- School of Physics and Technology, University of Jinan, Jinan, China
Excellent Q-switching operations modulated by new two-dimensional (2D) saturable absorber (SA) materials with stable performance is a hot topic in all-solid-state pulsed laser research. In this work, the watt-level high-stability passive Q-switching operation in a solid-state Nd:YVO4 laser utilizing the 2D germanene nanosheets as SA was first realized. The nonlinear optical properties of the germanene nanosheets (Ge-Ns) were characterized by experimental means. The stable Q-switched pulse sequence was acquired with a 60.6 ns narrowest pulse width and a 528.6 kHz maximal repetition rate. The average output power of 0.965 W and the corresponding pulse peak power of 30.12 W are obtained under the pump power of 7 W. The findings of the experiments demonstrate that germanene material has remarkable nonlinear optical properties and can be used as an excellent saturable absorber in the field of optical pulse modulation.
Introduction
Short pulse solid-state Q-switched lasers have a very wide range of applications in military, material processing, clinical applications, and nonlinear optics due to their high pulse energy and simple manufacturing [1–3]. At present, the Q-switching technique can be divided into passive Q-switched and active Q-switched according to the control mode. By contrast, the passive Q-switched technology has the advantages of simple structure and low cost [4, 5]. However, the saturable absorber (SA) is one of the key devices of passively Q-switched lasers. Extensive research has been carried out to better SAs since the first successful commercialization of semiconductor saturable absorber mirrors (SESAMs) in 1992 [6]. So far, in addition to SESAMs, many new two-dimensional (2D) nanomaterials have also been gradually proved to have excellent saturable absorption characteristics, such as graphene [7, 8], carbon nanotubes [9–11], topological insulators (TIs) [12, 13], transition metal dichalcogenides (TMDs) [14–17], MXenes [18, 19], Xenes [20–22], and black phosphorus (BP) [23–25]. Despite the fact that these 2D materials have been employed effectively as broadband SAs, there are still some drawbacks that limit their applications in all-solid-state lasers. For example, the smaller the modulation depth (MD) (∼2.3% for monolayer) of graphene, the larger the bandgap of TMDs, and the poorer the stability of BP [26–28]. Therefore, the exploration of new SA materials with better performance is necessary for Q-switched or mode-locking lasers.
As a germanium-based analog of graphene, germanene has been theoretically and experimentally proven to have the advantages of environmental friendliness, facile fabrication, and broadband absorption, indicating promising characteristics for optoelectronic fields [29]. In 2009, Cahangirov et al. first demonstrated that germanium has a stable honeycomb structure [30]. In 2014, Li et al. experimentally synthesized 2D germanene sheets on the Pt surface by electron beam evaporation technology [31]. In the same year, atom-thin, ordered 2D multiphase germanium film was prepared by Davila et al. using molecular beam epitaxy technology on the surface of gold [32]. In 2022, Sun et al. for the first time used germanene nanoplates as SA to realize the harmonic mode-locking operation with the order of fundamental frequency, second, fourth, fifth, and sixth based on the germanene–PVA film in an erbium-doped fiber laser [33]. Very recently, by the liquid-phase exfoliated method, the germanene nanosheets are prepared and used for the generation of ultrashort pulses in fiber lasers at 1,061.1, 1,559.3, and 1883.5 nm, respectively [34]. As a natural quantum well structure, the semiconductor layer of germanene acts as a reservoir, and the carriers in the layer have a substantially shorter relaxation period [29]. The optical bandgap of the germanium nanosheets has been calculated to be about 2.46 eV, corresponding to the wavelength of ∼506 nm [34]. The broadband saturable absorption of the germanene nanosheets indicates the existence of sub-bandgap absorption, which may be caused by the high edge to surface area ratio of 2D germanene nanosheets [35]. Moreover, atomic vacancy defects can also reduce the bandgap and hence make contributions to the sub-bandgap absorption [36]. The nonlinear absorption coefficient of germanene nanosheets is much higher than that of graphene and has superior environmental durability to BP [37]. All the studies so far show that germanene is a kind of optical material with excellent properties. However, the research on the pulse modulation characteristics of germanene in solid-state lasers is still insufficient, and the relevant research on germanene in solid-state Q-switched lasers has not been found yet.
In this work, using self-made germanene nanosheets (Ge-Ns) as SA, a passively Q-switched Nd:YVO4 laser with high stability was realized for the first time. The nonlinear optical parameters of the germanene nanosheets are characterized. Stable Q-switched pulses were obtained with the narrowest pulse width of 60.6 ns and the corresponding repetition rate of 528.6 kHz at the pump power of 7 W. The single pulse energy and peak power of 1.83 μJ and 30.12 W are calculated, respectively. As far as we know, this is the first time that the saturable absorption feature of germanene SA in a solid-state laser was demonstrated, indicating its capability of generating high stable Q-switched pulses in an all-solid-state laser.
