- 1Department of Medical Biophysics, Jessenius Faculty of Medicine in Martin, Comenius University in Bratislava, Martin, Slovakia
- 2Department of Radiobiology, Cancer Research Institute, Biomedical Research Center, Slovak Academy of Sciences, Bratislava, Slovakia
- 3Department of Laboratory Medicine, Faculty of Health Care, Catholic University in Ružomberok, Ružomberok, Slovakia
- 4Department of Public Health, Jessenius Faculty of Medicine in Martin, Comenius University in Bratislava, Martin, Slovakia
Cell phones expose significant parts of the human brain and head to extremely low frequency (ELF) magnetic fields (MF) classified by the IARC as a 2B carcinogen. ELF MF was measured on the front and back sides of 15 cell phones in standby, speaking, and listening modes for 2G and 3G standards in two frequency bands, LF1: 5 Hz–200 Hz and LF2: 120 Hz—10 kHz. The highest MF value was 70.03 µT (RMS) in LF1 (2G, listening mode, front side) and 12.67 µT (RMS) in LF2 (2G, speaking mode, front side). The 3G cell phones consistently emitted a lower ELF MF compared to the 2G ones. The exposure to ELF MF was also simulated at various locations (head, thorax, pelvis) using the CST Studio Suite. The simulations revealed 8.45 µT, 7.5 µT, and 6.09 µT in the middle of the head (midbrain), 3.89 µT, 3.98 µT, and 2.83 µT for the middle of the thorax (heart), and 2.03 µT, 1.96 µT, and 1.56 µT in the middle of the pelvis (scrotum) for 10 Hz, 50 Hz, and 200 Hz, respectively. These values are comparable to those reported to induce biological and health effects, including those related to carcinogenesis. The results can be used in future studies concerning the ELF exposure or the combined effects of electromagnetic fields of radiofrequency and ELF.
1 Introduction
Cell phones not only expose the user to radiofrequency (RF), but also to a magnetic field (MF) of extremely low frequency (ELF: 1 Hz—100 kHz according to the ICNIRP [1] produced by either the RF signal envelope or the hardware itself (e.g., battery management) [2–7]. Consumption of electrical current produces MF in the vicinity of the wires, which can also have a significant impact on ELF exposure. Different hardware architectures may cause different exposure among cell phone models [8]. The nature of the cell phone ELF signal was described elsewhere [9,10]. The second generation Global System for Mobile Communications (2G GSM) uses eight time slots. The basic frame has a period of 4.61 ms sending information every 0.57 ms. Then the signal envelope creates a 217 Hz EMF component. Every 26th frame is omitted in GSM protocols, resulting in a 8.3 Hz EMF component in the output signal. The time frame used in the third-generation Universal Mobile Telecommunications System (3G UMTS) is 10 ms creating a 100 Hz component. A 50 Hz component follows from the 20 ms speech frame (except for the main power supply).
Detailed measurement of five cell phones was performed by the ITIS Foundations [9]. All phones have shown the maximum B-field on the back side with extrapolated pulse heights between 35 and 75 μT. The B-field on the front side of the tested phones was lower by a factor of 2-6-fold and varied between 8 and 20 μT.
[11] measured the ELF MF from commercial GSM handsets using probes, which covered the frequency range from static MF (0 Hz) to 2 GHz. Peak ELF fields were assessed on the front sides of five GSM phones and a maximum of 22.4 µT was reported. The main ELF component at 217 Hz was approximately 1 μT at the distance of 3 cm from the front of the handset. The overall pulse peak was 4.2 times higher than the 217 Hz component. The 217 Hz MF decreased with distance and reached 0.3 μT at approximately 5 cm from the front handset site.
[8] measured 47 phones released between 1997–2008. Maximum resultant magnetic flux density (B-field) at 25 mm from the center of the phone varied from 21 to 1178 nT with a geometric mean of 221 (+198/-104) nT. No correlation was observed between the B-field and any particular specification of the phones (e.g., size of battery, size and shape of the phone, year of release). Subsequent study by the same authors [12] provided an insight into the main factors contributing to ELF exposure. They concluded that factors that affect RF exposure (such as carrier frequency of communication system) do not necessarily affect ELF exposure, which is more dependent on the RF frame structure.
Weak ELF MF produce similar effects as non-thermal (NT) RF with significant overlap of molecular biological pathways [13–20]. The ELF MF effects were reported by many research groups at intensities lower than those produced by cell phones [21,22]. In particular, stress response, production of reactive oxygen species (ROS), increased sensitivity of stem cells, and inhibition of melatonin production were associated with increased cancer risks related to ELF exposure [23,24]. The ELF biological effects at intensities below the ICNIRP standards have been shown to manifest a complex dependence upon biological and physical variables similar to the NT RF effects [16,25–29] and have been considered in the frames of the same physical models [15,30–35].
The combined ELF and RF EMF exposure to DNA damage was studied by [36]. Exposure to ELF (30–100 Hz, 4.5 µT) did not significantly affect DNA, while combined exposure ELF and RF (2450 MHz, 1 mW/cm2) induced DNA breaks. [37] exposed human lens epithelial cells by RF (1.8 GHz with 217 Hz modulation) what induced ROS and DNA damage. However, superposing the 2 µT 30–90 Hz signal to the RF blocked ROS and DNA damage. [38] exposed amniotic epithelial cells by 15 min exposure to 217 Hz modulated 1800 MHz RF, 2 μT ELF, or concurrently to RF and ELF. Exposure to RF induced epidermal growth factor (EGF) receptor clustering and enhanced phosphorylation compared to ELF which did not cause the effects. However, when superimposed, complete inhibition of clustering and phosphorylation of the RF-induced EGF receptor occurred.
