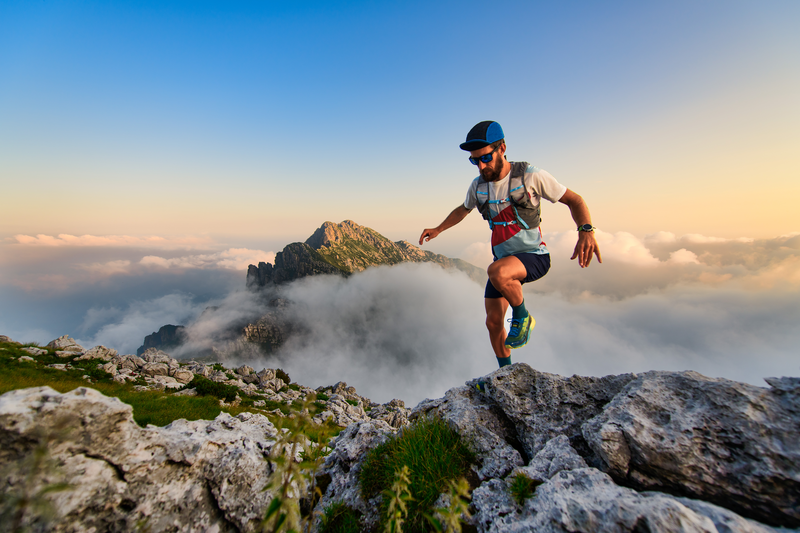
95% of researchers rate our articles as excellent or good
Learn more about the work of our research integrity team to safeguard the quality of each article we publish.
Find out more
ORIGINAL RESEARCH article
Front. Phys. , 31 August 2022
Sec. Optics and Photonics
Volume 10 - 2022 | https://doi.org/10.3389/fphy.2022.986795
This article is part of the Research Topic Miniaturized High-Power Solid-state Laser and Applications View all 23 articles
High-quality GaInSn liquid nanospheres are successfully fabricated by the ultrasonic method as a novel saturable absorber in the mid-infrared range. An open-aperture Z-scan technique is applied to study the saturation absorption property, presenting a modulation depth of 34.3% and a saturable fluence of 0.497 GW/cm2 at 2.3 μm, respectively. With GaInSn nanospheres as a saturable absorber, a stable Q-switched Er:CaF2 crystal laser operating at 2.75 μm is realized. The maximum Q-switched output power of 361 mW is obtained under the absorbed pump power of 2.9 W. The shortest pulse width of 500 ns and the highest repetition rate of 67 kHz are generated, corresponding to maximum peak power and single pulse energy of 10.78 W and 5.39 μJ, respectively. These findings indicate a promising potential of GaInSn nanospheres SA for generating nanosecond mid-infrared laser pulses.
The mid-infrared (MIR) pulsed lasers play a crucial role in technological developments because of its wide application value in environmental monitoring, optoelectronic countermeasure, space communication, frontier physics, and so on [1–5]. Especially, the high absorption coefficient of water at 3 μm makes it great potential to be utilized in medical treatments. An effective and concise way to acquire high energy pulsed lasers is the passively Q-switched (PQS) method in which the saturable absorber (SA) is the key component. For the past few years, SAs based on one-dimensional (1D) and two-dimensional (2D) materials have attracted much attention and some encouraging application results in the mid-infrared region have been demonstrated [6–10]. However, there are still some intrinsic drawbacks which limit their further application. For example, the weak absorption efficiency of graphene, the selective absorption of carbon nanotubes, the heavy inherent defects of TIs, and the instability of BP in the ambient environment need to be improved [11]. Besides, zero-dimensional metal nanoparticles have been studied and then applied to realize mid-infrared pulsed lasers due to their localized surface plasmon resonance (LSPR). In 2018, Duan et al. realized a 734 ns Q-switched Ho,Pr:LLF laser at 3 μm by using gold nanoparticles (Au-NPs) as a SA [11]. In addition, a 2 μm Q-switched laser based on Ag-NPs SA has also been developed [12]. Nevertheless, the disadvantage of the high cost of these noble metals has limited the incentive for further research.
