- Laser Fusion Research Center, CAEP, Mianyang, China
In this letter, we report a diode-pumped nano-second laser with the output energy 10 J, the repetition rate 50 Hz, and the average power 500 W. The main amplifier was made up of eight amplify modules. Each amplify module was pumped by an 808 nm laser diode and cooled by water from the back end of the Nd:YAG slab. To our knowledge, this represents the highest pulse repetition rate for the 10 J class nano-second single-aperture Nd:YAG laser. The beam quality was controlled by means of mechanical design and adjustment and compensation by a home-made deforming mirror. The beam quality was controlled well with the beam quality of the laser 2.61DL.
Introduction
Diode-pumped re-frequency high-energy nano-second laser is one of the developing directions of the new generation of lasers and has a wide range of application prospects. It can be used for industrial applications and laboratory research, such as laser peening, pump source of Ti:sapphire femtosecond laser, high-energy density physics, strong field physics, high-energy and high-brightness X-ray source, or high-energy and high-brightness particle beams. Many industrial applications require high repetition of laser frequency to realize high processing rate.
In recent years, 10 Hz nano-second laser has developed rapidly, and the output energy had increased from 10 J to 100 J [1–7]. However, at that energy level, higher frequency just as 50 Hz or even 100 Hz was not realized before 2020. In 2018, we used Nd:YAG crystals as laser media and obtained 12 J–10 Hz laser output [8]. Taking the advantage of the low pumping intensity, the laser system has a large potential to increase the operation frequency to 50 Hz in 2020 [9]. In this paper, we introduce the details of the laser system to readers. We present the configuration of the laser, introduce the key problems of thermal management and ASE inhibition, and give the experimental results in this paper.
Experimental Setup
Figure 1 shows the schematic diagram of the laser system. A Q switch cavity with a side face pumped Nd:YAG rod was the seed. Two side face pumped rods were used as pre-amplifiers. The maximal output energy was 375 mJ. The beam was shaped by a glass diaphragm to a square with the output energy of about 190 mJ. The main amplifier was composed of eight water-cooled laser amplifier modules which were pumped from the back end by 808 nm LD arrays. The laser beam run double passes in the main amplifier and then output from the polaroid plate (P5). A deformable mirror (DM) was used to compress the phase aberration of the laser beam after the output polaroid plate (P5).
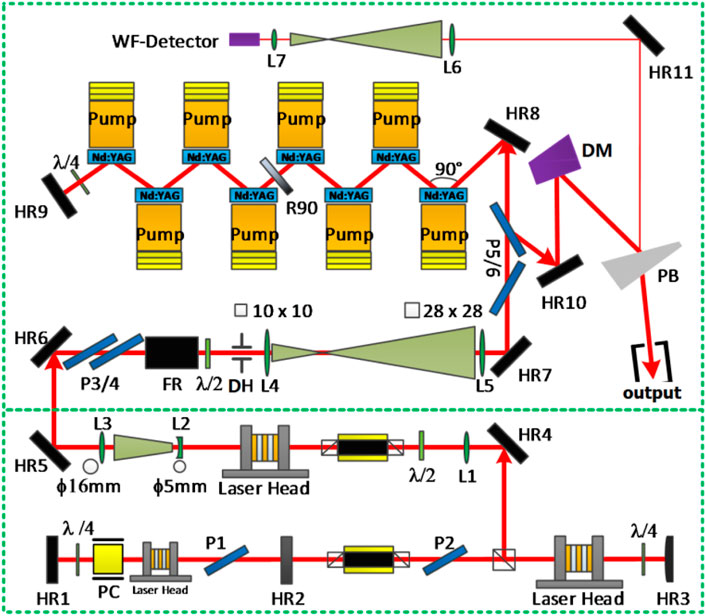
FIGURE 1. Schematic diagram of the 10 J–50 Hz laser system. HR is the high-reflection mirror. P is the polarization mirror. L is the lens. λ/4 is the 1/4 wave plate. FR is the Faraday rotator. λ/2 is the 1/2 wave plate. DM is the deformable mirror. DH is the diaphragm. WF-Detector is the wave front detector. PB is the sampling mirror with obliquity.
