be well regarded as “meta-atom” and rationally engineered to form meta-molecules that exhibit some quantum optical phenomena analogue to atomic systems. For example, the spectral characteristics of electromagnetically induced transparency (EIT) have been reproduced in different types of meta-molecules, demonstrating well the low absorption and steep dispersion effects [27–30]. In spontaneous emission cancellation (SEC) analogue, the SEC-like effect is achieved in a meta-molecule by properly tailoring the destructive interference of two different emitting meta-atoms [31, 32]. It is noteworthy that, in contrast to the EIT analogue, the SEC-like effect realized by meta-molecules has an extremely deep stop-band in the transmission spectrum [33–35]. Up to now, a large number of researches have confirmed that it is feasible to realize EM modulators in actively controlled EIT-like meta-molecules [36–40]. However, the electrically controlled SEC-like effect for multi-frequency and high-performance EM modulating is still not reported.
In this paper, we propose to electrically control the SEC-like spectrum in a meta-molecule as an EM modulator. Two detuned side-coupled meta-atoms, namely ZIM-based resonators, are arranged symmetrically in a cut microstrip to construct a meta-molecule with SEC-like in-unit interaction. A PIN diode behaving as an adjustable resistor is embedded in the gap of microstrip. By electrically biasing the diode, the destructive interference between two meta-atoms can be finely tuned, which induces remarkable modulation on the SEC-like spectrum. Numerical simulations indicate that the transmission of EM waves at three characteristic peak/dips of the SEC-like spectrum can be effectively modulated by changing the resistance from 9,000 Ω (unconnected) to 10 Ω (connected). Microwave experiments confirm that the prediction of electromagnetic modulation in three narrow bands on the SEC-like spectrum, and a peak modulation contrast of 52.1 dB on the transmission at 2.59 GHz is achieved through electric biasing. Our findings may be useful as a reference for the future study of electrically controlled classic analogues to quantum interference phenomena in coherent media.
2 Model design
To illustrate our idea more explicitly, a simple schematic is demonstrated in Figure 1. As shown in Figure 1A, an upper meta-atom1 is coupled with a discontinuous single-mode waveguide, resulting in a transparent resonance. This phenomenon is similar to a two-level atomic system, where the atom can transition from an excited state to a ground state under certain conditions (see Figure 1B). For the single-mode waveguide coupled with only a lower meta-atom2, the same physical mechanism occurs, yield another typical resonance peek in transmission (see Figures 1C, D). Owing to their resonant frequencies are close to each other, we set the two detuned meta-atoms symmetrically in the cut waveguide to resemble the original physical mechanism of SEC (see Figure 1E). As is known, the SEC effect is the result of quantum destructive interference between two direct excitation pathways in a three-level V-type atomic system [31]. When two different excited states
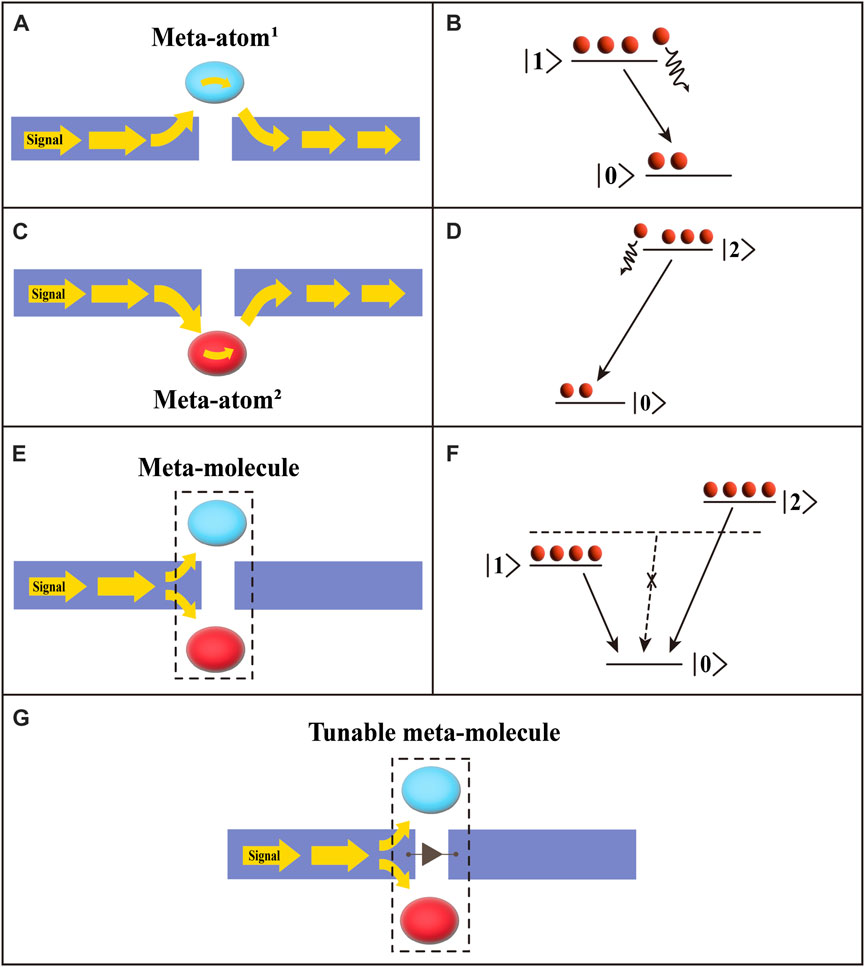
FIGURE 1. Schematic diagram of (A) an upper side-coupled meta-atom1 analogous to (B) a two-level atomic system. Schematic diagram of (C) another lower side-coupled meta-atom2 analogous to (D) a two-level atomic system with a different excited state. Schematic diagram of (E) a meta-molecule consisting of two coexistent symmetrically side-coupled meta-atoms, analogous to (F) a three-level V-type atomic system. (G) Schematic diagram of a tunable SEC-like meta-molecule.
