- 1State Key Laboratory of Crystal Materials, Shandong University, Jinan, China
- 2Key Laboratory of Functional Crystal Materials and Device, Shandong University, Ministry of Education, Jinan, China
- 3Research Center of Laser Fusion, China Academy of Engineering Physics, Mianyang, China
A series of 98% deuterated DKDP crystals were grown in solutions with different pD values (2.9, 3.3, 3.8, and 4.3) using the rapid growth method. Samples were cut along the z-direction and fourth-harmonic-generation (FHG) direction which contained both pyramidal and prismatic regions. The nonlinear absorption (NLA) coefficient β of 98% deuterated DKDP crystals was obtained using the Z-scan method operated at the FHG wavelength (266 nm) of a picosecond Nd:YAG laser. According to the results, the nonlinear absorption at 266 nm could be identified as two-photon absorption. The β values of crystal grown in the solution with 3.3 pD value were higher than those of crystals grown in solutions with other pD values under higher laser fluence. The results also indicated that FHG device samples should be prepared from the pyramidal region due to its lower β value. This work will help optimizing the application of 98% DKDP crystals as FHG elements in high-power laser systems.
Introduction
KDP and deuterated DKDP crystals are one of the nonlinear optical and electro-optic crystal materials with outstanding performance such as low half-wave voltage, large nonlinear electro-optic coefficient, wide transparency range, relatively high laser-induced damage threshold (LIDT), and the unique ability to grow into a single crystal with large size, making them the only nonlinear optical crystal materials that are used in a high-power laser system for inertial confinement fusion (ICF) [1–6]. Compared with KDP crystals, DKDP crystals have a better electro-optic performance. The electro-optic performance will be enhanced with the increase in deuterium content [7]. Thus, the highly deuterated DKDP crystals with a deuteration level of 98% (described as “98% DKDP” in the later text) would be more suitable for ICF. Moreover, 98% DKDP crystals can be used as nonlinear optical elements for noncritical phase matching (NCPM) FHG of Nd:YAG laser (1064 nm) at 39.7–40 °C [8], which provides a foundation for FHG applications of 1 μm laser with DKDP crystals. Therefore, the optical performance at the frequency of FHG for DKDP crystals should be completely explored.
However, the laser-induced damage (LID) of 98% deuterated DKDP occurs at the working fluence, which greatly restricts the output power of pulsed laser and the working life of components [9, 10]. The LIDT of DKDP crystals decreases with the increase in deuterium content [11]. LID is observed in the bulk and surface of 70% DKDP crystals at the working fluences [12], which predicts that the FHG 98% DKDP is under greater threat. Furthermore, the crystal is more likely to be damaged at shorter wavelengths due to its higher photon energy. It has been considered in many research studies that the laser damage was induced by localized absorption by either foreign nanoparticles incorporated during growth or intrinsic defect clusters formed during crystal growth [13–15]. Even though substantial improvements are made in DKDP growth technology to reduce defects over the past several years [16–19], the actual LIDT is still much lower than the theoretical value. Therefore, clarifying the LID mechanism is urgently needed. NLA of UV light is believed to make great contribution to the LID [20]. The NLA is commonly considered as multiphoton absorption. During harmonic generation, the potential damage to the optical crystal and the adjacent optics caused by the amplified NLA is still of greater concern [21, 22]. Although there has been much research on the NLA of KDP and 70% DKDP [23–27], the NLA property of 98% DKDP is seldom mentioned. So, it is necessary to measure the nonlinear absorption characteristics of 98% DKDP crystals.
In this study, in order to investigate the NLA properties of the 98% deuterated DKDP crystals, the Z-scan method was employed for measuring the NLA coefficient β of FHG wavelength at 266 nm with a picosecond laser. This method is simple and highly sensitive.
