- 1School of Optics and Photonics, Beijing Institute of Technology, Beijing, China
- 2Key Laboratory of Photoelectronic Imaging Technology and System, Ministry of Education, Beijing, China
- 3Key Laboratory of Photonics Information Technology, Ministry of Industry and Information Technology, Beijing, China
Tunable 2∼3-μm femtosecond lasers are of high interest in various applications, such as medical diagnostics and molecular spectroscopy. Cr:ZnSe/ZnS is extremely suited for broadband tunable femtosecond lasers due to its excellent emission bands. In this article, we demonstrate a wavelength-tunable Kerr-lens mode-locked Cr:ZnS laser by utilizing a birefringent filter. The group delay dispersion of the operation and the thickness of the birefringent are finely optimized. With the rotation of the birefringent filter, the scheme offers a tuning bandwidth of over 300 nm from 2,220 nm to 2,520 nm. To the best of our knowledge, it is the broadest tuning range among the reported femtosecond Cr:ZnS lasers.
Introduction
Broadband mid-infrared (MIR) laser sources are in great demand for a plethora of applications, including biological identification, medical diagnostics, and molecular spectroscopy, due to the presence of strong fundamental and overtone vibrational absorption lines of different gases [1, 2]. To be more specific, the range between 2 and 3 μm covers the absorption lines of NH2, CO, CO2, and water related to typical biological tissue absorption [3]. Femtosecond laser sources covering 2–3 μm could be used for time-resolved spectroscopy of such molecules [4]. Besides, such sources can be also used for remote sensing and driving various nonlinear processes, such as MIR supercontinuum generation [5] and high-harmonic generation [6]. Compared to untunable broadband MIR sources, wavelength-tunable femtosecond lasers are more attractive due to the better matching of their wavelength range with detected molecules or various nonlinear processes.
Cr:ZnSe/ZnS is an ideal material for generating tunable femtosecond mode-locked lasers in 2–3 μm. Cr:ZnSe/ZnS with ultrabroad emission bands provides the accessibility of broadband femtosecond lasers and a large range of tunability. The broad absorption bands of the gain media cover some sufficient and reliable pump sources, such as commercial fiber lasers or laser diodes. Furthermore, Cr:ZnSe/ZnS provides no excited state absorption, provides room temperature operation, and is close to a four-level energy structure. Hence, with their favorable physical characteristics, these materials have been considered as “Ti:sapphire” in the MIR [7].
Femtosecond oscillators are commonly achieved by Kerr-lens passively mode-locked schemes or saturable absorber passively mode-locked schemes based on semiconductors or graphenes [8–11]. The first mode-locked Cr:ZnSe/ZnS laser was based on a semiconductor saturable absorber mirror (SESAM) reported in 2005 [12]. Several advantages, such as enabling self-starting and low-noises, offered by SESAM mode-locking schemes are appealing. However, the bandwidth of the SESAM restricts the realization of few-cycle pulses and the range of tunability. Compared to saturable absorber passive mode-locking, Kerr-lens mode-locked oscillators break through these restrictions and become more attractive to fulfill broadband femtosecond lasers with a large tunable bandwidth.
Cr:ZnSe/ZnS has been demonstrated as an excellent material for tunable continuous wave laser, which could be tuned from 1880 to 3,350 nm [13, 14]. Meanwhile, some tunable femtosecond oscillators based on Cr:ZnSe/ZnS are reported, in which a birefringent filter (BRF) or a pair of prisms is used [15–17]. Although the first tunable femtosecond Cr:ZnSe laser is achieved by adjusting a slit working with a prism pair [16], the space chirp induced by the prism pair has a severe negative influence on beam quality. In Ref. [17], the tunable range is expended to 180 nm achieved by the BRF. Until now, the broadest tunable range in femtosecond Cr:ZnSe/ZnS laser has been from 2,120 nm to 2,408 nm [15]. All these works only offer pulses with the wavelength below 2,400 nm and tunable bandwidth below 300 nm.