Figure 2 depicts a proof-of-principle demonstration of the tunable SEC-like molecule, which composes of a pair of ZIM-based resonators with different resonant frequencies. The two side-coupled ZIM-based resonators serving as meta-atoms are fabricated on the microstrip transmission line by loading lumped-element series capacitances and shunt inductances. The length and width of the entire structure are 60 mm and 20 mm, respectively. The width of the microstrip line used here is w = 2.72 mm, leading to a characteristic impedance of Z0 = 50 Ω. The sample is fabricated on a 1.0-mm-thick F4B substrate. The permittivity and loss tangent of the F4B substrate are εr = 2.65 and 0.001, respectively. A copper layer with a thickness of 0.035 mm is attached to the bottom of the substrate. The schematic and circuit models of the ZIM-based meta-atom with loading series capacitor and shunt inductors are given in Figures 2B, C. It is well-known that, an effective ZIM can be realized in a balanced composite right-left handed (CRLH) transmission line at a certain frequency [26, 41]. Accordingly, the effective relative permittivity and permeability of CRLH microstrip line are determined by using the following formula:
where C0 and L0 each describe the distributed parameters of microstrip line, d0 denotes the unit length of negative index materials, p is the structure constant given by
where d describes the unit length of the normal microstrip transmission line. The length of the ZIM-based meta-atoms is d = 20 mm, which is less than 1/7 of the wavelength in vacuum at 2.16 GHz. The distance between ZIM-based meta-atoms and microstrip line is set to be 0.2 mm. The values of all the capacitances used in this paper are chosen as C = 2.5 pF. Thus, the resonant frequency of ZIM-based meta-atoms is mainly determined by the values of two shunt inductances L1 and L2. A PIN diode (model: SMP1345) is soldered at the middle gap of microstrip line to and is biased through two copper wires. Numerical simulations are carried out by a commercial software CST Microwave Studio. In experiments, a vector network analyzer Keysight 85033E and a DC voltage source Keysight E3640A are used to obtain the electrically controlled modulating response.
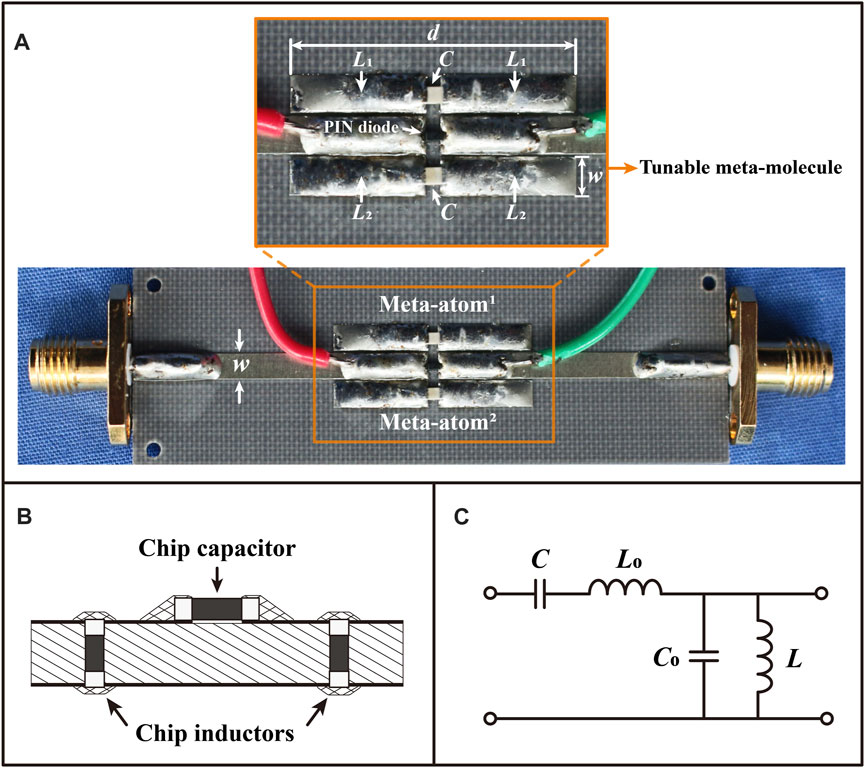
FIGURE 2. (A) Photograph of the fabricated electrically tunable SEC-like molecule. The (B) schematic model and (C) circuit model of ZIM-based meta-atom with the loading chip capacitor and chip inductors.
