- 1Key Laboratory of Photonic Control Technology (Tsinghua University), Ministry of Education, Beijing, China
- 2State Key Laboratory of Precision Measurement Technology and Instruments, Department of Precision Instrument, Tsinghua University, Beijing, China
We demonstrate an active mirror Q-switched laser with Nd:YAG/Nd:LuAG hybrid gain media, achieving a laser output of 1 J, 10 Hz, 8 ns. Using this hybrid oscillator as well as Nd:YAG and Nd:LuAG amplifiers, the difference in extraction efficiency of hybrid amplification was measured and analyzed, which is useful for high-energy hybrid amplification chains.
Introduction
The high-energy diode-pumped nanosecond laser is gaining attention because of its excellent performance. In a face-cooled multi-slab configuration based on cryogenic gas-cooled Yb:YAG disks, the DiPOLE100 system has first obtained an output energy of 105 J at 10 Hz [1]. Recently, the output level was raised to 150 J at 10 Hz [2]. Another efficient laser configuration is the active mirror (AM) because of its round-trip extraction structure. In 2013, Lucia achieved 14 J at 2 Hz laser output using a Yb:YAG AM [3]. In 2021, a 9.3 J, 33 Hz output was achieved using the Yb:YAG cryogenically-cooled active-mirror amplifier [4]. Our group obtained room-temperature 10 J level at 10 Hz using Nd:YAG and Nd:LuAG AMs [5, 6]. High-energy AM oscillator configuration has also been studied [7, 8], but few results have been reported for the Q-switched laser.
For laser systems operating at room temperature, one of the most commonly used gain media is Nd:YAG, but its relatively low saturation fluence limits its scaling performance to high energy level. Nd:LuAG has recently been demonstrated with excellent scaling performance [6, 9], as its saturation fluence of 1.93 J/cm2 is three times that of Nd:YAG [10]. Therefore, a hybrid amplification chain which uses Nd:YAG at low fluence and Nd:LuAG at high fluence is an effective method for high-energy lasers. However, the fluorescence spectrum of Nd:LuAG has a small red shift compared to Nd:YAG, so it is necessary to investigate the gain characteristics in detail in the case of spectral mismatch.
Theory
When the injected laser is not the center frequency of the gain, the ratio of the emission cross-section of the laser frequency and the center frequency needs to be known in order to perform the calculation of the output energy. The ratio is expressed as follows:
Then the saturation fluence can be expressed as follows:
where
where
Experimental Setup
The schematic diagram of the hybrid Nd:YAG/Nd:LuAG Q-switched laser oscillator is shown in Figure 1. The gain medium of the hybrid laser consisted of two pieces of Nd:YAG crystal slabs and two pieces of Nd:LuAG crystal slabs with the AM configuration. The Nd:YAG crystal slabs were 0.6 % doped, with dimensions of 30 × 20 × 8 mm3, while the Nd:LuAG crystal slabs were 0.8 % doped, with dimensions of 30 × 20 × 7 mm3. The front surface of each slab was anti-reflection (AR) coated at 1,064 nm and high-reflection (HR) coated at 808 nm relative to air, and the back surface was HR coated at 1,064 nm and AR coated at 808 nm relative to water. The laser at the incidence angle of 45° was reflected on the back surface and then passed through the gain medium for the second time. Cooling water flowed through the 1-mm-thick channel on the back of the slabs at a speed of 5 m/s to efficiently take away the heat.
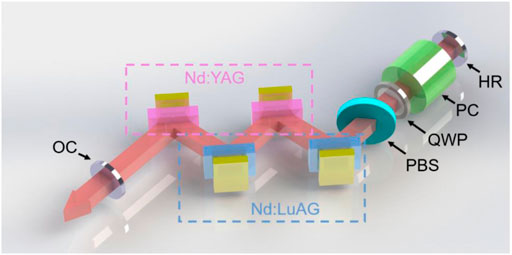
FIGURE 1. Schematic diagram of the Nd:YAG/Nd:LuAG hybrid Q-switched laser oscillator. OC, output coupler; HR, high reflective mirror; PBS, polarizing beam splitter; PC, Pockels cell; QWP, quarter-wave plate.
Each slab was pumped from the back surface by a laser diode (LD) array which consists of 30 laser diode bars and can provide a peak output power of 6.4 kW with an emitting area of 12.5 × 10 mm2. After being collimated using a microlens, each diode bar has the divergence angles of 8 and 5° along the slow and fast axes, respectively. Therefore, without optical coupling optics, a maximum peak intensity of 3.6 kW/cm2 can be obtained at the pump surface of the slabs. Each LD can be driven independently so that the oscillator can be operating in the hybrid mode with both types of gain media or in the pure mode with a single type.