Materials and Methods
Calculation
According to multiphoton absorption theories, in the spectral range of Eg/2< hν< Eg, the two-photon absorption (2 PA) plays a dominant role in NLA. The band gap Eg of 98% DKDP crystals was evaluated using the Tauc plot method [28] and is shown in Figure 1 with the value of 5.32 eV. Therefore, the NLA of DKDP crystals at 266 nm (4.66 eV) can be theoretically ascribed as 2 PA, and the absorption coefficient can be described as follows:
where I, α, and β are the laser energy intensity, the linear absorption coefficient, and the nonlinear 2 PA coefficient, respectively. The linear absorption coefficient α can be estimated through the following formula:
where T0, R, and L are the linear transmittance, the reflectivity, and the thickness of sample, respectively. The 2 PA coefficient β can be calculated using the following equation:
where T2PA, I0(t), z, ω0, and λ are the normalized transmittance, the peak intensity, the sample position, the waist radius, and the wave length, respectively.
Sample Preparation
DKDP crystals were grown in deuterated solutions, which were synthesized using heavy water, phosphorus pentoxide, and potassium carbonate. The solution acidity can affect the morphology and optical quality of crystal, which can be characterized by pD value. pD value is the negative of the usual logarithm of the deuterium ion concentration (activity) in an aqueous solution, that is, −lg [D+], just like pH value. 98% DKDP crystals with different pD values of 2.9, 3.3, 3.8, and 4.3 were grown using the rapid growth method. Samples of 1 mm thickness were cut using an HGJ-1300 diamond wire cutting machine from each as-grown crystal along the z-direction and FHG direction, respectively. The sampling locations were optimized to ensure that each sample contained both the pyramidal region and prismatic region. Specific cut is shown in Figure 2. Then, the samples were fine polished using the single-point diamond turning method. Photographs of some samples are shown in Figure 3.
Experimental Procedures
The transmittance spectrum was obtained by Hitachi with a test band from 200 to 2500 nm at room temperature in order to evaluate crystal quality of the pyramidal region and prismatic region. Moreover, the linear absorption coefficient α can be estimated through the transmittance spectrum.
The NLA characteristics of DKDP crystals of FHG wavelength (266 nm) were investigated with a picosecond Nd:YAG laser using the Z-scan method. The schematic representation of the Z-scan experimental setup is shown in Figure 4. FHG wavelength laser was obtained by frequency conversion of 532 nm laser through ADP crystal. A mirror which is HR coated at 532 nm and AR coated at 266 nm was applied in order to avoid the influence of residual 532 nm laser. The polarization direction of 266 nm laser was of vertical polarization, as is shown in Figure 5. The focus is set as zero, and the sample moves from −z to + z. During this procedure, the transmitted laser energy Eb decreased first and then increased, reaching a minimum at the focus. Thus, the ratio of Eb/Ea could be considered as a transmittance function, which is related to the displacement. The laser pulse width and frequency employed in the experiment were 30 ps and 10 Hz, respectively. The radius of the beam waist was 35 μm, and the power densities at focal point I0 were 32 and 22 GW/cm2, respectively.
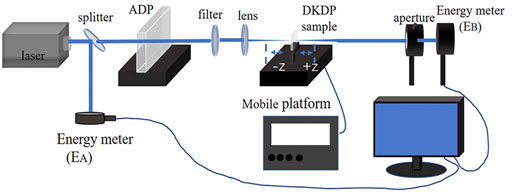
FIGURE 4. The schematic representation of the Z-scan experimental setup (samples were taken from four different positions).
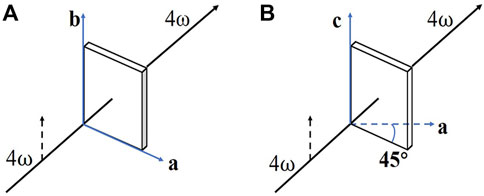
FIGURE 5. Diagram of the polarization direction of FHG laser [(A): z-cut sample; (B) FHG sample. The black dotted arrow shows the polarization direction of incident laser].
Results and Discussion
The transmittance spectra are shown in Figure 6. The linear absorption coefficient α at 266 nm was calculated and listed in Table 1. All the samples showed high transmittance at 350–1600 nm, indicating the high crystallographic quality of as-grown crystals with no visible defects and the good processing of surface. For the prismatic samples, whether in z-cut or FHG cut, there was a drop in transmittance in the range of 200–350 nm, indicating that there was a strong linear absorption at 266 nm. It is believed to be caused by the presence of trivalent metal impurity ions, that is, Fe3+ and Al3+, and the intrinsic defects, that is, hydrogen vacancy, interstitial oxygen, and Frenkel pair [29]. It should be noted that the ultraviolet region transmittance of the 3.3 pD value sample was much lower than that of other pD value samples. In order to reveal the reasons for the differences between them, the metal impurity ion content was determined using the ICP-MS method. According to the ICP-MS results shown in Figure 7, the content of Fe3+ in the 3.3 pD value sample is much higher than that of other samples, indicating that the enrichment of Fe3+ in the 3.3 pD value sample leads to the stronger linear absorption at 266 nm, while for the cut direction, the transmittance of the z-cut samples was approximately the same as that of FHG samples.
