- 1College of Petroleum Engineering, Xi’an Shiyou University, Xi’an, China
- 2State Key Laboratory of Continental Dynamics and Department of Geology, Northwest University, Xi’an, China
- 3No.1 Gas Production Plant of PetroChina Changqing Oilfield Company, Jingbian, China
- 4School of Human Settlements and Civil Engineering, Xi’an Jiaotong University, Xi’an, China
- 5Xi’an Key Laboratory of Tight Oil (Shale Oil) Development, Xi’an Shiyou University, Xi’an, China
It is generally believed that conventional hydrocarbon accumulates in sandstone traps after migrating at a certain distance. Unconventional hydrocarbon only migrates at a short distance before accumulation or being imbibed in the tiny pores of shale and tight sandstone reservoir, and there is no obvious trap boundary. There are also different views on the time interval between hydrocarbon accumulation and migration. In this paper, microscale simulation experiments are used to study the process of oil accumulation during migration. In the single pore-throat model, oil can 100% saturate the pore in the process of migration with continuous oil supply. In the plane sandstone model, when oil migrates through the entire model, the oil-bearing area can reach more than 50% of the model area. In the sandstone reservoir model, when the front edge of oil migrates through the entire sandstone layer, the oil saturation near the injection point can reach more than 90%, while the oil saturation of the front part of the model is only about 50%. It shows that, in sandstone reservoir, when there are sufficient oil source and continuous charging pressure, the oil saturation near the charging point continues to increase during oil migration, and the range of high oil-bearing area continues to expand. Therefore, there can be a certain number of hydrocarbon accumulations along the migration path in sandstone reservoir under sufficient oil supply and continuous charging pressure, rather than just in traps.
Introduction
Conventional hydrocarbon is mainly considered as accumulating in sandstone traps. There are various theories of conventional hydrocarbon accumulation, such as anticlinal reservoir theory [1–3], subtle reservoir [4], petroleum system [5–7], etc. It is believed that conventional hydrocarbon accumulations in sandstone reservoir are formed after a certain distance of secondary migration. The vertical migration distance of conventional hydrocarbon can generally reach several kilometers, which depends on the strata thickness and the vertical extension distance of the fault. If there is sufficient source, when the migration channel is well connected, hydrocarbon can migrate a long distance laterally before accumulation, and the flow of oil and gas in sandstone reservoir has also been studied [9–12]. For unconventional hydrocarbon in tight sandstone reservoir, it is considered that buoyancy makes little contribution to the hydrocarbon migration and accumulation, and the residual pressure difference between source rock and reservoir is the main driving force [13–21]. The migration distance of unconventional hydrocarbon is considered to be short. Unconventional hydrocarbon mainly accumulates in the “sweet spots” sandstone with relatively high porosity and permeability in the reservoir or be imbibed in the tiny pores of shale and tight sandstone reservoir [18, 20, 22–25].
The process and time of hydrocarbon accumulation has been studied. Some theories believe that the time when sandstone traps are formed and the time when the source rock is faulted should not be later than the large-scale hydrocarbon expulsion period, so that hydrocarbon can accumulate on a large scale. Even if the time of the formation of sandstone traps is later than the time of hydrocarbon migration, it is better if the time interval is smaller [26, 27]. Some other theories believe that the time of trap formation, oil source fault activity, and reservoir forming of many large and medium-sized hydrocarbon accumulations are significantly later than the time of large-scale hydrocarbon expulsion from source rock [28]. Niu Chengmin et al. consider that the time of hydrocarbon accumulation is much later than the time of massive hydrocarbon generation, and there is a time lag in hydrocarbon migration and accumulation [29]. Based on existing theories, this paper uses physical simulation experiments to study the process of hydrocarbon accumulation during migration in sandstone reservoir.
Materials and Methods
Single Pore-Throat Water-Wet Model
This experiment aims to simulate the oil accumulation during migration in a single pore. The experimental model is a water-wet pipe, with two thin glass pipes with 2-mm diameter (simulating throat) and a spherical glass with 8-mm diameter (simulating pore) combined together. The water sample is tap water:
The model is saturated with water, and the kerosene is injected into the thin pipe at the speed of 0.3 ml/min to observe the process of oil migration and accumulation.
Plane Sandstone Model
This experiment observes the change of oil saturation during the migration process from a plane perspective. The experimental model is composed of two 50-mm-long and 30-mm-wide water-wet thin slices, sandwiched with 1-mm-thick and 1–5-mm-long quartz particle slices. The water sample is tap water:
The model is filled with water first, and then kerosene is injected from one end of the model at the speed of 0.3 ml/min to observe the process of oil migration and accumulation.