In recent years, a novel nano-liquid metal material, GaInSn, has attracted great attention due to its excellent physico-chemical properties [13–16]. Nevertheless, most studies on GaInSn have focused on electrons and thermal conductivity, while few researches have been done on its nonlinear optical properties. Similar to the properties of most zero-dimensional materials, the saturation absorption characteristics of GaInSn nanospheres are also caused by LSPR [17]. It is worth noting that the amorphous form and high ductility of the liquid metal materials could produce diverse plasma microcavities, which may exhibit a broader LSPR response region than the traditional nanometals, such as Au-NPs and Ag-NPs. In 2020, Zhang et al. reported broadband saturation absorption characteristics of the GaInSn nanospheres and successfully realized Q-switched laser operations at 1.3 μm and 2 μm, separately [17]. Considering its broader LSPR response range, the nonlinear optical properties at ∼3 μm, especially its performance as a SA applied in a Q-switched pulse laser, need to be further investigated.
In this paper, high-quality GaInSn nanospheres were successfully prepared by the ultrasonic method. The saturation absorption property of the GaInSn nanospheres was measured via the open-aperture Z-scan technique, presenting a modulation depth of 34.3% and a saturable fluence of 0.497 GW/cm2, respectively. By utilizing GaInSn nanospheres as a SA in a 2.75 μm all-solid-state laser, a maximum Q-switched output power of 361 mW was obtained with the shortest pulse width of 500 ns.
The GaInSn nanospheres were fabricated by the ultrasonic method. The ultrasonic method is a simple, rapid, and large-scale method for the preparation of liquid metal nanospheres. First, the three metals were mixed with the proportions of 68% Ga, 22% In, and 10% Sn and then heated for 10 min at 350°C to form GaInSn alloy. The prepared alloy was encapsulated in a needle tubing, as shown in Figure 1A. To comminute it into nanospheres, the alloy was subjected to ultrasonic in acetone for 20 h. By optimizing the ultrasonic process parameters and multiple sedimentations, GaInSn nanospheres with uniform shapes and diameters less than 100 nm could be obtained. Figure 1B displays the suspension of the GaInSn nanospheres. The morphology of GaInSn nanospheres was characterized by transmission electron microscope (TEM), as described in Figure 1C. The TEM image showed that the as-prepared GaInSn nanospheres were almost spherical and the corresponding size distribution was illustrated in Figure 1D.
FIGURE 1. (A) GaInSn liquid alloy encapsulated in needle tubing. (B) As-prepared suspension of the GaInSn nanospheres. (C) TEM image. (D) The corresponding size distribution.
To explore the nonlinear saturable absorption property of the as-prepared GaInSn nanospheres, the open-aperture Z-scan technique was employed. The longest wavelength of the optical parametric laser applied in our Z-scan measurement is at 2.3 µm with a pulse width of 120 fs and a repetition rate of 5 kHz. The saturation absorption curve at 2.3 µm was fitted by the following formula [18]:
where Is, ΔR, and Ans represent saturable fluence, modulation depth, and non-saturable loss, respectively. The saturation fluence and modulation depth of the as-prepared GaInSn nanospheres was fitted to be 0.497 GW/cm2 and 34.3%, respectively. The fitting curve was shown in Figure 2. The experimental results confirm that the GaInSn nanospheres possess excellent saturation absorption characteristics and could be adopted as a saturable absorber in the mid-infrared range.