NLA With an I0 of 32 GW/cm2
The dependence of NLA versus displacement z was obtained by fitting the experimental data into 2 PA curves. Each sample was irradiated with the same laser fluence at five different positions, and the average results are shown in Figure 8. The test points agreed well with the 2 PA fitting curves, which proves the dominant place of 2 PA in NLA. The specific NLA coefficient β values are shown in Table 2 and Table 3.
All samples exhibited an obvious NLA effect with reverse saturation absorption. During the movement of samples from the beginning to the end, the transmittance curves are valley shaped, which indicated the existence of multiphoton absorption, leading to the decrease in transmittance. The NLA of z-cut samples was stronger than that of the FHG samples, indicating that the NLA of 98% deuterated DKDP was anisotropic. It was mentioned in other research studies that 2 PA is related to the third-order susceptibility [30, 31], with the relationship of
where
On the other hand, the NLA in the prismatic region was stronger than that in the pyramidal region. These results indicate the influence of the growth process on NLA.
Furthermore, the NLA can also be affected by the pD value of the growth solution. Whether for z-cut or FHG-cut samples, the 3.3 pD value samples showed a stronger NLA. It can be found that the proper adjustment of pD values may decrease the NLA to some extent, since the intrinsic NLA of 98% DKDP was very strong at 266 nm. As a result, the effect of pD value on NLA seems limited. Meanwhile, the adjustment of solution acidity may change the growth rate of 98% DKDP crystal greatly. The lower acidity will restrict the growth rate of prismatic faces, and this property has been expounded in other research studies [33, 34]. Therefore, it is still necessary to choose a proper pD value solution for crystal growth, which can both shorten the growth period and reduce NLA.
NLA With an I0 of 22 GW/cm2
The experimental parameters are consistent with those in chapter 3.1 except the lower energy fluence. The fitted curves are shown in Figure 9. The specific NLA coefficient β values are shown in Table 4 and Table 5.
As shown in Figure 9, the test points agreed well with fitted curves with 2 PA, indicating the dominant place of 2 PA in NLA with lower energy. The normalized transmittance curves appeared very close with a minimum around 0.6. As for samples with the same pD value, the NLA coefficient β of z-cut is larger than that of FHG cut, whether in the pyramidal or prismatic region, which is in agreement with the conclusions in higher energy. By comparing the curves in Figure 8, the valleys in prismatic regions seem shallower under an I0 of 22 GW/cm2, while there is no significant difference in valleys in pyramidal regions. Table 4 and Table 5 reveal that the NLA coefficient β of the prismatic region is larger than that of the pyramidal region. However, the difference between the prismatic region and pyramidal region is less under lower laser fluence. The NLA coefficient β in lower energy is larger than that in higher energy, which may be due to the fact that the too deep valley reduces the accuracy of fitting curves. Meanwhile, the effect of pD values on samples seems even smaller than that in high energy since there was no apparent regularity of NLA coefficient β with the varying pD values.
Conclusion
The NLA of 98% deuterated DKDP crystals grown in solutions with different pD values (2.9, 3.3, 3.8, and 4.3) which were cut along the z-direction and FHG direction was measured using the Z-scan method at 266 nm with a picosecond laser. The NLA absorption coefficient β was obtained by fitting the experiment data into 2 PA under different laser fluences. The fitting curves closely agreed to the original points, revealing the fact that the NLA at 266 nm could be identified as 2 PA. According to the results, two main conclusions could be drawn. First, the NLA at 266 nm was anisotropic and closely connected to the grown region. The NLA in the z-direction was stronger than that in the FHG direction. As for same pD value samples, the NLA in the prismatic region was stronger than that in the pyramidal region. Second, the strength of NLA could be affected by the acidity of the growth solution. Under a laser fluence I0 of 32 GW/cm2, the sample of 3.3 pD value has a larger NLA coefficient β, while under a lower I0 of 22 GW/cm2, no apparent regularity is present. These findings could provide a reference in future crystal growth work and understanding the LID mechanism related to NLA. Furthermore, it also indicated that FHG device samples should be cut from the pyramidal region due to its lower β value to extend the working life and efficiency.
