- 1Meiji Institute for Advanced Study of Mathematical Sciences, Meiji University, Nakano, Japan
- 2Graduate School of Integrated Sciences for Life, Hiroshima University, Higashi-Hiroshima, Japan
Ants flexibly change their behavioral modes at various levels, from individuals to whole colonies, based on external stimuli, such as finding a new food source or being attacked by predators. In this study, we investigated mode changes in the foraging behavior of garden ants, Lasius japonicus. When extracted pheromone was deposited along a circular path connected to the nest entrance and no food was supplied, a large fraction of the ants continued to walk along the path. In contrast, if a food source was placed on the same circle at the opposite edge to the nest entrance, a large fraction of ants soon came to shuttle directly between the nest and the food source using a shortcut path. We analyzed the process of shifting the foraging path from the initial circular path to the shortcut path, and found that the foragers who reached the food source in the initial stage along the circular pheromone path chose the shortcut path for their homing instead of the circular path; thereafter, the traffic along the shortcut path gradually increased both in the outward and homeward directions. This strongly indicates that the ants changed the foraging mode after the food-intake from the pheromone-following mode to another mode. The present result serves as an example of the switching of collective behavior of animals caused by that of the primarily relying information for individuals according to the input of external stimuli.
1 Introduction
Ants are typical eusocial insects. Despite their small brain size, ants perform various tasks in a highly organized manner when working together as a colony through autonomously functioning task allocation with no particular leader. To date, various types of collective ant behavior have been investigated. In particular, foraging behavior has been intensively studied because it is the most fundamental task for survival. One of the characteristic features of the foraging process of many ant species is pheromone-mediated trail formation. According to previous studies, pheromone trails are formed using the following procedures [4, 16]: first, scout ants who reach new food sources deposit. Trail pheromone on their way back to the nest. Thereafter, the foragers who have been waiting in the nest respond to the information provided by the returned scout ants and depart to forage by following the pheromone trail to reach the food source. On their way back to the nest, they deposit another trail pheromone, similar to the above-mentioned scouts. Repetition of these processes increases the density of pheromones along the trail, thereby enabling the colony to effectively carry food to the nest. Information shared among numerous ants through deposition and sensing pheromones is categorized as social information. In contrast, several ant species do not form pheromone trails during foraging. Instead, they rely only on information acquired by the abilities of each individuals, such as the visually obtained route memory to food sources [1, 5, 10, 11, 14] and memory of the required steps to reach the food, which is categorized as private (or personal) information. Another style of foraging without pheromone trail formation involves recruiters showing nest-mates how to reach food sources through tandem running, during which the information exchanged among foragers is categorized as social information. In these ways, ants utilize various types of information in foraging, with the primary information used depending on the species and situation. The combined use of more than one piece of information has been observed in some ant species. For example, the parallel use of remembering the steps required for foragers to reach food sources from the nest (i.e., odometer) and sensing the polarization of sunlight (i.e., sun-compass) enables path integration in desert ants, with which they recognize the temporal location at every moment during foraging [2, 17]. This is a combination of the different types of private information. Another form of combinatory use of multiple information is hierarchical use. For example, in a food-seeking experiment with Lasius niger, the ants primarily relied on the stored memory of the feeding location for individuals when the indicated feeding directions conflicted, as opposed to the usual information from the pheromone trail [1, 10]. Also in this study, we investigated the mode changes in the foraging behavior of Lasius japonicus, however, unlike the previous studies [3–5], we investigated the mode-change caused by the input of stimulus, i.e. the food intake.
2 Materials and Methods
2.1 Experimental Animals
We collected three colonies of Lasius japonicus from the Higashi-Hiroshima campus of Hiroshima University. Each colony was housed in a breeding box (30 × 40 cm) containing a plastic nest box (10 × 10 cm). The bottom of each nest box was covered with a layer of plaster. The top of each nest box was covered with cardboard to block light. A rubber tube was connected to the nest box at the entrance. The colonies were queenless, comprising 500–700 workers and a small number of broods. We fed the colonies once every 2 days with 0.5 ml of 1 M sugar solution and once a week with a mealworm. The experiments were performed in a temperature-controlled room, with a light-dark cycle of 12:12 h and the lights turned on at 8:00. The room windows were covered to prevent natural light. The room contained artificial room lights, different types of lab equipment, and furniture, which would provide visual landmarks for the ants.
