- 1China Academy of Aerospace Science and Innovation, Beijing, China
- 2Beijing National Laboratory for Condensed Matter Physics, Institute of Physics, Chinese Academy of Sciences, Beijing, China
- 3Shanghai Pinghe Bilingual School, Shanghai, China
- 4Songshan Lake Materials Laboratory, Dongguan, Guangdong, China
We report a 1.04 GHz high-power Kerr-lens mode-locked Yb:CaYAlO4 laser pumped by a single-mode fiber laser at 976 nm. Based on a bow-tie cavity, stable unidirectional mode-locked operation is obtained with an output coupler of 1.6%. The oscillator delivers pulses with an average power of 1.46 W and with the pulse duration of 99 fs, which, to the best of our knowledge, is the first gigahertz-level Kerr-lens mode-locked laser based on the Yb:CaYAlO4 gain medium. We believe that the watt-level solid-state femtosecond laser at GHz would be an excellent source for developing time-resolved broadband dual-comb spectroscopy.
1 Introduction
Femtosecond optical frequency combs (OFCs) with high repetition rate and high average power are becoming desirable with the emerge of new application requirements, such as microwave photonics, Terahertz generation, analog-to digital conversion, bio-optical imaging, astronomical calibration, to name a few [1–5]. Especially for the time-resolved broadband dual-comb spectroscopy (DCS), the repetition rate and the average output power are two of the most important factors for the following reasons [6–8]. Firstly, according to the Nyquist condition, the obtainable spectral bandwidth of DCS satisfies
There are several approaches to generate high repetition rate femtosecond lasers. Electro-optical frequency comb could access >10 GHz regime, but it is challenging to achieve power scaling and self-referenced frequency locking, which is the same as the microcomb [12–14]. Femtosecond mode-locked lasers can be ideal candidates for developing <10 GHz OFCs. Compared to fiber mode-locked lasers as well as semiconductor lasers, all-solid-state femtosecond mode-locked lasers have the advantages of flexible cavity alignment, high average output power as well as the intrinsic low-noise performances [15]. Several significant results delivering gigahertz repetition rate ultrafast pulses based on the Ytterbium (Yb) doped gain medium around 1
Compared to the SESAM mode-locking, the Kerr-lens mode-locked (KLM) mechanism based on self-focusing principle is much more beneficial for sub-100 fs ultrashort pulse generation and could access higher repetition rate with more flexible cavity alignment. In 2012, M. Endo et al. developed a diode-pumped 4.6 GHz repetition-rate Yb:KYW KLM laser with an output power of 14.6 mW and a pulse duration of 146 fs [20]. In 2013, M. Endo et al. reported a 6 GHz KLM Yb:Lu2O3 ceramic laser with an average power of 10 mW [21]. They further developed a direct 15 GHz optical frequency comb based on the KLM Yb:Y2O3 ceramic laser with an average power of 60 mW in 2015 [22]. In 2019, S. Kimura presented a compact KLM Yb:Y2O3 laser with a only three-element setup, delivering pulses with a repletion rate of 23.8 GHz and a pulse duration of 120 fs at the average power of 20 mW [23]. Although the reported KLM femtosecond laser have broken through 20 GHz repetition rate, the output powers are limited to 100-mW level.
In this paper, we present a gigahertz watt-level KLM Yb:CaYAlO4 (Yb:CYA) laser with sub-100 fs pulse duration. A 976 nm single-frequency fiber laser with high brightness and good beam quality was employed as the pump. The Yb:CYA crystal is chosen as the gain medium, since it exhibits good performances in wide spectral emission, thermal conductivity as well as other optical and mechanical properties [24]. W. Tian et al. have achieved 10.4 W KLM Yb:CYA laser delivering sub-100 fs pulses with the peak power of 1.14 MW [25]. Up to now, results have been only limited to 100 MHz repetition rate regime. Here based on the Yb:CYA laser medium, we developed the KLM oscillator, delivering pulses with a repetition rate of 1.04 GHz, a pulse duration of 99 fs at an average power of 1.46 W. It is, to the best of our knowledge, the first watt-level KLM Yb:CYA femtosecond laser at gigahertz repetition rate, which would be a potential source for the time-resolved dual comb spectroscopy.
