- 1School of Physical Science and Technology, ShanghaiTech University, Shanghai, China
- 2Shanghai High Repetition Rate XFEL and Extreme Light Facility, Shanghai, China
- 3Shanghai Advanced Research Institute, Chinese Academy of Sciences, Shanghai, China
The compound refractive lens (CRL) is a commonly used X-ray optical component for photon beam conditioning and focusing on the beamlines of the X-ray facilities. The normal preparation materials are beryllium, aluminum, silicon of current lenses, and they all suffered from high heat load fatigue and short pulse damage risks. Hard materials based CRL is engaged attention for the advanced X-ray application. Sapphire crystal has the advantages of high density, high melting point, low thermal expansion coefficient. In this paper, properties of the refraction and absorption ratio of Sapphire and parameters of Sapphire lenses of effective aperture, transmittance, resolution, number of lenses needed for a certain focus, are taken into account for the CRL design, comparing with those of several common materials as well. The calculation results show that the performance of the sapphire lens is better than that of the aluminum lens and silicon lens, and inferior to that of the beryllium lens and diamond lens, but the number of lenses used is less. In the meantime, performances of sapphire lenses focusing are simulated and thermal effects on lenses are analyzed. Analysis and discussion are carried out under the same conditions as the metal Aluminum ones. The focusing simulation shows that the sapphire lenses can obtain a smaller spot with more intensity. The thermal analysis indicates that the temperature during use of the sapphire lens is much lower than the melting point of sapphire, and the thermal deformation is negligible.
Introduction
With the development of the forth-generation synchrotron radiation sources and X-ray free electron lasers facilities, beamlines with high energy, high intensity, low divergence have been produced, and X-ray analysis technology has been more widely used in material structure and material dynamics, biophysics and protein crystallography, geophysics and environmental science and the research in other subject areas [1]. With the deepening of these researches, the requirements for X-ray resolution are getting higher and higher. At the same time, higher standards are put forward for X-ray optical devices, and X-ray nano-focusing devices have also caused more and more focus.
Since the refractive index of material for X-rays is less than 1, the focusing of X-rays could only be done employing reflection and diffraction. Until 1996, researchers such as Snigirev demonstrated focusing capabilities of the refractive optics, so called compound refractive lens (CRL) [2]. They machined 30 circular holes with a diameter of 600 μm on an aluminum plate, focused X-rays with an energy of 14 keV at 1.8 m, and obtained a spot of 8 μm. Compared with other X-ray optics, compound refractive lenses have many advantages. CRL has low requirements on the surface roughness of the lens, does not change the direction of the optical path, can work at high temperature, and is easy to align. Therefore, CRL has developed rapidly. Therefore, it has been developed rapidly. At present, compound refractive lenses include cylindrical, parabolic [3], planar [4], saw-tooth [5], spherical [6], etc. Among them, the parabolic lens is the most widely used due to its small aberration.
At present, the most commonly used X-ray compound refractive lenses are made from elements of beryllium (Be) [7], diamond (C) [8], aluminum (Al) [2], silicon (Si) [9]. Be is the earliest applied lens material and is widely used in wide-spectrum X-rays ranging from 3 to 60 keV. However, Be is highly toxic. And after processing and manufacturing, the single crystal Be will be transformed into polycrystalline, and the single crystal Be lens cannot be obtained. Imperfections of Be leads to the increase of small-angle scattering, which is easy to produce spots, and spoil the focusing. The resolution of Be lens is only about 100 nm [10]. In addition, the thermal expansion coefficient of Be is relatively large, and the long-term high thermal load operation makes the Be lens prone to recrystallization behavior, resulting in fatigue damage and thermal deformation [11].
Diamond has the advantages of high density, low X-ray absorption, low thermal expansion coefficient, high thermal conductivity coefficient, and high thermal damage threshold [12]. Therefore, diamond has been the material of choice for several crucial high-heat component applications in X-ray optics, including double-crystal monochromators [13] and high-reflectivity Bragg mirrors [14]. Using single crystal diamond to prepare a composite refractive lens can improve the uniformity of electron density, small angle scattering signal, less speckle, and more conducive to focusing. A resolution of about 40 nm can be obtained [15]. However, the hardness of the diamond is relatively high, and the processing is complex. At present, diamond composite refractive lenses can be manufactured by ion beam lithography [15] and laser pulse technology [8] can be used to manufacture diamond composite refractive lenses at present. The surface roughness of the processed diamond lens can currently reach about 20 nm [16].
