- 1Institute of Laser and Optoelectronics, School of Precision Instrument and Optoelectronics Engineering, Tianjin University, Tianjin, China
- 2Key Laboratory of Optoelectronic Information Science and Technology (Ministry of Education), Tianjin University, Tianjin, China
In this work, a single-frequency fiber amplifier with output power of 703 W was demonstrated at 1,064.4 nm in an all-fiber configuration. Cascaded Yb3+-doped fiber structure with different dopant concentration and hybrid 915/976 nm pump scheme were employed in power scaling stage to improve the gain saturation for higher transverse mode instability threshold. An overall optical efficiency of 67.5% was achieved at the maximum output power and the M2 was measured to be ∼1.4. A spectral linewidth of 2 kHz was obtained from the 703-W single-frequency laser. To the best of our knowledge, this is the first time that a single-frequency all-fiber amplifier with kHz-level spectral linewidth is achieved at such high output power.
Introduction
High power single-frequency laser sources have been attracting extensive interest due to the superior performance on extremely narrow spectral linewidth in kilohertz level and low noise for the applications ranging from coherent LIDAR, gravitational wave detection to coherent beam combination [1–3]. For higher laser power achievement, single-frequency laser source is generally configured with a master oscillation power amplifier (MOPA). Compared with solid-state amplifiers [4–6], fiber amplifiers exhibit the advantages on high thermal dissipation capability, good beam quality and high compactness. However, the narrow laser spectral linewidth facilitates gain accumulation of stimulated Brillouin scattering (SBS) process [7–10], resulting in an SBS-limited single-frequency fiber laser amplification to 500-W level with regular fiber structure. Actually, as early as 2007, [11] has demonstrated a 511-W random-polarized single-frequency fiber amplifier, where Yb3+-doped fiber with core diameter up to 43 μm was used in power scaling stage in associated with a counter-pumped scheme to increase the SBS threshold [11]. However, the large fiber inner cladding diameter of 650 μm, designed for improved pump power coupling, resulted in a low pump absorption coefficient of only 1.5 dB/m at 975 nm and thus ∼9 m of gain fiber needed in the experiment compromised the SBS-limited laser power.
On the other hand, novel fiber structures have also been developed for SBS suppression. In 2007, S. Gray et al. reported another 500-W level single-frequency fiber amplifier, where the SBS was suppressed by adjusting the composition of an Al/Ge co-doped gain fiber to reduce the interaction between acoustic and optical modes [12]. 8.5-m Yb3+-doped fiber with core diameter of 39 μm and pump absorption coefficient of 3.2 dB/m was used to realize 502 W laser power while near single-mode operation with an M2 of 1.4 was obtained due to the low core numerical aperture of 0.05. In 2011, [13]. developed an acoustically segmented Yb3+-doped photonic crystal fiber (PCF) for single-frequency laser amplification, where the SBS threshold can be improved by manipulating the acoustic index of refraction of fiber core to form two regions with distinct Brillouin peaks [13]. 500-W single-frequency laser output was achieved from the power amplifier stage with 5-m Yb3+-doped PCF, which has a core diameter of 40 μm and pump absorption coefficient of 4 dB/m at 976 nm. Whereas further power increment was not limited by SBS effect but transverse mode instability (TMI) effect, which induced the degradation of beam quality. In 2014, an acoustic- and gain-tailored Yb3+-doped PCF was developed by the same group to suppress the TMI threshold with the introduction of another core segment free of Yb3+ ions to reduce the fiber heat load, laser gain ratio between the LP11 and LP01 modes, as well as direct mode coupling [14]. A 9.2-m Yb3+-doped PCF with core diameter of 38 μm and pump absorption coefficient of 1.6 dB/m at 976 nm was used to realize a record single-frequency laser power of 811 W. The beam quality was maintained well with an M2 of ∼1.2 at the maximum output power, indicating that the TMI effect was well suppressed. Further power amplification was still limited by the SBS effect eventually. In 2020, Lai et al. reported a 550-W single-frequency all-fiber amplifier at 1,030 nm with tapered Yb3+-doped fiber, where gradually increased fiber core diameter provided a larger mode field diameter and a broadened SBS gain spectrum for higher SBS threshold [15]. Moreover, high absorption coefficient up to 12 dB/m at 975 nm allowed for only around 1-m-long gain fiber used in the experiment for increased SBS threshold. However, too large core diameter, up to 57.6 μm at the output end of the tapered fiber, induced the onset of TMI effect, which hindered the laser power increase.