In this letter, a widely tunable femtosecond Cr:ZnS oscillator is reported. The KML Cr:ZnS laser operating at 2347 nm allows the generation of stable126-fs pulses with an average output power of 344 mW at a repetition rate of 72.6 MHz. A BRF offers less loss, a broadband tunable range, and better beam quality. By utilizing a 0.5-mm-thick BRF with a broad free spectral range, we achieved more than 300 nm tuning bandwidth from 2,220 to 2,520 nm. Large negative dispersion compensation was set for ideal soliton mode-locking operation and a broad tunable bandwidth. To the best of our knowledge, it is the broadest tuning range in femtosecond Cr:ZnSe/ZnS oscillators and fills the gap in the long-wave range beyond 2,400 nm. This tunable wavelength range not only contains the absorption lines of NH2 and CO (below 2,400 nm) but also contains the absorption lines of HF and COS (beyond 2,400 nm) [18]. This work has unique potential applications in molecular spectroscopy and nonlinear processes.
Experimental Setup
The experimental setup is depicted in Figure 1. The 2 × 2 × 9-mm3 polycrystalline Cr:ZnS was used as the gain medium of the oscillator, which is AR-coated at both 1908 nm and 2,100–2,600 nm, with 10% low-signal transmission ratios at a wavelength of 1908 nm. The gain element is mounted in a copper heat sink, and its temperature is maintained by thermal electric coolers (TECs) to 20°C. A 1908-nm linear polarized Tm-doped fiber laser system with an output power of 5.4 W is used to pump the Cr:ZnS. The Cr:ZnS is placed in the middle of the first stability zone of an X-folded cavity. The two dichroic mirrors (DMs) are coated with high reflection over 2.1–2.7 μm and high transmission around 1.91 μm with a radius of curvature (ROC) of −100 mm. All the plane mirrors are high reflection coated from 2,100 to 2,700 nm. The output coupler (OC) is a plane mirror based on 6-mm-thick fused-silica (IR) with a transmission of 10% from 1700 to 2,700 nm. A hard aperture arranged close to the end mirror was used to stabilize Kerr-lens mode-locking operation. The whole cavity length is 2066 mm, corresponding to a pulse repetition rate of 72.6 MHz.
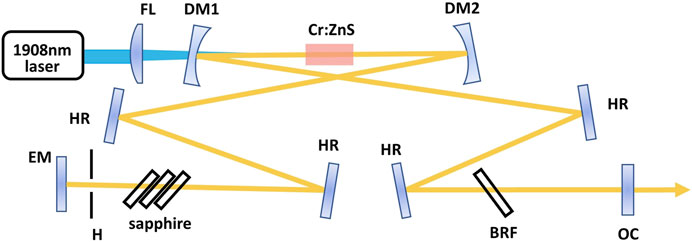
FIGURE 1. Kerr-lens mode-locked Cr:ZnS oscillator. FL, focusing lens (f = 100 mm); DM, curved dichroic mirror (ROC = 100 mm); EM, end mirror (the same as HR, high reflection, at 2,150–2,700 nm); OC, output coupler, T = 10% at 1,700–2,700 nm; H, hard aperture; BRF, birefringent filter.
In order to optimize the group delay dispersion (GDD) in the cavity, three 5-mm-thick sapphires were placed near the end of the short arm at Brewster’s angle (59.95°), providing −4,250 fs2 negative GDD at 2,400 nm. The 9-mm-thick Cr:ZnS leads to 1,080 fs2 positive GDD at 2,400 nm. The round-trip net GDD curve of the asymmetric cavity shown in Figure 2 indicates that the anomalous dispersion condition is provided from 2,000 to 2,700 nm. A MgF2 BRF inserted in the cavity at Brewster’s angle (53.77°) allowed a tunable output center wavelength from 2,220 nm to 2,520 nm under anomalous dispersion conditions in the soliton KLM regime. The thickness of the BRF was selected as 0.5 mm, corresponding to the transmission range (FWHM, full width at half maximum) of 740 nm for a central wavelength of 2.4 μm.
