- Fine Optical Engineering Research Center, Chengdu, China
High-repetition rate laser-induced damage of Ta2O5:SiO2 coatings was investigated at 355 nm wavelength and a repetition rate of 30 kHz. Laser-induced damage thresholds of coatings with different mixture ratios were measured. The relationships between laser-induced damage threshold and the material band gap and defect absorption were analyzed. Laser-induced damage threshold decreased with increase in number of laser pulses owing to enhancement of the absorption. Enhancement of absorption is attributed to the increase in density of mid-state defects induced by the picosecond laser pulses. The relationship between mid-state defects and the material mixture ratio was also analyzed.
Introduction
In recent years, ultrafast high-repetition rate high-power laser systems have been the subject of intensive research because of their great potential for material modification and machining applications [1]–[3]. The optical coating of a high-power laser system is its weakest part with respect to laser-induced damage. The output power and lifetime of the laser system is, to a large degree, determined by the laser-induced damage threshold (LIDT) of the optical coating. Oxide coatings with low absorption and high optical band gap are suitable for high-power laser systems. With the increase of laser repetition rate and pulse energy, the LIDT has become the limiting property of laser systems. It is hence of great importance to research laser-induced damage mechanisms of optical coatings.
Mixed optical coatings with adjustable refractive index and optical band gap are an area of intense study. Based on innovative designs of oxide mixtures, graded index multilayer optical coatings with lower mechanical stress and a higher LIDT than those of classical multilayer coatings have been achieved owing to the absence of significant interfaces [4]–[9]. Research into laser-induced damage properties of mixed-oxide optical coatings has mainly focused on the damage mechanism of thin films under irradiation of a single ultrafast pulse (from 100 fs to 10 ps) based on band-gap and absorption parameters [10, 11]. Laser-induced damage characteristics of a series of mixed oxide (Al2O3:SiO2, Nb2O5:SiO2, HfO2:SiO2, Ta2O5:SiO2) and single oxide (Al2O3, Nb2O5, HfO2, SiO2, Ta2O5, ZrO2) coatings under 500 fs single-pulse laser irradiation have been investigated. The dominant damage mechanism of single-oxide optical coatings under a single ultra-short pulse laser is photoionization of the material caused by the ultra-high electric-field intensity and avalanche ionization. The damage processes are closely related to band-gap parameters of the optical coating [12, 13]; therefore, the LIDTs of single-oxide optical coatings under 500 fs laser pulse present a strong linear correlation with band-gap parameters. The LIDTs of some mixed-oxide coatings (HfO2:SiO2, Al2O3:SiO2) showed a linear correlation with energy-level parameters, but others (Ta2O5:SiO2, Nb2O5:SiO2, ZrO2:SiO2) did not show this characteristic. It was concluded that the damage characteristics of mixed-oxide films are not only affected by the intrinsic band-gap parameters of the materials. It is necessary to further study the defect characteristics of mixed-oxide coatings. A clear understanding of the relationship between single ultrafast pulse-laser damage characteristics and intrinsic parameters of mixed-oxide coatings has been presented [14]; however, the influence of defect parameters of mixed-oxide coatings on ultrafast pulse-laser damage characteristics needs investigation. For multi-shot high-repetition rate laser-induced damage properties of optical dielectric coatings, the mechanism is more complicated than that under a single shot [15]–[18]; in particular, the high-frequency ultrafast pulse-laser damage characteristics of mixed-oxide coatings remain to be further studied.
High-repetion rate picosecond laser-induced damage properties of mixed Ta2O5:SiO2 coatings were investigated at 355 nm wavelength and a frequency of 30 kHz. The mixed-oxide coating samples were fabricated by ion-beam sputtering. LIDTs were measured for several pulse durations. The LIDT decreased with increase of pulse duration owing to enhancement of the absorption. This enhancement of absorption is attributed to the increase in density of mid-state defects induced by the picosecond laser pulses. The relationship between mid-state defects and the material mixture ratio was also analyzed.
Experimental and materials
Laser-induced damage measurement setup
Laser-induced damage property measurement was carried out using the equipment shown in Figure 1A. A picosecond laser (355 nm wavelength, 5 ps full-width-at-half-maximum pulse, 30 kHz frequency) was focused on the sample surface to a diameter of 20 μm at an incident angle of 0°. In the LIDT measurement setup, an optical shutter with minimum opening time of 1 ms was used to control the number of pulses interacting with the sample. Pulse intensity was modulated by an attenuator. A small portion of the beam was deflected to an energy meter and beam profile to measure in situ the pulse energy and beam quality. A charge-coupled device camera was placed near the sample to monitor the surface damage event.