Overall, the SBS and TMI effects have been concluded as two main limitations for the development of high-power single-frequency fiber amplifiers. Especially the TMI effect, originating from the mode interference pattern (MIP) between fundamental mode (FM) and higher order modes (HOMs) [16, 17], has been observed in most of the recently developed single-frequency fiber amplifiers [18, 19], since few-mode large-mode-area (LMA) fibers are generally employed for laser power extraction and SBS suppression.
In this work, a 703-W single-frequency all-fiber amplifier at 1,064.4 nm was demonstrated with commercially available LMA Yb3+-doped fibers. The TMI effect was suppressed by virtue of improved gain saturation through the employment of two pieces of cascaded Yb3+-doped LMA fibers in power amplifier stage with hybrid 915/976 nm pump scheme. The overall optical efficiency was calculated to be ∼67.5% at the maximum output power of 703 W. Further power scaling was limited by the onset of SBS.
The threshold of SBS and TMI effect were also investigated in this work under different polarization extinction ratio (PER) of the single-frequency seed laser. A lower threshold came to TMI effect as the seed laser depolarized from linear-polarization to a state with PER of ∼6.8 dB. As the seed laser PER further decreased to 4.3 dB, SBS effect became the main limitation on power amplification, which is just the condition of the demonstrated 703-W single-frequency laser. The properties of laser linewidth were investigated during the power amplification, where a narrow linewidth of 2 kHz was obtained at the maximum output power of 703 W with only a bit broadening compared with the 1.3-kHz single-frequency laser seed.
Experimental setup
The experimental arrangement of the demonstrated single-frequency all-fiber amplifier was shown in Figure 1. A linear-polarized single-frequency DBR fiber laser at 1,064.4 nm (NP Photonics, RFLS-25-3-1,064) with output power of 30 mW, spectral linewidth of 1.3 kHz and optical signal-to-noise ratio (OSNR) of 70 dB, was used to serve as the laser seed. Before launching into the power amplifier (PA) stage, the single-frequency laser was boosted to 7.5 W through one core-pumped and one cladding-pumped pre-amplifiers.
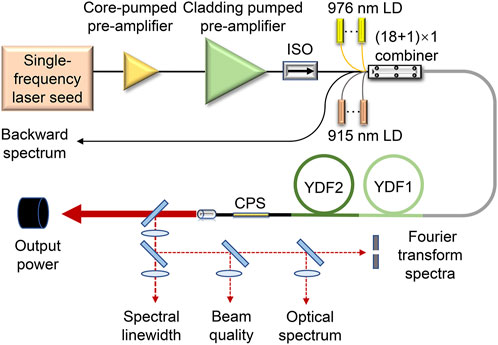
FIGURE 1. Schematic of the high-power single-frequency all-fiber amplifier. ISO: isolator; CPS: cladding pump stripper; YDF1: 1-m low Yb3+-doped fiber with a 976-nm absorption coefficient of 8 dB/m; YDF2: 1-m high Yb3+-doped fiber with a 976-nm absorption coefficient of 14.2 dB/m; LD: laser diode.
In PA stage, cascaded Yb3+-doped fiber structure was used as shown in Figure 1. 1-m low Yb3+-doped fiber with a 976-nm absorption coefficient of 8 dB/m (labelled as YDF1, Brightcore, LMA-YDF-30/250, MFD of 23.0 μm) was selected as the first segment to mitigate the thermal load at the beginning of the amplifier. The intensity noise generated by amplified spontaneous emission (ASE) due to insufficient population extraction under low signal power can be avoided to contribute the onset of TMI [20]. 1-m high Yb3+-doped fiber with an absorption coefficient of 14.2 dB/m at 976 nm (labelled as YDF2, Liekki, Yb1200-30/250, MFD of 22.9 μm) was employed in the later segment to guarantee the optical efficiency. The active fibers were coiled on a water-cooled aluminum plate with diameter around 17 cm. Since improved gain saturation can be achieved through decreased pump absorption [21], hybrid pump scheme with 440-W pump power at 915 nm and 600-W pump power at 976 nm was designed to further increase the TMI threshold. The pump laser was coupled into the active fiber via an (18 + 1) × 1 combiner, whose input and output signal ports have the core/inner cladding diameter of 12/125 μm and 30/250 μm, respectively. A mode field adapter was incorporated into the combiner to eliminate the mismatch between the input and output port of the signal. A homemade cladding pump striper (CPS) is used after the cascaded gain fiber to remove the residual cladding pump light, after which a homemade endcap was spliced for laser output. 90% of the laser output power was detected by the power meter and the rest part was used for the measurement of spectral linewidth, beam quality, optical spectrum and temporal behavior, respectively.