Although data on ELF for some old-type cell phones have been published, new models use improved technology and there is still a lack of information on ELF from the modern cell phones. Despite of new technologies, the fourth and fifth generations (4G and 5G); 2G and 3G are still fully employed primarily for making standard calls. Due to technological neutrality of the permits, providers can use for 5G the same frequencies, which they currently use for 2G, 3G, or 4G networks [39]. Although previous studies have generally analyzed ELF MF while cell phones being in standby or active mode, listening or speaking modes have not been evaluated. Therefore, the objective of this study was to compare exposure to 2G and 3G ELF at different modes using cell phones not older than 5 years at the time of measurement. For the first time, we performed simulations that utilized the real measured values for the most common cell phone position on the body—on the head, thorax, and pelvis areas.
2 Materials and methods
The ELF was measured on the front (LCD—Liquid Crystal Display) and back side of 15 cell phones in standby, speaking and listening mode (Figure 1). To measure B-field, the Narda NBM550 m (Narda STS, Germany) was fitted by 3-axis sensing Narda EHP50-D antenna (Narda STS, Germany) (Supplementary Figure S1) with the dimensions of 9.0 × 9.0 × 8.5 cm. Each phone was located 1 cm from the antenna using polystyrene foam. Before each measurement, the background ELF field was also measured. Just before measurements, the battery of each phone was charged to 100%, WiFi and data transmission were turned off, and signal level was checked using the indicator on the screen. To simulate a continuous phone call, the audiobook was played from the speakers.
Each cell phone was examined within two distinct frequency bands: LF1 5 Hz–200 Hz and LF2 100 Hz—10 kHz. Measurements were carried out between 8 am and 12 am at three modes: standby, 2G and 3G. The standby mode was divided into three routines: 1) with charger applied and LCD “off”, 2) no charger and LCD “off”, 3) no charger and LCD “on”. In both frequency bands, the highest MF peaks were recorded at each mode by the Narda meters (Supplementary Figure S2). Although there were no significant differences between the 2G/3G standby modes, we provided data only for the 3G standby mode. Each 2G/3G mode was divided into two routines: 1) listening mode (downlink), LCD off, no charger, and 2) speaking mode (uplink), LCD off, no charger. For each routine, cell phones were inspected from the front and back sides. Thus, the total number of measurements was 140 for each phone.
Comparison between groups for pooled data that fit normal distribution (analyzing designs with a single categorical independent variable) was performed by the one-way ANOVA, adjusted by the post hoc Fisher’s LSD test using Statistica 8.0 software (StatSoft Inc, Tulsa, USA). The results were considered significantly different at p < 0.05.
The experimental setup was numerically simulated using the CST Studio (Dassault Systèmes, France). The male phantom Gustav (CST Voxel Family) with the resolution of 2.08 × 2.08 × 2.0 mm was used to evaluate the MF intensity in three areas of interest: the head area simulating a cell phone position during calling; the thorax area simulating a cell phone placed in the shirt pocket in men or brassiere in women; and the pelvis area simulating a cell phone placed in the trouser pocket. The dielectric properties were adjusted for all tissues for each specific frequency by using the [40]. As demonstrated by [7], the ELF field of the phone can be adequately approximated by a circular loop. Thus, the single loop coil antenna with radius 80 mm, which was positioned 10 mm from the body surface, served as the ELF MF source approximating the exposure from the cell phones. The electrical current through the coil was set to ensure that the MF value on the coil equals to the maximal value on the phone (70.03 µT). The sine waveform was used to excite the coil made of an annealed copper strand conductor with open boundary conditions. Simulations were performed for three frequencies: 1) 10 Hz, which is the minimum frequency where the dielectric properties are defined in the Tissue properties database; 2) 50 Hz, which appeared most frequently during the measurements in LF1; and (iii) 200 Hz, which was an approximation for the LF2 band. Of note, the differences in the simulation results are negligible within the range of 195–225 Hz.
3 Results
The results of the magnetic flux density measurements are presented in Table 1. The “means” represent the averaged root-mean-square (RMS) values through the particular frequency bands (LF1 or LF2), while the “max” numbers represent the maximum RMS. The background MF values measured at the place of interest were 40 nT for LF1 and 50 nT for LF2.