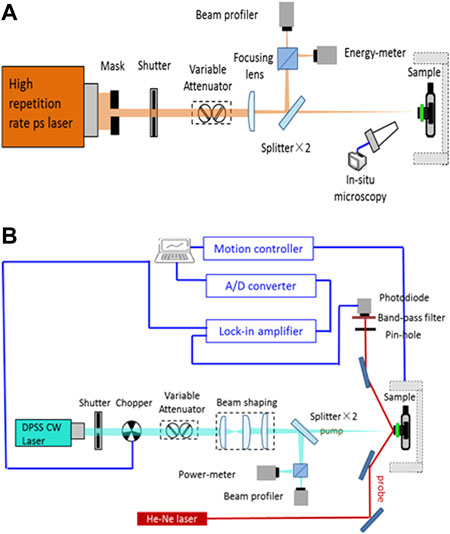
FIGURE 1. (A) High repetition rate picosecond laser induced damage experiment setup. (B) Photonthermal lens absorption measurement setup.
Fabrication of optical coating samples
Single-layer Ta2O5:SiO2 mixed coatings were deposited on fused-silica substrates with diameters of 25 mm by ion-beam sputtering. The fused-silica substrates were subjected to ultrasonic cleaning and optical polishing. The base pressure (<6.5 × 10−5 Pa) was produced using the depositing equipment, which comprised a mechanical pump and a cryogenic pump. A target with two zones of Ta (99.995% purity) and Si (99.999% purity) was sputtered by a radio-frequency ion source with high energy Xe gas (99.999% purity), as shown in Figure 2. A high-energy sputtered-ion beam was directed from the target toward the substrates to deposit Ta2O5:SiO2 mixed coatings. The oxygen flow rate was linearly varied from 75 to 100 standard-state cm3/min throughout the preparation process. The grown films were oxidized by introducing oxygen gas (99.999% purity) into the chamber. The deposition rates of the Ta2O5 and SiO2 films were 0.15 and 0.18 nm/s, respectively. A broad-band optical monitoring system was used to control the coating thickness. The target with two zones was moved from Ta to Si to obtain different mixture fractions of the two materials. A total of three different compositions were investigated, with silica fractions designed from 15% to 85% in increments of approximately 35%. The samples with mixed coatings containing approximately 15%, 50%, and 85% of the low-index material were labeled as “SiO2-high/Ta2O5-low,” “SiO2-half/Ta2O5-half,” and “SiO2-low/Ta2O5-high,” respectively.
Characterization methods
Spectra were measured using a Lambda 950 spectrophotometer. Absorption of the coating was measured using a photon thermal lens setup, as shown in Figure 1B [19]. Morphology of the optical coatings after laser irradiation was obtained by Nomarski microscopy (Leica-DMRXE).
Results and discussion
The optical thickness of the samples was approximately 550 nm at a wavelength of 355 mm. Measured transmittance spectra of the stacked layers of the Ta2O5:SiO2 mixed coatings at an angle near 0° are shown in Figure 3A. Refractive indices were calculated based on these spectra, as shown in Figure 3B. The contents of SiO2 and Ta2O5 were calculated using the Lorentz–Lorenz model, described by Eq. 1:
where na and nb are the refractive indices of the two pure materials, n is the refractive index of the Ta2O5:SiO2 mixed coating, and fa and fb are the volume fractions of the high- and low-index materials, respectively, where fa + fb = 1.
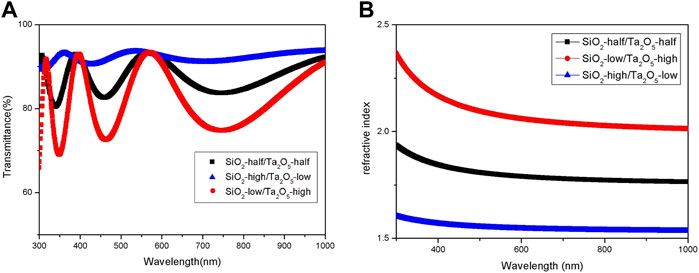
FIGURE 3. (A) Measured transmittance spectra of the stacked layers of the Ta2O5SiO2 mixed coatings. (B) Calculated refractive indices.