Another popular material for compound refractive lenses are polymer materials, such as polymethyl methacrylate (PMMA). Lenses are often prepared in combination with 3D printing technology [17]. A resolution of around 100 nm can be obtained [18]. It has many advantages. However, the lens made of this material has low thermal conductivity, low melting point, strong small-angle scattering signal, and poor resistance to thermal damage and radiation.
Alumina is a compound material of Aluminum Oxide (Al2O3) with high hardness, high density, high temperature resistance and low thermal expansion coefficient. In the past, alumina was not used for compound refractive lenses due to the limitation of processing techniques, since that it was difficult to manufacture a lens with sub-nanometer surface roughness and sub-microradian shape errors of hard materials. But at present, advanced processing techniques, such as ultrafast laser ablation, offers a higher level of surface quality (that is, low surface roughness values). Alumina has a variety of structures, commonly alpha-alumina (α-alumina) and gamma-alumina. Among them, α-alumina has the densest structure and the highest density, and at high temperature, all crystalline aluminas will be converted into α-alumina. Sapphire is α-alumina, doped with a small amount of titanium and iron, has been increasingly used in optical components for high heat load optics of light sources like powerful lasers, bright synchrotron radiation, etc.
In this article, we analyze the optical parameters, machinability, uniformity, etc. of sapphire material for X-ray compound refractive lenses, and the focusing simulation of the sapphire X-ray compound refractive lenses as designed is carried out compared to the ones made of metal Aluminum. As an addition, we also considered the thermal stability of the sapphire lenses proposed to be used for high heat load X-ray source as free electron lasers as well.
Material Selection Consideration
The refractive index of X-rays in the material is:
where
The focal length of the lens is:
where R is the radius of curvature at the vertex of the lens (Figure 2), and N is the number of lenses. The number of lenses used can be determined after determining the required focal length, working energy, and materials used. Calculate the number of lenses as are necessary for several common materials within 5–100 keV. The radius of curvature is set to 50 μm. The focal length is set to 10m, and the calculation results are shown in Figure 3 (The result is not rounded). Compared with other materials, under the condition of achieving the same focusing effect, the number of sapphire lenses used is the least, even half the number of lenses required by other materials. Then, in the process of actual engineering use, can reduce the cost of the lenses. Certainly that, it can reduce alignment errors caused by the many-groups lenses stacks.
Considerations of sapphire lenses will be discussed from the following aspects.
i) Consideration of the effective aperture of the sapphire lens. Due to the absorption and scattering effects of X-rays in materials, only part of the photons of X-rays can pass through the lens. Assuming that the intensity of the transmitted X-rays is evenly distributed on a circle, the diameter of the circle is the effective diameter of the lens. The effective aperture of the lenses is:
where
ii) Consideration of the transmittance of the sapphire lens during use, it is the ratio of the total light intensity passing through the lens to the full light intensity that the lens can receive. The transmittance of the lens is:
It can be clearly seen from the formula that the transmittance. It is related to the choice of material and the distance between the two vertices of the lens. The transmittance of several common materials in the range of 5–100 keV is calculated. The distance between the two paraboloids is set to 50 μm. Other settings are the same as Figure 3 and Figure 4. The calculation results are shown in Figure 5. In the range of low energy, the transmittance of sapphire is higher, even better than that of the diamond; in the middle energy range, the transmittance of sapphire is lower than that of diamond, etc. In the high energy range, there is little difference between several materials.