Sandstone Reservoir Model
This experiment is to observe the change of oil saturation during the migration process in sandstone sediment. The experimental model is a glass pipe with the diameter of 9 mm and the length of 120 cm, which is loaded with a 95-cm-long sandstone layer composed of homogeneous medium grain quartz sand particles. The water sample is tap water:
The model is saturated with water and is placed at an inclination of 28° to simulate the tilted strata. Kerosene is injected at the speed of 0.1 ml/min until the front edge of oil breaks through the top sand layer to observe the process of oil migration and accumulation.
Results and Discussion
Oil Migration and Accumulation in the Single Pore-Throat Water-Wet Model
When injected in the thin pipe (simulating throat), the oil phase moves forward continuously with the front edge in a convex shape that pointing to the water phase (Figure 1A). When oil enters the spherical space (simulating pore), with the continuous injection, the front edge of oil no longer moves forward but expands in a spherical shape, drives away the water in the pore gradually (Figure 1B), and then occupies the whole pore (Figure 1C). After the oil fills the entire pore, it begins to migrate to the next throat (Figure 1D). The oil continuously enters the water saturated pipe and drives the water out.
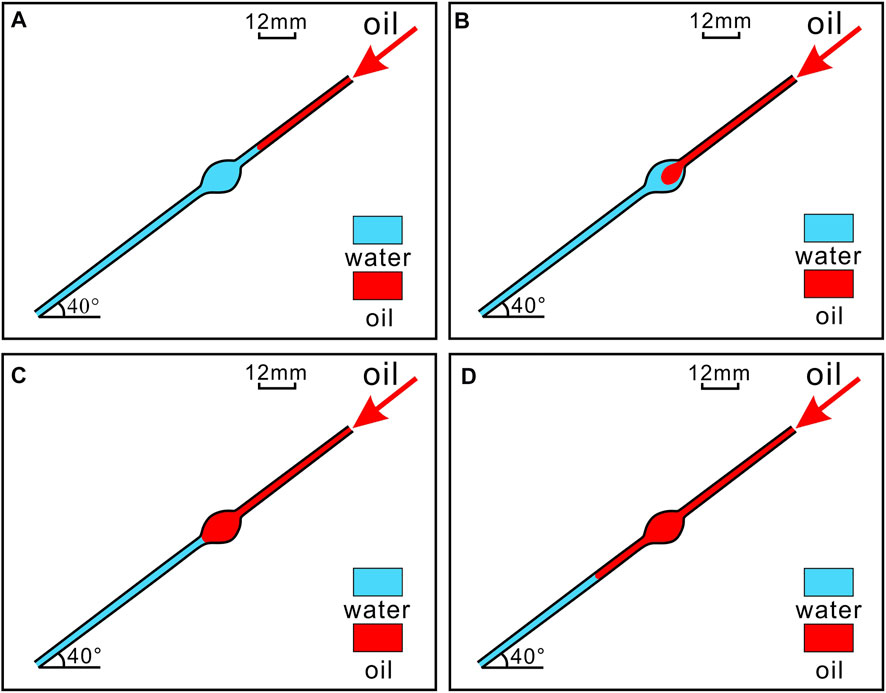
FIGURE 1. Oil accumulation characteristics in the water-wet single pore-throat model. (A) Oil migrating in the throat. (B) The volume of oil in the pore increases in a spherical shape. (C) Oil fills the entire pore. (D) Oil migrates into another throat.
Because of surface tension, the oil phase is surrounded by equal force all around, so that the oil does not directly enter the next throat, but the volume of it increases in a spherical shape. When the oil phase expands to fill the whole pore, under the resistance of the pore wall, the front edge of the oil begins to migrate to the new throat, indicating that when the source is sufficient, oil will migrate in a continuous phase, and under the resistance of the throat and pore wall, oil can 100% saturate a single pore.