Preparation and characterization of germanene saturable absorber
Using the liquid-phase exfoliated (LPE) method, the preparation process of 2D Ge-Ns is similar to our previous study [15]. The 2D germanene dispersion is ultrasonic for 10 h and centrifuged for 15 min at 5,000 rpm to remove the large size germanium crystals. Then, the desired 2D Ge-N solution was obtained. The rotary coating evaporation technology with a spin coating speed of 300 rpm was used to deposit Ge-Ns on the facet of an uncoated sapphire substrate.
The microscopic morphology and saturable absorption properties of the Ge-Ns are characterized. Figure 1A shows the configuration of the surface of the Ge crystal powder sample as seen through a field-emission scanning electron microscope (SEM, Sigma 500, Zeiss). The picture shows solid agglomerates of several microns with distinct layered structures which include the cleavage plane and cleavage step, indicating that the bonding force between layers is a weak van der Waals force. Figure 1B shows a picture of the prepared Ge-Ns measured using a high-resolution transmission electron microscope (HRTEM, JEM-2100). It can be seen that Ge-Ns are very thin and almost transparent. At a resolution of 50 nm, the layered structure of Ge-Ns can be easily observed, which is very different from Figure 1A. Changing the HRTEM resolution to 10 nm, the lattice spacing of 3.1 Å of the few-layer Ge-Ns can be observed from the HRTEM image, as shown in Figure 1C. The atomic force microscope (AFM, Bruker Multimode 8) was used to measure the thickness of the Ge-N sample. Figure 1D exhibits the obtained AFM image. The corresponding thickness profile of the sample is given in Figure 1E. It can be found that the average thickness of the sample is about ∼ 4 nm, corresponding to 13 layers [38].
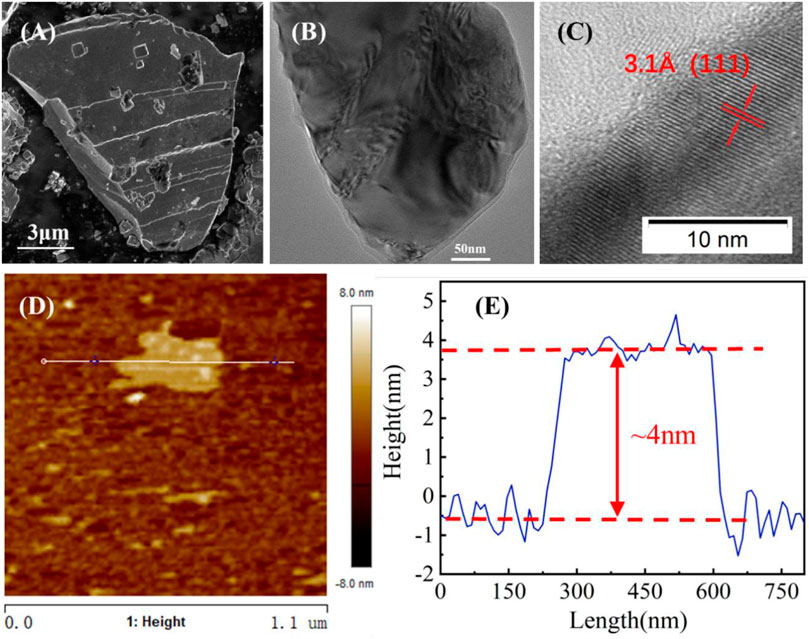
FIGURE 1. Characterizations of few-layer Ge-Ns. (A) SEM image of the germanene crystal powder sample; (B,C) HRTEM image with higher resolution; (D) AFM images; and (E) corresponding thickness profile of Ge-Ns.
Figures 2A,B show the UV-Vis-NIR absorption spectrum and Raman spectrum of the Ge-N sample. The broadband absorption characteristic curve is measured from 400 to 1600 nm using a UV-Vis-NIR spectrophotometer. At 1064 nm, the light absorption rate of the Ge-N sample is 9.1%. The Raman spectrum was measured to analyze the chemical structure and vibration mode of the Ge-N sample. From the diagram, the prominent peak (E2g) at 304.4 cm−1 can be observed, which is consistent with the previous reports [29, 34].