Results and discussions
To increase the SBS threshold, the linearly polarized laser seed was depolarized by using the polarization controller (PC) after the core-pumped pre-amplifier. The PER of laser seed can be decreased to a minimum value of 4.3 dB limited by the performance of the PC. The corresponding power evolution in PA stage was measured with a power meter (Ophir, FL1100A-BB-65). The backward optical spectrum was monitored at the same time by an optical spectrum analyzer (OSA, Yokogawa, AQ6370D) with a resolution of 0.02 nm from the idle pump port of the combiner. As shown in Figure 2A, the output power was firstly amplified to 220 W using 440-W pump power at 915 nm with an optical efficiency of around 50%. Then the optical efficiency was improved significantly to 80% with the employment of pump laser at 976 nm. When the launched 976-nm pump power reached 600 W, at which point the total pump power was 1040 W, an SBS-limited output power of 703 W was obtained with an overall optical efficiency of 67.5%. Figures 2B–D show the measured optical spectrum of the PA in backward direction as the output power increased. The backward Stokes light emerged at the output power of 620 W, and then increased gradually with laser power. When the laser output power reached 703 W, the intensity of Stokes light suddenly exceeded that of backward Rayleigh scattering light, as shown in Figure 2B. The pump power was not increased thereafter. Considering the seed laser used here exhibited a PER of 4.3 dB, further SBS threshold enhancement can be anticipated with a circularly polarized laser seed.
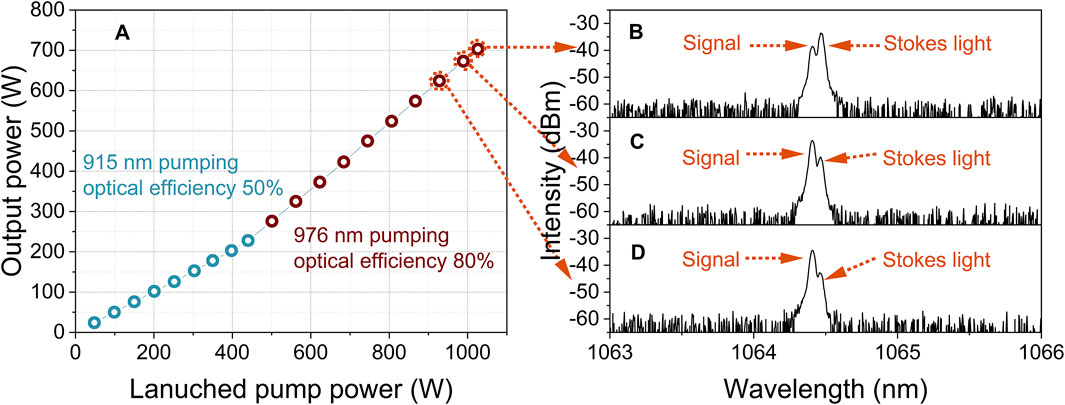
FIGURE 2. (A) Laser output power evolution of the single-frequency Yb3+-doped all-fiber amplifier as a function of 915 nm pump power (blue dot) and 976 nm pump power (red dot), (B)–(D) backward optical spectra at output power of 703 W, 674, and 620 W.