Oil Migration and Accumulation in the Plane Sandstone Model
When injected in the model, oil first fills the large pores with lower capillary resistance near the injection end of the model. Since there are no small throats in the direction perpendicular to the injection direction at the injection end of the model, the oil does not migrate along the injection pressure, but first almost 100% saturates the large pores in the direction perpendicular to the injection pressure, and then oil breaks through a throat in the direction of injection pressure (Figure 2A). In the pore, the volume of oil increases in a circular shape until it saturates the whole pore and drives away the water. Then, the oil continues to break through the second throat and enters another pore, with only a thin water film retained between the oil phase and the pore skeleton (Figure 2B). The process of oil migration repeats. The oil does not always migrate along the injection direction but continuously saturates the pores on the migration path. When the oil migrates to about 1/3 position of the model, the oil-bearing area exceeds 80% of the migration area (Figure 2C). When the front edge of oil reaches the other end of the model, the oil-bearing area exceeds 50% of the model area (Figure 2D).
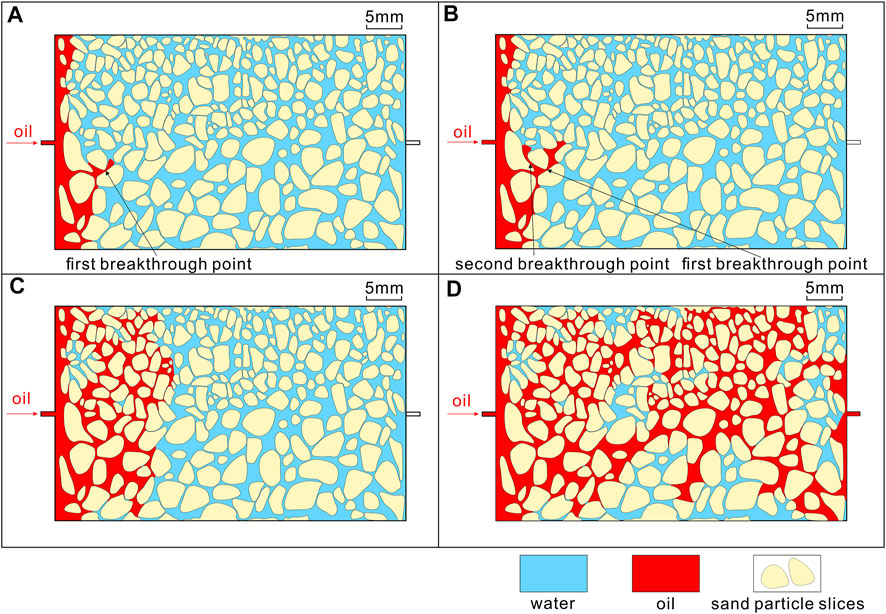
FIGURE 2. Oil migration and accumulation characteristics in the plane sandstone model. (A) Oil saturates the large pores in the direction perpendicular to the injection pressure and breaks through the throat for the first time. (B) After oil fills a pore, it breaks through the throat in one direction. (C) The oil does not always migrate along the injection direction, but continuously fills the pores on the migration path. (D) Distribution characteristics of oil-bearing area when the oil migrates to the other end of the model.
In the process of oil injection, the pores on the migration path will be filled by oil, but not all large pores contain oil. In Figure 3, the pores that are enclosed by the green circles are excellent storage space, but none of the throats around the pores is broken through by oil, so there is no oil accumulation in these large pores. Near the discharge end of the model, some large pores with no throat surrounded are still not filled by oil because the oil preferentially flows from the discharge end. Therefore, whether a pore can be filled by oil depends on the pressure and the connectivity between the pore and the throats around it. If there are several throats around a pore and their ability of connectivity is different, the oil will preferentially migrate to the throat with good connectivity and fill the pore it connects. When the oil migrates out of the pore through a throat, the pressure in the pore is continuously released, and the pores connected by the throats with poor connectivity will not be filled by oil, no matter how large the pores are.
The Process of Oil Migration and Accumulation in the Sandstone Reservoir Model
During the injection process, the front edge of migrating oil is finger-shaped and often extends forward along a main channel. There are many bifurcations on the front edge of the oil, resulting in a conical migration area. When the front edge of oil moved about 15 cm, the oil saturation near the injection point is more than 90%, but only about 20% at the front edge (Figure 4A). Continue to inject oil slowly and the oil saturation near the injection point continues to increase and the shape of the front part of oil is winding (Figure 4B). When oil migrates through the entire sandstone layer and breaks through the top of the sandstone layer, the injection is stopped. At this time, the front part of oil is more winding with about 50% oil saturation, and the oil saturation near the breakthrough point is only about 20%. However, the lower part of sandstone layer is almost saturated with oil, in which the oil saturation can reach more than 90% (Figure 4C). It shows that under a sufficient oil source and continuous pressure, the oil continues to accumulate in the process of migration. Due to the higher pressure near the injection point, it is easier for oil to break through the constraints of the throats and enter the pores. As the migration distance increases, the friction of oil migration increases, and the injection pressure directly acts on oil near the breakthrough point is smaller. Therefore, the oil saturation at the injection point increases the fastest, while the oil saturation at the far end of the injection point increases slowly, and the oil migration path is winding. The range of high oil-bearing area constantly expands in the direction of oil migration during continuous injection.