Results and Discussion
The resonator was at first adjusted for maximizing the CW output power. Then fine alignment of the position of DM2 and the EM is needed to realize Kerr-lens mode-locking operation, which was initiated by shaking the EM. The operation mode of the oscillator varied with pump power. It is under CW operation at low pump power (3 W), transferred to Q-switched ML when further increasing pump power, and converted to continuous wave mode-locking (CWML) with sufficient pump power (over 4.26 W). The output power versus incident pump power under varied operations is shown in Figure 3 when the center wavelength is at 2,347 nm.
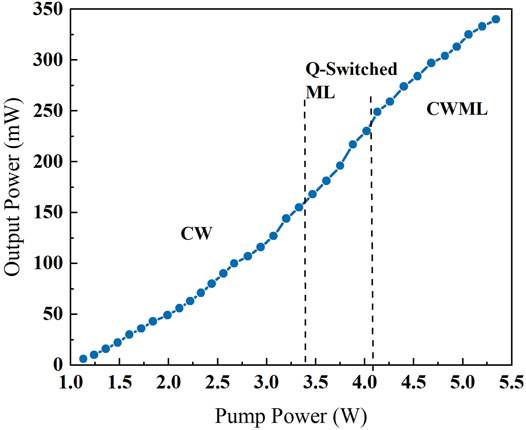
FIGURE 3. Measured output power and operation mode versus incident pump power at 2,347 nm center wavelength. CW, continuous wave; Q-switched ML, Q-switched mode-locking; CWML, continuous wave mode-locking.
The laser routinely produced pulses of 126 fs, whose center wavelength is 2,347 nm, at the highest pump power of 5.4 W. The average output power is 344 mW, corresponding to a pulse energy of 4.7 nJ with a peak power of 38 kW. The optical spectrum is measured by an APE waveScan spectrometer. As shown in Figure 4A, the sidebands distributed at both sides of the center wavelength are called Kelly sidebands. Kelly sidebands are often observed at soliton mode-locked schemes with large abnormal dispersion. The sidebands are induced by dispersion and nonlinearities when pulses produce energy beyond the restriction of soliton area [19, 20]. The center wavelength of the left sideband is at around 2,236 nm, and the right one is at around 2,459 nm with a similar frequency shift. As shown in Figure 4B, the interferometric autocorrelation trace is measured with an APE. pulseCheck. By assuming a sech2-pulse shape, a spectral bandwidth of 48 nm (FWHM) and a pulse duration of 126 fs demonstrated a time-bandwidth product of 0.328, near the Fourier transform limitation of 0.315. Figure 5 shows the radio frequency spectrum of the fundamental frequency at 72.6 MHz, measured by a frequency spectrum analyzer with a resolution bandwidth of 1 kHz. The mode-locking stability is confirmed by the clear single peak with a high extinction down to 68 dBc without any side lobes at fundamental cavity repetition.
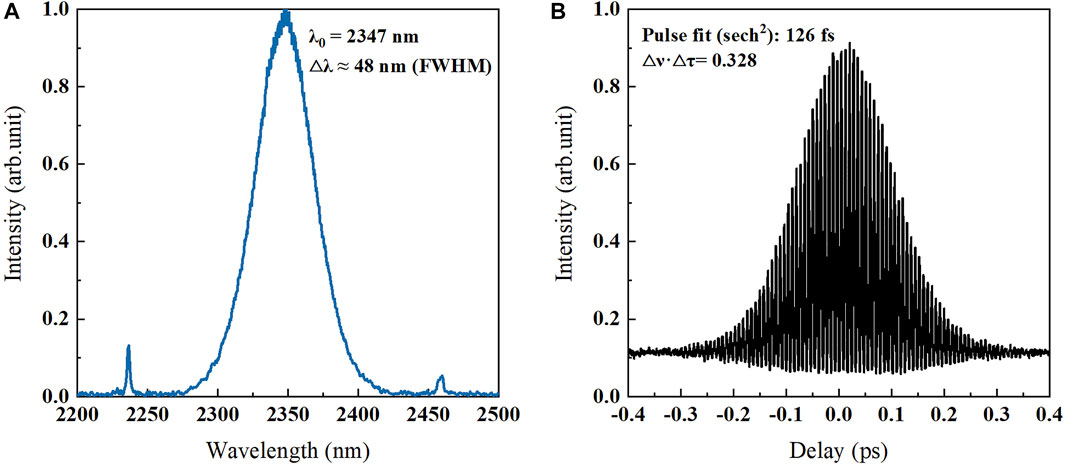
FIGURE 4. Measured spectrum and autocorrelation trace at 2,347 nm center wavelength. (A) Optical spectrum. (B) Interferometric autocorrelation trace.