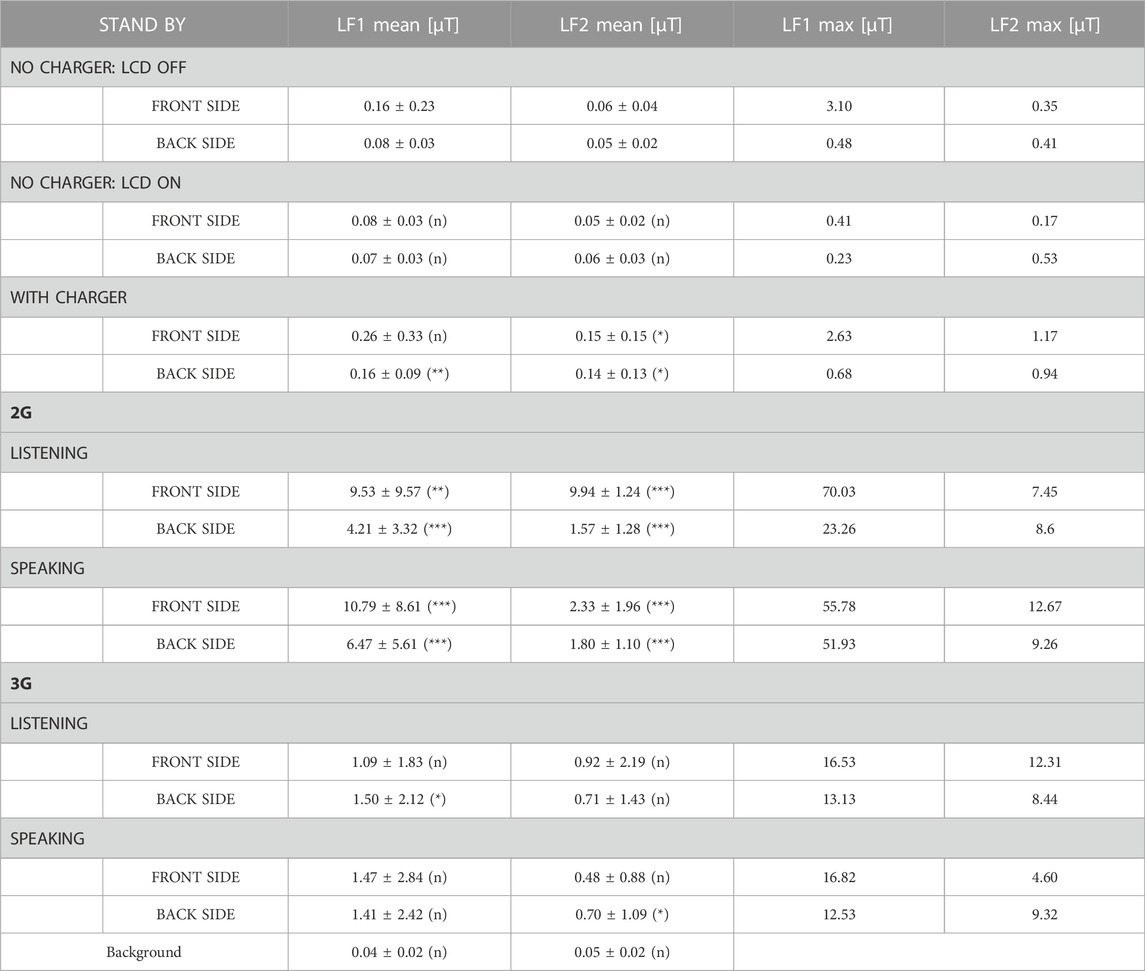
TABLE 1. ELF magnetic field measured on the 15 cell phones within two distinct frequency bands—LF1: 5 Hz,—200 Hz and LF2: 120 Hz—10 kHz. The measurements were carried out at three modes: standby mode, 2G mode and 3G mode. LF1 mean and LF2 mean represent mean RMS values of all 15 cell phones; LF1 max and LF2 max represent maximum RMS values out from all measurements. The values were statistically compared to the “no charger: LCD off” mode shown in the brackets (n—not significant; *—p < 0.05; **—p < 0.01; ***—p < 0.001).
Considering all cell phone models, multivariate ANOVA has revealed a charger as factor statistically increasing MF. The ELF measured in the “with charger” mode (0.16–0.26 µT in LF1 and 0.14–0.15 µT in LF2) compared to the ELF values measured in no charger: LCD “off” (0.08–0.16 µT in LF1 and 0.05–0.06 µT in LF2) and also no charger: LCD “on” (0.07–0.08 µT in LF1 and 0.05–0.06 µT in LF2) modes were significantly higher (p < 0.0001 for all modes). No statistically significant differences were observed between no charger: LCD “off” and no charger: LCD “on”. These results indicate that users should not charge their phones while using them.
Significant differences were revealed when comparing no charger: LCD “off” and 2G. The ELF values measured in the 2G were significantly higher (4.21–10.79 µT in LF1 and 1.57–9.94 µT in LF2; p < 0.001) compared to the ELF values measured in no charger: LCD “off” (0.08–0.16 µT in LF1 and 0.05–0.06 µT in LF2) in all modes. Interesting was that no significant differences were observed between no charger: LCD “off” and all 3G. The highest maximum RMS values were detected in the 2G listening mode, front side (70.03 µT for the Model 15 cell phone) for LF1 and 2G speaking mode, back side (12.67 µT for the Model 6) for LF2. In addition, the MF measured in the 2G (4.21–10.79 µT in LF1 and 1.57–9.94 µT in LF2; p < 0.001) were significantly higher than the MF values measured in the 3G (1.09–1.50 µT in LF1 and 0.48–0.92 µT in LF2) for almost all modes except for the MF measured in the listening mode LF2 that did not show statistically significant differences. These results suggest that the 2G standard is a factor that increases MF and the use of the 3G can decrease exposure to ELF MF.
In Table 2, the frequencies at which the highest MF values were detected, are shown. In the standby mode, the major contribution was 50 Hz (fundamental frequency of the main power supply) in LF1 and 100 Hz (second harmonic of the main power supply) in LF2. In the 2G, the frequency peaks were mostly observed at 5.6 Hz, 5.86 Hz, and 8.3 Hz in LF1 and 100 Hz, 217 Hz and 225 Hz in LF2 for both the listening and speaking modes. For the 3G mode, the frequency peaks were mostly observed at 5.37 Hz, 7.5 Hz, 8 Hz and 50 Hz for the listening mode, 5.86 Hz, 8.5 Hz, and 50 Hz for the speaking mode in LF1 and 100 Hz, 125 Hz, and 195 Hz for both the listening and speaking modes in LF2.
The MF was simulated using the highest measured value (70.03 µT) was set as the “worst-case scenario” for each simulated area (Figure 2). The values of the B-field (1 A/m = 1.25 µT), were 8.45 µT, 7.5 µT and 6.09 µT for the middle of the head (midbrain), 3.89 µT, 3.98 µT and 2.83 µT for the middle of the thorax (heart), and 2.03 µT, 1.96 µT and 1.56 µT for the middle of the pelvis (scrotum) at 10 Hz, 50 Hz, and 200 Hz, respectively. The MF would certainly be higher on the skin surface in comparison to what has been found in the middle of the head, thorax, and pelvis.