Key Issues of the Laser System
Thermal Management of the Amplifier
Under 50 Hz pumping, the thermal effect of the amplifier was significant. We present the thermal management in detail with theory and numerical simulation in our earlier paper [10]. The surface temperature of each crystal was measured, and the results are shown in Figure 2. The average surface temperature of each crystal was about 48–49°C. The surface temperature modulation in the pumping area of a typical surface was 1.05. The defocus of the double pass amplifier chain from thermal was as large as 35λ, which is shown in Figure 3. The defocus was pre-compressed by the image transfer lens (L4 and L5) before the laser seed injecting to the main amplifier. The focal length of L4 and L5 was 500 and 1500 mm, respectively, and we set the distance of them to 2400 mm to pre-compress the defocus. The astigmatism from each amplifier module was about 0.9λ. We adjusted the astigmatism at the laser head by mechanism. The residual astigmatism after adjustment was less than 0.1λ, which is shown in Figure 4. The depolarization of the amplifier chain was measured, and the result was 16% on a single pass. To compensate the depolarization, we put a 90° quartz rotator (R90) in the middle of the amplifier chain. We use 120 mJ energy as the measurement beam, and the minimum energy of the energy meter was 100 μJ. After compensation, the depolarization could not be measured by an energy meter, which means the depolarization must be lower than 0.1%.
ASE Control of the Laser Amplifier
The Nd:YAG crystal has a large emission cross area which makes the ASE very remarkable in a large area amplifier. The size of the Nd:YAG crystal was 60 mm × 40 mm × 8 mm, which was larger than that we used in 2018 [6]. Although the aperture was larger, the thickness was increased from 7 to 8 mm, which was a benefit to reduce ASE. The simulation results of different thicknesses and doping concentrations are shown in Figure 5. To obtain high energy conversion efficiency, we use a relatively high pumping power with a relatively short duration. The pumping current was 360 A, and the pumping duration was set to 140 μs with a rise edge of 20 μs. To absorb the ASE light, we use Cr4+:YAG as the edge cladding. The absorption coefficient and the width of Cr4+:YAG were 8/cm and 8 mm, respectively. In this condition, the small signal gain was measured and is shown in Figure 6.
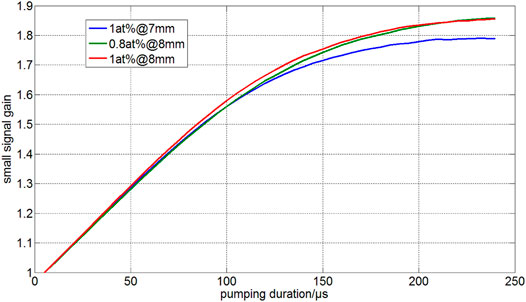
FIGURE 5. Simulation results of different thicknesses and doping concentrations to a small signal gain.
On the contrary, the edge cladding was used as a thermal balance method to obtain a good beam quality. The principle has been described in [7]. Figure 7 shows the near field of one pass of an amplifier without edge cladding and double pass of eight amplifiers with edge cladding.
Output Parameters of the Laser
The output parameters of the laser were measured. The output energy was 10 J with the stability of 0.8% (RMS@6000shots). The output energy is shown in Figure 7. The far field was compensated by adaptive optics (AO). The far field of the laser before and after compensation is shown in Figure 8. The beam quality of the laser was 11 diffraction limited (DL) and 2.6 DL before and after compensation, respectively. The origin of the side-lobes was the marginal residual high-frequency component which could not be compressed by AO. The near field is shown in Figure 9. The overall outline of beam distribution was supergauss with thin modulation on the top due to polishing of the crystal. The total modulation of the near field was 1.9.
Conclusion
In this letter, a laser beam of energy 10 J with the repetition rate of 50 Hz was realized. For such a high repetition rate, the thermal effect was controlled by uniform pumping and cooling, adjustment of astigmatism and compensation of defocus, and compensation of AO. The beam quality less than 3DL was realized. This confirms the viability of active mirror Nd:YAG amplifier concept, which is scalability to the kilowatt level. Further increases in the average power to 1000 W are expected at the frequency of 100 Hz.
Data Availability Statement
The original contributions presented in the study are included in the article/Supplementary Material, and further inquiries can be directed to the corresponding authors.
Author Contributions
XJ was responsible for overall laser design and thermal management. KX was responsible for overall laser integration. XY was responsible for laser energy flow design. ZW was responsible for pump coupling design. XJ was responsible for overall structural design. QX was involved in the development and commissioning of deformation mirror. WW was responsible for laser head design. JC was responsible for switch power supply development. CZ was involved in the development of soft-edge light appendix with high threshold. JZ was responsible for fluid homogenization design. ZP was responsible for miscellaneous light management. KZ was involved in clean management. PL was involved in self-shock inhibition. DH was involved in optical transmission design. QZ gave beam quality control guidance. WZ gave overall guidance.
FUNDING
The research was supported in part by the National Natural Science Foundation of China (NSFC) (Grant No. 62105310).