The experimental setup of the GaInSn nanospheres Q-switched Er:CaF2 laser was illustrated in Figure 3. A 3 × 3 × 11 mm3 Er:CaF2 crystal with a doped concentration of 1.7 at% was employed and both end surfaces of the laser crystal were well polished. To mitigate the thermal load, the laser crystal was wrapped with indium foil and embedded in a copper fixture. The copper was water-cooled with a temperature of 12.8°C. A plane-concave laser cavity was selected with the cavity length of 23 mm. The input mirror (IM) had a curvature radius of R = −100 mm with anti-reflectivity (AR) coated around 972 nm and high reflectivity (HR) coated near 3 μm. Three plane mirrors were adopted as output couplers (OCs) with different transmittance of 2%, 5%, and 10% at 2.8–3.0 μm. The pump source was a commercial 972 nm fiber-coupled laser diode (LD) with a numerical aperture (N.A.) of 0.22 and core diameter of 200 μm. The pump light was focused into the Er:CaF2 crystal with a diameter size of 400 μm by an optical couple lenses. Moreover, a dichroic beam splitter was placed behind OCs to filter the residual pump light.
The CW laser output power was first achieved by using the three different transmittances of OCs. The absorption efficiency of the Er:CaF2 crystal at 972 nm was measured to be about 54.8%. Figure 4A shows the variation of average output powers with absorbed pump powers. In order to prevent the crystal from thermal damage, the absorbed pump power was limited to 2.9 W. Under a maximum absorbed pump power, a maximum average output power of 0.493 W was achieved with an output mirror transmittance of 2%, corresponding to a slope efficiency of 18.2% and an optical-to-optical conversion efficiency of 9.6%.
The GaInSn nanospheres were directly spin-coating on the two output couplers with the transmittance of 2% and 5%, which can reduce insertion loss. The relationships between Q-switching output powers and absorbed pump power are displayed in Figure 4B. Under a maximum absorbed pump power of 2.9 W, the highest Q-switching output power of 0.361 W was achieved with the transmission of 2%, corresponding to a slope efficiency of 14%. The slope efficiency is much higher than the previous result obtained by Graphene as SA [19], which may attribute to the low insertion loss of the GaInSn nanospheres.
A mid-infrared detector (VIGO System S.A., PVI-4TE-6) with a response time of 50 ns was used to detect the signal and the Q-switched pulses were synchronously displayed on a digital oscilloscope (Rohde & Schwarz, RTO2012, 1 GHz bandwidth, 10 Gs/s sampling rates). Figure 5A,B show the pulse width and pulse repetition frequency as a function of the absorbed pump power. The pulse repetition rate increased with increasing absorbed pump power, while the pulse width decreased. Under the maximum absorbed pump power, the shortest pulse width and maximum pulse repetition rate with the transmittance of 2% are 500 ns and 67 kHz, respectively. A maximum single-pulse energy was reached to 5.39 μJ, corresponding to a maximum peak power of 10.78 W, as described in Figure 5C,D. Typical pulse trains and a single pulse profile in Q-switching operation are illustrated in Figure 6. The output performance of Q-switched laser is obviously superior to that of graphene and graphdiyne as SA [19–21]. Beyond the absorbed pump power of 2.9 W, the pulse width increased, which resulted from the full saturation of the SA and the thermal lens effect in the gain medium. The regular Q-switched pulse could be recovered by slightly decreasing the pump power.
FIGURE 5. Pulse repetition rate and pulse duration versus absorbed pump power at (A) T = 2%, (B)T = 5%; the dependence of pulse energy and peak power on the absorbed pump power at (C) T = 2%, (D)T = 5%.
In conclusion, high-quality GaInSn nanospheres were fabricated by employing the ultrasonic method. The saturation absorption property and its application as a Q-switcher in the mid-infrared region were demonstrated. The saturation absorption property at 2.3 μm was studied through the open aperture Z-scan technique, presenting a saturable intensity and a modulation depth of 0.497 GW/cm2 and 34.3%, respectively. Using the OC transmittance of 2%, the maximum Q-switching output power of 0.361 W was obtained, corresponding to a slope efficiency of 14%. The shortest pulse width of 500 ns was generated with the highest repetition rate of 67 kHz. The corresponding maximum peak power and single pulse energy were 10.78 W and 5.39 μJ, respectively. The findings indicate that the GaInSn nanospheres could act as an outstanding optical switcher device at 2.75 μm, which may help to explore potential applications in mid-infrared nonlinear optics.
The original contributions presented in the study are included in the article/Supplementary Material, further inquiries can be directed to the corresponding authors.