2.2 Pheromone Extraction and Column Chromatography
To obtain trail pheromone during the experimental trials, solutes were extracted from 100 ants using hexane (10 ml) for 3 days. The extract solutes were concentrated to 4 ml in an evaporator. The concentrated solutes were then separated using column chromatography as follows. A column was prepared by packing glass wool and silica gel powder into the tip of a disposable glass Pasteur pipette. First, the extracted solutes were loaded onto the column. Subsequently, the column was eluted with mixture solutes (50 ml per eluent) containing hexane and diethyl ether, and the mixing ratio of each eluent was set stepwise (hexane/diethyl ether ratios of 10:0, 9:1, 7:3, and 5:5). Every 10 ml of the eluate fraction was collected into glass bottles (the bottle was labeled as ID:1–20).
To select an eluate, including the trail pheromone, the eluates of the bottles were examined using a biological assay. We compared the attractiveness of the eluate for three consecutive bottle IDs [i.e., (1,2,3), (2,3,4), (3,4,5)…(18, 19, 20)]. Three solutes were placed along a trident shape on fresh paper, in which each pronged spear comprised one solute in the bottle. We then forced ants to forage on the paper, recorded their foraging behavior with a video camera, and then counted the number of ants walking on each spear for 5 min. Eluates, including the trail pheromone, were selected based on the number of ants following the corresponding spear. The eluate from the bottle was diluted 30–50 times by mixing with hexane and was then used for the subsequent foraging experiments.
2.3 Experimental Setup
We placed a fresh paper over a foraging platform for each trial. We then prepared a pheromone trail that had a circle shape using the selected extracted pheromone. We applied the pheromone trail along the circle with a radius of 10 cm on the paper using a microsyringe as an initial pheromone path. It is known that the ants following the trail tried to find a new path when they reached the point where the two straights crossed [7, 12]. The ants following the circle path could reach the food source without being disturbed because the circle path is smooth. A glass plate was provided as a feeder platform on the edge of the circle, and the nest box was placed on the other side, as shown in Figure 1.
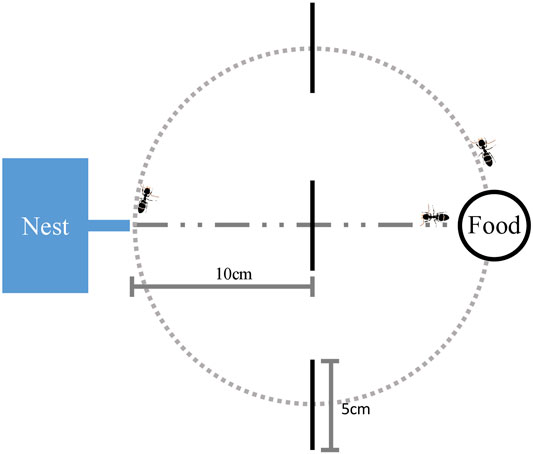
FIGURE 1. Experimental setup. The dotted line indicates the initial pheromone path, where the trail pheromone was deposited along a circle with a radius of 10 cm. The dash-dotted line indicates the shortcut path. A feeder platform was placed on the edge of the circle and the nest was placed on the other side. The black vertical lines represent the decision lines.
Two experiments without a food source and twelve experiments with a food source in total (four subgroups of three colonies) were conducted. The two experiments were set up without sugar solution on the feeder platform to confirm that the ants could follow the initial pheromone path for 60 min. The twelve experiments with a food source were performed in the same place in the room and every few days thereafter. The ants starved during the days between the conducted experiments. The experiments with a food source were recorded for 90 min per trial. The video for all experiments provided images at a resolution of 1280 × 720 at 30 frames/s.