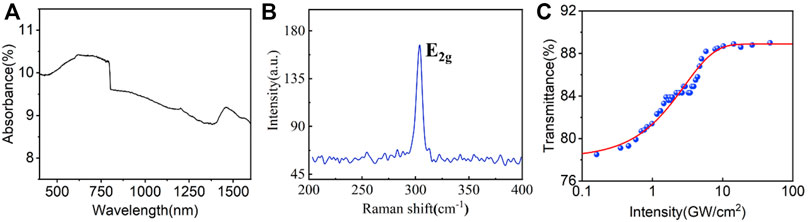
FIGURE 2. (A) Absorption spectrum; (B) Raman spectrum; and (C) nonlinear optical response of the Ge-N SA.
The nonlinear transmittance of Ge-N SA is measured by the double-optical-path method [15]. A solid-state Q-switched laser at 1.06 µm (120 ns pulse width, 15 kHz 95 repetition rate) was used as the laser source for testing. The experimental data of transmission T(I) shown in Figure 2C with symbols are fitted by the formula as follows:
Here, I and Isat are the input intensity of the laser and saturation intensity of Ge-N SA, respectively, and ∆T is the modulation depth with a fitting value of 11.6%. The nonsaturable loss Tns is about 12.2%.
Experimental setup and results
Experimental setup
Figure 3 shows the experimental setup for studying the lasing characteristics of Q-switched pulses using a passively Q-switched Nd:YVO4 laser with Ge-N SA. To accomplish stable and effective Q-switching, a conventional 3-cm-long plane–plane cavity was used. A fiber-coupled laser diode (LD) emitting 808 nm was used as the pump source. Using an optical imaging system with a 1:1 imaging ratio, the pump beam with a spot radius of 200 µm was focused into a 3 mm3 × 3 mm3 × 8 mm3 Nd:YVO4 crystal. The Nd:YVO4 crystal with the Nd-doping concentration of 0.5 at% was antireflection (AR) coated at 808 and 1,064 nm for a facet and AR coated at 1,064 nm for the other facet. As mature laser media for diode-pumped solid-state lasers, neodymium-doped crystals owe broad absorption bands and large emission cross sections as well as high environmental stability, which are beneficial to generate high peak power and narrow pulse width [39, 40]. M1 is a plane mirror with AR coated at 808 nm and high reflection (HR) coated at 1,064 nm. For the plane output coupler (OC) M2, the transmission (T) at 1,064 nm is 5%. The germanene SA was placed as close to the OC as possible to provide steady and effective Q-switching. A long-pass filter was also put behind the OC to reduce the residual pump power. The temporal pulse morphology was recorded using a digital oscilloscope (Tektronix DPO 4104B, United States) with a fast photo-detector (Thorlabs DET08 C/M, United States).
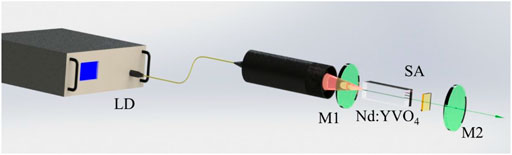
FIGURE 3. Schematic setup of the Nd:YVO4 laser with germanene as the saturable absorber. LD: laser diode; SA: Ge-N saturable absorber.
Experimental results and discussion
In the Nd:YVO4 laser shown in Figure 3, the stable Q-switched laser oscillation based on Ge-N SA is realized when the pump power is increased above 1.8 W. As the pump power gradually increases, the output characteristics of the continuous wave (CW) and passively Q-switched pulses are measured.
The output power of CW and Q-switched pulses is shown in Figure 4A as a function of pump power. With the pump power of 7 W and the output coupler of 5%, the CW average output power is 1.762 W with the corresponding slope efficiency of 26%. Then, the Ge-N SA is put into the resonant cavity, and the average output powers of the passively Q-switched operation are recorded. At the pump power of 7 W, the maximum average output power of 0.965 W with the corresponding slope efficiency of 16.4% can be obtained. We have also been focusing on the state of Ge-N SA with increasing pump power. When the pump power reaches 7 W, the fluctuation of the average output power is measured at about 2.8%. By observation, there is no damage to Ge-N SA, and the distribution of Ge-Ns on the substrate seems to be uniform.