iii) Consideration of the resolution of the sapphire lens. Resolution is divided into horizontal resolution and vertical resolution, which refers to the distance between the smallest two points that can be distinguished through the lens. That is to say, when the distance between two points is smaller than the resolution, it cannot be discerned. The resolution of the lens is
Consideration of the uniformity of the lens. The uniformity of the material will affect the wavefront of the X-ray, which will affect the refraction and focusing effect of the lens. The better the uniformity of the material, the better the refraction and focusing effect of the lens. Density variations, stacking faults, dislocations, inclusions, and voids affect the uniformity of the material. These cause intensity variations across the beam cross-section, halos from small-angle X-ray scattering, and different phase shifts in the beam’s wavefront, all expressed as wavefront distortion. To evaluate the uniformity of the material, it can be measured by small-angle scattering [21]. For the same material, the small-angle scattering signal of single-crystal is smaller than that of polycrystalline material. Sapphire crystal has two forms of single-crystal and polycrystalline. Single-crystal sapphire will not be transformed into polycrystalline after mechanical processing or special processing, which can reduce the small-angle scattering signal, reduce the wavefront distortion, and is more conducive to focusing. A lens made of a single crystal material may cause Bragg diffraction when specific incident photon energies are satisfied, resulting in a decrease in the transmission intensity. Still when only one set of crystal planes is met, the diffraction can be ignored [22]. In addition to single crystal polycrystals affecting the uniformity of sapphire, the growth method of the sapphire also affects the uniformity. Tsai et al. [23] found that optical-grade sapphire grown by the heat exchange method (HEM) had a much lower dislocation density than sapphire grown by the Kylopoulos method (KYM). Obviously, the sapphire grown by the HEM method has better uniformity and is more suitable as a material for sapphire lenses.
iv) Consideration of the machinability of the sapphire lenses. The material of the lens needs to be capable of mechanical processing or special processing, and the properties of the material remain basically unchanged before and after processing. Lenses need to have a good surface profile and surface roughness but are much less demanding than mirrors. This is because the beam propagates almost perpendicular to the interface, rather than at grazing incidence, so high surface precision is not required [7]. Due to the advantages and characteristics of high-resolution processing capability, arbitrary designability, and a wide range of processing materials, femtosecond laser micro-nano processing technology has been widely used in micro-mechanics, micro-optics, optical sensing, and bionic devices. Any complex three-dimensional micro-nano structures preparation. Using femtosecond laser processing technology can realize the preparation of the micro-nano structure of the sapphire crystal. After fabrication, the surface roughness of sapphire can reach several tens of nanometers. The surface roughness of the sapphire-based Fresnel zone plate prepared by Li et al. achieved 12 nm [24]; Wang et al. found that the surface roughness can reach about 15 nm in the anisotropy study of sapphire [25].
Sapphire Lens Focusing Simulation and Thermal Analysis
Focusing Performance Simulation
After comprehensively considering optical performance parameters of the sapphire lenses, the SRW (Synchrotron Radiation Workshop) code [26] is used to perform a case of focusing simulation. The parameter settings are the same as the previous calculation. The simulation layout is shown in Figure 7. The simulation results are shown in Figure 8.
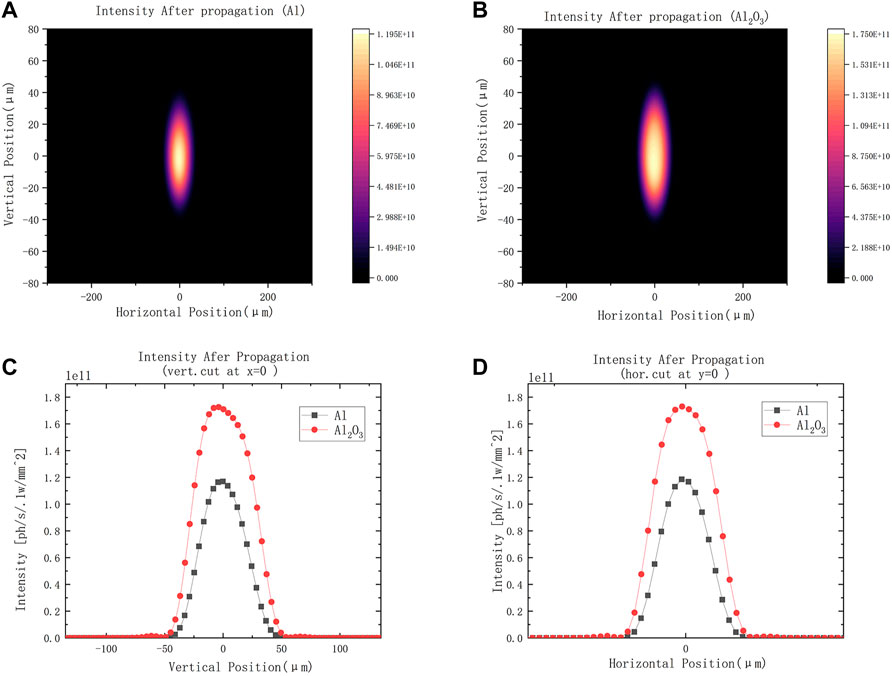
FIGURE 8. (A) Intensity distribution of ∼10 keV X-ray focused with aluminum lens. (B) Intensity distribution of ∼10 keV X-ray focused with sapphire lens. (C) The intensity after propagation at x = 0. (D) The intensity after propagation at y = 0.