Data Availability Statement
The original contributions presented in the study are included in the article/Supplementary Material; further inquiries can be directed to the corresponding author.
Author Contributions
All authors listed have made a substantial, direct, and intellectual contribution to the work and approved it for publication.
Funding
This work was supported by the Young Scholars Program of Shandong University (grant no. 2018WLJH65) and National Natural Science Foundation of China (Grant No. 51902299).
Conflict of Interest
The authors declare that the research was conducted in the absence of any commercial or financial relationships that could be construed as a potential conflict of interest.
Publisher’s Note
All claims expressed in this article are solely those of the authors and do not necessarily represent those of their affiliated organizations or those of the publisher, the editors, and the reviewers. Any product that may be evaluated in this article, or claim that may be made by its manufacturer, is not guaranteed or endorsed by the publisher.
Acknowledgments
Prof. Zhengping Wang (State Key Laboratory of Crystal Materials, Shandong University, China) is acknowledged for providing experimental platform.
References
1. De Yoreo JJ, Burnham AK, Whitman PK. Developing KH2PO4 and KD2PO4 Crystals for the World's Most Power Laser. Int Mater Rev (2013) 47(3):113–52. doi:10.1179/095066001225001085
2. Campbell JH, Hawley-Fedder RA, Stolz CJ, Menapace JA, et al. Nif Optical Materials and Fabrication Technologies: An Overview. In: MA Lane, and CR Wuest, editors Optical Engineering at the Lawrence Livermore National Laboratory II: The National Ignition Facility. San Jose, CA: SPIE (2004).
3. Hawley-Fedder RA, Geraghty P, Locke SN, McBurney MS, et al. Nif Pockels Cell and Frequency Conversion Crystals. In: MA Lane, and CR Wuest, editors Optical Engineering at the Lawrence Livermore National Laboratory II: The National Ignition Facility. San Jose, CA: SPIE (2004).
4. Maunier C, Bouchut P, Bouillet S, Cabane H, Courchinoux R, Defossez P, et al. Growth and Characterization of Large KDP Crystals for High Power Lasers. Opt Mater (2007) 30(1):88–90. doi:10.1016/j.optmat.2006.11.019
5. Jin R-B, Cai N, Huang Y, Hao X-Y, Wang S, Li F, et al. Theoretical Investigation of a Spectrally Pure-State Generation from Isomorphs of KDP Crystal at Near-Infrared and Telecom Wavelengths. Phys Rev Appl (2019) 11(3), 034067-1–034067-9. doi:10.1103/PhysRevApplied.11.034067
6. Sun Z, Cui Z, Sun M, Yuan Y, Li Q, Liu Da., et al. Electro-optic Coefficient Measurement of a K(H1−xDx)2PO4 crystal Based on χ(2) Nonlinear Optical Technology. Opt Express (2021) 29(2):2647–57. doi:10.1364/OE.415262
7. Zhang L, Yu G, Zhou H, Li L, Xu M, Liu B, et al. Study on Rapid Growth of 98% Deuterated Potassium Dihydrogen Phosphate (DKDP) Crystals. J Cryst Growth (2014) 401:190–4. doi:10.1016/j.jcrysgro.2013.10.035
8. Zhang L, Zhang F, Xu M, Wang Z, Sun X. Noncritical Phase Matching Fourth Harmonic Generation Properties of KD2PO4 Crystals. Opt Express (2015) 23(18):23401–13. doi:10.1364/OE.23.023401
9. Wang D, Li T, Wang S, Wang J, Wang Z, Xu X, et al. Study on Nonlinear Refractive Properties of KDP and DKDP Crystals. RSC Adv (2016) 6(18):14490–5. doi:10.1039/c5ra24761f