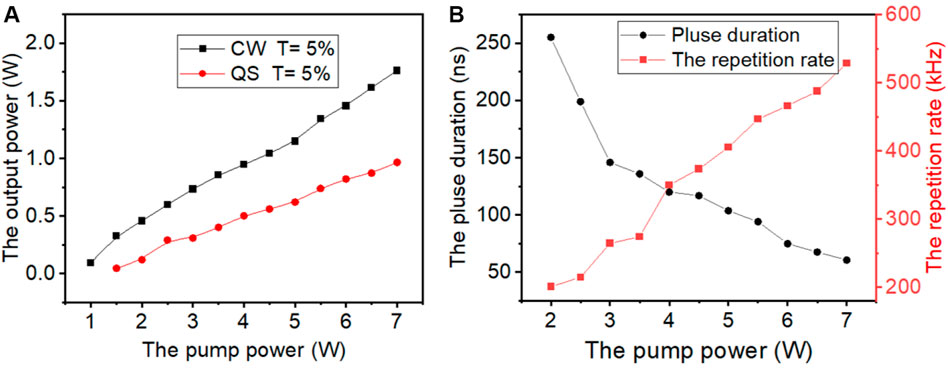
FIGURE 4. (A) Average output power. (B) Pulse duration and pulse repetition rate of the Ge-N SA-based Q-switched Nd:YVO4 laser versus the pump power.
The pulse duration and repetition rate of the Ge-N-based Q-switched laser are measured and exhibited in Figure 4B. With the pump power ranging from 2 to 7 W, the pulse repetition rate gradually increases from 200.9 to 528.6 kHz, and the pulse width gradually narrows from 255 to 60.6 ns. This trend is consistent with the principle of passively Q-switched pulses [41].
The peak power and single pulse energy of passively Q-switched pulses are calculated by the measured pulse width, repetition rate, and average output power. Figure 5 shows the relationship between peak power and pulse energy and pump power. The maximum peak power of 30.12 W and single pulse energy of 1.83 μJ are obtained at the pump power of 7 W.
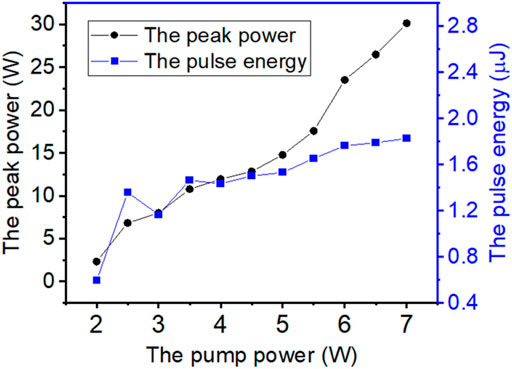
FIGURE 5. Peak powers and pulse energies of the Ge-N SA-based Q-switched Nd:YVO4 laser versus the pump power.
Figures 6A,B show the pulse train and single Q-switched pulse diagrams of the Ge-N SA-based Q-switched Nd:YVO4 laser for a pump power of 2.5, 4.5, and 7 W, respectively. To further validate the stability of the Q-switching characteristics of germanene, the Q-switched laser based on Ge-Ns SA ran 6 h a day for 5 days under the pump power of 7 W. The Q-switched pulses keep high repeatability, and the amplitude fluctuation is less than 7%. By employing the 90.0/10.0 scanning-knife-edge method, the beam quality factor M2 of the Q-switched laser based on Ge-N SA was also measured to further demonstrate the robustness of the Q-switching system. In the horizontal and longitudinal planes, the calculated Mx2 and My2 are 1.96 and 1.51, respectively.
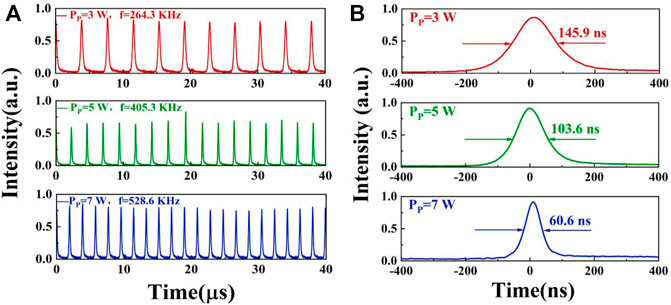
FIGURE 6. (A) Pulse sequence and (B) single pulse profiles of the passively Q-switched laser under the pump power of 3 W, 5 W, and 7 W.
Table 1 lists some key parameters of all-solid-state Q-switched laser using different 2D nanomaterials as SA at 1,064 nm. It can be found that the single pulse energy and pulse peak power produced by germanene SA are larger than those of most other two-dimensional materials. The results show that germanene has good nonlinear optical properties and is suitable for generating stable Q-switched pulses with high peak power in a solid-state laser.