BC was responsible for investigation, experiment, and writing. KL was responsible for experiment and editing. YJ was responsible for review. PW was responsible for data curation. NZ was responsible for experiment. KZ was responsible for data curation. SL was responsible for conceptualization, methodology, writing, and funding acquisition. JX was responsible for experiment, data curation, and editing.
This work was supported by National Key R&D Program of China (2017YFA0303700), National Natural Science Foundation of China (62175133), the Natural Science Foundation of Shandong Province (ZR2020MF115), and the SDUST Research Fund (skr21-3-049, 2019TDJH103).
The authors declare that the research was conducted in the absence of any commercial or financial relationships that could be construed as a potential conflict of interest.
All claims expressed in this article are solely those of the authors and do not necessarily represent those of their affiliated organizations, or those of the publisher, the editors and the reviewers. Any product that may be evaluated in this article, or claim that may be made by its manufacturer, is not guaranteed or endorsed by the publisher.
1. Fan M, Li T, Zhao S, Li G, Ma H, Gao X, et al. Watt-level passively Q-switched Er:Lu2O3 laser at 2.84 μm using MoS2. Opt Lett (2016) 41:540. doi:10.1364/OL.41.000540
2. Schliesser A, Picqué N, Hänsch TW. Mid-infrared frequency combs. Nat Photon (2012) 6:440–9. doi:10.1038/nphoton.2012.142
3. Arslanov DD, Spunei M, Mandon J, Cristescu SM, Persijn ST, Harren FJM. Continuous-wave optical parametric oscillator based infrared spectroscopy for sensitive molecular gas sensing: Cw OPO spectroscopy for chemical gas sensing. Laser Photon Rev (2013) 7:188–206. doi:10.1002/lpor.201100036
4. Popmintchev T, Chen M-C, Popmintchev D, Arpin P, Brown S, Ališauskas S, et al. Bright coherent ultrahigh harmonics in the keV X-ray regime from mid-infrared femtosecond lasers. Science (2012) 336:1287–91. doi:10.1126/science.1218497
5. Li X, Guo Y, Ren J, Liu J, Wang C, Zhang H, et al. Narrow-bandgap materials for optoelectronics applications. Front Phys (Beijing) (2022) 17:13304. doi:10.1007/s11467-021-1055-z
6. Yao Y, Zhang F, Chen B, Zhao Y, Cui N, Sun D, et al. Nonlinear optical property and mid-infrared Q-switched laser application at 2.8 μm of PtSe2 material. Opt Laser Tech (2021) 139:106983. doi:10.1016/j.optlastec.2021.106983
7. Yao Y, Li X, Song R, Cui N, Liu S, Zhang H, et al. The energy band structure analysis and 2 μm Q-switched laser application of layered rhenium diselenide. RSC Adv (2019) 9:14417–21. doi:10.1039/C9RA02311A
8. Fan M, Li T, Zhao S, Li G, Gao X, Yang K, et al. Multilayer black phosphorus as saturable absorber for an Er:Lu2O3 laser at ∼3 μm. Photon Res (2016) 4:181. doi:10.1364/PRJ.4.000181
9. Fan M, Li T, Li G, Zhao S, Yang K, Zhang S, et al. Passively Q-switched Ho, Pr:LiLuF4 laser with graphitic carbon nitride nanosheet film. Opt Express (2017) 25:12796. doi:10.1364/OE.25.012796
10. Liu J, Liu J, Guo Z, Zhang H, Ma W, Wang J, et al. Dual-wavelength Q-switched Er:SrF2 laser with a black phosphorus absorber in the mid-infrared region. Opt Express (2016) 24:30289. doi:10.1364/OE.24.030289
11. Duan W, Nie H, Sun X, Zhang B, He G, Yang Q, et al. Passively Q-switched mid-infrared laser pulse generation with gold nanospheres as a saturable absorber. Opt Lett (2018) 43:1179. doi:10.1364/OL.43.001179