2.4 Video Analysis
The two-dimensional position coordinates for each ant were measured from the recorded video sequences using the background subtraction method. First, every frame in the video sequence was converted to grayscale frames, and then the background image was calculated by storing the highest intensity value of each pixel from the series of preceding frames. Second, we subtracted the background image from all frames to calculate the foreground images. Thus, we binarized the extracted foreground images. Third, we defined the ant position coordinate as the center of the labeled pixel region whose size was between 5 and 100 pixels to retain only ants. Following these three steps, we measured the position of each ant in each frame; however, the relation between the positions in the subsequent frame was unknown. Thus, we defined the nearest positional coordinate in the subsequent frame as the coordinate of the same ant if the magnitude of the difference vector between the coordinates was less than 1 cm. The difference vector between the two coordinates served as a velocity vector for the ant.
Following the above processes, we measured the trajectories of the ants within the two frames and velocity vector sequences, as shown in Figure 2. Using the measured information, we classified the outward and homeward ants based on velocity vectors. We quantified the temporal dynamics of the ant traffic flow on decision lines, which were defined at the center of the circle and at the initial pheromone path, as shown in Figure 1. We counted the number of ants crossing each decision line for outward and homeward independently as traffic flow, and defined no,s, nh,s, no,i, and nh,i as the number of ants crossing the shortcut path for outward and homeward and the initial path for outward and homeward, respectively. In this study, we regarded that the ants went foraging or homed after reaching the food source when they crossed decision lines, respectively, but the ants could cross the outside of the decision lines. To confirm that the ants walked the two paths, we counted all ants crossing the centerline for outward and homeward as no and ns. If no,s + no,i ≊ no and nh,s + nh,i ≊ nh, we could assume that the ants in the experiments attended the foraging and homing after finding the food source. In addition, we did not follow all ants’ trajectories to confirm that the ants turned around while walking on the two paths without reaching the food source and whether the ants reached the food source or not because of technical difficulty. However, we confirmed that these cases were relatively rare cases while checking the original videos. Each traffic flow was normalized by the total number of traffic flows for each direction, no and nh, and was referred to as the proportion of traffic flow. We compared the proportion of traffic flow between the shortcut path and the initial pheromone path. We referred to the proportion of the traffic flow of ants on the shortcut path as fo,s = no,s/no for outward and fh,s = nh,s/nh for homeward, and that on the initial pheromone path as fo,i = no,i/no for outward and fh,i = nh,i/nh for homeward.
2.5 Statistical Analysis
Significant differences in the proportion of traffic flow on the shortcut path and that on the initial pheromone path every 3 min were confirmed using a paired t-test in R software v.3.3.1 [15].
3 Results
3.1 Experiments Without a Food Source
We initially set up two experiments to examine whether foragers followed a pheromone trail along a circular path. For this purpose, a pheromone trail was deposited on a circular path without food. In this situation, a large fraction of foragers kept walking on the circular path until the end of the two experiments, both of which continued for 60 min, as shown in Figure 3.
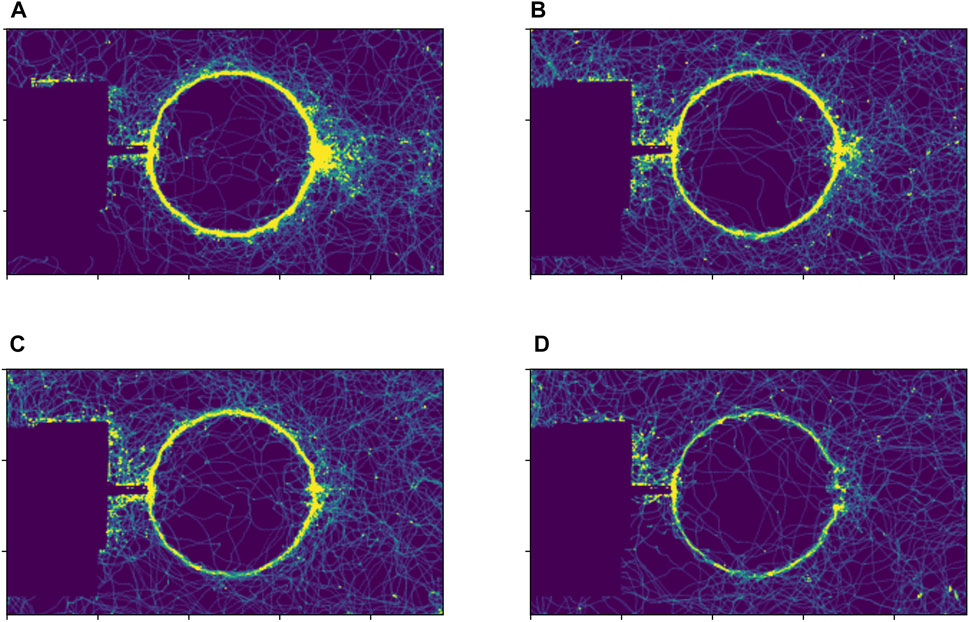
FIGURE 3. Example of the density of foraging ants in the experiment without a food source: (A) an interval from 0 to 3 min, (B) an interval from 3 to 6 min, (C) an interval from 6 to 9 min, (D) an interval from 9 min to 12 min.