2 Experimental setup
The pump laser is an Ytterbium doped single-frequency fiber laser with high brightness, which is an ideal laser source for pumping high repetition rate femtosecond KLM laser. The used fiber laser delivers an average power of up to 8.5 W at the wavelength of 976 nm. The pump light is firstly collimated by a lens with a focal length of 300 mm and then focused by a lens with a focal length of 50 mm. A tight focus is used to enhance the Kerr effect and the focal spot size at the center of the crystal is measured to be 43 × 39 μm2. The focused power density could reach 5.85 × 105 W/cm2, which avoids the Q-switched instabilities to some extent.
The gain medium was an a-cut 3 mm-thick Yb:CYA crystal with a doping concentration of 8 at%, which is mounted on a water-cooled copper heat sink with the temperature of 13°C. The gain crystal is coated with anti-reflection in the range of 900 nm–1200 nm on both sides. The broad and flat emission cross section of the Yb:CYA gain crystal is ideal for generating sub-100 fs ultrashort laser pulses.
The laser setup is based on a bow-tie cavity, as shown in Figure 1. The radius of curvature of the two concave mirrors M1 and M2 are 50 mm. One concave mirror M1 serves as the pump mirror with a high transmission of the 976 nm pump wavelength and high reflection of the oscillating wavelengths centered at 1048 nm. Since the limited cavity elements makes it difficult for dispersion compensation, the other concave mirror M2 is chosen to be a Gires-Tournois interferometer (GTI) mirror providing a group delay dispersion of −550 fs2. The flat mirror M3 is also a GTI mirror with the group delay dispersion of −800 fs2. An output coupler with the transmission of 1.6% is selected to extract the pulse energy. The four-mirror ring cavity is designed with the length of 288 mm, corresponding to a repetition rate of 1.04 GHz. According to the ABCD matrix, the beam diameter of the intracavity laser mode in center of the crystal is about 46 μm, which is slightly bigger than the pump spot and is suitable for the Kerr-lens mode locking.
3 Results and discussion
The cavity is properly aligned at first and stable continuous wave operation is obtained in two directions with the total power of 1.6 W. To achieve Kerr-lens mode-locking, the position of the concave mirror M2 is scanned across the stability region. When the concave mirror M2 arrived the inner edge of the stability region, the output power becomes unstable and mode-locking could be achieved with fine tuning of the concave mirror M2. Once the Kerr-lens mode-locking is initiated, the output power jumped to 1.2 W and the output spectrum is broadened. The Figure 2 Shows that the output power of the Yb:CYA laser varies with the pump power and the pumping threshold for Kerr-lens mode-locking is measured to be 6.3 W. As the pump power increases to maximum of 8.5 W, the Kerr lens mode-locked pulses with an output power up to 1.46 W was obtained. Besides, it can be seen from the change trend of the mode-locked operation curve in Figure 2 that there is still room for improving the output power.
The output optical spectrum is measured by an optical spectrum analyzer, as shown in Figure 3. The full width at half maximum (FWHM) of the spectrum is 20 nm centered at 1048 nm, which corresponds to a Fourier transform-limited pulse duration of 54 fs. The pulse duration is measured by using a commercial autocorrelator (APE PulseCheck). As shown in Figure 4, The sech2-fit pulse duration is 99 fs. The single-pulse operation is not only verified by the autocorrelation trace but also by the microwave frequency spectrum and the temporal trace recorded by the oscilloscope.
Figure 5 shows the radio frequency spectrum of the repetition rate, which is recorded by a photodetector and measured by an RF spectrum analyzer (Rohde & Schwarz FSW 26). Under the resolution bandwidth (RBW) of 1 MHz and the frequency span of 1.2 GHz, the SNR is about 60 dB. Under the RBW of 100 kHz and the frequency span of 100 MHz, the SNR is about 80 dB, as shown by the inset of Figures 5, 6 Shows the temporal trace of the pulse sequences, which is recorded by the photodetector and an oscilloscope (Rohde & Schwarz, RTM 3004). Combing the RF spectrum and the temporal profile of the laser pulses, we could verify that no Q-switching modulations arises in the pulse trains.
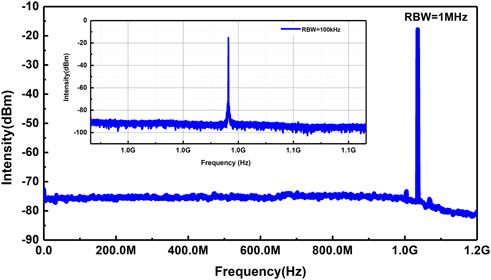
FIGURE 5. Radio frequency spectrum of the laser with the resolution bandwidth (RBW) of 1 MHz and 100 kHz (inset).