12. Ahmad H, Samion MZ, Muhamad A, Sharbirin AS, Ismail MF. Passively Q-switched thulium-doped fiber laser with silver-nanoparticle film as the saturable absorber for operation at 2.0 µm. Laser Phys Lett (2016) 13:126201. doi:10.1088/1612-2011/13/12/126201
13. Chen B, Wang P, Zhang N, Li K, Zhang K, Liu S, et al. GaInSn liquid nanospheres as a saturable absorber for Q-switched pulse generation at 639 nm. Opt Express (2022) 30:28242. doi:10.1364/OE.467944
14. Liu Y, Chen H, Zhang H, Li Y. Heat transfer performance of lotus-type porous copper heat sink with liquid GaInSn coolant. Int J Heat Mass Transfer (2015) 80:605–13. doi:10.1016/j.ijheatmasstransfer.2014.09.058
15. Zavabeti A, Daeneke T, Chrimes AF, O’Mullane AP, Zhen Ou J, Mitchell A, et al. Ionic imbalance induced self-propulsion of liquid metals. Nat Commun (2016) 7:12402. doi:10.1038/ncomms12402
16. Ou M, Qiu W, Huang K, Feng H, Chu S. Ultrastretchable liquid metal electrical conductors built-in cloth fiber networks for wearable electronics. ACS Appl Mater Inter (2020) 12:7673–8. doi:10.1021/acsami.9b17634
17. Zhang T, Wang M, Xue Y, Xu J, Xie Z, Zhu S. Liquid metal as a broadband saturable absorber for passively Q-switched lasers. Chin Opt Lett (2020) 18:111901. doi:10.3788/COL202018.111901
18. Liu S, Cui N, Liu S, Wang P, Dong L, Chen B, et al. Nonlinear optical properties and passively Q-switched laser application of a layered molybdenum carbide at 639 nm. Opt Lett (2022) 47:1830. doi:10.1364/ol.454047
19. Li C, Liu J, Jiang S, Xu S, Ma W, Wang J, et al. 2.8 μm passively Q-switched Er:CaF2 diode-pumped laser. Opt Mater Express (2016) 6:1570. doi:10.1364/OME.6.001570
20. Zong M, Yang X, Liu J, Zhang Z, Jiang S, Liu J, et al. Er:CaF2 single-crystal fiber Q-switched laser with diode pumping in the mid-infrared region. J Lumin (2020) 227:117519. doi:10.1016/j.jlumin.2020.117519
Keywords: 3 μm mid-infrared laser, GaInSn nanospheres, liquid metal, passively Q-switched lasers, saturable absorber
Citation: Chen B, Li K, Jin Y, Wang P, Zhang N, Zhang K, Liu S and Xu J (2022) GaInSn liquid nanospheres as a saturable absorber for an Er:CaF2 laser at 2.75 μm. Front. Phys. 10:986795. doi: 10.3389/fphy.2022.986795
Received: 05 July 2022; Accepted: 12 August 2022;
Published: 31 August 2022.
Edited by:
Baitao Zhang, State Key Laboratory of Crystal Materials, Shandong University, ChinaCopyright © 2022 Chen, Li, Jin, Wang, Zhang, Zhang, Liu and Xu. This is an open-access article distributed under the terms of the Creative Commons Attribution License (CC BY). The use, distribution or reproduction in other forums is permitted, provided the original author(s) and the copyright owner(s) are credited and that the original publication in this journal is cited, in accordance with accepted academic practice. No use, distribution or reproduction is permitted which does not comply with these terms.
*Correspondence: Shande Liu, cGVwc2xfbGl1QDE2My5jb20=; Jinlong Xu, bG9uZ25vLjJAMTYzLmNvbQ==
Disclaimer: All claims expressed in this article are solely those of the authors and do not necessarily represent those of their affiliated organizations, or those of the publisher, the editors and the reviewers. Any product that may be evaluated in this article or claim that may be made by its manufacturer is not guaranteed or endorsed by the publisher.
Research integrity at Frontiers
Learn more about the work of our research integrity team to safeguard the quality of each article we publish.