A KD*P Pockels cell (PC), a polarizing beam splitter (PBS), and a quarter wave plate (QWP) were inserted in the cavity acting as the Q-switcher. A planar–planar cavity was used for the oscillator, and the output coupler (OC) with the transmittance of 60, 70, 80, and 90% was tested. The pump duration of LD was set as 280 μs at the repetition frequency of 10 Hz.
For the subsequent experiments on scaling efficiency, large-size Nd:YAG and Nd:LuAG AMs were used as amplifier modules, as shown in Figure 2. The seed laser output from the oscillator was expanded using a telescope and apodized using a serrated aperture (SA) with a diameter of 28 mm to ensure uniform amplification. Then, the laser double passed through three pieces of Nd:YAG AMs or Nd:LuAG AMs for investigation.
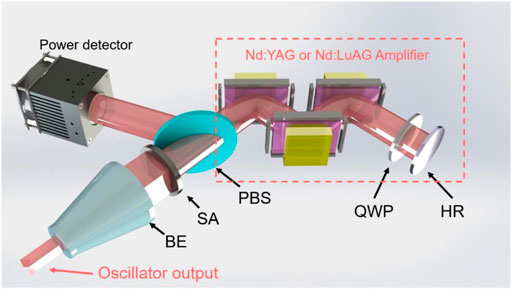
FIGURE 2. Schematic diagram of the measurement setup for investigating the amplification efficiency. BE, beam expander; SA, serrated aperture; PBS, polarizing beam splitter; QWP, quarter-wave plate; HR, high reflective mirror.
Results and Discussions
Spectra of Nd:YAG and Nd:LuAG
The measured fluorescence spectra and lasing spectra of Nd:YAG and Nd:LuAG are shown in Figure 3. The central wavelengths of Nd:YAG and Nd:LuAG were 1,064.42 nm and 1,064.60 nm, respectively, and both of them have the same laser bandwidth of 0.1 nm (FWHM) and the fluorescence bandwidth of 0.6 nm (FWHM). The fluorescence spectra of the two crystals overlap for most portions, which allows efficient scaling of hybrid master oscillator power amplifier (MOPA) structure combing two types of gain media. Furthermore, when the two crystals are inserted in one oscillator and operate together, their mixing gain remains as single-peaked as the output laser wavelength that was measured as 1,064.46 nm. Since Nd:YAG has a higher gain than Nd:LuAG, the spectrum of the hybrid output is more biased toward Nd:YAG.
The shape of the fluorescence spectrum is consistent with the shape of the emission cross-section of
where
Oscillator Output
The output characteristics were measured with three modes of Nd:YAG slabs pumped only, Nd:LuAG slabs pumped only, and four slabs fully pumped, with the results demonstrated in Figure 4A. In addition, the output characteristics under different transmittance (60, 70, 80, and 90%) were compared in Figure 4B.
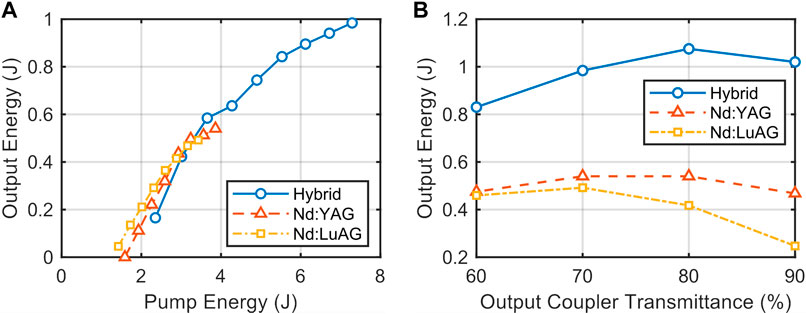
FIGURE 4. Output performance of the hybrid Nd:YAG/Nd:LuAG Q-switched laser oscillator. (A) Output energy versus the pump energy; (B) maximum output energy versus the output coupler transmittance.
With the optimum OC transmittance of 80%, a maximum output energy of 1.08 J was obtained at the pump energy of 7.3 J, corresponding to an optical-optical efficiency of 14.8%. Figure 5 shows the measured oscilloscope trace of the Q-switched pulse shape, with the pulse width of 8 ns. The spot size of the output beam is 10 × 11 mm2. When Nd:YAG and Nd:LuAG slabs were pumped separately, the maximum energy obtained was 540 and 490 mJ, respectively, at the optimum OC transmittance of 70%.