3 Results and discussions
To clearly illustrate the SEC-like effect in meta-molecule, the transmission spectra of the individual and coexisting side-coupled ZIM-based meta-atoms are simulated and depicted in Figure 3. Here, we choose L1 = 3 nH for meta-atom1 and L2 = 3.9 nH for meta-atom2. In this case, the effective zero-index condition can be satisfied at two separated resonant frequencies of fI = 2.16 GHz and fIII = 2.42 GHz. For a cut microstrip transmission line, a sharp and deep stopband emerges at fII = 2.26 GHz between the two individual resonant transmission peaks, explicitly demonstrating the SEC-like destructive interference effect, as shown in Figure 3A. While for a continuous microstrip, the situation is completely opposite. It is found that the two detuned ZIM-based meta-atoms instead behave as radiative resonators. As we connect the gap in the middle of the microstrip line, the original transmission dip becomes a transparency window in the spectrum separating by two narrow radiative resonant dips, as shown in Figure 3B. This phenomenon is often referred to as a direct analogue of EIT quantum destructive interference effect in a V-type atom. Moreover, group delays of the above two meta-molecules are also simulated, as shown in Figures 3C, D. It is clear that, the deep negative group delay effect near the SEC-like frequency can be transformed to a large EIT-like slow-light effect by altering the microstrip line from unconnected to connected. Take the case of a diode as an adjustable resistor, by changing the “on/off” state of the microstrip line, a remarkable modulation on the SEC-like spectrum can be directly achieved.
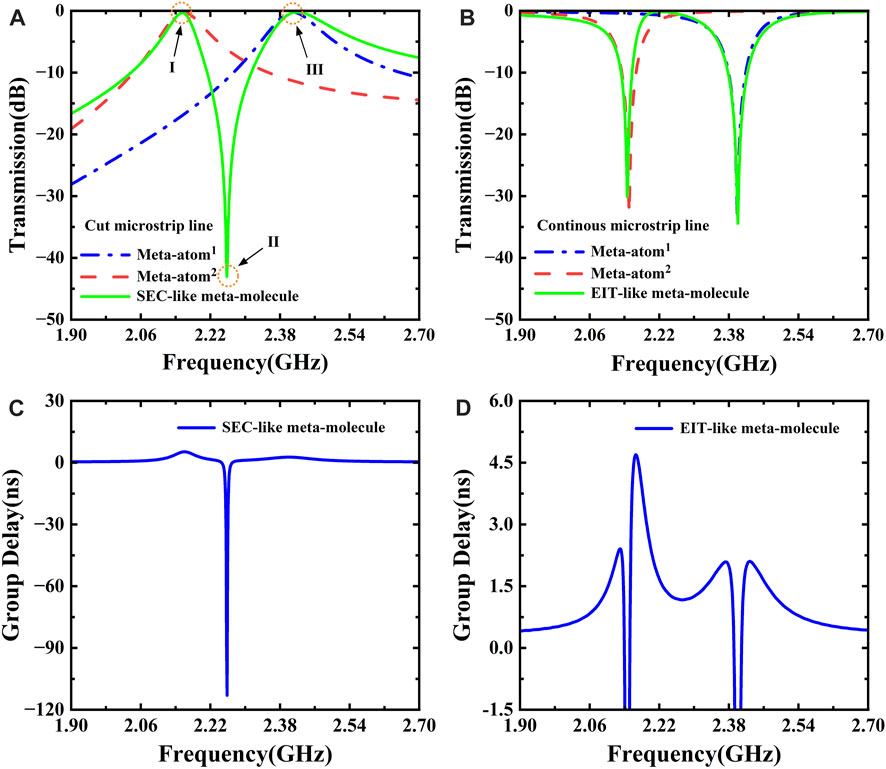
FIGURE 3. Simulated transmission spectra of the ZIM-based meta-atoms and the related combinative meta-molecule in a (A) cut and (B) continuous microstrip transmission line. The group delay of (C) SEC-like meta-molecule and (D) EIT-like meta-molecule.