10. Wang SL, Gao ZS, Fu YJ, Duan AD, Sun X, Fang CS, et al. Study on Rapid Growth of Highly-Deuterated DKDP Crystals. Cryst Res Technol (2003) 38(11):941–5. doi:10.1002/crat.200310117
11. Liu B, Hu G, Zhao Y, Xu M, Ji S, Zhu L, et al. Laser Induced Damage of DKDP Crystals with Different Deuterated Degrees. Opt Laser Technol (2013) 45:469–72. doi:10.1016/j.optlastec.2012.06.008
12. Demos SG, DeMange P, Negres RA, Feit MD. Investigation of the Electronic and Physical Properties of Defect Structures Responsible for Laser-Induced Damage in DKDP Crystals. Opt Express (2010) 18(13):13788–804. doi:10.1364/OE.18.013788
13. Feit MD, Exarhos GJ, Rubenchik AM, Guenther AH, Kaiser N, Lewis KL, et al. Implications of Nanoabsorber Initiators for Damage Probability Curves, Pulselength Scaling, and Laser Conditioning. In: 35th Annual Symposium on Optical Materials for High-Power Lasers; SEP 22-24, 2003; Boulder, Colorado, United States. SPIE (2004). doi:10.1117/12.523862
14. Negres RA, DeMange RAN, Radousky HB, Demos SG Differentiation of Defect Populations Responsible for Bulk Laser-Induced Damage in Potassium Dihydrogen Phosphate Crystals. Opt Eng (2006) 45(10):104205. doi:10.1117/1.2363166
15. DeMange P, Negres RA, Radousky HB, Demos SG. Laser-Induced Defect Reactions Governing Damage Performance in KDP and DKDP Crystals. In: PE Powers, editor Nonlinear Frequency Generation and Conversion: Materials, Devices, and Applications V. San Jose, CA: SPIE (2006).
16. Zhang L, Zhang F, Xu M, Wang Z, Sun X. Rapid Growth of a Large Size, Highly Deuterated DKDP Crystal and its Efficient Noncritical Phase Matching Fourth-Harmonic-Generation of a Nd:Yag Laser. RSC Adv (2015) 5(91):74858–63. doi:10.1039/c5ra14772g
17. Cai X, Lin X, Li G, Lu J, Hu Z, Zheng G. Rapid Growth and Properties of Large-Aperture 98%-Deuterated DKDP Crystals. High Power Laser Sci Eng (2019) 7:e46. doi:10.1017/hpl.2019.24
18. Bhagavannarayana G, Rajesh P, Ramasamy P. Interesting Growth Features in Potassium Dihydrogen Phosphate: Unravelling the Origin and Dynamics of Point Defects in Single Crystals. J Appl Cryst (2010) 43(6):1372–6. doi:10.1107/s0021889810033649
19. Anis M, Muley GG, Baig MI, Rabbani G, Ghramh HA, Ramteke SP. Doping Effect of Ni2+ on Structural, UV-Visible, SHG Efficiency, Dielectric and Microhardness Traits of KH2PO4 (KDP) crystal. Optik (2019) 178:752–7. doi:10.1016/j.ijleo.2018.10.061
20. Chai X, Zhu Q, Feng B, Li F, Feng X, Wang F, et al. Nonlinear Absorption Properties of DKDP Crystal at 263 Nm and 351 Nm. Opt Mater (2017) 64:262–7. doi:10.1016/j.optmat.2016.12.010
21. Reyné S, Duchateau G, Natoli J-Y, Lamaignère L. Laser-induced Damage of KDP Crystals by 1ω Nanosecond Pulses: Influence of crystal Orientation. Opt Express (2009) 17(24):21652–65. doi:10.1364/OE.17.021652
22. Wang D, Li T, Wang S, Wang J, Shen C, Ding J, et al. Characteristics of Nonlinear Optical Absorption and Refraction for KDP and DKDP Crystals. Opt Mater Express (2017) 7(2):533. doi:10.1364/ome.7.000533
23. Cai D, Wang M, Zhang L, Xu M, Wang Z, Chai X, et al. Third-Harmonic-Generation Nonlinear Absorption Coefficient of 70% Deuterated DKDP Crystal. Opt Mater Express (2017) 7(12):4386. doi:10.1364/ome.7.004386