4 Conclusion
In conclusion, we have demonstrated a fiber laser pumped 1.04 GHz Yb:CYA femtosecond Kerr-lens mode-locked laser, which is, to the best of our knowledge, the first gigahertz ever reported for all-solid-state Yb:CYA laser. The pulse duration is measured to be 99 fs with the maximum output power of 1.46 W. The optical spectrum of the femtosecond laser is 20 nm FWHM centered at 1048 nm, corresponding to 54 fs Fourier transfer limited pulse duration. Therefore, with optimized dispersion management, even shorter pulse durations could be obtained. The developed oscillator with nJ-level pulse energy is appropriate for the succeeding spectral broadening by use of the photonic crystal fiber and for the CEO detection in the f-2f interferometer. Such results would provide suitable sources for broadband dual-comb spectroscopy with sufficient resolution, optical bandwidth as well as the sampling time.
Data availability statement
The raw data supporting the conclusion of this article will be made available by the authors, without undue reservation.
Author contributions
ZZ, JM, and, GZ contributed to the laser design and experimental schemes, and performed the experiments. They are also responsible for the data processing. YZ contributed to the data processing. ZZ, HH, and ZW contributed to write and edit the manuscript. All authors contributed to the article and approved the submitted version.
Funding
This work was supported by the Strategic Priority Research Program of the Chinese Academy of Sciences (XDA1502040404, XDB2101040004) and the National Natural Science Foundation of China (Grant Nos. 91850209 and 11774234).
Conflict of interest
The authors declare that the research was conducted in the absence of any commercial or financial relationships that could be construed as a potential conflict of interest.
Publisher’s note
All claims expressed in this article are solely those of the authors and do not necessarily represent those of their affiliated organizations, or those of the publisher, the editors and the reviewers. Any product that may be evaluated in this article, or claim that may be made by its manufacturer, is not guaranteed or endorsed by the publisher.
References
1. Fortier T, Kirchner M, Quinlan F, Taylor J, Bergquist JC, Rosenband T, et al. Generation of ultrastable microwaves via optical frequency division. Nat Photon (2011) 5:425–9. doi:10.1038/nphoton.2011.121
2. Fobbe F, Vogel T, Wulf F, Kohlhaas R, Globisch B, Rudin B, et al. THz generation with photoconductive emitters with a low-noise GHz repetition rate laser. In: 2021 46th International Conference on Infrared, Millimeter and Terahertz Waves; 29 August 2021 - 03 September 2021; Chengdu, China (2021). p. 1–2.
3. Ji N, Magee JC, Betzig E. High-speed, low-photodamage nonlinear imaging using passive pulse splitters. Nat Methods (2008) 5(2):197–202. doi:10.1038/nmeth.1175
4. Cundiff S, Weiner A. Optical arbitrary waveform generation. Nat Photon (2010) 4:760–6. doi:10.1038/nphoton.2010.196
5. Li CH, Benedick A, Fendel P, Glenday AG, Kartner FX, Phillips DF, et al. A laser frequency comb that enables radial velocity measurements with a precision of 1 cm/s-1. Nature (2008) 452:610–2. doi:10.1038/nature06854
6. Picqué N, Hänsch TW. Frequency comb spectroscopy. Nat Photon (2019) 13:146–57. doi:10.1038/s41566-018-0347-5
7. Coddington I, Newbury N, Swann W. Dual-comb spectroscopy. Optica (2016) 3:414–26. doi:10.1364/OPTICA.3.000414
8. Link SM, Maas DJHC, Waldburger D, Keller U. Dual-comb spectroscopy of water vapor with a free-running semiconductor disk laser. Science (2017) 356:1164–8. doi:10.1126/science.aam742410.1126/science.aam7424
9. Hoghooghi N, Cole RK, Rieker GB. 11-μs time-resolved, continuous dual-comb spectroscopy with spectrally filtered mode-locked frequency combs. Appl Phys B (2021) 127:17. doi:10.1007/s00340-020-07552-y