Figure 3A shows the Fourier transform spectra of temporal laser intensity measured at the output power of 650 and 703 W, respectively, for the single-frequency seed laser with a PER of 4.3 dB. There is no kHz-level oscillation frequency peak observed even at the maximum output power of 703 W, which manifests the absence of TMI effect [22]. Overall, higher single-frequency laser output power was only restrained by the SBS effect. It should be pointed that a few frequency spikes emerged below 1 kHz at the 703-W laser power was generated by the intensity noise of pump source induced by the current fluctuation of pump driver.
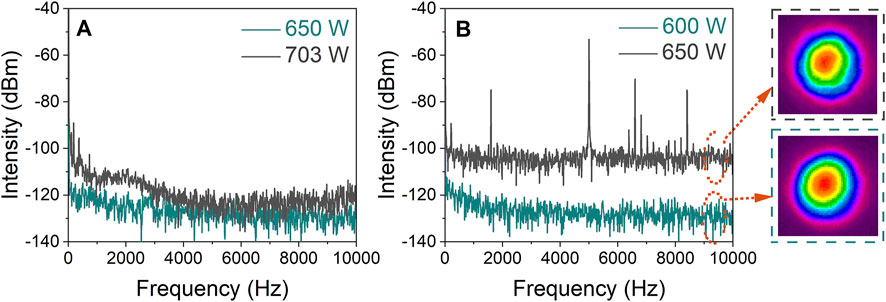
FIGURE 3. Fourier transform spectra of the temporal laser intensity (A) at the output power of 650 and 703 W with a laser seed PER of 4.3 dB, (B) at the output power of 600 and 650 W with a laser seed PER of 6.8 dB. Insets: Corresponding beam profiles recorded at laser power of 650 W (surrounded by gray dotted line) and 600 W (surrounded by green dotted line) with a laser seed PER of 6.8 dB.
The TMI and SBS threshold were also investigated under different PER of the single-frequency laser seed, and the corresponding maximum available output power is shown in Figure 4A. When the PER of the seed laser increased to 6.8 dB, TMI was observed before the onset of SBS. As shown in Figure 3B, when the laser power increased from 600 to 650 W, oscillation frequency peaks were seen with the interval in kHz level, which indicates the occurrence of TMI [22]. The beam profiles were also monitored by a CCD camera (Ophir, SP907) during the power amplification and beam profile distortion was observed at the laser power of 650 W as shown in the inset of Figure 3B, which confirms the presence of TMI. Compared with the case that laser seed PER was 4.3 dB, here the TMI threshold decreased more than 50 W. When the seed laser PER further increased from 6.8 to 14.5 dB, a continuous decrease of TMI threshold from 650 to 489 W was obtained. Since a depolarized signal laser could weaken the mode interference between the FM and HOMs, the strength of thermally induced refractive index grating (RIG) could be reduced correspondingly, thus enhancing the TMI threshold [16]. Therefore, a higher TMI threshold can be obtained here with a lower seed laser PER. It should be pointed out that, as the PER of the seed laser increased, the intensity of backward Stokes light was monitored and shown to be lower than that of backward Rayleigh scattering light at the power level in Figure 4A, which means that the SBS threshold was not reached before the onset of TMI.