To explore the underlying physics of SEC-like interference behavior of two parallelly side-coupled ZIM-based meta-atoms in a cut microstrip line, the electric energy density distributions at frequencies corresponding to the resonant features I, II and III marked in Figure 3A are calculated and figured out in Figure 4. The results demonstrate clearly that at the frequency fI (or fIII), a predominant cavity resonance is formed in either upper or lower ZIM-based meta-atom. In such situation, the incident waves can tunnel efficiently. While at the frequency fII, the situation is completely opposite. It is found that both ZIM-based meta-atoms are excited, but oscillate in opposite phase yielding almost all EM waves are blocked in the compact meta-molecule. Due to the completely destructive interference effect, the deep SEC-like stopband is achieved effectively on the transmission at fII = 2.26 GHz.
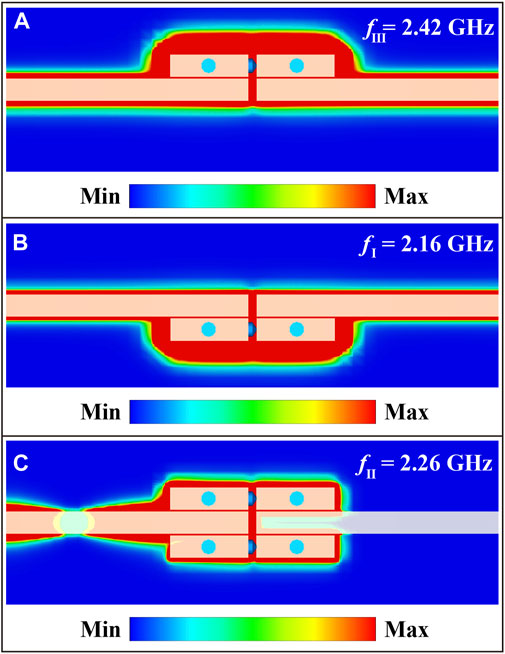
FIGURE 4. The simulated electric energy density distributions in (A) the individual upper ZIM-based meta-atom, (B) the individual lower ZIM-based meta-atom, and (C) the combinative SEC-like meta-molecule.
Subsequently, the dynamic control of SEC-like effect in the meta-molecule integrated with the PIN diode is investigated with numerical simulations. In this case, a variable lumped resistance is loaded assigned to the diode, and we set L1 = 3 nH for meta-atom1 and L2 = 7.5 nH for meta-atom2. Figure 5A shows the simulated transmission spectra (in dB) of the meta-molecule with the resistance ranging from 9,000 Ω to 10 Ω. It can be seen that, the initial SEC-like spectrum for high resistance gradually changes to EIT-like spectrum with the resistance decreasing to small values like 70 Ω, 30 Ω, and 10 Ω. Specifically, when the value of resistance is chosen to be 9,000 Ω, the middle of microstrip line can be approximated as a disconnected state. Via destructive interference between the two parallelly side-coupled meta-atoms along the cut microstrip line, a sharp and deep stopband emerges among the two individual resonant transmission peaks. With the resistance of 10 Ω, the cut microstrip line is almost connected. The individual side-coupled meta-atom in present acting as a reflector exhibiting a non-transparent response analogue to a two-level atom with absorption. Thus, the destructive interference of the two side-coupled meta-atoms at the same position along the continuous microstrip line leads to an EIT-like transmission spectrum. In result, the original transmission peak at 1.74 GHz eventually turns into a sharp transmission dip, demonstrating a modulation depth of 23.2 dB, and similar modulation feature is shown at 2.59 GHz. Especially, the initial deep stopband at 2.24 GHz induced by SEC-like effect tends to be an EIT-like transmission peak close to −1 dB with a modulation depth up to 39.6 dB, indicating the effective manipulation of the SEC-like effect by the PIN diode. Moreover, we have investigated that, by hiring a linear chain of meta-molecules with periodic number and periodic length equal to 11 mm and 20.1 mm, the bandwidth of the initial SEC-like deep stopband can be further expanded to a broad bandgap. The numerical simulations in Figure 5B also verify that, the spectrum shape of the broad bandgap can be tuned conveniently by changing the lumped resistance from 9,000 Ω to 10 Ω, indicating a broadband EM modulator.
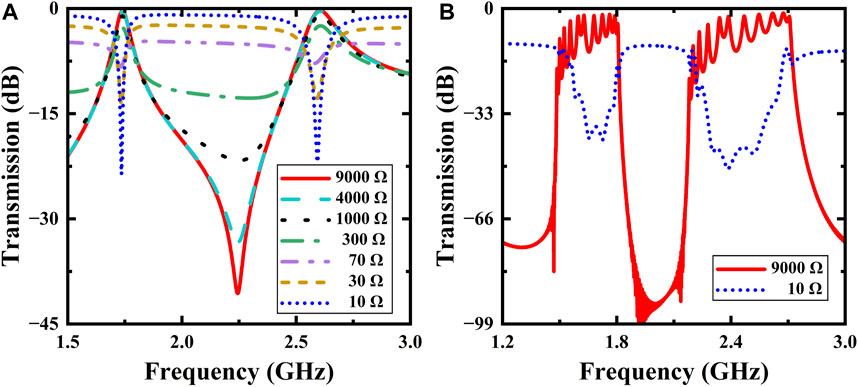
FIGURE 5. The simulated transmission spectra of tunable (A) a SEC-like meta-molecule and (B) a linear chain of SEC-like meta-molecules under different values of lumped resistance ranging from 9,000 Ω to 10 Ω.