By rotation of the 0.5-mm-thick MgF2 BRF, the central wavelength of the mode-locked Cr:ZnS laser at a pump power of 5.4 W shifts from 2,220 nm to 2,520 nm, as shown in Figure 6A. The tuning range is over 300 nm, and to the best of our knowledge, it is the broadest one in Cr:ZnS/ZnSe mode-locked lasers ever reported. In a similar situation, the CW laser could be tuned from 2,155 nm to 2,623 nm by rotating the BRF, equal to a 500 nm tuning range. The tuning limit of the short wavelength is affected by HR, whose high reflection range is from 2,150 nm to 2,700 nm. At the central wavelength below 2,220 nm, the stable pulses are not accessible. Besides, the experimental setup was operating in the air, so the long-wave tuning range was restricted by absorption lines of vapor (Figure 6A with dash lines). The output power of the CW laser reduced sharply, induced by strong absorption of vapor (depicted in Figure 6B with a gray line). In our experiment, some absorption peaks also appeared at the spectrum curve of 2,520 nm in CWML operation, and mode-locked pulses disappeared when further rotating the BRF to a longer wavelength. Therefore, the tuning range can be expanded if we use mirrors with a broader coating range or operate the scheme in vacuum to avoid strong absorption of vapor.
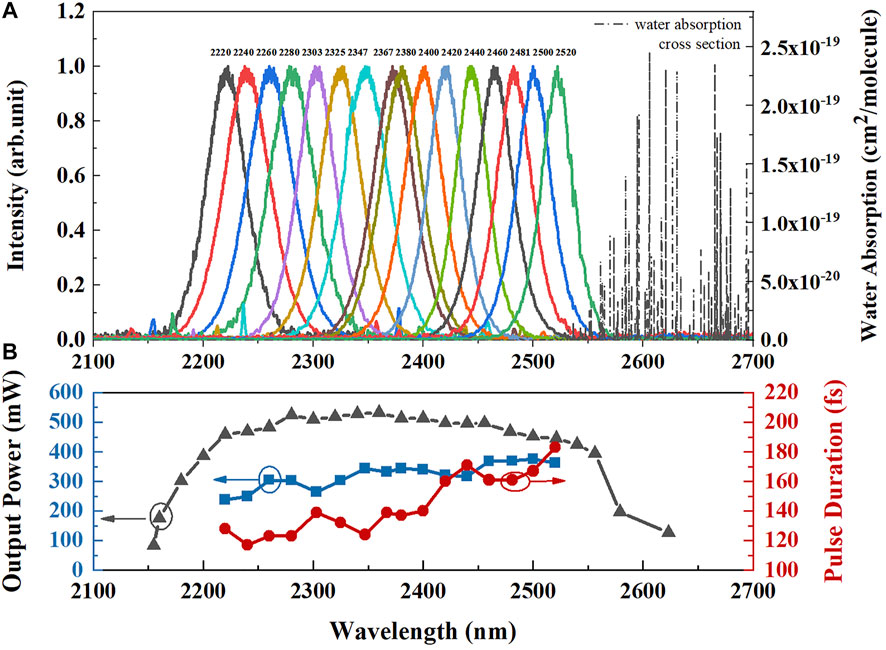
FIGURE 6. Measured details with the tuning of the central wavelength of KLM laser. (A) Various mode-locked optical spectrums at different tuning wavelengths (solid lines) and water absorption lines (dash lines). (B) CW output power (gray triangle), CWML output power (blue square), and pulse duration (red circle) versus central wavelength.
The various average output powers of CWML operation and duration times of pulses are depicted in Figure 6B with blue and red lines. The widening duration of the pulse is attributed to the increasing abnormal dispersion at a long wavelength range (Figure 2).