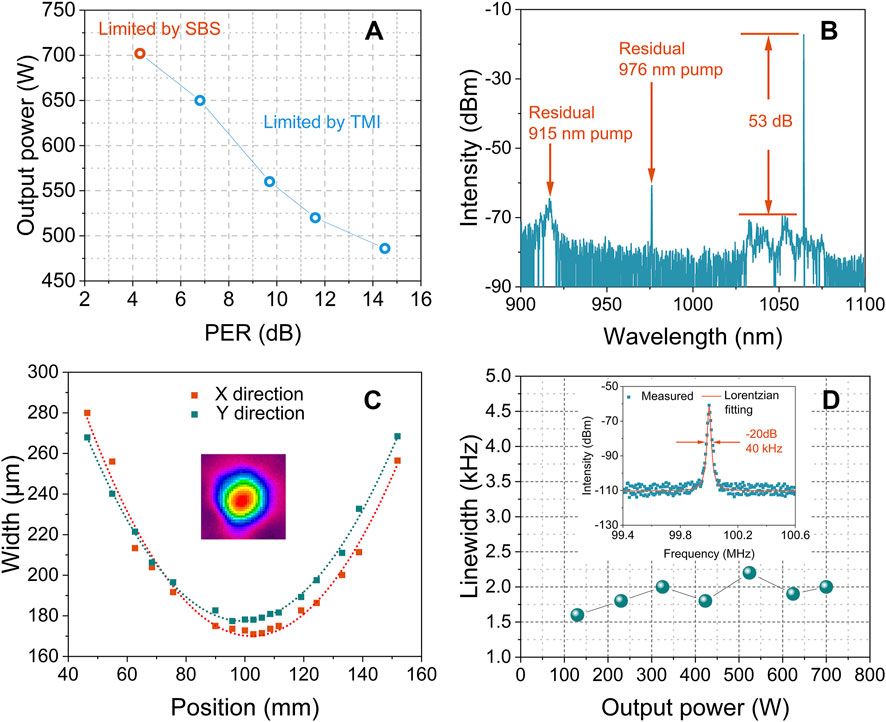
FIGURE 4. (A) The dependence of maximum available output power of the single-frequency fiber amplifier on the laser seed PER, (B) optical spectrum of the single-frequency fiber amplifier at the maximum output power of 703 W measured with an OSA resolution of 0.02 nm in the wavelength range from 900 to 1,100 nm, (C) measured beam quality (M2) in both axes at the maximum output power of 703 W and (D) measured laser spectral linewidth of the single-frequency fiber amplifier as a function of laser output power. Inset (C) Corresponding beam profile and (D) measured spectral linewidth at the maximum laser output power of 703 W.
The optical spectrum at the maximum output power of 703 W was measured by the OSA with a resolution of 0.02 nm, and is shown in a wavelength range from 900 to 1,100 nm in Figure 4B. The ASE, generated from the residual upper-level population due to the high pump absorption, can be observed between 1,030 and 1,055 nm. Considering the ASE component, the OSNR of the single-frequency fiber amplifier was still around 53 dB. By measuring the beam size at different positions using a CCD camera (Ophir, SP907) as shown in Figure 4C, the beam quality factor M2 was calculated to be 1.43 and 1.47 in x and y directions, respectively. The inset shows the beam profile recorded at the maximum laser power of 703 W.
The laser spectral linewidth during the power scaling was investigated by a delayed self-heterodyne interferometer (DSHI) with 36 km delay line. The optical beat signal was detected by a photodetector (Thorlabs, DET01CFC) and measured by a frequency spectrum analyzer (Agilent, N9030A) with a resolution bandwidth (RBW) of 3 kHz and video bandwidth (VBW) of 1 kHz. Figure 4D shows the measured laser spectral linewidth evolution as a function of the output power of the single-frequency amplifier, where significant linewidth broadening due to nonlinear effects, such as self-phase modulation and four-wave mixing [23, 24], was not expected. A 20-dB linewidth of 40 kHz was achieved from the optical beat signal at the maximum laser power of 703 W, as shown in the inset of Figure 4D, which corresponded to a 3-dB laser linewidth of 2 kHz. The achieved kHz-level spectral linewidth at such high laser power confirms that the hybrid pump scheme and cascaded gain fiber structure effectively enhance the level of gain saturation, which reduces the generation of ASE component and suppresses the spectral linewidth broadening.
Conclusion
In conclusion, a 703-W single-frequency near-diffraction-limitation all-fiber amplifier at 1,064.4 nm was demonstrated with a cascaded Yb3+-doped fiber structure hybridly pumped at 915 and 976 nm in power scaling stage. The laser has an overall optical efficiency of 67.5% at the maximum output power of 703 W and the corresponding laser spectral linewidth was measured to be 2 kHz. To the best of our knowledge, this is the first time that a single-frequency all-fiber amplifier with kHz-level spectral linewidth was achieved at such high output power. The threshold of TMI and SBS effect were also investigated under different seed PER. The TMI effect exhibited lower threshold when the single-frequency seed laser was depolarized from linear-polarization to a state with PER of 6.8 dB, while lower seed PER of 4.3 dB resulted in the onset of SBS with a lower threshold, which is the condition of the demonstrated 703-W single-frequency laser power. Higher single-frequency laser output power can be anticipated with a circularly polarized seed laser.
Data availability statement
The raw data supporting the conclusions of this article will be made available by the authors, without undue reservation.