In what follows, we experimentally investigate the dynamic tunability of SEC-like effect in the meta-molecule by applying bias voltage on the PIN diode. Figure 6 presents the measured transmission spectra with bias voltage varying from 0.0 V to 0.8 V. As no or low bias voltages is applied, taking the bias voltage of 0.2 V as an example, two resonant transmission peaks emerge at 1.74 GHz and 2.79 GHz, along with a transmission dip as deep as -52.1 dB around 2.59 GHz. As the bias voltage gradually increases to 0.8 V, the initial transmission dip around 2.59 GHz changes dramatically to a transmission peak of about −2.7 dB. We also notice that the transmission peak at 1.74 GHz presents a down and up trend, declining from −7.2 dB to −26.7 dB and then rise to a higher level at −8.6 dB. Meanwhile, the original transmission peak near 2.79 GHz decreases firstly from −5.5 dB to −22.8 dB and then increases close to −16.6 dB. We confirm that the inconsistent phenomenon with simulations is due possibly to the introduction of the biasing arms, the approximated lumped elements, as well as the accumulated errors during fabrication. Moreover, the frequency shifts and the transmittance decreases mainly result from the discrepancies between theoretical and real material parameters as well as the loss of the loaded elements. Apart from that, the measured electrical modulation behaviors match well with our theoretical prediction as illustrated in Figure 5. This indicate that the inductive modification on the destructive interference in meta-molecule can be used for practical modulating application at a subwavelength scale.
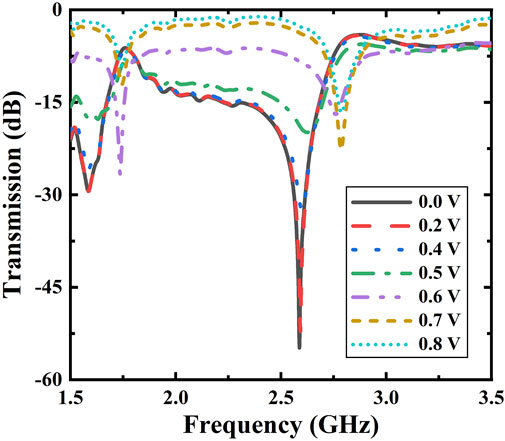
FIGURE 6. Measured transmission spectra of the tunable SEC-like meta-molecule under different biasing voltage changing from 0.0 V to 0.8 V.
At last, to show more details on the working performance of the EMM, we plot the measured transmission at three characteristic peak/dips frequencies of the SEC-like spectrum in Figure 7. Remarkable modulation in transmission can be achieved through varying the DC voltage at 1.74 GHz, 2.59 GHz, and 2.79 GHz. At the frequency of 1.74 GHz, for example, the transmission of the SEC-like structure shows a downward trend from −7.1 dB to −26.7 dB as the bias voltage varies from 0.0 V to 0.6 V, with a modulation ratio of 19.6 dB. As the bias voltage further increasing the voltage to 0.8 V, a growing transmission trend from −26.7 dB to −8.6 dB is observed with a modulation ratio of 18.1 dB. The modulations at 2.59 GHz show changing in the same bias voltage range, nevertheless, the transmission monotonously increases from the minimum −54.8 dB to the maximum −2.7 dB as the bias voltage varies from 0.0 V to 0.8 V, demonstrating a modulation depth of 52.1 dB. It is much higher than the value reported in Ref. 38 (the modulation contrast is 31 dB for the structure comprising of a single wire coupled to a paired wires loading the same PIN diode). Besides, it is obviously higher than the reported modulation depth (27 dB) in Ref. 39, which is made of orthogonal electric dipolar resonators with embedding the same PIN diode. Moreover, it is noteworthy that the proposed compact EM modulator has a two-port advantage over the above mentioned ones in integrated optical circuits and optical interconnection systems. Another obvious modulation effect is shown at 2.79 GHz, the transmission decreases from high transmission level to low transmission level as the bias voltage increases from 0.0 V to 0.7 V, with a modulation ratio of about −17.4 dB. In this sense, the electrically controllable SEC-like meta-molecule can act as a triple-band amplitude modulator.