Simulations were performed at 10 keV for sapphire and aluminum lenses. The radii of curvature of the sapphire lens and the aluminum lens were both set to 250 μm, and the geometric apertures were both set to 1000 μm. To obtain the same focal length, the number of sapphire lenses is set to 2. And the number of aluminum lenses is set to 3. The calculated focus of the sapphire lens is about 7.66 m and the effective aperture is 279 μm. The calculated focus of the sapphire lens is about 7.64 m and the effective aperture is 221 μm. Figure 8A is the simulation result of the aluminum compound refracting lens, which is the light spot near the focal point. Figure 8B is the simulation result of the sapphire compound refracting lens, which is the light spot near the focal point. Figure 8C is the aluminum lens and the sapphire lens at x = The intensity at 0, Figure 8D is the intensity of the aluminum lens and the sapphire lens at y = 0. It can be seen from Figures (A) and (B) that the light spot formed by the aluminum lens is slightly smaller than that formed by the sapphire lens. The focal spot formed by the sapphire lens is significantly smaller than that formed by the aluminum lens. From Figure (C), the light spot passing through the sapphire lens has a longitudinal half-width of about 50 μm; the light spot passing through the aluminum lens has a longitudinal half-height width of about 47 μm. From Figure (D), the light spot passing through the sapphire lens has a lateral half-height width of about 54 μm; the light spot passing through the aluminum lens has a lateral half-height width of about 38 μm. Under the condition of obtaining the same focal length, the effective aperture of the sapphire lens is larger, and the required number is smaller, and the obtained beam intensity is higher.
Thermal Effects Analysis
The brightness of the third-generation synchrotron radiation source is significantly improved, and the thermal load is also considerably increased, which may cause related deformation. Therefore, the compound refractive lens material needs to have good thermal properties, high-temperature resistance, and low thermal expansion coefficient. For refractive parabolic lenses, the change of focal length with temperature is
Conclusion
The parameters of several commonly used X-ray compound refractive lens materials are calculated and compared, including refraction and absorption ratio, number of lenses, effective aperture, transmittance, resolution, etc. Most calculations show that sapphire is superior to materials such as aluminum and silicon and inferior to materials such as beryllium and diamond. However, in the case of reaching the same focal length, the number of sapphire lenses used is the least, which can reduce the error caused by the length of the lens group. At the same time, the possible cost of the lens during actual use is reduced. Through the lens simulation, it can be found that under the same focal length, the number of sapphire lenses is less, and the transmitted X-ray light is stronger. Through thermal analysis of sapphire, it is found that the highest temperature that may be generated is much lower than the melting point of sapphire, and the resulting thermal stress can also be ignored. In actual use, the processing difficulty of sapphire lens is far less than that of diamond. Considering these factors, the use of sapphire lenses is feasible in future X-ray optics engineering.
In future work, we will conduct further simulations and calculations on the sapphire lens, including the phase defect of the sapphire lens based on X-ray wave optics for diffraction-limited synchrotrons and free-electron lasers [28]. Currently, Sapphire lenses are being fabricated by ultra-fast laser ablation. Experiments will be conducted soon for quality characterization of the sapphire lenses and focusing performances as well.
Data Availability Statement
The original contributions presented in the study are included in the article/Supplementary Material, further inquiries can be directed to the corresponding author.
Author Contributions
YW is responsible for calculations and writing paper. XD is responsible for guidance and revision. JH is responsible for providing guidance.
Funding
This work was supported by the National Natural Science Foundation of China (Grant No.U1832172), and the Science and Technology Major Project of Shanghai (Grant No.2017SHZDZX02), and grants from Talent Program (2018) and R&D program of optics (2021) for large scientific infrastructures of the Chinese Academy of Sciences.
Conflict of Interest
The authors declare that the research was conducted in the absence of any commercial or financial relationships that could be construed as a potential conflict of interest.
Publisher’s Note
All claims expressed in this article are solely those of the authors and do not necessarily represent those of their affiliated organizations, or those of the publisher, the editors and the reviewers. Any product that may be evaluated in this article, or claim that may be made by its manufacturer, is not guaranteed or endorsed by the publisher.