Conflict of Interest
The authors declare that the research was conducted in the absence of any commercial or financial relationships that could be construed as a potential conflict of interest.
Publisher’s Note
All claims expressed in this article are solely those of the authors and do not necessarily represent those of their affiliated organizations, or those of the publisher, the editors, and the reviewers. Any product that may be evaluated in this article, or claim that may be made by its manufacturer, is not guaranteed or endorsed by the publisher.
References
1. Bayramian A, Armstrong P, Ault E, Beach R, Bibeau C, Caird J, et al. The Mercury Project: A High Average Power, Gas-Cooled Laser for Inertial Fusion Energy Development. Fusion Sci Technol (2007) 52:383–7. doi:10.13182/fst07-a1517
2. Marrazzo S, Gonçalvès-Novo T, Millet F, Chanteloup JC. Low Temperature Diode Pumped Active Mirror Yb^3+:YAG Disk Laser Amplifier Studies. Opt Express (2016) 24(12):12651–60. doi:10.1364/oe.24.012651
3. Yasuhara R, Kawashima T, Sekine T, Kurita T, Ikegawa T, Matsumoto O, et al. 213 W Average Power of 24 GW Pulsed Thermally Controlled Nd:glass Zigzag Slab Laser with a Stimulated Brillouin Scattering Mirror. Opt Lett (2008) 33(15):1711–3. doi:10.1364/ol.33.001711
4. Mason P, Divoký M, Ertel K, Pilař J, Butcher T, Hanuš M, et al. Kilowatt Average Power 100 J-Level Diode Pumped Solid State Laser. Optica (2017) 4(4):438–9. doi:10.1364/optica.4.000438
5. Mason P, Banerjee S, Smith J, Butcher T, Phillips J, Höppner H, et al. Development of a 100 J, 10 Hz Laser for Compression Experiments at the High Energy Density Instrument at the European XFEL. High Pow Laser Sci Eng (2018) 6:e65. doi:10.1017/hpl.2018.56
6. Liu T, Sui Z, Chen L, Li Z, Liu Q, Gong M, et al. 12 J, 10 Hz Diode-Pumped Nd:YAG Distributed Active Mirror Amplifier Chain with ASE Suppression. Opt Express (2017) 25(18):21981. doi:10.1364/oe.25.021981
7. Liu T, Feng T, Sui Z, Liu Q, Gong M, Zhang L, et al. 50 Mm-Aperture Nd:LuAG Ceramic Nanosecond Laser Amplifier Producing 10 J at 10 Hz. Opt Express (2019) 27(11):15595. doi:10.1364/oe.27.015595
8. Jiang X, Wang Z, Yan X, Xue Q, Dai W, Wu W, et al. LD End-Pumped 12J-10Hz Nd:YAG Pulse Laser. Proc SPIE (2019) 2019:113330. doi:10.1117/12.2543001
9. Jiang X, Xiao K, wang Z, Yan XW, Jiang X, Xue Q, et al. Progress of 10J-50Hz Nd:YAG Laser[C]. In: LOT2020; Shanghai (2020).
Keywords: re-frequency pulse laser, high-energy laser, high conversion efficiency, thermal management, Nd:YAG laser
Citation: Jiang X, Xiao K, Yan X, Wang Z, Jiang X, Xue Q, Wu W, Chen J, Zhang C, Zheng J, Peng Z, Zheng K, Li P, Hu D, Zhu Q and Zheng W (2022) Diode-Pumped 50 Hz–10 J Nano-Second Nd:YAG Laser. Front. Phys. 10:923710. doi: 10.3389/fphy.2022.923710
Received: 19 April 2022; Accepted: 23 May 2022;
Published: 30 June 2022.
Edited by:
Xing Fu, Tsinghua University, ChinaReviewed by:
Yulei Wang, Hebei University of Technology, ChinaJing Wang, Shanghai Jiao Tong University, China
Copyright © 2022 Jiang, Xiao, Yan, Wang, Jiang, Xue, Wu, Chen, Zhang, Zheng, Peng, Zheng, Li, Hu, Zhu and Zheng. This is an open-access article distributed under the terms of the Creative Commons Attribution License (CC BY). The use, distribution or reproduction in other forums is permitted, provided the original author(s) and the copyright owner(s) are credited and that the original publication in this journal is cited, in accordance with accepted academic practice. No use, distribution or reproduction is permitted which does not comply with these terms.
*Correspondence: Xinying Jiang, amlhbmd4aW55aW5nQGNhZXAuY24=; Wanguo Zheng, d2d6aGVuZ19jYWVwQHNpbmEuY29t