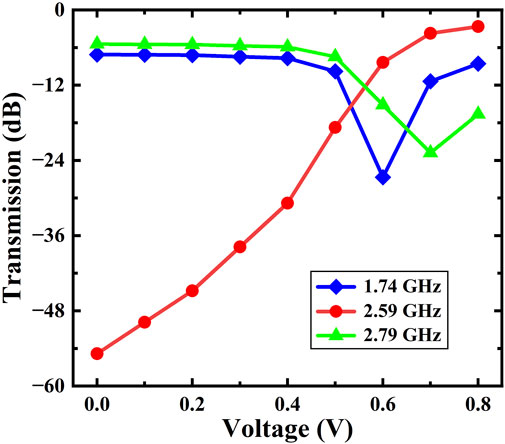
FIGURE 7. Measured transmission versus bias voltage at three characteristic frequencies of 1.74 GHz, 2.59 GHz, and 2.79 GHz, respectively.
4 Summary
In conclusion, we have proposed an EM modulator based on tunable SEC-like effect in a subwavelength meta-molecule. ZIM-based resonators serving as meta-atoms are aligned symmetrically in a cut microstrip to construct the meta-molecule with SEC-like in-unit interaction. A PIN diode is embedded in the gap of microstrip to manipulate the destructive interference between two detuned side-coupled meta-atoms. By electrically biasing the diode, the transmission properties of the SEC-like meta-molecule can be tuned flexibility. As a consequence, the transmission of electromagnetic waves in three characteristic bands located around characteristic peak/dips of the SEC-like spectrum is remarkably modulated. This configuration is also found to generate an extremely high modulation depth of up to 52.1 dB at a working frequency of 2.59 GHz. The findings may be helpful in realizing classical analogue to quantum phenomena for active photonic applications.
Data availability statement
The original contributions presented in the study are included in the article/Supplementary Material, further inquiries can be directed to the corresponding authors.
Author contributions
YC and YD conceived the research. YS supervised the project. LG, ZJ, YF, and XW performed the research and analyzed the data. LG and ZJ wrote the manuscript. All authors contributed to manuscript revision, read, and approved the submitted version.
Funding
This work was supported by the National Natural Science Foundation of China (Grant No. 11974261), and Jiangsu Province Key Discipline of China’s 14th five-year plan (Grant No. 2021135).
Conflict of interest
The authors declare that the research was conducted in the absence of any commercial or financial relationships that could be construed as a potential conflict of interest.
Publisher’s note
All claims expressed in this article are solely those of the authors and do not necessarily represent those of their affiliated organizations, or those of the publisher, the editors and the reviewers. Any product that may be evaluated in this article, or claim that may be made by its manufacturer, is not guaranteed or endorsed by the publisher.
References
1. Chen L, Ma Q, Nie Q, Hong Q, Cui H, Ruan Y, et al. Dual-polarization programmable metasurface modulator for near-field information encoding and transmission. Photon Res (2021) 9:116–24. doi:10.1364/prj.412052
2. Markovich DL, Andryieuski A, Zalkovskij M, Malureanu R, Lavrinenko AV. Metamaterial polarization converter analysis: Limits of performance. Appl Phys B (2013) 112:143–52. doi:10.1007/s00340-013-5383-8
3. Xu G, Gao L, Chen Y, Ding Y, Wang J, Fang Y, et al. Broadband polarization manipulation based on W-shaped metasurface. Front Mater (2022) 9:850020. doi:10.3389/fmats.2022.850020
4. Li D, Ma H, Zhan Q, Liao J, Yin W-Y, Chen H, et al. High-speed efficient on-chip electro-optic modulator based on midinfrared hyperbolic metamaterials. Phys Rev Appl (2021) 16:034002. doi:10.1103/PhysRevApplied.16.034002
5. Rout S, Sonkusale SR. A low-voltage high-speed terahertz spatial light modulator using active metamaterial. APL Photon (2016) 1:086102. doi:10.1063/1.4958739
6. Li Y, Gao W, Guo L, Chen Z, Li C, Zhang H, et al. Tunable ultra-broadband terahertz perfect absorber based on vanadium oxide metamaterial. Opt Express (2021) 29:41222–33. doi:10.1364/oe.444761
7. Guina M, Vainionpää A, Harkonen A, Orsila L, Lyytikäinen J, Okhotnikov OG. Vertical-cavity saturable-absorber intensity modulator. Opt Lett (2003) 28:43–5. doi:10.1364/ol.28.000043
8. Tripathi SM, Kumar A, Marin E, Meunier J-P. Single-multi-single mode structure based band pass/stop fiber optic filter with tunable bandwidth. J Lightwave Technol (2010) 28:3535–41. doi:10.1109/jlt.2010.2090035
9. Fan K, Suen JY, Padilla WJ. Graphene metamaterial spatial light modulator for infrared single pixel imaging. Opt Express (2017) 25:25318–25. doi:10.1364/OE.25.025318