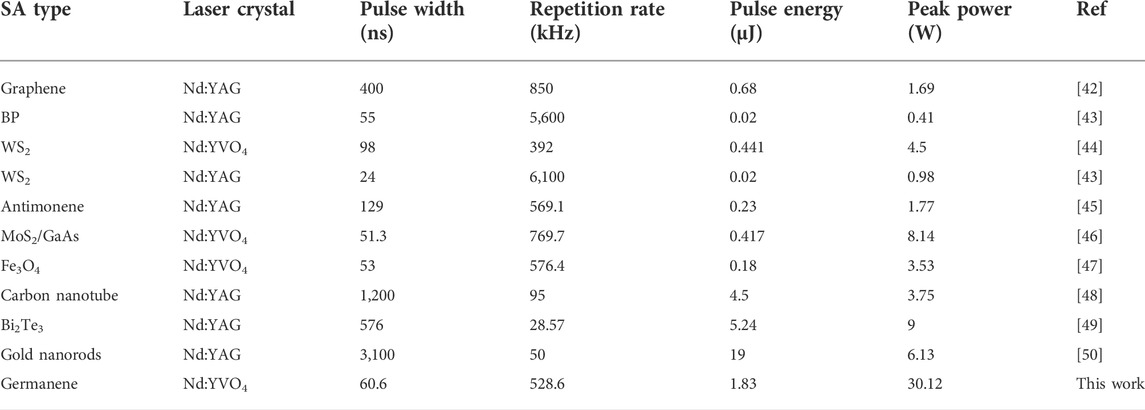
TABLE 1. Passively Q-switching performances for the 1064 nm solid-state laser with different 2D SA materials.
Conclusion
In summary, a 4-nm-thick germanene nanosheet was fabricated successfully by the LPE method and applied as SA to generate a Q-switched pulse in Nd:YVO4 all-solid-state lasers for the first time. The nonlinear optical parameters of the germanene nanosheet at 1,064 nm are measured by experimental methods. The stable Q-switching operation with large single pulse energy and high peak power is realized. The shortest pulse width of 60.6 ns was obtained with a maximum repetition rate of 528.6 kHz, and the associated single pulse energy and peak power are 1.83 μJ and 30.12 W, respectively, when the pump power is 7 W. The results indicate that germanene has excellent optical nonlinear properties and has broad application prospects in the field of all-solid-state laser.
Data availability statement
The original contributions presented in the study are included in the article/Supplementary Material; further inquiries can be directed to the corresponding authors.
Author contributions
BX and WT conceived and designed the experiments, performed the experiments and analyzed the data, and drafted the manuscript; WS and JW contributed to performing the theoretical analysis; XH and KJ fabricated and characterized the saturable absorber; WX provided experimental equipment, and all authors contributed to writing and editing the manuscript. All authors read and agreed to the published version of the manuscript.
Funding
This research was funded by the National Natural Science Foundation of China, under grant number 62005094; Program of Jinan Introduced Innovation Team, under grant number 2018GXRC011; Natural Science Foundation of Shandong Province, under grant number ZR2021MF128; and Doctoral Fund Project from the University of Jinan, under grant number XBS1917.
Conflict of interest
The authors declare that the research was conducted in the absence of any commercial or financial relationships that could be construed as a potential conflict of interest.
Publisher’s note
All claims expressed in this article are solely those of the authors and do not necessarily represent those of their affiliated organizations, or those of the publisher, the editors, and the reviewers. Any product that may be evaluated in this article, or claim that may be made by its manufacturer, is not guaranteed or endorsed by the publisher.
References
1. Byer RL. Diode laser-pumped solid-state lasers. Science (1988) 239(4841):742–7. doi:10.1126/science.239.4841.742
2. Juhasz T, Lai ST, Pessot MA. Efficient short-pulse generation from a diode-pumped Nd:YLF laser with a piezoelectrically induced diffraction modulator. Opt Lett (1990) 15(24):1458–60. doi:10.1364/ol.15.001458
3. Ma YF, Zhang Y, Yu X, Li XD, Chen F, Yan RP, et al. Doubly Q-switched GdVO4/Nd:GdVO4 laser with AO modulator and Cr4+:YAG saturable absorber under direct 879nm diode pumping to the emitting level. Opt Commun (2011) 284:2569–72. doi:10.1016/j.optcom.2011.01.048
4. Zhang S, Huang H, Xu L, Wang M, Chen F, Xu J, et al. Continuous wave and passively Q-switched Nd:LuxY1-xVO4 laser at 1.34 µm with V3+ :YAG as the saturable absorber. Opt Express (2011) 19(3):1830–5. doi:10.1364/oe.19.001830
5. Ma YF, Zhang SC, Ding SJ, Liu XX, Yu X, Peng F, et al. Passively Q-switched Nd:GdLaNbO4 laser based on 2D PdSe2 nanosheet. Opt Laser Technol (2020) 124:105959. doi:10.1016/j.optlastec.2019.105959
6. Keller U, Miller DAB, Boyd GD, Chiu TH, Ferguson JF, Asom MT, et al. Solid-state low-loss intracavity saturable absorber for Nd:YLF lasers: an antiresonant semiconductor fabry-perot saturable absorber. Opt Lett (1992) 17(7):505–7. doi:10.1364/ol.17.000505