3.2 Experiments With a Food Source
Next, the food source was placed on the path, and 12 experiments were conducted in total (four subgroups of three colonies). Within the 12 experiments, we only analyzed the data of the experiments in which the first five ants reached the food source following the initial pheromone trail to investigate the collective behavioral change of the foragers, that is, the primary foraging route changes from the initial pheromone path to the shortcut path. Eight of the 12 experiments satisfied this criterion. Most ants traveled outward from the nest entrance to the food source on the initial pheromone trail during the first 3 minutes. However, there was no statistically significant difference between the number of homeward ants along the initial pheromone path and that along the shortcut path. In addition, we discuss the results of video analysis for all experiments from the start to 45 min, because the foraging ants finished taking the sugar solution at 45 min.
During the next time interval from 3 to 15 min, the fraction of outward ants, fo,s, that crossed the decision line, including the shortcut path, monotonically increased, whereas the fraction of outward ants, fo,i, that crossed the decision lines, including the initial pheromone path, remained constant, as shown in Figure 4. The ratio fo,s also increased, however, fo,s and fo,i did not exhibit significant differences until 18 min (p > 0.05), as shown in Figure 5. fo,s and fo,i in a 18–21 interval showed a significant difference (p < 0.05). In contrast to the outward ants, the number of homeward ants, fh,s, that crossed the decision line on the shortcut path was consistently larger than fh,i that crossing the decision line on the initial pheromone path, and fh,s and fh,i were significantly different (p < 0.01) except a 15–18 interval (p > 0.05). The time series of the number of ants that crossed the decision lines as shown in Figure 4 revealed that the foraging ants that relied on the initial pheromone path ignored social information and instead used other information. After 3 min, fh,s was approximately 0.7, which was significantly different from fh,i (p < 0.01) except a 15–18 interval (p > 0.05).
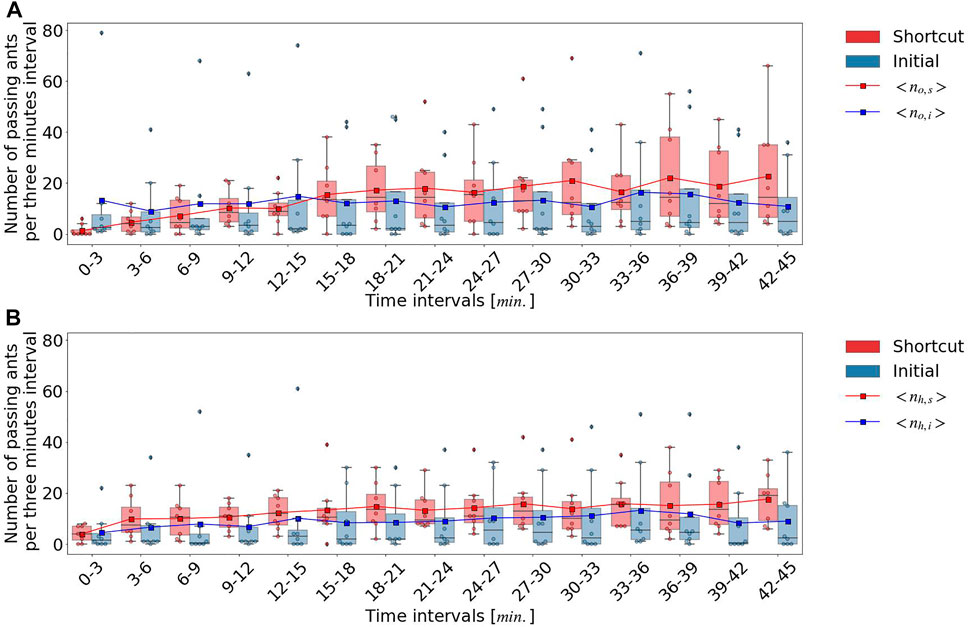
FIGURE 4. Number of ants passing each decision line per 3 min interval: (A) outward ants. (B) homeward ants.