10. Shi C, Luxmoore IJ, Nash GR. Gate tunable graphene-integrated metasurface modulator for mid-infrared beam steering. Opt Express (2019) 27:14577–84. doi:10.1364/OE.27.014577
11. Wen Q-Y, Tian W, Mao Q, Chen Z, Liu W-W, Yang Q-H, et al. Graphene based all-optical spatial terahertz modulator. Sci Rep (2014) 4:7409. doi:10.1038/srep07409
12. Chen H-T, Padilla WJ, Zide JMO, Gossard AC, Taylor AJ, Averitt RD. Active terahertz metamaterial devices. Nature (2006) 444:597–600. doi:10.1038/nature05343
13. Chen H-T, Padilla WJ, Cich MJ, Azad AK, Averitt RD, Taylor AJ. A metamaterial solid-state terahertz phase modulator. Nat Photon (2009) 3:148–51. doi:10.1038/nphoton.2009.3
14. Gu J, Singh R, Liu X, Zhang X, Ma Y, Zhang S, et al. Active control of electromagnetically induced transparency analogue in terahertz metamaterials. Nat Commun (2012) 3:1151. doi:10.1038/ncomms2153
15. Feng XD, Pu MB, Zhang F, Pan R, Wang S, Gong JT, et al. Large-area low-cost multiscale-hierarchical metasurfaces for multispectral compatible camouflage of dual-band lasers, infrared and Microwave. Adv Funct Mater (2022) 32(36):2205547. doi:10.1002/adfm.202205547
16. He PH, Niu LY, Fan Y, Zhang HC, Zhang LP, Yao D, et al. Active odd-mode-metachannel for single-conductor systems. Opto-electron Adv (2022) 5(8):210119. doi:10.29026/oea.2022.210119
17. Edwards B, Alù A, Young ME, Silveirinha M, Engheta N. Experimental verification of epsilon-near-zero metamaterial coupling and energy squeezing using a Microwave waveguide. Phys Rev Lett (2008) 100:033903. doi:10.1103/PhysRevLett.100.033903
18. Silveirinha MG, Engheta N. Theory of supercoupling, squeezing wave energy, and field confinement in narrow channels and tight bends usingεnear-zero metamaterials. Phys Rev B (2007) 76:245109. doi:10.1103/PhysRevB.76.245109
19. Liu R, Cheng Q, Hand T, Mock JJ, Cui T, Cummer SA, et al. Experimental demonstration of electromagnetic tunneling through an epsilon-near-zero metamaterial at Microwave frequencies. Phys Rev Lett (2008) 100:023903. doi:10.1103/PhysRevLett.100.023903
20. Enoch S, Tayeb G, Sabouroux P, Guérin N, Vincent P. A metamaterial for directive emission. Phys Rev Lett (2002) 89:213902. doi:10.1103/PhysRevLett.89.213902
21. Moniruzzaman M, Islam MT, Samsuzzaman M, Salaheldeen MM, Sahar NM, Al-Bawri SS, et al. Gap coupled symmetric split ring resonator based near zero index ENG metamaterial for gain improvement of monopole antenna. Sci Rep (2022) 12:7406. doi:10.1038/s41598-022-11029-7
22. Yang JJ, Francescato Y, Maier SA, Mao F, Huang M. Mu and epsilon near zero metamaterials for perfect coherence and new antenna designs. Opt Express (2014) 22:9107–14. doi:10.1364/OE.22.009107
23. Hao J, Yan W, Qiu M. Super-reflection and cloaking based on zero index metamaterial. Appl Phys Lett (2010) 96:101109. doi:10.1063/1.3359428
24. Guo Z, Jiang H, Chen H. Linear-crossing metamaterials mimicked by multi-layers with two kinds of single negative materials. J Phys Photon (2020) 2:011001. doi:10.1088/2515-7647/ab5ecb
25. Guo Z, Jiang H, Zhu K, Sun Y, Li Y, Chen H. Focusing and super-resolution with partial cloaking based on linear-crossing metamaterials. Phys Rev Appl (2018) 10:064048. doi:10.1103/PhysRevApplied.10.064048
26. Eleftheriades GV, Iyer AK, Kremer PC. Planar negative refractive index media using periodically L-C loaded transmission lines. IEEE T Micro Theor (2002) 50:2702–12. doi:10.1109/tmtt.2002.805197
27. Papasimakis N, Fedotov VA, Zheludev NI, Prosvirnin SL. Metamaterial analog of electromagnetically induced transparency. Phys Rev Lett (2008) 101:253903. doi:10.1103/PhysRevLett.101.253903
28. Zhang S, Genov DA, Wang Y, Liu M, Zhang X. Plasmon-induced transparency in metamaterials. Phys Rev Lett (2008) 101:047401. doi:10.1103/PhysRevLett.101.047401
29. Liu N, Langguth L, Weiss T, Kästel J, Fleischhauer M, Pfau T, et al. Plasmonic analogue of electromagnetically induced transparency at the Drude damping limit. Nat Mater (2009) 8:758–62. doi:10.1038/nmat2495