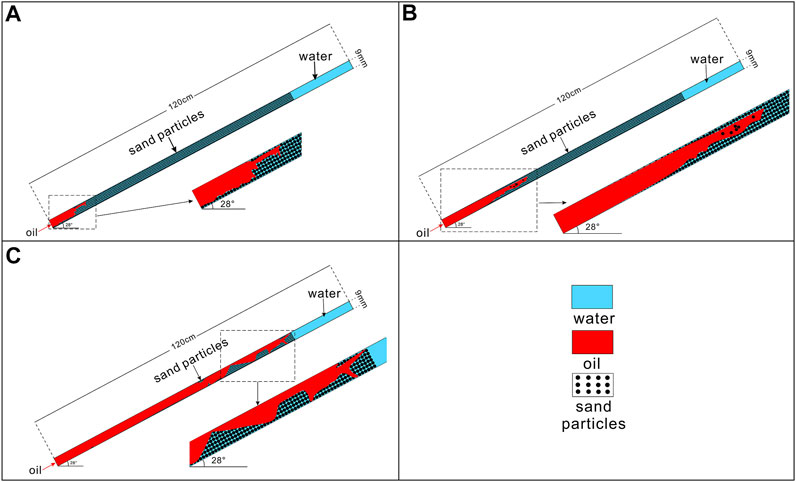
FIGURE 4. Oil migration and accumulation characteristics in the sandstone reservoir model. (A) The front edge of migrating oil is in finger shape. (B) The oil saturation near the injection end increases, and the shape of the front part of oil is winding. (C) When oil breaks through the top of sandstone layer, there is a high oil saturation in the lower part of the model and the front part of oil is more winding with low oil saturation.
The results of the three experiments showed the change of oil saturation during migration from one single pore to real sandstone model, which is from micro perspective to macro perspective. The results indicate that under continuous pressure and sufficient oil source, oil does not always migrate in the direction of pressure reduction in sandstone reservoir but continuously charges the pores on the migration path in irregular directions. Depending on the difference of the resistance of throats around the pores and the charging pressure, oil preferentially breaks through the throats with low resistance near the charging point and charges the pores connected by them. Then oil breaks through other throats to charge the pores. Unconventional sandstone reservoir is very tight, but it is close to the source rock and can get great charging pressure. Therefore, oil can migrate a short distance in tight sandstone reservoir and some tiny pores can be charged by oil during the migration process. For conventional hydrocarbon, although the sandstone reservoir has better physical properties, it is usually far away from source rock, and the main driving force is buoyant force, which is much smaller than the charging pressure of source rock. Therefore, conventional hydrocarbon mainly migrates through the throats with good connectivity and accumulates in the large pores in traps. However, the saturation of hydrocarbon keeps increasing during the process of migration, so there can be a certain number of hydrocarbon accumulations along the migration path in sandstone reservoir, rather than just in traps.
Conclusion
(1) Under sufficient oil supply and continuous pressure, oil does not directly migrate forward in a single pore, but the volume increases in a spherical shape until the oil 100% saturated the pore, and then oil migrates through a throat into another pore.
(2) In sandstone reservoir, the oil does not always migrate along the direction of charging pressure but continuously saturates the pores on the migration path in irregular directions. The pores near the charging point and connected by the throats with good connectivity are preferentially charged by oil, and as the migration distance increases, the proportion of oil-bearing pores along the migration path decreases.
(3) In sandstone reservoir, when the oil supply is sufficient and with a continuous charging pressure, the oil saturation along the migration path will continue to increase and some oil accumulations will be formed. Therefore, there can be a certain amount of hydrocarbon accumulations along the migration path in sandstone reservoir, rather than just in traps.
Data Availability Statement
The original contributions presented in the study are included in the article/Supplementary Material; further inquiries can be directed to the corresponding author.
Author Contributions
DR and ZW contributed to the conception and design of the study. HZ organized the database and performed the statistical analysis. DL wrote the first draft of the manuscript and drew the figures. BG and RZ contributed to manuscript revision, read, and approved the submitted version.