7. Cho W, Kim J, Lee H, Bae S, Hong B, Choi S, et al. High-quality large-area monolayer graphene for efficient bulk laser mode-locking near 1.25 μm. Opt Lett (2011) 36:4089–91. doi:10.1364/ol.36.004089
8. Tang WJ, Zhao J, Yang KJ, Zhao SZ, Li GQ, Li DC, et al. 1.38 MW peak power dual-loss modulated sub-nanosecond green laser with EO and graphene. Opt Mater (Amst) (2016) 62:23–7. doi:10.1016/j.optmat.2016.09.036
9. Yim JH, Cho WB, Lee S, Ahn YH, Kim K, Lim H, et al. Fabrication and characterization of ultrafast carbon nanotube saturable absorbers for solid-state laser mode-locking near 1μm. Appl Phys Lett (2008) 93:161106. doi:10.1063/1.2999593
10. Yang Q, Wang Y, Liu D, Liu J, Zheng L, Su L, et al. Fabrication and characterization of double-wall carbon nanotube absorber for passive mode-locked Nd:GdVO4 laser. Laser Phys (2011) 21:1689–93. doi:10.1134/s1054660x11170233
11. Lin XC, Zhang L, Tsang YH, Wang YG, Yu HJ, Yan SL, et al. Multi-walled carbon nanotube as a saturable absorber for a passively mode-locked Nd:YVO4 laser. Laser Phys Lett (2013) 10:055805. doi:10.1088/1612-2011/10/5/055805
12. Liu H, Zheng XW, Liu M, Zhao N, Luo AP, Luo ZC, et al. Femtosecond pulse generation from a topological insulator mode-locked fiber laser. Opt Express (2014) 22(6):6868–73. doi:10.1364/oe.22.006868
13. Liu X, Yang K, Zhao S, Li T, Qiao W, Zhang H, et al. High-power passively Q-switched 2 μm all-solid-state laser based on a Bi2Te3 saturable absorber. Photon Res (2017) 5:461–6. doi:10.1364/prj.5.000461
14. Zhang H, Lu SB, Zheng J, Du J, Wen SC, Tang DY, et al. Molybdenum disulfide (MoS2) as a broadband saturable absorber for ultra-fast photonics. Opt Express (2014) 22:7249–60. doi:10.1364/oe.22.007249
15. Tang W, Wang Y, Yang K, Zhao J, Zhao S, Li G, et al. 1.36 W passively Q-switched YVO4/Nd:YVO4 laser with a WS2 saturable absorber. IEEE Photon Technol Lett (2017) 29(5):470–3. doi:10.1109/lpt.2017.2657325
16. Fan MQ, Li T, Zhao SZ, Li GQ, Ma HY, Gao XC, et al. Watt-level passively Q-switched Er:Lu2O3 laser at 2.84μm using MoS2. Opt Lett (2016) 41(3):540–3. doi:10.1364/ol.41.000540
17. Fan M, Li T, Zhao J, Zhao S, Li G, Yang K, et al. Continuous wave and ReS2 passively Q-switched Er:SrF2 laser at ∼3 μm. Opt Lett (2018) 43(8):1726–9. doi:10.1364/ol.43.001726
18. Cao LH, Chu HW, Pan H, Wang R, Li Y, Zhao SZ, et al. Nonlinear optical absorption features in few-layered hybrid Ti3C2(OH)2/Ti3C2F2 MXene for optical pulse generation in the NIR region. Opt Express (2020) 28(21):31499–509. doi:10.1364/oe.406035
19. Jhon YI, Koo J, Anasori B, Seo M, Lee JH, Gogotsi Y, et al. Metallic MXene saturable absorber for femtosecond mode-locked lasers. Adv Mater (2017) 29(40):1702496. doi:10.1002/adma.201702496
20. Xu N, Ma P, Fu S, Shang X, Jiang S, Wang S, et al. Tellurene-based saturable absorber to demonstrate large-energy dissipative soliton and noise-like pulse generations. Nanophotonics (2020) 9(9):2783–95. doi:10.1515/nanoph-2019-0545
21. Xing C, Xie Z, Liang Z, Liang W, Fan T, Ponraj JS, et al. Selenium nanosheets: 2D nonlayered selenium nanosheets: Facile synthesis, photoluminescence, and ultrafast photonics (advanced optical materials 24/2017). Adv Opt Mater (2017) 5(24):1770118. doi:10.1002/adom.201770118
22. Lu L, Liang Z, Wu L, Chen Y, Song Y, Dhanabalan SC, et al. Few-layer bismuthene: sonochemical exfoliation, nonlinear optics, and applications for ultrafast photonics with enhanced stability. Laser Photon Rev (2018) 12:1700221. doi:10.1002/lpor.201700221
23. Lu SB, Miao LL, Guo ZN, Qi X, Zhao CJ, Zhang H, et al. Broadband nonlinear optical response in multi-layer black phosphorus: an emerging infrared and mid-infrared optical material. Opt Express (2015) 23(9):11183–94. doi:10.1364/oe.23.011183
24. Zhang B, Lou F, Zhao R, He J, Li J, Su X, et al. Exfoliated layers of black phosphorus as saturable absorber for ultrafast solid-state laser. Opt Lett (2015) 40(16):3691–4. doi:10.1364/ol.40.003691