30. Tassin P, Zhang L, Koschny T, Economou EN, Soukoulis CM. Low-loss metamaterials based on classical electromagnetically induced transparency. Phys Rev Lett (2009) 102:053901. doi:10.1103/PhysRevLett.102.053901
31. Scully MO, Zhu S-Y. Quantum control of the inevitable. Science (1998) 281:1973–4. doi:10.1126/science.281.5385.1973
32. Zhu S-Y, Scully MO. Spectral line elimination and spontaneous emission cancellation via quantum interference. Phys Rev Lett (1996) 76:388–91. doi:10.1103/physrevlett.76.388
33. Li Y, Dong Z, Sun Y, Tan W, Jiang H, Wang Z, et al. Ultra-deep stopband induced by spontaneous-emission-cancellation–like interference between two side-coupled zero-index-metamaterial–based resonators. Europhys Lett (2012) 100:34003. doi:10.1209/0295-5075/100/34003
34. Chen Y, Li Y, Wu Q, Jiang H, Zhang Y, Chen H. Tuning the hybridization bandgap by meta-molecules with in-unit interaction. J Appl Phys (2015) 118:094505. doi:10.1063/1.4930038
35. Chen Y, Li Y, Wu Q, Jiang H, Zhang Y, Chen H. Quantum well effect based on hybridization bandgap in deep subwavelength coupled meta-atoms. Physica B (2015) 472:1–5. doi:10.1016/j.physb.2015.05.009
36. Bai Y, Chen K, Liu H, Bu T, Cai B, Xu J, et al. Optically controllable terahertz modulator based on electromagnetically-induced-transparency-like effect. Opt Commun (2015) 353:83–9. doi:10.1016/j.optcom.2015.05.005
37. Pitchappa P, Manjappa M, Ho CP, Singh R, Singh N, Lee C. Active control of electromagnetically induced transparency analog in terahertz MEMS metamaterial. Adv Opt Mater (2016) 4:541–7. doi:10.1002/adom.201500676
38. Fan Y, Qiao T, Zhang F, Fu Q, Dong J, Kong B, et al. An electromagnetic modulator based on electrically controllable metamaterial analogue to electromagnetically induced transparency. Sci Rep (2017) 7:40441. doi:10.1038/srep40441
39. Yang R, Fu Q, Fan Y, Fu Q, Cai W, Qiu K, et al. Active control of EIT-like response in a symmetry-broken metasurface with orthogonal electric dipolar resonators. Photon Res (2019) 7(9):955–60. doi:10.1364/prj.7.000955
40. Huang Y, Nakamura K, Takida Y, Minamide H, Hane K, Kanamori Y. Actively tunable THz filter based on an electromagnetically induced transparency analog hybridized with a MEMS metamaterial. Sci Rep (2020) 10:20807. doi:10.1038/s41598-020-77922-1
41. Sanada A, Caloz C, Itoh T. Characteristics of the composite right/left-handed transmission lines. IEEE Micro Wirel Co (2004) 14:68–70. doi:10.1109/lmwc.2003.822563
42. Allen CA, Leong KMKH, Itoh T. Design of microstrip resonators using balanced and unbalanced composite right/left-handed transmission lines. IEEE T Micro Theor (2006) 54(7):3104–12. doi:10.1109/TMTT.2006.877048
43. Weng Y, Wang ZG, Chen H. Band structures of one-dimensional subwavelength photonic crystals containing metamaterials. Phys Rev E (2007) 75:046601. doi:10.1103/PhysRevE.75.046601
Keywords: electromagnetic modulator, spontaneous emission cancellation, zero-index-metamaterials, meta-molecule, Pin diode
Citation: Gao L, Jiang Z, Chen Y, Fang Y, Wu X, Ding Y and Sun Y (2023) An electromagnetic modulator based on electrically controllable meta-molecule analogue to spontaneous emission cancellation. Front. Phys. 11:1142792. doi: 10.3389/fphy.2023.1142792
Received: 12 January 2023; Accepted: 02 February 2023;
Published: 10 February 2023.
Edited by:
Yang Long, Nanyang Technological University, SingaporeReviewed by:
Mingbo Pu, Institute of Optics and Electronics (CAS), ChinaZhuo Li, Nanjing University of Aeronautics and Astronautics, China
Copyright © 2023 Gao, Jiang, Chen, Fang, Wu, Ding and Sun. This is an open-access article distributed under the terms of the Creative Commons Attribution License (CC BY). The use, distribution or reproduction in other forums is permitted, provided the original author(s) and the copyright owner(s) are credited and that the original publication in this journal is cited, in accordance with accepted academic practice. No use, distribution or reproduction is permitted which does not comply with these terms.
*Correspondence: Yongqiang Chen, yqchen@usts.edu.cn; Yaqiong Ding, dyq192@163.com
†These authors share first authorship