Funding
This study is financially supported by the Young Science and Technology Talents Foundation of Shaanxi Province (2019KJXX-054), Shaanxi Province Key Research and Development Plan (2021GY-140), National Natural Science Foundation of China (51874240, 51934005, and 52074226), Open Fund of Key Laboratory of Coal Resources Exploration and Comprehensive Utilization, Ministry of Natural Resources (KF2021-3), and Open Fund of Shaanxi Key Laboratory of Advanced Stimulation Technology for Oil and Gas Reservoirs (20JS120).
Conflict of Interest
Author BG was employed by the No.1 Gas Production Plant of PetroChina Changqing Oilfield Company.
The remaining authors declare that the research was conducted in the absence of any commercial or financial relationships that could be construed as a potential conflict of interest.
Publisher’s Note
All claims expressed in this article are solely those of the authors and do not necessarily represent those of their affiliated organizations, or those of the publisher, the editors, and the reviewers. Any product that may be evaluated in this article, or claim that may be made by its manufacturer, is not guaranteed or endorsed by the publisher.
References
1. Wilson WB. In: Wrather. F Lahee WE, editor. H.Problems of Petroleum Geology. TulsaUnited States: OklahomaAAPG Memoir (1934). p. 433–45.Proposed Classification of Oil and Gas Reservoirs
4. A. I. Levorsen AI. Big Geology for Big Needs. Bulletin (1964) 48(5):141–56. doi:10.1306/bc743bd7-16be-11d7-8645000102c1865d
5. W. G. Dow WG. Application of Oil Correlation and Source-Rock Data to Exploration in Williston Basin: Abstract. Bulletin (1972) 56(3):615. doi:10.1306/819a3f00-16c5-11d7-8645000102c1865d
6. Wallace G. Dow WG. Application of Oil-Correlation and Source-Rock Data to Exploration in Williston basin. Bulletin (1974) 58(7):125–1262. doi:10.1306/83d91655-16c7-11d7-8645000102c1865d
7. Magoon LB, Dow WG. The Petroleum System Status of Research and Methods. USGS Bull (1992) 20(7):98.
8. Tissot BP. Migration of Hydrocarbon in Sedimentary Basins: A Geological, Geochemical and Historical Perspective. In: Doligez B, editor. Migration of Hydrocarbons in Sedimentary Basins. Paris: Editions Technip (1987). p. 1–26.
9. Luo XR, Zhou B, Zhao SX, Zhang FQ, Vasseur G. Quantitative Estimates of Oil Losses during Migration, Part I: the Saturation of Pathways in Carrier Beds. J Pet Geol (2007) 30(4):375–87. doi:10.1111/j.1747-5457.2007.00375.x
10. Luo X. Understandings on Dynamical Studies of Hydrocarbon Migration and Accumulation. Nat Gas Geosci (2008) 19(2):149–56. doi:10.11764/j.issn.1672-1926.2008.02.149
11. Luo X. Simulation and Characterization of Pathway Heterogeneity of Secondary Hydrocarbon Migration. Bulletin (2011) 95(6):881–98. doi:10.1306/11191010027
12. Wang H, Kou Z, Bagdonas DA, Phillips EHW, Alvarado V, Johnson AC, et al. Multiscale Petrophysical Characterization and Flow Unit Classification of the Minnelusa Eolian Sandstones. J Hydrol (2022) 607:127466. doi:10.1016/j.jhydrol.2022.127466
13. B. E. Law, W. W. Dickinson BE, Dickinson WW. Conceptual Model for Origin of Abnormally Pressured Gas Accumulations in Low-Permeability Reservoirs. Bulletin (1985) 69(8):1295–304. doi:10.1306/ad462bd7-16f7-11d7-8645000102c1865d
14. Law BE, Curtis JB. Introduction to Unconventional Petroleum Systems. Bulletin (2002) 86(11):1851–2. doi:10.1306/61eedda0-173e-11d7-8645000102c1865d
15. Zhang J, Chang X, Zhang J. Deep basin Gas Trap in the Upper Paleozoic of Ordos Basin. Pet Exploration Dev (2000) 27(4):30–5. doi:10.3321/j.issn:1000-0747.2000.04.007