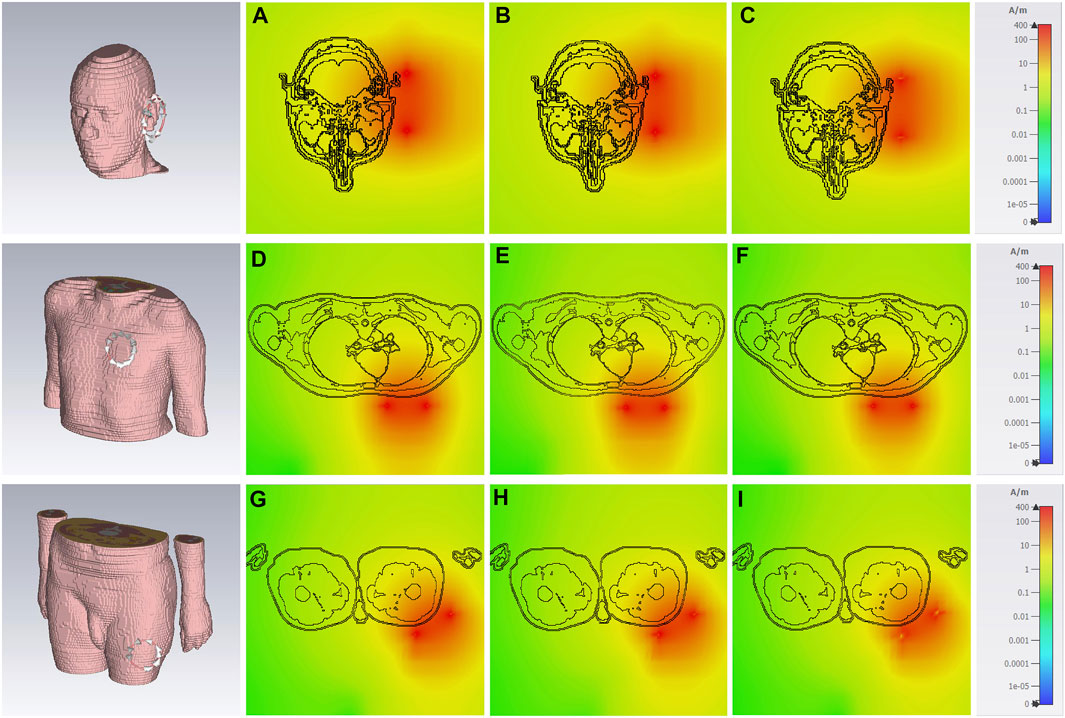
FIGURE 2. The figure shows the magnetic field distribution in the human body simulated in CST studio. The highest measured value (70.03 µT) was set as “worst-case scenario” for each simulated area. The first row (A–C) displays field distribution in the head, the second row (D–F) display field distribution in the thorax, and third row (G–I) display field distribution in the pelvis area. The first column shows the coil placement, and the second, third and fourth columns show results for the 10 Hz, 50 Hz, and 200 Hz, respectively.
4 Discussion
It is well known that the 2G technology uses higher maximal RF radiation power (2 W) to ensure proper connection with base transceiver stations (BTS). For the first time, our results revealed that the 2G phones also emit higher ELF MF as compared to the 3G phones. In particular, while the LF2 MF of 2G and 3G phones were comparable, the LF1 field values were approximately 2-4-fold higher.
When comparing the LCD “on” and LCD “off” modes, the MF fields were comparable to the background in both LF1 and LF2. As expected, almost all MF values (LF1/LF2) were significantly higher with the charger plugged in comparison to the “no charger” mode. While the charger serves as a source of DC current, the 50 Hz signal from the main power supply can also be delivered to the cell phone and the user. However, these 50 Hz MF were approximately 20- to 60-fold lower as compared to the active phone use (listening and speaking mode).
The previously published study by [9] focused on the MF measurements of five phones. All phones showed the maximum B-field on the back side with pulse heights between 35 and 75 μT (measured 5 mm from the phone surface). The front side B-field varied between 8 μT and 20 μT. These results are in line with our study, where the maximum B-field was 70.03 μT and 51.93 µT on the front and back sides, respectively. [7] measured B-field in 5 older cell phone models and found a maximum of 94.8 µT on the back surface of the phone. This value is higher as compared to our results probably due to better technology in the newer cell phone models. According to the ELF assessment by [10], MF at 217 Hz used in GSM and 100 Hz used in UMTS are the most distinctive component for the 2G and 3G mode, respectively. Our results have also revealed the 217 Hz/100 Hz component in the LF2 band of 2G/3G. In addition, we recorded the 8.3 Hz component in the 2G LF1, which corresponds to the periodicity of the GSM source. The B-field ranged from 0.021 to 1.178 μT at 25 mm from the center of the front side of the phone in the study by [8]. These values were lower in comparison to our results measured at the distance of 10 mm. However, Calderon et al. focused mainly on the 217 Hz component, while our measurements recorded the values through the whole frequency band, either LF1 or LF2 (i.e., more spectral components were included). The subsequent study by the same authors [12] reported the 100 Hz and 50 Hz signals, which were observed independently of RF radiation.