The SiO2 fractions of the SiO2-high/Ta2O5-low, SiO2-half/Ta2O5-half, and SiO2-low/Ta2O5-high samples were 90%, 44%, and 15%, respectively. The calculated contents differ from the design values because of deviations of the sputtering parameters. The ideal ion beam used in the deposition was gaussian shape. If the shape devited from gaussian shape, the coating material contents also changed, Band gaps for the different oxide mixtures were calculated from the refractive indices. Band gaps of mixed-oxide coatings change with the ratio of high- and low-index material contents. Band gaps of the SiO2-high/Ta2O5-low, SiO2-half/Ta2O5-half, and SiO2-low/Ta2O5-high samples were 7.16, 5.87, and 4.52 eV, respectively.
Figure 4 shows the LIDTs of the optical coatings under a frequency of 30 kHz for laser pulse durations from 1 to 50 ms. The LIDT measured spot number was 20 for each sample. The given LIDT was the average value of all the measure LIDTs. The irradiation spot size on the sample surface was 20 μm. As shown in Figure 4, the LIDT of the optical coatings decreased with increasing Ta2O5 content for all irradiation conditions. Under irradiation of a picosecond-pulse laser, free electrons can be ionized by multi-photon absorption. When the free electron density reaches 1021 cm−3, breakdown of the optical coating will occur. Coating mixtures with higher Ta2O5 content had a higher ionization rate, indicating that the probability of breakdown was also higher. In a previous report [1], the LIDT of optical coatings was found to be determined by intrinsic material properties (rather than by defects and impurities) when subjected to single-pulse laser irradiation with a pulse duration below 10 ps, and a linear dependency of threshold with band gap was observed. In our measurements, with irradiation by high-frequency ultrafast pulses with a duration of 5 ps, there was no significant linear correlation between the damage threshold and band gap of the thin coatings. It can be concluded that the laser-induced damage mechanism at high frequency is not only determined by intrinsic material properties, unlike the laser-induced damage mechanism for a single pulse: defects and impurities also play an important part.
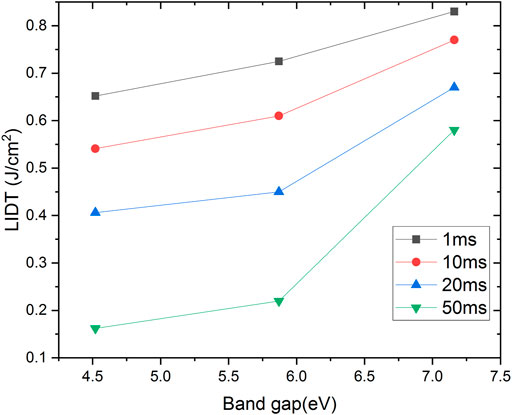
FIGURE 4. LIDTs of the optical coatings under repetition rate of 30 Khz with laserduration from 1 ms to 50 ms.
Figure 5 shows typical damage sites of the Ta2O5:SiO2 mixed coatings for irradiation times of 1, 10, 20, and 50 ms. It can also be concluded from the damage site morphology that laser-induced damage properties of mixed-oxide optical coatings by high-frequency ultrafast laser are influenced by both free electron generation and thermal effects. For a laser duration of less than 2 ms, the morphology shows that there was seldom melted material around the damage site (Figures 5A,B). The damage can be attributed to the electric field as a consequence of the high density of free electrons. A pulse duration of less than 2 ms is not long enough for laser energy accumulation to increase the temperature. For laser durations exceeding 10 ms, melted material was observed at the damage site (Figures 5C,D), indicating that the damage is partly attributed to the high temperature. Defects generated during the fabrication process enhance the absorption intensity of the coating. Under high-frequency picosecond laser irradiation, a portion of the pulse energy will be absorbed by the defect. For a single picosecond laser pulse, the thermal effect caused by the absorbed energy is small and will not cause fatal damage to the coating. For picosecond lasers with a frequency of 30 kHz, the time interval between pulses is 10−6 s. Because of the short time interval between laser pulses, the energy absorbed by the defect accumulates. As the number of laser pulses increases, the accumulated laser energy will increase the temperature. The coating could thus be melted. The probability of laser-induced damage also increases. Damage sites induced by high temperature are shown in Figures 5C,D. Heat accumulation may be partly induced by laser damage. With the increase of frequency and irradiation duration, the influence of the thermal effect was more significant.