Comparison of Amplification Efficiencies
The laser output from the Q-switched oscillator was expanded using a telescope and then entered the amplifier stage of Nd:YAG or Nd:LuAG. Figure 6 compares the gain of Nd:YAG amplifier with different seed lasers. By fitting the gain data, k1 and k2 were obtained as 0.85 and 0.91, respectively. We claim that the values fitted, which are larger than those obtained by fluorescence spectra as presented in Section 4.1, are considered to be closer to the actual values. The measured fluorescence spectrum of the gain medium, which was used to infer the emission cross-section in Section 4.1, was actually narrower than the real fluorescence spectrum due to a gain narrowing effect that the fluorescence near the center of the gain gets amplified more effectively, leading to the underestimate of k1 and k2 in Section 4.1.
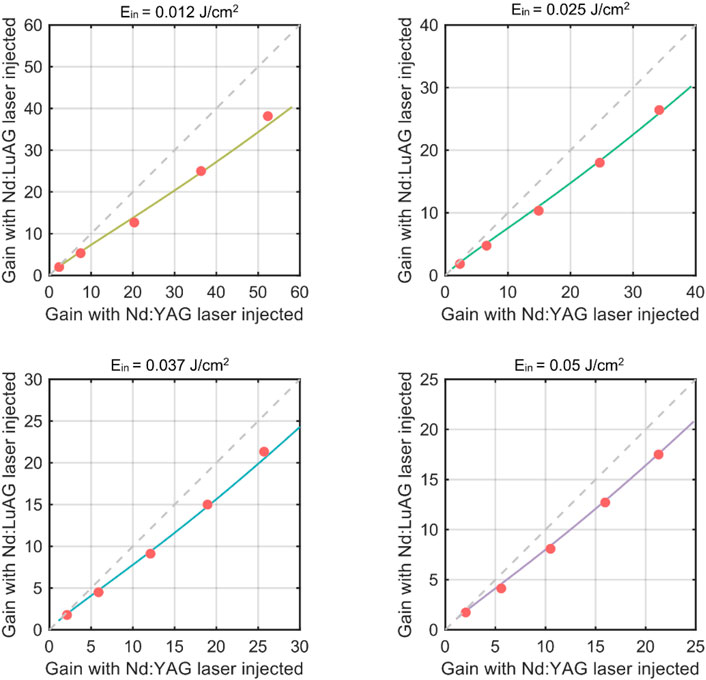
FIGURE 6. Gain of the Nd:YAG amplifier with different laser injected. solid line, fitted with k1 = 0.85; circle, measured; dashed line, reference line with a slope of 1.
Figure 7 shows the ratio of the gain of the Nd:YAG amplifier with Nd:YAG and Nd:LuAG seed injected, versus injected laser fluence at the same pump energy. When the incident fluence increased, the extraction efficiency increased, and the ratio of the gain increased. Therefore, to reduce the loss due to spectral mismatch, a large incident fluence is required to keep the amplifier operating at a heavily saturated condition.
Conclusion
In this study, we reported a Q-switched oscillator with a hybrid gain media of Nd:YAG and Nd:LuAG, producing a laser output of 1.08 J, 10 Hz, 8 ns. Furthermore, the ratios of the emission cross-section for two types of gain medium were obtained via a hybrid scaling experiment, which can be used to calculate the amplified output energy in the case of spectral mismatch. The results indicate that the overlapping gain spectra of Nd:YAG and Nd:LuAG makes it possible to obtain high gain even when the spectra are mismatched.
Data Availability Statement
The raw data supporting the conclusion of this article will be made available by the authors, without undue reservation.
Author Contributions
XL performed the experiments and made the data analysis. XL, XF, and QL contributed to writing and editing the manuscript.
Funding
Supported by the Open-Foundation of Key Laboratory of Laser Device Technology, China North Industries Group Corporation Limited (No. KLLDT202101).
Conflict of Interest
The authors declare that the research was conducted in the absence of any commercial or financial relationships that could be construed as a potential conflict of interest.
Publisher’s Note
All claims expressed in this article are solely those of the authors and do not necessarily represent those of their affiliated organizations, or those of the publisher, the editors, and the reviewers. Any product that may be evaluated in this article, or claim that may be made by its manufacturer, is not guaranteed or endorsed by the publisher.