ICNIRP established the ELF-MF guidelines in 1998 [41] and revised them in 2010 [1]. For the frequency band of 1 Hz–10 kHz, the limit value of the B-field is 40 mT—27 µT (200 µT for frequency range 25–400 Hz). Our results are far below these guidelines. However, the BioInitiative report defined the ELF limits in the range of 0.2–0.4 µT for the general population and 0.1 µT for children and pregnant women [42]. Similarly, the EUROPAEM recommends that the averaged ELF MF should not exceed 0.1 µT for daytime and nighttime exposures [43]. Of note, the BioInitiative/EUROPAEM precautionary guidance values are based on the scientific evidence and not on the induced current density, as the ICNIRP guidelines. It should be mentioned that all ELF values measured by us in all 2G modes, LF1 3G modes, as well as the MF values resulting from simulations (midbrain, heart, and scrotum) exceeded the BioInitiative/EUROPAEM precautionary guidance values.
4.1 Effects of the ELF fields
Cell phones can expose significant parts of the human head (mostly parietotemporal areas) and other parts of the body by ELF MF. These fields are in the range of those that have been reported to induce biological and health effects, including carcinogenesis. The International Agency for Research on Cancer (IARC) classified ELF MF as a 2B carcinogen (possibly carcinogenic to humans) based on consistent data showing increased risks of childhood leukemia (ChL) in children exposed to ELF MF greater than 0.3 μT [21]; [44] found that the hazard ratio of Alzheimer’s disease (AD) of employees exposed to ELF above 0.2 μT was 2.30 as compared to employees exposed to lower ELF. [45] found that in chronically exposed train drivers (average exposure 21 µT with major component 16.7 Hz), the hazard ratio was 1.96 for senile dementia and 3.15 for AD in comparison to station masters (average exposure 1 µT). [46] reported health risks related to the CNS in workers exposed to ELF (>1.0 µT). The incident rate ratios were 1.44, 1.78, 1.40, and 1.34 for dementia, motor neuron disease, multiple sclerosis, and epilepsy, respectively, as compared to the population not employed in electric utilities.
The results of a cross-sectional study of power plant workers exposed to 0.85 μT ELF MF indicated that occupational exposure to ELF is associated with DNA single-strand breaks [47]. As reported by Wertheimer and Leeper, children living near power lines have an increased risk of ChL [48]. The maximal ELF MF measured 75 cm above ground under the power lines was 3.5 µT. Recent meta-analyses concluded that there is evidence of elevated risk of ChL with respect to residential proximity to high-voltage power lines [49]. Other pooled analysis has concluded that exposure to ELF MF at 0.4 μT increases the risk of ChL twofold [50]. The maximum MF measured in our study in standby mode was 3.1 µT (in LF1, no charger: LCD “off”). Therefore, it should be recommended not to keep a cell phone on the body or in its proximity at any time.
[51] investigated the relationship between occupational exposure to MF and brain cancer in men. They observed a positive association for all brain tumors at average ELF exposure ≥0.6 μT vs. < 0.3 μT. Our data showed that the highest B-field in the middle of the brain was 8.45 µT. These results suggest that the MF in the range of those emitted by cell phones may be sufficiently high for the effect to occur.
[52] proposed the inhibitory action of melatonin on the growth of human breast cancer cells in vitro (MCF-7) under exposure to the MF of 1.2 μT RMS at 60 Hz. This study was later replicated by [53] with the same result, suggesting that ELF MF may represent a risk factor for breast cancer. [54] observed an elevated risk of breast cancer in men exposed to ELF ≥0.6 μT. Our simulations revealed that the highest value observed in the middle of the thorax was 3.98 µT. This result warrants further investigation whether holding a cell phone near the chest may increase the risk of breast cancer.
[55] reported that long term exposure to 50 Hz MF at approximately 25 µT could have adverse effects on mammalian fertility and reproduction. Our simulation results have shown that the MF in the middle of the scrotum is 1.96 μT at 50 Hz by applying approximately 70 µT 10 mm from the body surface. These results indicate that keeping a cell phone in the trouser pocket can potentially affect reproduction and fertility.
Cell phones emit relatively high values of ELF MF, which have been reported to induce a variety of biological and health effects, including cancer. These data, along with high variability in ELF from different cell phone models, call manufacturers to provide values of ELF in the manuals for cell phones along with the SAR values for RF radiation. The previously published data [36–38] along with our results indicate that the combined effects of ELF MF and RF emitted by phones may also differ significantly from the effects of RF exposure alone. This circumstance should be considered when analyzing the biological and health effects of cell phone exposures.
4.2 Limitations
A limitation of this study is the dimensions of the sensing antenna, which did not allow a detailed MF mapping. The authors are also aware that our short-term measurements did not consider the changes in a BTS radiation power in-between full and base load, which can possibly affect the ELF MF values. However, this limitation was partially eliminated by controlling the signal level on the cell phone screens. For future studies, it would be worthwhile to perform ELF measurements in the 4G and 5G modes. It will also be interesting to perform the same measurements with the applied smartphone case or any type of a smartphone shielding tool (EMF protection).
5 Conclusion
Cell phones expose significant parts of the human head and other parts of the body to ELF MF classified by the IARC as a 2B carcinogen. The MF values measured in the LCD “off” mode were slightly higher compared to the LCD “on”. However, the MF in both modes were comparable to the background. The standby MF were approximately 20- to 60-fold lower compared to active phone use (listening and speaking mode). For the 2G, the highest RMS values were detected in the listening mode, front side (70.03 µT) within the range of 5 Hz–200 Hz and in the speaking mode, front side (12.67 µT) within the range of 120 Hz—10 kHz. For 3G, the highest RMS values were detected in the speaking mode, front side (16.82 µT) within the first range and the listening mode, front side (12.31 µT). The 3G cell phones consistently emitted a lower ELF MF as compared to the 2G ones. The measured and also simulated ELF MF were comparable with MF values that have been reported to induce biological and health effects, including those related to carcinogenesis.