We used the thermal lens method to investigate the relationship between defects and coating damage. Initial defect absorption characteristics of the samples were tested. To investigate mid-state defects generated during irradiation of the laser pulses, we also measured absorption of the coatings after laser irradiation with a pulse energy of 80% of the LIDT to ensure that the coating would not be destroyed. We measured absorption of areas conditioned by irradiation for 1, 2, 10, and 50 ms, as shown in Figure 6. The initial absorption distribution and absorption distribution after 50 ms laser conditioning in 1 mm × 1 mm measured area of sample with SiO2-half/Ta2O5-half are shown in Figure 7. It can be found that after the laser irradiation, the absorption intensity of some spots was greatly enhanced. The absorption intensity peak was attributed to the mid-state defects generated during laser irradiation. The extent of absorption enhancement was less for samples containing less Ta2O5. This indicated that less mid-state defects were generated in SiO2 than in Ta2O5 during the irradiation of high-frequency picosecond laser pulses. Laser energy deposition in the coating also increased with the increase of coating absorption. Owing to absorption enhancement, the sample with highest Ta2O5 content had the largest LIDT amplitude decrease with an increase in laser irradiation time.
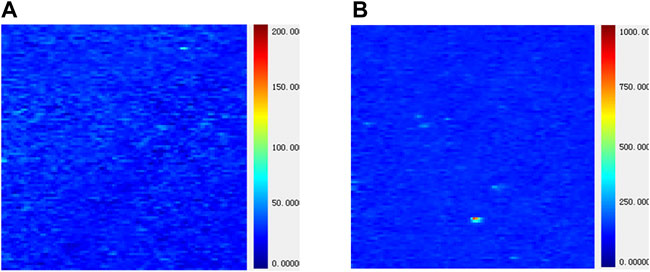
FIGURE 7. Absorption distribution of 1 mm × 1 mm measured area of sample with SiO2-half/Ta2O5-half (A) 0 ms (B) 50 ms.
Conclusion
High-repetition rate picosecond laser-induced damage properties of Ta2O5:SiO2 mixed coatings were investigated at 355 nm wavelength and a frequency of 30 kHz. Laser-induced damage thresholds of coatings with different mixture ratios were determined by both the material band gap and defect absorption. LIDT decreased with increase of frequency, owing to enhancement of the absorption. The enhancement of absorption is attributed to the increase in mid-state defects induced by the laser pulses. The relationship between mid-state defects and material mixture ratio was also investigated. Less mid-state defects were generated in SiO2 than in Ta2O5 during irradiation by high-frequency picosecond laser pulses. The absorption enhancement was higher for coatings with higher Ta2O5 content.
Data availability statement
The raw data supporting the conclusion of this article will be made available by the authors, without undue reservation.
Author contributions
MZ, FQ, ZQ, QZ, and YP: Conceptualization, Software, Investigation, Data curation, Funding acquisition, Writing—original draft preparation. BX, JD, and LL: Methodology, Writing—review and editing. PM: Resources and Supervision.
Funding
This work was supported by the National Natural Science Foundation of China (Grant No. 61905228).
Conflict of interest
The authors declare that the research was conducted in the absence of any commercial or financial relationships that could be construed as a potential conflict of interest.
Publisher’s note
All claims expressed in this article are solely those of the authors and do not necessarily represent those of their affiliated organizations, or those of the publisher, the editors and the reviewers. Any product that may be evaluated in this article, or claim that may be made by its manufacturer, is not guaranteed or endorsed by the publisher.