References
1. Ice GE, Budai JD, Pang JWL. The Race to X-Ray Microbeam and Nanobeam Science. Science (2011) 334(6060):1234–9. doi:10.1126/science.1202366
2. Snigirev A, Kohn V, Snigireva I, Lengeler B. A Compound Refractive Lens for Focusing High-Energy X-Rays. Nature (1996) 384(6604):49–51. doi:10.1038/384049a0
3. Lengeler B, Schroer CG, Benner B, Gerhardus A, Günzler TF, Kuhlmann M, et al. Parabolic Refractive X-Ray Lenses. J Sync. Radiat (2002) 9(Pt 3):119–24. doi:10.1107/s0909049502003436
4. Aristov V, Grigoriev M, Kuznetsov S, Shabelnikov L, Yunkin V, Weitkamp T, et al. X-Ray Refractive Planar Lens with Minimized Absorption. Appl Phys Lett (2000) 77(24):4058–60. doi:10.1063/1.1332401
5. Cederström B, Cahn RN, Danielsson M, Lundqvist M, Nygren DR. Focusing Hard X-Rays with Old Lps. Nature (2000) 404(6781):951. doi:10.1038/35010190
6. Beguiristain HR, Anderson IS, Dewhurst CD, Piestrup MA, Cremer JT, Pantell RH. A Simple Neutron Microscope Using a Compound Refractive Lens. Appl Phys Lett (2002) 81(22):4290–2. doi:10.1063/1.1524698
7. Lengeler B, Schroer C, Tümmler J, Benner B, Richwin M, Snigirev A, et al. Imaging by Parabolic Refractive Lenses in the Hard X-Ray Range. J Sync. Radiat (1999) 6(6):1153–67. doi:10.1107/s0909049599009747
8. Terentyev S, Blank V, Polyakov S, Zholudev S, Snigirev A, Polikarpov M, et al. Parabolic Single-Crystal Diamond Lenses for Coherent X-Ray Imaging. Appl Phys Lett (2015) 107(11):111108. doi:10.1063/1.4931357
9.V Aristov, M Grigoriev, S Kuznetsov, L Shabelnikov, V Yunkin, C Rauet al. Silicon Planar Parabolic Lenses. X-Ray Mirrors, Crystals, and Multilayers Conference; 2000 Aug 02-04; San Diego, Ca (2001).
10.I Snigireva, GBM Vaughan, and A Snigirev, editors. High-Energy Nanoscale-Resolution X-Ray Microscopy Based on Refractive Optics on a Long Beamline. 10th International Conference on X-ray Microscopy; 2010 Aug 15-20. Chicago, IL: Univ Chicago (2011).
11.L Zhang, A Snigirev, I Snigireva, G Naylor, A Madsen, F Zontoneet al. Thermo-Mechanical Analysis and Design Optimization of Front-End Compound Refractive Lens. Conference on Design and Microfabrication of Novel X-Ray Optics II; 2004 Aug 05-06; Denver, CO (2004).
12. Medvedev N, Jeschke HO, Ziaja B. Nonthermal Graphitization of Diamond Induced by a Femtosecond X-Ray Laser Pulse. Phys Rev B (2013) 88(22). doi:10.1103/PhysRevB.88.224304
13. Fernandez PB, Graber T, Lee WK, Mills DM, Rogers CS, Assoufid L. Test of a High-Heat-Load Double-Crystal Diamond Monochromator at the Advanced Photon Source. Nucl Instr Methods Phys Res Section a-Accelerators Spectrometers Detectors Associated Equipment (1997) 400(2-3):476–83. doi:10.1016/s0168-9002(97)01014-0
14. Shvyd'ko Y, Stoupin S, Blank V, Terentyev S. Near-100% Bragg Reflectivity of X-Rays. Nat Photon (2011) 5(9):539–42. doi:10.1038/nphoton.2011.197
15. Medvedskaya P, Lyatun I, Shevyrtalov S, Polikarpov M, Snigireva I, Yunkin V, et al. Diamond Refractive Micro-lenses for Full-Field X-Ray Imaging and Microscopy Produced with Ion Beam Lithography. Opt Express (2020) 28(4):4773–85. doi:10.1364/oe.384647
16. Celestre R, Antipov S, Gomez E, Zinn T, Barrett R, Roth T. Polished Diamond X-Ray Lenses. J Sync. Radiat (2022) 29(3):629–43. doi:10.1107/s1600577522001795
17. Mirzaeimoghri M, Morales Martinez A, Panna A, Bennett EE, Lucotte BM, DeVoe DL, et al. Nano-Printed Miniature Compound Refractive Lens for Desktop Hard X-Ray Microscopy. Plos One (2018) 13(8):e0203319. doi:10.1371/journal.pone.0203319
18. Lyubomirskiy M, Koch F, Abrashitova KA, Bessonov VO, Kokareva N, Petrov A, et al. Ptychographic Characterisation of Polymer Compound Refractive Lenses Manufactured by Additive Technology. Opt Express (2019) 27(6):8639–50. doi:10.1364/oe.27.008639
19. Serebrennikov D, Clementyev E, Semenov A, Snigirev A. Optical Performance of Materials for X-Ray Refractive Optics in the Energy Range 8–100 Kev. J Synchrotron Radiat (2016) 23:1315–22. doi:10.1107/S1600577516014508
20.X-Ray Optics Calculator. X-Ray Optics Calculator. Available at: http://purple.iptm.ru/xcalc/xcalc_mysql/ref_index.php (Accessed December 4, 2021).