A possible detrimental effect can occur under chronic ELF exposure even in standby mode. Therefore, it should be recommended to not keep cell phone on the body or in its proximity at any time. We also do not recommend charging the phones while using them as far as the charger can increase the exposure to MF ELF in the standby mode. According to our simulations, about 35 cm distance between body surface and a cell phone is needed to ensure the ELF MF values recommended by the EUROPAEM.
Data availability statement
The raw data supporting the conclusion of this article will be made available by the authors, without undue reservation.
Author contributions
JM and IB conceived the presented idea and planned the measurements. JM and MV performed the measurements. KH and JM performed the numerical simulations. LZ analyzed the data. JJ, IB, and VJ supervised the findings of this work. All authors discussed the results and contributed to the final manuscript.
Funding
This study was supported by the Slovak Research and Development Agency under the contract no. APVV-19-0214 and APVV-15-0250; the Operational Programme Integrated Infrastructure for the project: Long-term strategic research of prevention, intervention and mechanisms of obesity and its comorbidities, IMTS: 313011V344, co-financed by the European Regional Development Fund; and the projects VEGA 1/0173/20 and KEGA 057UK-4/2021.
Conflict of interest
The authors declare that the research was conducted in the absence of any commercial or financial relationships that could be construed as a potential conflict of interest.
Publisher’s note
All claims expressed in this article are solely those of the authors and do not necessarily represent those of their affiliated organizations, or those of the publisher, the editors and the reviewers. Any product that may be evaluated in this article, or claim that may be made by its manufacturer, is not guaranteed or endorsed by the publisher.
Supplementary material
The Supplementary Material for this article can be found online at: https://www.frontiersin.org/articles/10.3389/fphy.2023.1094921/full#supplementary-material
References
1.ICNIRP. For limiting exposure to time-varying electric and magnetic fields (1HZ – 100 kHZ). Health Phys (2010) 99(6):818 836.
2. Linde T, Mild KH. Measurement of low frequency magnetic fields from digital cellular telephones. Bioelectromagnetics (1997) 18(2):184–6. doi:10.1002/(sici)1521-186x(1997)18:2<184::aid-bem12>3.0.co;2-r
3. Heath B, Jenvey S, Cosic I. Investigation of analogue and digital mobile phone low frequency radiation spectrum characteristics. In Proceedings of the 2nd International Conference on Bioelectromagnetism (1998) 83–4.
4. Jokela K, Puranen L, Sihvonen AP. Assessment of the magnetic field exposure due to the battery current of digital mobile phones. Health Phys (2004) 86(1):56–66. doi:10.1097/00004032-200401000-00008
5. Ilvonen S, Sihvonen AP, Kärkkäinen K, Sarvas J. Numerical assessment of induced ELF currents in the human head due to the battery current of a digital mobile phone. Bioelectromagnetics (2005) 26(8):648–56. doi:10.1002/bem.20159
6. Cook CM, Saucier DM, Thomas AW, Prato FS. Exposure to ELF magnetic and ELF-modulated radiofrequency fields: The time course of physiological and cognitive effects observed in recent studies (2001-2005). Bioelectromagnetics (2006) 27(8):613–27. doi:10.1002/bem.20247
7. Perentos N, Iskra S, McKenzie R, Cosic I. Characterization of pulsed ELF magnetic fields generated by GSM mobile phone handsets. World Congress Med Phys Biomed Eng (2007) 1414(1-6):2706–9.
8. Calderon C, Addison D, Mee T, Findlay R, Maslanyj M, Conil E, et al. Assessment of extremely low frequency magnetic field exposure from GSM mobile phones. Bioelectromagnetics (2014) 35(3):210–21. doi:10.1002/bem.21827
9. Tuor M, Ebert S, Schuderer J, Kuster N. Assessment of ELF exposure from GSM handsets and development of an optimized RF/ELF exposure setup for studies of human volunteers: Final report. Zurich: IT IS FoundationBAG Reg (2005). No. 2.23. 02.-18/02.001778.
10. Gosselin M, Kühn S, Kuster N. Experimental and numerical assessment of low-frequency current distributions from UMTS and GSM mobile phones. Phys Med Biol (2013) 58:8339–57. doi:10.1088/0031-9155/58/23/8339
11. Perentos N, Iskra S, McKenzie R, Cosic I. Simulation of pulsed ELF magnetic fields generated by GSM mobile phone handsets for human electromagnetic bioeffects research. Australas Phys Eng Sci Med (2008) 31(3):235–42. doi:10.1007/bf03179350
12. Calderon C, Ichikawa H, Taki M, Wake K, Addison D, Mee T, et al. ELF exposure from mobile and cordless phones for the epidemiological MOBI-Kids study. Environ Int (2017) 101:59–69. doi:10.1016/j.envint.2017.01.005
13. Adey WR. Tissue interactions with non-ionizing electromagnetic fields. Physiol Rev (1981) 61(2):435–514. doi:10.1152/physrev.1981.61.2.435
14. Binhi VN, Alipov YD, Belyaev IY. Effect of static magnetic field on E. coli cells and individual rotations of ion-protein complexes. Bioelectromagnetics (2001) 22(2):79–86. doi:10.1002/1521-186x(200102)22:2<79::aid-bem1009>3.0.co;2-7
16. Blank M, Goodman R. Electromagnetic fields stress living cells. Pathophysiology (2009) 16(2-3):71–8. doi:10.1016/j.pathophys.2009.01.006
17. Davanipour Z, Sobel E. Long-term exposure to magnetic fields and the risks of Alzheimer's disease and breast cancer: Further biological research. Pathophysiology (2009) 16(2-3):149–56. doi:10.1016/j.pathophys.2009.01.005
19. Radil R, Barabas J, Janousek L, Bereta M. Frequency dependent alterations of S. Cerevisiae proliferation due to LF EMF exposure. Adv Electr Electron Eng (2020) 18(2):99–106. doi:10.15598/aeee.v18i2.3461
20. Sladicekova K, Bereta M, Misek J, Parizek D, Jakus J. Biological effects of a low-frequency electromagnetic field on yeast cells of the genus Saccharomyces Cerevisiae. Acta Med Martiniana (2021) 21(2):34–41. doi:10.2478/acm-2021-0006
21.IARC. Non-ionizing radiation, Part 1: Static and extremely low-frequency (ELF) electric and magnetic fields. IARC Monogr Eval Carcinog Risks Hum (2002) 80:1–395. Lyon, France, IARC Press.