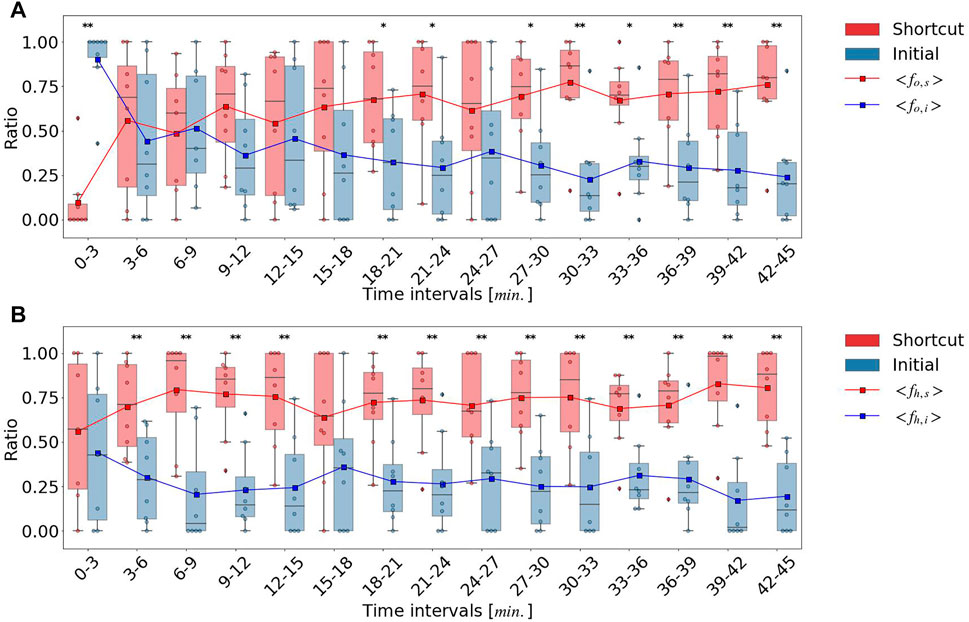
FIGURE 5. Ratio between the number of ants passing each decision line: (A) outward ants. (B) homeward ants.
This outcome indicates that the ants preferred to use the shortcut path as the main foraging trail. Notably, the outward foraging ants that walked along the initial pheromone path were retained in a certain fraction, indicating that the pre-set trail pheromone pheromone trail strongly attracted the outward foraging ants.
4 Discussion
We observed that the fed ants collectively tended to switch their foraging behavior mode. The workers that led foraging in the early stages of the experiments walked homeward in areas where no other workers had previously walked. This behavior indicates that the workers independently decided to ignore the trail pheromone and choose to walk across the shortcut path between the food source and their nest entrance.
It is natural to suppose that the workers’ decision might be based on some information, which should be the workers’ private information because they did not use any social information provided by the pheromone trail and, instead, started to walk to areas for which no social information existed. The possible candidates for the private information which the workers used were their visual information, odometer, or previous memory. In addition, our results indicated that ants may use private information for the homeward journey, which contradicted with the expected behavior based on the hypothesis that ants use pheromones for homing.