25. Tang W, Zhao J, Li T, Yang K, Zhao S, Li G, et al. High peak power mode-locking pulse generation in a dual-loss-modulated laser with BP-SA and EOM. Opt Lett (2017) 42(23):4820–3. doi:10.1364/ol.42.004820
26. Sun Z, Hasan T, Torrisi F, Popa D, Privitera G, Wang F, et al. Graphene mode-locked ultrafast laser. ACS Nano (2010) 4:803–10. doi:10.1021/nn901703e
27. Favron A, Gaufres E, Fossard F, Phaneuf-L’Heureux AL, Tang NYW, Levesque PL, et al. Photooxidation and quantum confinement effects in exfoliated black phosphorus. Nat Mater (2015) 14:826–32. doi:10.1038/nmat4299
28. Mao D, Zhang S, Wang Y, Gan X, Zhang W, Mei T, et al. WS2 saturable absorber for dissipative soliton mode locking at 1.06 and 1.55 μm. Opt Express (2015) 23:27509–19. doi:10.1364/oe.23.027509
29. Li C, Kang J, Xie J, Wang Y, Zhou LI, Hu H, et al. Two-dimensional monoelemental germanene nanosheets: facile preparation and optoelectronic applications. J Mater Chem C Mater (2020) 8(46):16318–25. doi:10.1039/d0tc03892j
30. Cahangirov S, Topsakal M, Aktürk E, ahin HS, Ciraci S. Two- and one- dimensional honeycomb structures of silicon and germanium. Phys Rev Lett (2009) 102(23):236804. doi:10.1103/physrevlett.102.236804
31. Li L, Lu SZ, Pan J, Qin Z, Wang YQ, Wang Y, et al. Buckled germanene formation on Pt(111). Adv Mater (2014) 26(28):4820–4. doi:10.1002/adma.201400909
32. Dávila ME, Xian L, Cahangirov S, Rubio A, Le Lay G. Germanene: a novel two-dimensional germanium allotrope akin to graphene and silicene. New J Phys (2014) 16:095002. doi:10.1088/1367-2630/16/9/095002
33. Sun WG, Jiang K, Tang WJ, Su J, Chen K, Liu Q, et al. Germanene nanosheets for mode-locked pulse generation in fiber lasers. Infrared Phys Technol (2020) 123:104128. doi:10.1016/j.infrared.2022.104128
34. Wang Q, Kang J, Wang P, He J, Liu Y, Wang Z, et al. Broadband saturable absorption in germanene for mode-locked Yb, Er, and Tm fiber lasers. Nanophotonics (2022) 11:3127–37. doi:10.1515/nanoph-2022-0161
35. Trushin M, Kelleher EJR, Hasan T. Theory of edge-state optical absorption in two-dimensional transition metal dichalcogenide flakes. Phys Rev B (2016) 94:155301. doi:10.1103/physrevb.94.155301
36. Zhao G, Han S, Wang A, Wu Y, Zhao M, Wang Z, et al. ‘Chemical weathering’ exfoliation of atom-thick transition metal dichalcogenides and their ultrafast saturable absorption properties. Adv Funct Mater (2015) 25:5292–9. doi:10.1002/adfm.201501972
37. Mu H, Liu Y, Bongu SR, Bao X, Li L, Xiao S, et al. Germanium nanosheets with Dirac characteristics as a saturable absorber for ultrafast pulse generation. Adv Mater (2021) 33(32):2101042. doi:10.1002/adma.202101042
38. Galbiati M, Motta N, De CM, Camilli L. Group-IV 2D materials beyond graphene on nonmetal substrates: challenges, recent progress, and future perspectives. Appl Phys Rev (2019) 6(4):041310. doi:10.1063/1.5121276
39. Zhang HJ, Zhao SZ, Zhao J, Yang KJ, Li GQ, Li DC, et al. Generation of low repetition rate sub-nanosecond pulses in doubly QML Nd:Lu0.5Y0.5VO4 and Nd:YVO4 lasers with EO and transmission SSA. Opt Laser Tech (2015) 69:39–43. doi:10.1016/j.optlastec.2014.12.017
40. Ma YF, Yu X, Li XD, Fan RW, Yu JH. Comparison on performance of passively Q-switched laser properties of continuous-grown composite GdVO4/Nd:GdVO4 and YVO4/Nd:YVO4 crystals under direct pumping. Appl Opt (2011) 50(21):3854–9. doi:10.1364/ao.50.003854
41. Maker GT, Ferguson AI. Mode locking and Q switching of a diode laser pumped neodymium-doped yttrium lithium fluoride laser. Appl Phys Lett (1989) 54(5):403–5. doi:10.1063/1.100976
42. Wang Q, Wei ZY, Lin JJ, Zhang YD, Guo LW, Zhang ZG. Few-layer graphene as saturable absorber for Q-switched laser at sub-MHz repetition rate. In: Advances in optical materials. Washington: Optica Publishing Group (2011). paper AIThF3.