22. Mild KH, Wilen J, Mattsson MO, Simko M. Background ELF magnetic fields in incubators: A factor of importance in cell culture work. Cell. Biol. Int. (2009) 33(7):755–7. doi:10.1016/j.cellbi.2009.04.004
23. Burch JB, Reif JS, Noonan CW, Yost MG. Melatonin metabolite levels in workers exposed to 60-hz magnetic fields: Work in substations and with 3-phase conductors. J Occup Environ Med (2000) 42(2):136–42. doi:10.1097/00043764-200002000-00006
24. Wyszkowska J, Stankiewicz M. Extremely low frequency electromagnetic field and nervous system – review of recent results. Przeglad Elektrotechniczny (2009) 85(12):170–3.
25. Belyaev IY, Alipov YD, Harms-Ringdahl M, Bersani F. Effects of weak ELF on E-coli cells and human lymphocytes: Role of genetic, physiological, and physical parameters. In Electricity and magnetism in biology and medicine New York: Kluwer Academic/Plenum Publ (1999). p. 481–4.
26. Belyaev IY, Alipov ED. Frequency-dependent effects of ELF magnetic field on chromatin conformation in Escherichia coli cells and human lymphocytes. Biochim Biophys Acta (2001) 1526(3):269–76. doi:10.1016/s0304-4165(01)00138-6
27. Olsson G, Belyaev IY, Helleday T, Harms-Ringdahl M. ELF magnetic field affects proliferation of SPD8/V79 Chinese hamster cells but does not interact with intrachromosomal recombination. Mutat Res - Genet Toxicol Environ Mutagenesis (2001) 493(1-2):55–66. doi:10.1016/s1383-5718(01)00158-9
28. Sarimov R, Markova E, Johansson F, Jenssen D, Belyaev I. Exposure to ELF magnetic field tuned to Zn inhibits growth of cancer cells. Bioelectromagnetics (2005) 26(8):631–8. doi:10.1002/bem.20146
29. Sarimov R, Alipov ED, Belyaev IY. Fifty hertz magnetic fields individually affect chromatin conformation in human lymphocytes: Dependence on amplitude, temperature, and initial chromatin state. Bioelectromagnetics (2011) 32(7):570–9. doi:10.1002/bem.20674
30. Chiabrera A, Bianco B, Caufman JJ, Pilla AA Quantum dynamics of ions in molecular crevices under electromagnetic exposure. In: Brighton C.T., Pollack S.R. (eds.) Electromagnetics in medicine and biology. San Francisco, CA: San Francisco Press (1991). P. 21–6.
31. Matronchik AI, Alipov ED, Belyaev IY. A model of phase modulation of high frequency nucleoid oscillations in reactions of E. coli cells to weak static and low-frequency magnetic fields. Biofizika (1996) 41(3):642–9.
32. Chiabrera A, Bianco B, Moggia E, Kaufman JJ. Zeeman-Stark modeling of the RF EMF interaction with ligand binding. Bioelectromagnetics (2000) 21(4):312–24. doi:10.1002/(sici)1521-186x(200005)21:4<312::aid-bem7>3.0.co;2-#
33. Panagopoulos DJ, Karabarbounis A, Margaritis LH. Mechanism for action of electromagnetic fields on cells. Biochem Biophys Res Commun (2002) 298(1):95–102. doi:10.1016/s0006-291x(02)02393-8
34. Matronchik AY, Belyaev IY. Model of slow nonuniform rotation of the charged DNA domain for effects of microwaves, static and alternating magnetic fields on conformation of nucleoid in living cells. Fröhlich Centenary International Symposium "Coherence and Electromagnetic Fields in Biological Systems (CEFBIOS-2005). J Pokorny Prague, Czech Republic (2005) 63–4. Institute of Radio Engineering and Electronics, Academy of Sciences of the Czech Republic.
35. Matronchik AY, Belyaev IY. Mechanism for combined action of microwaves and static magnetic field: Slow non uniform rotation of charged nucleoid. Electromagn Biol Med (2008) 27(4):340–54. doi:10.1080/15368370802493313
36. Lai H, Singh NP. Interaction of microwaves and a temporally incoherent magnetic field on single and double DNA strand breaks in rat brain cells. Electromagn Biol Med (2005) 24(1):23–9. doi:10.1081/jbc-200055046
37. Yao K, Wu W, Wang K, Ni S, Ye P, Yu Y, et al. Electromagnetic noise inhibits radiofrequency radiation-induced DNA damage and reactive oxygen species increase in human lens epithelial cells. Mol Vis (2008) 14:964–9.
38. Sun W, Shen X, Lu D, Lu D, Chiang H. Superposition of an incoherent magnetic field inhibited EGF receptor clustering and phosphorylation induced by a 1.8 GHz pulse-modulated radiofrequency radiation. Int J Radiat Biol (2013) 89(5):378–83. doi:10.3109/09553002.2013.754559
39.TELEOFF. The national table of frequency allocations (NTFA) Slovak republic. Regulatory authority for electronic communications and postal services [TELEOFF] of the Slovak republic (2021). Available at: https://www.vus.sk/ntfs/php/index.php?jazyk=ang (Last accessed May 14, 2021).