Another possible mechanism is the emergence of stochastic effects in the sensitivity of pheromone trails, which allow ants to ignore the established pheromone trail. Based on previous simulation studies, a circular path that is initially set is optimized to a shortcut path in the following steps [4, 6, 8]. First, the ants wander around the vicinity of the circular path and deposit pheromones there. Second, because the ants following the inside of the circular path reach the resources faster than those following the circular path, the trail pheromone on the inside of the circular path becomes more concentrated than that on the circular path. Finally, the primarily used path shifts to the inside of the circular path. By repeating these steps, the primarily used path gradually converges to the shortcut path between the nest and the resource. However, in our experiments, ants discontinuously switched their homeward path from the original pheromone path to the shortcut path. In addition, if the stochastic effects on the sensitivity of the pheromone trail are significant, the ants should spread out in all directions. As the ants headed directly to their nests in our experiment, it is likely that our ants used private information.
Reid et al. [13] evaluated the decision-making mechanism regarding information selection in ants when forced to choose particular information following the removal of the existing information and reported that ants head back to their nest using private information when a pheromone trail is physically blocked. In their study, the appropriate information was externally shut down, and thus, ants were forced to use private information to cover the loss. In contrast, we observed that ants switched the prior homing information from chemical cues to private cues, even though the foraging environment remained unchanged. However, the mechanism for selecting private information without the loss of chemical cues requires further investigation. Our results were consistent with those of Grueter et al. [9], who found that the costs and benefits of information use depend on the context, leading to a change in the primarily used information. In our experiments, the time cost of searching for unknown food sources was high in the outward journey because the pheromone trail was presented and the food source was known. Conversely, relying on private information is beneficial because the time taken for a straight path is less than that for a circular path. The ants that completed the outward journey then acquired up-to-date private information. In summary, the ants succeeded in gaining benefits and decreasing costs by selecting information.
Our data indicate that not only the initial circular pheromone path but the shortcut path also came to work as a pheromone trail with time proceeded. This is because the traffic flow on the shortcut path increased with time. In general, ants on pheromone trails and those who have previously experienced foraging move faster than inexperienced ants or those on unmarked trails [3]. Therefore, subsequent ants could use multiple types of information, potentially increasing the efficiency of ant transport.
In our experiments, we only analyzed the collective foraging behavior of the ants, and thus we indirectly concluded the possibility of private information use in their homeward foraging behavior. The individual ants are small but it still have complex nervous system. That means that the observed behavior might be related to multiple input information. To confirm the origin of their private information, we have to do experiments that strictly control the available cues related to the private information. We conduced the experiments in the laboratory with many instruments which might be used as landmarks to locate their heading direction. It is required to conduct an experiment controlling the visual environment.
Data Availability Statement
The raw data supporting the conclusion of this article will be made available by the authors, without undue reservation.
Author Contributions
Conceived and designed the experiments: OY, MS, SI, AA, and HN Performed the experiments: OY, YO, YI, and YT Analyzed the data: OY, MS, and HN Wrote the paper: OY, MS, and HN.
Funding
This study was financially supported by Grant-in-Aid for Scientific Research (B) (Grant No. 20H01871), Grant-in-Aid for Transformative Research Areas (A) (Grant No. 21H05297), and the Promotion Project for Development of a Characteristic Joint Research Base of the Ministry of Education, Culture, Sports, Science and Technology (JPMXP0620335886).
Conflict of Interest
The authors declare that the research was conducted in the absence of any commercial or financial relationships that could be construed as a potential conflict of interest.
Publisher’s Note
All claims expressed in this article are solely those of the authors and do not necessarily represent those of their affiliated organizations, or those of the publisher, the editors and the reviewers. Any product that may be evaluated in this article, or claim that may be made by its manufacturer, is not guaranteed or endorsed by the publisher.