16. Zhang J, Liu L, Tang X, Song X, Jiang S, Xu B, et al. Study on the Effect of Source-Contacting Gas Accumulations upon Abnormal Pressures in Western Sichuan Depression. Earth Sci Front (2008) 15(2):147–55. doi:10.1016/s1872-5791(08)60029-9
17. Nelson PH. Pore-throat Sizes in Sandstones, Tight Sandstones, and Shales. Bulletin (2009) 93(3):329–40. doi:10.1306/10240808059
18. Sonnenberg SA, Pramudito A. Petroleum Geology of the Giant Elm Coulee Field, Williston Basin. Bulletin (2009) 93(9):1127–53. doi:10.1306/05280909006
19. Zou C, Tao S, Yuan X, Zhu R. The Formation Conditions and Distribution Characteristics of Continuous Petroleum Accumulations. Acta Petrolei Sinica (2009) 30(3):324–31. doi:10.7623/syxb2009002
20. Zou C, Zhu R, Wu S. Types, Characteristics, Genesis and Prospects of Conventional and Unconventional Hydrocarbon Accumulations: Taking Tight Oil and Tight Gas in China as an Instance. Acta Petrolei Sinica (2012) 33(2):173–87. doi:10.7623/syxb201202001
21. Jia C, Zheng M, Zhang Y. Unconventional Hydrocarbon Resources in China and the prospect of Exploration and Development. Pet Exploration Dev (2012) 39(2):129–36. doi:10.1016/s1876-3804(12)60026-3
22. Hou Q, Zhaosheng W, Zhao Z. Deep basin Reservoir in Songliao Basin. Pet Exploration Dev (2006) 33(4):406–11. doi:10.3321/j.issn:1000-0747.2006.04.003
23. Yurewicz DA, Bohacs KM, Klimentidis RE, Meurer ME, Yeakel JD, Kendall J, et al. Controls on Gas and Water Distribution, Mesaverde basin-centered Gas Play, Piceance Basin, Colorado. AAPG Hedberg Ser (2008) 3:105–36. doi:10.1306/13131053H31639
24. Jia C, Pang X, Song Y. The Mechanism of Unconventional Hydrocarbon Formation: Hydrocarbon Self-Containment and Intermolecular Forces. Pet exploration Dev (2021) 48(03):437–52. doi:10.11698/PED.2021.03.0110.1016/s1876-3804(21)60042-3
25. Liu D, Ren D, Du K, Qi Y, Ye F. Impacts of mineral Composition and Pore Structure on Spontaneous Imbibition in Tight sandstone. J Pet Sci Eng (2021) 201(1):108397. doi:10.1016/j.petrol.2021.108397
26. Zhu G, Yang H, Zhang B, Si J. Ultra-long Distance Migration of Hydrocarbon. Acta Petrologica Sinica (2013) 29(9):3192–212. doi:10.1117/12.790809
27. Gao N, Fu G, Wang H. Time-Space Matching Relation between Source Rock and Oil-Source Faults of Ed1 Member in Nanpu Sag and its Control Effect on Hydrocarbon Accumulation. J Xi’an Shiyou Univ (Natural Science) (2017) 32(4):11–7. doi:10.3969/j.issn.1673-064X.2017.04.002
28. Lei DW, Zhang J, Chen N, Xiang B. Conditions for Gas Pooling in the Lower Assemblage in the Southern Margin of the Junggar Basin and the Exploration Prospect of Large Hydrocarbon fields. NATURAL GAS INDUSTRY (2012) 32(2):16–22. doi:10.3787/j.issn.1000-0976.2012.02.004
Keywords: oil, sandstone, pore, throat, accumulation
Citation: Ren D, Zhang H, Wang Z, Ge B, Liu D and Zhang R (2022) Experimental Study on Microscale Simulation of Oil Accumulation in Sandstone Reservoir. Front. Phys. 10:841989. doi: 10.3389/fphy.2022.841989
Received: 23 December 2021; Accepted: 17 February 2022;
Published: 06 April 2022.
Edited by:
Qingxiang Meng, Hohai University, ChinaReviewed by:
Meng Wang, Chang’an University, ChinaHuifei Tao, Northwest Institute of Eco-Environment and Resources (CAS), China
Copyright © 2022 Ren, Zhang, Wang, Ge, Liu and Zhang. This is an open-access article distributed under the terms of the Creative Commons Attribution License (CC BY). The use, distribution or reproduction in other forums is permitted, provided the original author(s) and the copyright owner(s) are credited and that the original publication in this journal is cited, in accordance with accepted academic practice. No use, distribution or reproduction is permitted which does not comply with these terms.
*Correspondence: Dazhong Ren, dzren@xsyu.edu.cn