40.Tissue properties database. ITIS foundation (2022). Available at: https://itis.swiss/virtual-population/tissue-properties/database/dielectric-properties/ (Last accessed September 23, 2022).
41.ICNIRP. For limiting exposure to time-varying electric, magnetic and electromagnetic fields (up to 300 GHz). Health Phys (1998) 74(4):494522.
42. Carpenter DO, Sage C. A rationale for biologically-based public exposure standards for electromagnetic fields (2007). Key scientific evidence and public health policy recommendations Available at: https://bioinitiative.org/wp-content/uploads/pdfs/sec24_2007_Key_Scientific_Studies.pdf (Last accessed September 26, 2022).
43. Belyaev I, Dean A, Eger H, Hubmann G, Jandrisovits R, Kern M, et al. Europaem emf guideline 2016 for the prevention, diagnosis and treatment of emf-related health problems and illnesses. Rev Environ Health (2016) 31(3):363–97. doi:10.1515/reveh-2016-0011
44. Qiu C, Fratiglioni L, Karp A, Winblad B, Bellander T. Occupational exposure to electromagnetic fields and risk of Alzheimer’s disease. Epidemiology (2004) 15(6):687–94. doi:10.1097/01.ede.0000142147.49297.9d
45. Röösli M, Lörtscher M, Egger M, Pfluger D, Schreier N, Lortscher E, et al. Mortality from neurodegenerative disease and exposure to extremely low-frequency magnetic fields: 31 years of observations on Swiss railway employees. Neuroepidemiology (2007) 28(4):197–206. doi:10.1159/000108111
46. Pedersen C, Poulsen AH, Rod NH, Rod NH, Frei P, Grell K, et al. Occupational exposure to extremely low-frequency magnetic fields and risk for central nervous system disease: An update of a Danish cohort study among utility workers. Int Arch Occup Environ Health (2017) 90:619–28. doi:10.1007/s00420-017-1224-0
47. Zendehdel R, Yu IJ, Hajipour-Verdom B, Panjali Z. DNA effects of low level occupational exposure to extremely low frequency electromagnetic fields (50/60 Hz). Toxicol Ind Health (2019) 35(6):424–30. doi:10.1177/0748233719851697
48. Wertheimer N, Leeper E. Electrical wiring configurations and childhood cancer. Am J Epidemiol (1979) 109(3):273–84. doi:10.1093/oxfordjournals.aje.a112681
49. Carpenter DO. Extremely low frequency electromagnetic fields and cancer: How source of funding affects results. Environ Res (2019) 178:108688. doi:10.1016/j.envres.2019.108688
50. Schüz J. Exposure to extremely low-frequency magnetic fields and the risk of childhood cancer: Update of the epidemiological evidence. Prog Biophys Mol Biol (2011) 107(3):339–42. doi:10.1016/j.pbiomolbio.2011.09.008
51. Villeneuve PJ, Agnew DA, Johnson KC, Mao Y. Canadian cancer registries epidemiology research group. Brain cancer and occupational exposure to magnetic fields among men: Results from a Canadian population-based case-control study. Int J Epidemiol (2002) 31(1):210–7.
52. Harland JD, Liburdy RP. Environmental magnetic fields inhibit the antiproliferative action of tamoxifen and melatonin in a human breast cancer cell line. Bioelectromagnetics (1997) 18(8):555–62. doi:10.1002/(sici)1521-186x(1997)18:8<555::aid-bem4>3.0.co;2-1
53. Blackman CF, Benane SG, House DE. The influence of 1.2 microT, 60 Hz magnetic fields on melatonin- and tamoxifen-induced inhibition of MCF-7 cell growth. Bioelectromagnetics (2001) 22(2):122–8. doi:10.1002/1521-186x(200102)22:2<122::aid-bem1015>3.0.co;2-v
54. Grundy A, Harris SA, Demers PA, Johnson KC, Agnew DA. Canadian Cancer Registries Epidemiology Research Group, Villeneuve PJ. Occupational exposure to magnetic fields and breast cancer among Canadian men. Cancer Med (2016) 5(3):586–96.
Keywords: ELF MF, Cell phone exposure, MF simulations, MF in cell phones, ELF exposure
Citation: Misek J, Jakus J, Hamza Sladicekova K, Zastko L, Veternik M, Jakusova V and Belyaev I (2023) Extremely low frequency magnetic fields emitted by cell phones. Front. Phys. 11:1094921. doi: 10.3389/fphy.2023.1094921
Received: 10 November 2022; Accepted: 16 January 2023;
Published: 27 January 2023.
Edited by:
Jonne Naarala, University of Eastern Finland, FinlandReviewed by:
Valentina Hartwig, Institute of Clinical Physiology, National Research Council (CNR), ItalyZhaowen Yan, Beihang University, China
Copyright © 2023 Misek, Jakus, Hamza Sladicekova, Zastko, Veternik, Jakusova and Belyaev. This is an open-access article distributed under the terms of the Creative Commons Attribution License (CC BY). The use, distribution or reproduction in other forums is permitted, provided the original author(s) and the copyright owner(s) are credited and that the original publication in this journal is cited, in accordance with accepted academic practice. No use, distribution or reproduction is permitted which does not comply with these terms.
*Correspondence: J. Misek, bWlzZWszQHVuaWJhLnNr; I. Belyaev, aWdvci5iZWxpYWV2QHNhdmJhLnNr