Conclusion
In conclusion, we demonstrated a high-performance broadly tunable femtosecond KML Cr:ZnS laser over a 300-nm tuning range. We use three 5-mm-thick sapphires to adjust the GDD of the resonator to realize stable soliton mode-locking in a wide spectral range. Kerr-lens mode-locked operation without the extra restriction of bandwidth allows a short pulse duration (126 fs, 2,347 nm) and a wide tuning range from 2,220 nm to 2,520 nm. We believe a broader tuning range could be achieved in a vapor-free atmosphere. To the best of our knowledge, this operation offered the widest tunable bandwidth among the reported femtosecond Cr:ZnSe/ZnS lasers. It is the first time that the tunable central wavelength is extended beyond 2.5 μm. This wavelength-tunable femtosecond laser source may provide potential applications in time-resolved molecular spectroscopy and MIR generation.
Data Availability Statement
The original contributions presented in the study are included in the article/Supplementary Material, further inquiries can be directed to the corresponding author.
Author Contributions
QW designed and supervised the research project. RW contributed to the specific experiment and the first draft of the manuscript. FY and YL measured the experimental data. All authors contributed to manuscript revision and approved the submitted version.
Funding
This work was supported by the Beijing Natural Science Foundation (No. 4222074).
Conflict of Interest
The authors declare that the research was conducted in the absence of any commercial or financial relationships that could be construed as a potential conflict of interest.
Publisher’s Note
All claims expressed in this article are solely those of the authors and do not necessarily represent those of their affiliated organizations, or those of the publisher, the editors, and the reviewers. Any product that may be evaluated in this article, or claim that may be made by its manufacturer, is not guaranteed or endorsed by the publisher.
References
1. Fried NM. Recent Advances in Infrared Laser Lithotripsy [Invited]. Biomed. Opt. Express (2018) 9:4552. doi:10.1364/BOE.9.004552
2. Scholle K, Lamrini S, Koopmann P, Fuhrberg P. 2 Μm Laser Sources and Their Possible Applications. In: Frontiers in Guided Wave Optics and Optoelectronics. London: IntechOpen (2010). doi:10.5772/39538
3. Sorokina IT. Broadband Mid-infrared Solid-State Lasers. In: M Ebrahim-Zadeh, and IT Sorokina, editors. Mid-Infrared Coherent Sources and Applications (2008). p. 225–60. doi:10.1007/978-1-4020-6463-0_7
4. Pupeza I, Huber M, Trubetskov M, Schweinberger W, Hussain SA, Hofer C, et al. Field-resolved Infrared Spectroscopy of Biological Systems. Nature (2020) 577:52–9. doi:10.1038/s41586-019-1850-7
5. Grassani D, Tagkoudi E, Guo H, Herkommer C, Yang F, Kippenberg TJ, et al. Mid Infrared Gas Spectroscopy Using Efficient Fiber Laser Driven Photonic Chip-Based Supercontinuum. Nat Commun (2019) 10:1553. doi:10.1038/s41467-019-09590-3
6. Leshchenko VE, Talbert BK, Lai YH, Li S, Tang Y, Hageman SJ, et al. High-power Few-Cycle Cr:ZnSe Mid-infrared Source for Attosecond Soft X-Ray Physics. OPTICA (2020) 7:981–8. doi:10.1364/OPTICA.393377
7. Sorokin E, Naumov S, Sorokina IT. Ultrabroadband Infrared Solid-State Lasers. IEEE J. Sel. Top Quantum Electron. (2005) 11:690–712. doi:10.1109/JSTQE.2003.850255