Author contributions
CS and XD contributed equally to this work. CS, XD, SF, and QS conceived the idea. CS and XD set up the experimental system. CS, XD, PJ, ZS, and YL measured the laser performance. CS, XD, SF, and QS wrote the manuscript. WS and JY supervised the project.
Funding
This work was supported by the National Natural Science Foundation of China (62105240, 62075159 and 61975146) and the Major Scientific and Technological Innovation Projects of Key R&D Plans in Shandong Province (2019JZZY020206).
Conflict of interest
The authors declare that the research was conducted in the absence of any commercial or financial relationships that could be construed as a potential conflict of interest.
Publisher’s note
All claims expressed in this article are solely those of the authors and do not necessarily represent those of their affiliated organizations, or those of the publisher, the editors and the reviewers. Any product that may be evaluated in this article, or claim that may be made by its manufacturer, is not guaranteed or endorsed by the publisher.
References
1. Wellmann F, Steinke M, Meylahn F, Bode N, Willke B, Overmeyer L, et al. High power, single-frequency, monolithic fiber amplifier for the next generation of gravitational wave detectors. Opt Express (2018) 27(20):28523–33. doi:10.1364/OE.27.028523
2. Wellmann F, Bode N, Wessels P, Overmeyer L, Neumann J, Willke B, et al. Low noise 400 W coherently combined single frequency laser beam for next generation gravitational wave detectors. Opt Express (2021) 29(7):10140–9. doi:10.1364/OE.420350
3. Fu S, Shi W, Feng Y, Zhang L, Yang Z, Xu S, et al. Review of recent progress on single-frequency fiber lasers. J Opt Soc Am B (2017) 34(3):A49–A62. doi:10.1364/JOSAB.34.000A49
4. Wang H, Pan J, Meng Y, Liu Q, Shen Y. Advances of Yb:CALGO laser crystals. Crystals (2021) 11:1131. doi:10.3390/cryst11091131
5. Ma Y, Shen Y, Xu L, Zhou T, Lin W, Gao Q, et al. High-power continuous-wave dual-wavelength diode-pumped Yb:YAG slab amplifier with temperature-dependent effects. Appl Phys B (2017) 123:258. doi:10.1007/s00340-017-6834-4
6. Shen Y, Gao G, Meng Y, Fu X, Gong M. Gain-phase modulation in chirped-pulse amplification. Phys Rev A (Coll Park) (2017) 96:043851. doi:10.1103/PhysRevA.96.043851
7. Bai Z, Yuan H, Liu Z, Xu P, Gao Q, Williams RJ, et al. Stimulated Brillouin scattering materials, experimental design and applications: A review. Opt Mater (Amst) (2018) 75:626–45. doi:10.1016/j.optmat.2017.10.035
8. Chen H, Bai Z, Yang X, Dong J, Qi Y, Yan B, et al. Enhanced stimulated Brillouin scattering utilizing Raman conversion in diamond. Appl Phys Lett (2022) 120:181103. doi:10.1063/5.0087092
9. Bai Z, Williams RJ, Kitzler O, Sarang S, Spence DJ, Wang Y, et al. Diamond Brillouin laser in the visible. APL Photon (2020) 5:031301. doi:10.1063/1.5134907
10. Shi C, Sheng Q, Fu S, Sun S, Zhang J, Shi W, et al. Power scaling and spectral linewidth suppression of hybrid Brillouin/thulium fiber laser. Opt Express (2020) 28(3):2948–55. doi:10.1364/oe.379013
11. Jeong Y, Nilsson J, Sahu JK, Payne DN, Horley R, Hickey LMB, et al. Power scaling of single-frequency ytterbium-doped fiber master-oscillator power-amplifier sources up to 500 W. IEEE J Sel Top Quan Electron (2007) 13(3):546–51. doi:10.1109/JSTQE.2007.896639
12. Jeong Y, Nilsson J, Sahu JK, Payne DN, Horley R, Hickey LMB, et al. 502 Watt, single transverse mode, narrow linewidth, bidirectionally pumped Yb-doped fiber amplifier. Opt Express (2007) 13(3):17044–551. doi:10.1364/OE.15.017044