24. Gurzadyan GG, Ispiryan RK. Two‐photon Absorption Peculiarities of Potassium Dihydrogen Phosphate crystal at 216 Nm. Appl Phys Lett (1991) 59(6):630–1. doi:10.1063/1.105406
25. Divall M, Osvay K, Kurdi G, Divall EJ, Klebniczki J, Bohus J, et al. Two-Photon-Absorption of Frequency Converter Crystals at 248 Nm. Appl Phys B (2005) 81(8):1123–6. doi:10.1007/s00340-005-1954-7
26. Xu L, Lu C, Wang S, Huang P, Liu H, Zhang L, et al. A Study on Nonlinear Absorption Uniformity in a KDP Crystal at 532 Nm. CrystEngComm (2020) 22(32):5338–44. doi:10.1039/d0ce00811g
27. Peng X, Zhao Y, Li D, Hu G, Zhang L, Shao J, et al. Characterization of Thermal Absorption and Nonlinear Absorption in KDP/DKDP Crystals with Different Orientations. In: Optical Measurement Systems for Industrial Inspection XI. Munich, Germany: SPIE (2019). doi:10.1117/12.2525460
28. Pétursson J, Marshall JM, Owen AE. Optical Absorption in As-Se Glasses. Philosophical Mag B (2006) 63(1):15–31. doi:10.1080/01418639108224428
29. Kang T, Lan J, Wang D, Liu G, Shen C, Hou R, et al. Influences of Fe3+ Ions on Rapid Growth and Laser-Induced Nonlinear Refraction of Potassium Dihydrogen Phosphate crystal. Opt Mater (2020) 104:109924. doi:10.1016/j.optmat.2020.109924
30. Fan H, Wang X, Ren Q, Li T, Zhao X, Sun J, et al. Third-Order Nonlinear Optical Properties in [(C4H9)4N]2[Cu(C3S5)2]-Doped Pmma Thin Film Using Z-Scan Technique in Picosecond Pulse. Appl Phys A (2009) 99(1):279–84. doi:10.1007/s00339-009-5521-7
31. Wang S, Zhang Y, Zhang R, Yu H, Zhang H, Xiong Q. High-Order Nonlinearity of Surface Plasmon Resonance in Au Nanoparticles: Paradoxical Combination of Saturable and Reverse-Saturable Absorption. Adv Opt Mater (2015) 3(10):1342–8. doi:10.1002/adom.201500240
32. Wang Y, Sun D, Chen J, Shen C, Liu G, Wang D, et al. Linear and Nonlinear Optical Characteristics Effected by Na+ Ions of Low Concentration for Potassium Dihydrogen Phosphate crystal. Optik (2022) 251:168481. doi:10.1016/j.ijleo.2021.168481
33. Bredikhin VI, Galushkina GL, Ershov VP, Rubakha VI, Shvetsova NR. Rapid Growth of DKDP Crystals from High-Acidity Solutions. J Cryst Growth (1999) 207(1-2):122–6. doi:10.1016/s0022-0248(99)00343-7
Keywords: 98% DKDP crystal, pD value, nonlinear optic absorption, fourth-harmonic-generation, Z-scan
Citation: Wu P, Zhang L, Xu M, Liu B, Wei L, Li Y, Chai X and Sun X (2022) Research on the Nonlinear Absorption Coefficient of 98% Deuterated DKDP Crystal at Fourth-Harmonic-Generation Wavelength. Front. Phys. 10:901370. doi: 10.3389/fphy.2022.901370
Received: 21 March 2022; Accepted: 06 June 2022;
Published: 05 July 2022.
Edited by:
Chengbao Yao, Harbin Normal University, ChinaReviewed by:
Yu Fang, Suzhou University of Science and Technology, ChinaXingzhi Wu, Suzhou University of Science and Technology, China
Copyright © 2022 Wu, Zhang, Xu, Liu, Wei, Li, Chai and Sun. This is an open-access article distributed under the terms of the Creative Commons Attribution License (CC BY). The use, distribution or reproduction in other forums is permitted, provided the original author(s) and the copyright owner(s) are credited and that the original publication in this journal is cited, in accordance with accepted academic practice. No use, distribution or reproduction is permitted which does not comply with these terms.
*Correspondence: Lisong Zhang, emhsc0BzZHUuZWR1LmNu