21.T Roth, L Helfen, J Hallmann, L Samoylova, P Kwasniewski, B Lengeleret al. X-Ray Laminography and Saxs on Beryllium Grades and Lenses and Wavefront Propagation through Imperfect Compound Refractive Lenses. Conference on Advances in X-Ray/EUV Optics and Components IX held as part of the SPIE 2014 International Symposium on Optics + Photonics; 2014 Aug 18-20; San Diego, CA (2014).
22. Terentyev S, Polikarpov M, Snigireva I, Di Michiel M, Zholudev S, Yunkin V, et al. Linear Parabolic Single-Crystal Diamond Refractive Lenses for Synchrotron X-Ray Sources. J Sync. Radiat (2017) 24:103–9. doi:10.1107/s1600577516017331
23. Tsai Y-W, Wu Y-H, Chang Y-Y, Liu W-C, Liu H-L, Chu C-H, et al. Sapphire Hard X-Ray Fabry-Perot Resonators for Synchrotron Experiments. J Sync. Radiat (2016) 23:658–64. doi:10.1107/s1600577516004999
24. Li Q-K, Yu Y-H, Wang L, Cao X-W, Liu X-Q, Sun Y-L, et al. Sapphire-Based Fresnel Zone Plate Fabricated by Femtosecond Laser Direct Writing and Wet Etching. IEEE Photon Technol Lett (2016) 28(12):1290–3. doi:10.1109/lpt.2016.2538270
25. Wang J, Guo B, Zhao Q, Zeng Z, Zhai W, Chen H, et al. Investigation into the Anisotropy of Cross-Grinding Surface Quality in C- and M-Planes of Sapphire. Proc Inst Mech Eng B: J Eng Manufacture (2019) 233(1):44–54. doi:10.1177/0954405417718596
26. Chubar O, Elleaume P. Accurate and Efficient Computation of Synchrotron Radiation in the Near Field Region. In: Proceedings, 6th European conference, EPAC'98; June 22-26, 1998; Stockholm, Sweden, 1-3 (1998). p. 1177–9.
27.Ansys. Ansys. Available from: https://www.ansys.com/ (Accessed October 25, 2021).
Keywords: compound refractive lens, sapphire, x-ray optics, focusing, photon beam conditioning
Citation: Wang Y, Dong X and Hu J (2022) Feasibility Analysis of Sapphire Compound Refractive Lenses for Advanced X-Ray Light Sources. Front. Phys. 10:908380. doi: 10.3389/fphy.2022.908380
Received: 30 March 2022; Accepted: 08 June 2022;
Published: 28 June 2022.
Edited by:
Jifeng Liu, Dartmouth College, United StatesCopyright © 2022 Wang, Dong and Hu. This is an open-access article distributed under the terms of the Creative Commons Attribution License (CC BY). The use, distribution or reproduction in other forums is permitted, provided the original author(s) and the copyright owner(s) are credited and that the original publication in this journal is cited, in accordance with accepted academic practice. No use, distribution or reproduction is permitted which does not comply with these terms.
*Correspondence: Xiaohao Dong, ZG9uZ3hpYW9oYW9AempsYWIub3JnLmNu