References
1 Baird BW. Picosecond laser processing of semiconductor and thin film devices. Proc.SPIE (2010) 7580:75800Q–19.
2 Jiang T, Koch J, Unger C, Fadeeva E, Koroleva A, Zhao Q, et al. Ultrashort picosecond laser processing of micro-molds for fabricating plastic parts with super hydrophobic surfaces. Appl Phys A (2012) 108:863–9. doi:10.1007/s00339-012-6985-4
3 Muhammad N, Whitehead D, Boor A, Oppenlander W, Liu Z, Li L. Picosecond laser micromachining of nitinol and platinum–iridium alloy for coronary stent applications. Appl Phys A (2012) 106:607–17. doi:10.1007/s00339-011-6609-4
4 Liu H, Chen S, Ma P, Pu Y, Qiao Z, Zhang Z, et al. Ion beam sputtering mixture films with tailored refractive indices. Opt Laser Technol (2014) 55:21–5. doi:10.1016/j.optlastec.2013.07.002
5 Melnikaits A, Tolenis T, Mazule L, Mirauskas J, Sirutkaitis V, Mangote B, et al. Characterization of zirconia– and niobia–silica mixture coatings produced by ion-beam sputtering. Appl Opt (2011) 50:C188–C196. doi:10.1364/ao.50.00c188
6 Zhou Q, Ma P, Qiu F, Pu Y, Zhao Q, Lv L, et al. Role of nano-precursors in ultraviolet-laser damage of HfO2/SiO2 mixture coatings. Thin Solid Films (2021) 739:138963. doi:10.1016/j.tsf.2021.138963
7 Pu Y, Kong P, Ma P, Lv L, Zhou Q, Zhang M, et al. The evolution of nano-precursors at film-substrate interface under nanosecond laser irradiation. Opt Laser Technol (2022) 151:108074. doi:10.1016/j.optlastec.2022.108074
8 Kong P, Pu Y, Ma P, Zhu J. The laser-induced damage mechanism of amorphous and crystalline Sc2O3 films. Vacuum (2021) 191:110332. doi:10.1016/j.vacuum.2021.110332
9 Kong P, Pu Y, Ma P, Zhu J. The characterization and properties of mixed Sc2O3/SiO2 films. Thin Solid Films (2020) 714:714138357. doi:10.1016/j.tsf.2020.138357
10 Mende M, Balasa I, Ehlers H, Ristau D, Douti D, Gallais L, et al. Relation of optical properties and femtosecond laser damage resistance for Al2O3/AlF3 and Al2O3/SiO2 composite coatings. Appl Opt (2014) 53:A383–A391. doi:10.1364/ao.53.00a383
11 Mangote B, Gallias L, Commandre M, Mende M, Jensen L, Ehlers H, et al. Femtosecond laser damage resistance of oxide and mixture oxide optical coatings. Opt Lett (2012) 37:1478–80. doi:10.1364/ol.37.001478
12 Stuart BC, Feit MD, Rubenchik AM, Shore BW, Perry MD. Laser-induced damage in dielectrics with nanosecond to subpicosecond pulses. Phys Rev Lett (1995) 74:2248–51. doi:10.1103/physrevlett.74.2248
13 Rethfelfd B. Unified model for the free-electron avalanche in laser-irradiated dielectrics. Phys Rev Lett (2004) 92:187401. doi:10.1103/physrevlett.92.187401
14 Jena S, Tokas R, Kamble N, Thakur S, Kamble N, Thakur S, et al. Optical properties and laser damage threshold of HfO2–SiO2 mixed composite thin films. Appl Opt (2014) 53:850–60. doi:10.1364/ao.53.000850
15 Nagy BJ, Gallais L, Vamos L, Oszetzky D, Racz P, Dombi P. Direct comparison of kilohertz- and megahertz-repetition-rate femtosecond damage threshold. Opt Lett (2015) 40:2525. doi:10.1364/ol.40.002525
16 Sozet M, Neauport J, Lavastre E, Roquin N, Gallais L, Lamaignere L. Laser damage growth with picosecond pulses. Opt Lett (2016)(41) 2342. doi:10.1364/ol.41.002342
17 Li Z, Du J, Zhao Y, Wang Y, Leng Y, Zhao J. Modeling the effect of nanosecond laser conditioning on the femtosecond laser-induced damage of optical films. Opt Express (2015) 23:14774. doi:10.1364/oe.23.014774
18 Chen S, Zhao Y, Li D, He H, Shao J. Effect of nanosecond laser pre-irradiation on the femtosecond laser-induced damage of Ta2O5/SiO2 high reflector. Appl Opt (2012) 51:1495. doi:10.1364/ao.51.001495
Keywords: high repetition rate picosecond laser, mixture oxide, optical coatings, laser damage, defect absorption
Citation: Zhang M, Qiu F, Qiao Z, Zhou Q, Pu Y, Xiong B, Die J, Lv L and Ma P (2022) High-repetition rate picosecond laser-induced damage properties of Ta2O5:SiO2 coatings. Front. Phys. 10:981024. doi: 10.3389/fphy.2022.981024
Received: 29 June 2022; Accepted: 15 July 2022;
Published: 13 October 2022.
Edited by:
Hongwei Chu, Shandong University, ChinaReviewed by:
Song Yue, Institute of Microelectronics (CAS), ChinaZhongben Pan, Max Born Institute for Nonlinear Optics and Short-Term Spectroscopy (LG), Germany
Saisai Chu, Peking University, China
Copyright © 2022 Zhang, Qiu, Qiao, Zhou, Pu, Xiong, Die, Lv and Ma. This is an open-access article distributed under the terms of the Creative Commons Attribution License (CC BY). The use, distribution or reproduction in other forums is permitted, provided the original author(s) and the copyright owner(s) are credited and that the original publication in this journal is cited, in accordance with accepted academic practice. No use, distribution or reproduction is permitted which does not comply with these terms.
*Correspondence: Mingxiao Zhang, OTk1NjYzNDJAMTYzLmNvbQ==