43. Tan Y, Guo Z, Ma L, Zhang H, Akhmadaliev S, Zhou S, et al. Q-switched waveguide laser based on two-dimensional semiconducting materials: tungsten disulfide and black phosphorous. Opt Express (2016) 24(3):2858–66. doi:10.1364/oe.24.002858
44. Zhang N, Zhang T, Wang Y, Song J. Compact stable passively Q-switched Nd: YVO4 laser with tungsten disulfide saturable absorber. Optik (2016) 127(19):7689–93. doi:10.1016/j.ijleo.2016.05.109
45. Wang MX, Zhang F, Wang ZP, Wu ZX, Xu XG. Passively Q-switched Nd3+ solid-state lasers with antimonene as saturable absorber. Opt Express (2018) 26(4):4085–95. doi:10.1364/oe.26.004085
46. Zhang Hk, Zhang F, Li X, Chen LJ, Wang J, Wang LL, et al. Passively Q-switched Nd:YVO4 laser with MoS2/GaAs saturable absorber. Opt Mater (Amst) (2017) 70:153–7. doi:10.1016/j.optmat.2017.05.033
47. Wang X, Wang YG, Mao D, Li L, Chen ZD. Passively Q-switched Nd:YVO4 laser based on Fe3O4 nanoparticles saturable absorber. Opt Mater Express (2017) 7(8):2913–21. doi:10.1364/ome.7.002913
48. Li CY, Bo Y, Zong N, Wang YG, Jiang BX, Pan YB, et al. LD-end-pumped passively Q-switched Nd:YAG ceramic laser with single wall carbon nanotube saturable absorber. Opt Laser Tech (2012) 44(7):2149–53. doi:10.1016/j.optlastec.2012.03.010
49. Lin YY, Lee P, Xu JL, Wu CL, Chou CM, Tu CY, et al. High-pulse-energy topological insulator Bi2Te3-based passive Q-switched solid-state laser. IEEE Photon J (2017) 8(4):1502710. doi:10.1109/jphot.2016.2581490
Keywords: Germanene, passively Q-switched, solid-state laser, high peak power, high stablity
Citation: Xu B, Tang W, Sun W, Wang J, Jiang K, Hu X and Xia W (2022) Watt-level high-stability all-solid-state passively Q-switched laser based on germanene nanosheets. Front. Phys. 10:972054. doi: 10.3389/fphy.2022.972054
Received: 17 June 2022; Accepted: 01 July 2022;
Published: 26 July 2022.
Edited by:
Yufei Ma, Harbin Institute of Technology, ChinaReviewed by:
Ruijun Lan, Yantai University, ChinaCunguang Zhu, Liaocheng University, China
Xiaohui Li, Shaanxi Normal University, China
Copyright © 2022 Xu, Tang, Sun, Wang, Jiang, Hu and Xia. This is an open-access article distributed under the terms of the Creative Commons Attribution License (CC BY). The use, distribution or reproduction in other forums is permitted, provided the original author(s) and the copyright owner(s) are credited and that the original publication in this journal is cited, in accordance with accepted academic practice. No use, distribution or reproduction is permitted which does not comply with these terms.
*Correspondence: Wenjing Tang, c3BzX3Rhbmd3akB1am4uZWR1LmNu; Wei Xia, c3BzX3hpYXdAdWpuLmVkdS5jbg==