13. Robin C, Dajani I. Acoustically segmented photonic crystal fiber for single-frequency high-power laser applications. Opt Lett (2011) 36(14):2641–3. doi:10.1364/OL.36.002641
14. Robin C, Dajani I, Pulford B. Modal instability-suppressing, single-frequency photonic crystal fiber amplifier with 811 W output power. Opt Lett (2014) 39(3):666–9. doi:10.1364/OL.39.000666
15. Lai W, Ma P, Liu W, Huang L, Li C, Ma Y, et al. 550 W single frequency fiber amplifiers emitting at 1030 nm based on a tapered Yb-doped fiber. Opt Express (2020) 28(14):20908–19. doi:10.1364/OE.395619
16. Jauregui C, Stihler C, Limpert J. Transverse mode instability. Adv Opt Photon (2020) 12(2):429–84. doi:10.1364/AOP.385184
17. Jauregui C, Eidam T, Otto H-J, Stutzki F, Jansen F, Limpert J, et al. Physical origin of mode instabilities in high-power fiber laser systems. Opt Express (2012) 20(12):12912–25. doi:10.1364/OE.20.012912
18. Hochheim S, Brockmüller E, Wessels P, Koponen J, Lowder T, Novotny S, et al. Single-frequency 336 W spliceless all-fiber amplifier based on a chirally-coupled-core fiber for the next generation of gravitational wave detectors. J Lightwave Technol (2022) 40(7):2136–43. doi:10.1109/JLT.2021.3133814
19. Matniyaz T, Bingham SP, Kalichevsky-Dong MT, Hawkins TW, Pulford B, Dong L. High-power single-frequency single-mode all-solid photonic bandgap fiber laser with kHz linewidth. Opt Lett (2022) 47(2):377–80. doi:10.1364/OL.449412
20. Stihler C, Jauregui C, Kholaif SE, Limpert J. Intensity noise as a driver for transverse mode instability in fiber amplifiers. PhotoniX (2020) 1:8. doi:10.1186/s43074-020-00008-8
21. Smith AV, Smith JJ. Increasing mode instability thresholds of fiber amplifiers by gain saturation. Opt Express (2013) 21(13):15168–82. doi:10.1364/OE.21.015168
22. Johansen MM, Laurila M, Maack MD, Noordegraaf D, Jakobsen C, Alkeskjold TT, et al. Frequency resolved transverse mode instability in rod fiber amplifiers. Opt Express (2013) 21(19):21847–56. doi:10.1364/OE.21.021847
23. Turitsyn SK, Bednyakova AE, Fedoruk MP, Latkin AI, Fotiadi AA, Kurkov AS, et al. Modeling of CW Yb-doped fiber lasers with highly nonlinear cavity dynamics. Opt Express (2011) 19(9):8394–405. doi:10.1364/OE.19.008394
Keywords: single-frequency fiber laser, high-power laser, stimulated brillouin scattering, transverse mode instability, spectral linewidth
Citation: Shi C, Deng X, Fu S, Sheng Q, Jiang P, Shi Z, Li Y, Shi W and Yao J (2022) 700 W single-frequency all-fiber amplifier at 1064 nm with kHz-level spectral linewidth. Front. Phys. 10:982900. doi: 10.3389/fphy.2022.982900
Received: 30 June 2022; Accepted: 25 July 2022;
Published: 29 August 2022.
Edited by:
Xuezong Yang, University of Chinese Academy of Sciences, ChinaReviewed by:
Huawei Jiang, (CAS), ChinaYijie Shen, University of Southampton, United Kingdom
Zhenxu Bai, Hebei University of Technology, China
Copyright © 2022 Shi, Deng, Fu, Sheng, Jiang, Shi, Li, Shi and Yao. This is an open-access article distributed under the terms of the Creative Commons Attribution License (CC BY). The use, distribution or reproduction in other forums is permitted, provided the original author(s) and the copyright owner(s) are credited and that the original publication in this journal is cited, in accordance with accepted academic practice. No use, distribution or reproduction is permitted which does not comply with these terms.
*Correspondence: Shijie Fu, shijie_fu@tju.edu.cn; Quan Sheng, shengquan@tju.edu.cn; Wei Shi, shiwei@tju.edu.cn