References
1. Aron S, Beckers R, Deneubourg JL, Pasteels JM. Memory and Chemical Communication in the Orientation of Two Mass-Recruiting Ant Species. Ins Soc (1993) 40:369–80. doi:10.1007/bf01253900
2. Collett M, Collett TS. How Do Insects Use Path Integration for Their Navigation? Biol Cybernetics (2000) 83:245–59. doi:10.1007/s004220000168
3. Czaczkes TJ, Grüter C, Jones SM, Ratnieks FLW. Synergy between Social and Private Information Increases Foraging Efficiency in Ants. Biol Lett (2011) 7:521–4. doi:10.1098/rsbl.2011.0067
4. Czaczkes TJ, Grüter C, Ratnieks FL. Trail Pheromones: an Integrative View of Their Role in Social Insect colony Organization. Annu Rev Entomol (2015) 60:581–99. doi:10.1146/annurev-ento-010814-020627
5. Czaczkes TJ, Salmane AK, Klampfleuthner FA, Heinze J. Private Information Alone Can Trigger Trapping of Ant Colonies in Local Feeding Optima. J Exp Biol (2016) 219:744–51. doi:10.1242/jeb.131847
6. Deneubourg J-L, Aron S, Goss S, Pasteels JM. The Self-Organizing Exploratory Pattern of the Argentine Ant. J Insect Behav (1990) 3:159–68. doi:10.1007/bf01417909
7. Forster A, Czaczkes TJ, Warner E, Woodall T, Martin E, Ratnieks FLW. Effect of Trail Bifurcation Asymmetry and Pheromone Presence or Absence on Trail Choice byLasius nigerAnts. Ethology (2014) 120:768–75. doi:10.1111/eth.12248
8. Goss S, Aron S, Deneubourg JL, Pasteels JM. Self-organized Shortcuts in the Argentine Ant. Naturwissenschaften (1989) 76:579–81. doi:10.1007/bf00462870
9. Grueter C, Leadbeater E. Insights from Insects about Adaptive Social Information Use. Trends Ecol Evol (2014) 29:177–84.
10. Grüter C, Czaczkes TJ, Ratnieks FL. Decision Making in Ant Foragers (Lasius niger) Facing Conflicting Private and Social Information. Behav Ecol Sociobiol (2011) 65:141–8.
11. Minoura M, Sonoda K, Sakiyama T, Gunji Y-P. Rotating Panoramic View: Interaction between Visual and Olfactory Cues in Ants. R Soc Open Sci (2016) 3:150426. doi:10.1098/rsos.150426
12. Ogihara Y, Yamanaka O, Akino T, Izumi S, Awazu A, Nishimori H. Switching of Primarily Relied Information by Ants: A Combinatorial Study of experiment and Modeling. In: Mathematical Approaches to Biological Systems. Berlin, Germany: Springer (2015). p. 119–37. doi:10.1007/978-4-431-55444-8_6
13. Reid CR, Sumpter DJT, Beekman M. Optimisation in a Natural System: Argentine Ants Solve the Towers of Hanoi. J Exp Biol (2011) 214:50–8. doi:10.1242/jeb.048173
14. Sakiyama T, Gunji YP. Garden Ant Homing Behavior in a Maze Task Based on Local Visual Cues. Insect Soc (2013) 60:155–62. doi:10.1007/s00040-012-0279-x
16. Wilson EO. A Chemical Releaser of Alarm and Digging Behavior in the Ant Pogonomyrmex Badius (Latreille). Psyche: A J Entomol (1958) 65:41–51. doi:10.1155/1958/57483
Keywords: foraging behavior, pheromone trail, ant, Lasius japonicus, private information, navigation
Citation: Yamanaka O, Oki Y, Imamura Y, Tamura Y, Shiraishi M, Izumi S, Awazu A and Nishimori H (2022) Ants Alter Collective Behavior After Feeding and Generate Shortcut Paths on a Two-Dimensional Foraging Area. Front. Phys. 10:896717. doi: 10.3389/fphy.2022.896717
Received: 15 March 2022; Accepted: 22 April 2022;
Published: 11 May 2022.
Edited by:
Nobuhiko J. Suematsu, Meiji University, JapanReviewed by:
Adam Lipowski, Adam Mickiewicz University, PolandTomoko Sakiyama, Sōka University, Japan
Copyright © 2022 Yamanaka, Oki, Imamura, Tamura, Shiraishi, Izumi, Awazu and Nishimori. This is an open-access article distributed under the terms of the Creative Commons Attribution License (CC BY). The use, distribution or reproduction in other forums is permitted, provided the original author(s) and the copyright owner(s) are credited and that the original publication in this journal is cited, in accordance with accepted academic practice. No use, distribution or reproduction is permitted which does not comply with these terms.
*Correspondence: Masashi Shiraishi, c2hpcmFpc2hpLm11QGdtYWlsLmNvbQ==