8. Nagl N, Grobmeyer S, Potzlberger M, Pervak V, Krausz F, Mak KF (2020). Directly Diode-Pumped Few-Optical-Cycle Cr:ZnS Laser at 800 mW of Average Power. In: Conference on Lasers and Electro-Optics OSA Technical Digest. Washington, DC: OSA. doi:10.1364/CLEO_SI.2020.SF3H.5
9. Wang Y, Fernandez TT, Coluccelli N, Gambetta A, Laporta P, Galzerano G. 47-fs Kerr-Lens Mode-Locked Cr:ZnSe Laser with High Spectral Purity. Opt. Express (2017) 25:25193. doi:10.1364/OE.25.025193
10. Cizmeciyan MN, Kim JW, Bae S, Hong BH, Rotermund F, Sennaroglu A. Graphene Mode-Locked Femtosecond Cr:ZnSe Laser at 2500 Nm. Opt. Lett. (2013) 38:341. doi:10.1364/ol.38.000341
11. Barh A, Heidrich J, Alaydin BO, Gaulke M, Golling M, Phillips CR, et al. Watt-level and Sub-100-fs Self-Starting Mode-Locked 24-µm Cr:ZnS Oscillator Enabled by GaSb-SESAMs. Opt. Express (2021) 29:5934. doi:10.1364/oe.416894
12. Pollock CR, Brilliant NA, Gwin D, Carrig TJ, Alford WJ, Heroux JB, et al. Mode Locked and Q-Switched Cr:ZnSe Laser Using a Semiconductor Saturable Absorbing Mirror (SESAM). In: Advanced Solid-State Photonics. Washington, D.C: OSA. TuA6. doi:10.1364/ASSP.2005.TuA6
13. Demirbas U, Sennaroglu A. Intracavity-pumped Cr2+:ZnSe Laser with Ultrabroad Tuning Range between 1880 and 3100 Nm. Opt. Lett. (2006) 31:2293. doi:10.1364/OL.31.002293
14. Sorokin E, Sorokina IT, Mirov MS, Fedorov VV, Moskalev IS, Mirov SB. Ultrabroad Continuous-Wave Tuning of Ceramic Cr:ZnSe and Cr:ZnS Lasers. In: Lasers, Sources and Related Photonic Devices. (Washington, D.C: OSA) (2010). doi:10.1364/ASSP.2010.AMC2
15. Cho WB, Choi SY, Zhu C, Kim MH, Kim JW, Kim JS, et al. Graphene Mode-Locked Femtosecond Cr^2+:ZnS Laser with ∼300 Nm Tuning Range. Opt. Express (2016) 24:20774. doi:10.1364/oe.24.020774
16. Ma J, Xie G, Lv P, Gao W, Yuan P, Qian L, et al. Wavelength-Versatile Graphene-Gold Film Saturable Absorber Mirror for Ultra-broadband Mode-Locking of Bulk Lasers. Sci Rep (2014) 4. doi:10.1038/srep05016
17. Mirov SB, Fedorov VV, Martyshkin D, Moskalev IS, Mirov M, Vasilyev S. Progress in Mid-IR Lasers Based on Cr and Fe-Doped II-VI Chalcogenides. IEEE J. Sel. Top Quantum Electron. (2015) 21:292–310. doi:10.1109/JSTQE.2014.2346512
18. Sorokina IT, Sorokin E. Femtosecond Cr2+-Based Lasers. IEEE J. Sel. Top Quantum Electron. (2015) 21:273–91. doi:10.1109/JSTQE.2014.2341589
19. Smith NJ, Blow KJ, Andonovic I. Sideband Generation through Perturbations to the Average Soliton Model. J. Light Technol. (1992) 10:1329–33. doi:10.1109/50.166771
Keywords: Kerr-lens mode-locking, mid-infrared, femtosecond laser, tunability, Cr:ZnS
Citation: Wang Q, Wang R, Yang F and Li Y (2022) Wavelength-Tunable Kerr-Lens Mode-Locked Femtosecond Cr:ZnS Laser With a ∼300-nm Tuning Range From 2.2 to 2.5 μm. Front. Phys. 10:912196. doi: 10.3389/fphy.2022.912196
Received: 04 April 2022; Accepted: 19 April 2022;
Published: 20 May 2022.
Edited by:
Xing Fu, Tsinghua University, ChinaCopyright © 2022 Wang, Wang, Yang and Li. This is an open-access article distributed under the terms of the Creative Commons Attribution License (CC BY). The use, distribution or reproduction in other forums is permitted, provided the original author(s) and the copyright owner(s) are credited and that the original publication in this journal is cited, in accordance with accepted academic practice. No use, distribution or reproduction is permitted which does not comply with these terms.
*Correspondence: Qing Wang, qingwang@bit.edu.cn