References
1. Mason P, Divoký M, Ertel K, Pilař J, Butcher T, Hanuš M, et al. Kilowatt Average Power 100 J-Level Diode Pumped Solid State Laser. Optica (2017) 4:438–9. doi:10.1364/optica.4.000438
2. Martin D, Jan P, Martin H, Navrátil P, Denk O, Severová P, et al. 150 J DPSSL Operating at 1.5 kW Level. Opt Lett (2021) 46:5771–3. doi:10.1364/OL.444902
3. Gonçalvès-Novo T, Albach D, Vincent B, Arzakantsyan M, Chanteloup J-C. 14 J/2 Hz Yb3+:YAG Diode Pumped Solid State Laser Chain. Opt Express (2013) 21:855–66. doi:10.1364/OE.21.000855
4. Ogino J, Tokita S, Kitajima S, Yoshida H, Li Z, Motokoshi S, et al. 10 J Operation of a Conductive-Cooled Yb:YAG Active-Mirror Amplifier and Prospects for 100 Hz Operation. Opt Lett (2021) 46:621–4. doi:10.1364/ol.414926
5. Liu T, Sui Z, Chen L, Li Z, Liu Q, Gong M, et al. 12 J, 10 Hz Diode-Pumped Nd:YAG Distributed Active Mirror Amplifier Chain with ASE Suppression. Opt Express (2017) 25:21981–92. doi:10.1364/oe.25.021981
6. Liu T, Feng T, Sui Z, Liu Q, Gong M, Zhang L, et al. 50 Mm-Aperture Nd:LuAG Ceramic Nanosecond Laser Amplifier Producing 10 J at 10 Hz. Opt Express (2019) 27:15595–603. doi:10.1364/oe.27.015595
7. Fu X, Liu T, Deng X, Li P, Liu Q, Yan X, et al. Ultrahigh-efficiency 4-J, 10-Hz, Nd:YAG Quasi-Continuous-Wave Active Mirror Oscillator. Appl Phys B (2015) 121:453–7. doi:10.1007/s00340-015-6252-4
8. Ding J, Wang J, Yu G, Zhou J, Zhu X, Chen W, et al. Theoretical and Experimental Study of Diode-Pumped Nd:LuAG Disk Lasers. In: Proc. SPIE 11849, Fourth International Symposium on High Power Laser Science and Engineering (HPLSE 2021) (2021). p. 1184910. doi:10.1117/12.2599034
9. Liu Q, Gong M, Liu T, Sui Z, Fu X. Efficient Sub-joule Energy Extraction from a Diode-Pumped Nd:LuAG Amplifier Seeded by a Nd:YAG Laser. Opt Lett (2016) 41:5322–5. doi:10.1364/ol.41.005322
10. Wang X-D, Xu X-D, Zang T-C, Ma C-L, Zhao Z-W, Xu J. Growth and Spectral Properties of Nd:Lu3Al5O12 Crystal. J Inorg Mater (2010) 25(4):435–40. doi:10.3724/sp.j.1077.2010.00435
11. Frantz LM, Nodvik JS. Theory of Pulse Propagation in a Laser Amplifier. J Appl Phys (1963) 34(8):2346–9. doi:10.1063/1.1702744
Keywords: hybrid gain medium, nanosecond laser, active mirror, Nd:LuAG, spectral mismatch
Citation: Lei X, Fu X and Liu Q (2022) Hybrid Nd:YAG/Nd:LuAG Nanosecond Laser Oscillator and Amplifier. Front. Phys. 10:922651. doi: 10.3389/fphy.2022.922651
Received: 18 April 2022; Accepted: 09 May 2022;
Published: 16 June 2022.
Edited by:
Ivan Divliansky, University of Central Florida, United StatesReviewed by:
Kang Zhijun, Aerospace Information Research Institute (CAS), ChinaQi Wang, China South Industrial Academy, China
Copyright © 2022 Lei, Fu and Liu. This is an open-access article distributed under the terms of the Creative Commons Attribution License (CC BY). The use, distribution or reproduction in other forums is permitted, provided the original author(s) and the copyright owner(s) are credited and that the original publication in this journal is cited, in accordance with accepted academic practice. No use, distribution or reproduction is permitted which does not comply with these terms.
*Correspondence: Xing Fu, fuxing@tsinghua.edu.cn; Qiang Liu, qiangliu@tsinghua.edu.cn