10. Muraviev AV, Smolski VO, Loparo ZE, Vodopyanov KL. Massively parallel sensing of trace molecules and their isotopologues with broadband subharmonic mid-infrared frequency combs. Nat Photon (2018) 12:209–14. doi:10.1038/s41566-018-0135-2
11. Lesko DMB, Timmers H, Xing S, Kowligy A, Lind AJ, Diddams SA. A six-octave optical frequency comb from a scalable few-cycle erbium fibre laser. Nat Photon (2021) 15:281–6. doi:10.1038/s41566-021-00778-y
12. Carlson DR, Hickstein DD, Zhang W, Metcalf AJ, Quinlan F, Diddams SA, et al. Ultrafast electro-optic light with subcycle control. science (2018) 361(6409):1358–63. doi:10.1126/science.aat6451
13. Parriaux A, Hammani K, Millot G. Electro-optic frequency combs. Adv Opt Photon (2020) 12:223–87. doi:10.1364/AOP.382052
14. Chang L, Liu S, Bowers JE. Integrated optical frequency comb technologies. Nat Photon (2022) 16:95–108. doi:10.1038/s41566-021-00945-1
15. Schilt S, Südmeyer T. Carrier-envelope offset stabilized ultrafast diode-pumped solid-state lasers. Appl Sci (2015) 5(4):787–816. doi:10.3390/app5040787
16. Pekarek S, Klenner A, Südmeyer T, Fiebig C, Paschke K, Erbert G, et al. Femtosecond diode-pumped solid-state laser with a repetition rate of 4.8 Ghz. Opt Express (2012) 20(4):4248–53. doi:10.1364/OE.20.004248
17. Klenner A, Golling M, Keller U. A gigahertz multimode-diode-pumped Yb:KGW enables a strong frequency comb offset beat signal. Opt Express (2013) 21:10351–7. doi:10.1364/OE.21.010351
18. Hakobyan S, Wittwer VJ, Brochard P, Gürel K, Schilt S, Mayer AS, et al. Full stabilization and characterization of an optical frequency comb from a diode-pumped solid-state laser with GHz repetition rate. Opt Express (2017) 25:20437–53. doi:10.1364/OE.25.020437
19. Mayer AS, Phillips CR, Keller U. Watt-level 10-gigahertz solid-state laser enabled by self-defocusing nonlinearities in an aperiodically poled crystal. Nat Commun (2017) 8:1673. doi:10.1038/s41467-017-01999-y
20. Endo M, Ozawa A, Kobayashi Y. Kerr-lens mode-locked Yb:KYW laser at 4.6-GHz repetition rate. Opt Express (2012) 20:12191–7. doi:10.1364/OE.20.012191
21. Endo M, Ozawa A, Kobayashi Y. 6-GHz, Kerr-lens mode-locked Yb:Lu_2O_3 ceramic laser for comb-resolved broadband spectroscopy. Opt Lett (2013) 38:4502–5. doi:10.1364/OL.38.004502
22. Endo M, Ito I, Kobayashi Y. Direct 15-GHz mode-spacing optical frequency comb with a Kerr-lens mode-locked Yb:Y_2O_3 ceramic laser. Opt Express (2015) 23:1276–82. doi:10.1364/OE.23.001276
23. Kimura S, Tani S, Kobayashi Y. Kerr-lens mode locking above a 20 GHz repetition rate. Optica (2019) 6:532–3. doi:10.1364/OPTICA.6.000532
24. Li D, Xu X, Zhu H, Chen X, De Tan W, Zhang J, et al. Characterization of laser crystal Yb:CaYAlO_4. J Opt Soc Am B (2011) 28:1650–4. doi:10.1364/JOSAB.28.001650
Keywords: ultrafast Laser, optical frequency comb, Kerr-lens mode-locked, all-solid-state-laser, Yb:CYA
Citation: Zhang Z, Ma J, Zhao G, Shao X, Zheng Y, Han H and Wei Z (2023) Sub-100 fs watt-level Kerr-lens mode-locked Yb:CaYAlO4 laser with a gigahertz repetition rate. Front. Phys. 11:1116054. doi: 10.3389/fphy.2023.1116054
Received: 05 December 2022; Accepted: 16 January 2023;
Published: 25 January 2023.
Edited by:
Guoqiang Xie, Shanghai Jiao Tong University, ChinaCopyright © 2023 Zhang, Ma, Zhao, Shao, Zheng, Han and Wei. This is an open-access article distributed under the terms of the Creative Commons Attribution License (CC BY). The use, distribution or reproduction in other forums is permitted, provided the original author(s) and the copyright owner(s) are credited and that the original publication in this journal is cited, in accordance with accepted academic practice. No use, distribution or reproduction is permitted which does not comply with these terms.
*Correspondence: Hainian Han, hnhan@iphy.ac.cn