- 1State Key Laboratory of Petroleum Resources and Prospecting, China University of Petroleum, Beijing, China
- 2College of New Energy and Materials, China University of Petroleum, Beijing, China
- 3Beijing Key Laboratory of Optical Detection Technology for Oil and Gas, China University of Petroleum, Beijing, China
- 4Key Laboratory of Oil and Gas Terahertz Spectroscopy and Photoelectric Detection, Petroleum and Chemical Industry Federation, China University of Petroleum, Beijing, China
The depositional products of sedimentary rocks provide vital references for investigating the paleoenvironment, paleogeography, and tectonic evolution history. However, the detection methods of geological evolution are still relatively complicated, and how to combine geological age and geological evolution is confusing. Based on the sensitivity of terahertz waves to organic matter, a THz dating method was introduced for characterizing the geological age of sedimentary rocks. In this study, the geological evolution of Liujiang Basin was analyzed by using terahertz time domain spectroscopy (THz-TDS). According to the close relation between organic matter content and sedimentary environment, it can be inferred that the geological deposition in this area is affected by Marine cover. In addition, the refractive index of Ordovician sedimentary rocks is significantly higher than that of other sedimentary rocks. Based on these results, it is inferred that the sedimentary environment of the Liujiang Basin gradually changed from continental deposition to deep-water marine deposition from the Neoproterozoic to the Ordovician, and the sea water gradually retreated due to the crustal movement, resulting in a transition from deep-water deposition to continental inshore ocean facies deposition. These findings are highly consistent with the geological history of the study area. Combined with principal component analysis (PCA) technology, the relative geological age of sedimentary rocks can be divided. Our study con-firmed the reliability of this THz dating technique, which provides an effective way to study the geological evolution history of sedimentary rocks.
Introduction
Sedimentary strata provide natural records of geologic history, and the accurate determination of their sequence and age is a prerequisite for studying their paleoenvironment, paleogeography, and tectonic evolution history [1–3]. Time, space, materials, and movement are the basic elements used to study geological processes and environmental evolution; and time is the basis for studying geological problems. A clear understanding of the interactions between the above elements enables the clear analysis of the evolution of the Earth and the conditions of rock formation. In addition, determining the geological evolution of rocks is also of great significance to the exploration and development of mineral deposits [4–8]. Certain minerals are usually associated with a particular geological body, and the particular geological body is formed during a particular geological period, which requires a definite sedimentary environment for the rocks. Therefore, it is of great significance to identify the geological ages of rocks.
In order to deeply understand and discuss the occurrence and development of major geological events during Earth’s evolution, it is necessary to determine the ages of the geological systems that preserve this important information. The chemical compositions of sedimentary rocks are influenced by their diagenetic compositions, their tectonic environments, and the weathering transport of materials from the source area. The stratigraphic sequence constitutes a record of large- and small-scale events that occurred throughout geologic history. The geochemical compositions of sedimentary rocks can be studied to obtain information about the chemical environment, composition, and tectonic environment of the source area when they were deposited. The sedimentary rocks investigated in this study only underwent very shallow metamorphism, so they retain the physical characteristics of the original rocks. At present, the dating of sediments relies entirely on the radio-carbon dating of organic matter, which includes problems such as scarcity, age limitations, and associations. Radiocarbon dating methods-key methods which are currently being used - have significant analytical limitations. These approaches may therefore result in significant chronological uncertainty for stratum, hampering a direct comparison with historical data [9–23]. However, because this dating method has age limitations, after obtaining the approximate age, it is necessary to analyze the geological evolution law together with other testing methods. Recently, the terahertz (THz) spectrum has also been used in geological investigations [24, 25]. THz radiation can penetrate nonpolar materials and exhibits strong absorption when it encounters proteins, polar organics, and others [26–30]. The deposition of organic matter is an important index used to investigate geological evolution. Thus, the THz wave can be used to simultaneously analyze the geological evolution of the strata when it is used to determine the geological ages of the strata, named as THz dating.
In this study, the THz spectroscopy was used to study sedimentary rock samples from nine areas with six different geological ages. The evolution of the strata was deduced using the changes in the absorption coefficient and the refractive index with geologic age. Then, principal component analysis (PCA) was employed to classify the geologic ages.
Experiment
The principle of terahertz time domain spectroscopy (THz-TDS) (Figure 1) begins with a femtosecond laser producing an optical-pulse train. Each pulse separates into two paths. Two beams are obtained, i.e., the pump pulse and the beam. Once it reaches the THz emitter, it is excited by the pump light, and then, it is focused by a hemispheric lens onto the parabolic mirror M1 and is reflected onto the indium titanium oxide. After the beam passes through the focus position used to place the sample, it reaches the lens, obtains the THz parallel pulse after the focus, and enters the THz detector of the detection system after reflecting off M4. The other part of the pulse also reaches the detector after passing through a time-delay stage.
The rocks were cored from area with different ages (Table 1). The geographical locations of the rocks are shown in Figure 2. The x-ray diffraction (XRD) images and thermogravimetric images of the three samples are shown in Figure 3. Based on the result of XRD, sample I of Jurassic Period was composed of quartz and calcite, in contrast, sample of Cambrian Period and sample II Jurassic Period were composed of quartz, calcite and soda felspar. Besides, on the basis of thermogravimetric image, sample I of Jurassic Period is similar with sample of Cambrian Period. The second sample showed a significant radiation peak at 754.1°C. Samples with the same geological age may not have the same properties, and samples with similar physical properties may not have the same geological age.
In this research, a Ti:sapphire laser is utilized with a center wavelength of 800 nm, a pulse width of 100 fs, and a repetition rate of 80 MHz. The temperature and humidity were 294.6 ± 0.5K and 0–0.5%, respectively in this experiment. Multiple samples from each age are selected for testing and each sample is further processed. The rocks were cut into thin slices about 2 mm thick, and then, they were polished to confirm the parallelism between the upper and lower surfaces of the cores before the THz measurement. In order to be accurate and eliminate the influence caused by the uneven distribution in the sample, three random points in each sample are selected for testing, and the average value is used to calculate the optical parameters. A fast Fourier transform was applied to the data to obtain the frequency-dependent spectra. The refractive index and absorption coefficient of the sample are obtained by further analysis.
Result and Discussion
Initially, we performed a basic characterization of the THz dielectric effects of the sedimentary rocks with six different geologic ages. Figure 4A shows the THz field signal amplitude as a function of time after the transmission of the THz pulses through the six sedimentary rocks. The THz signal peaks and the relative delay times of the samples are different, indicating that the physical properties can be differentiated using the THz spectra.
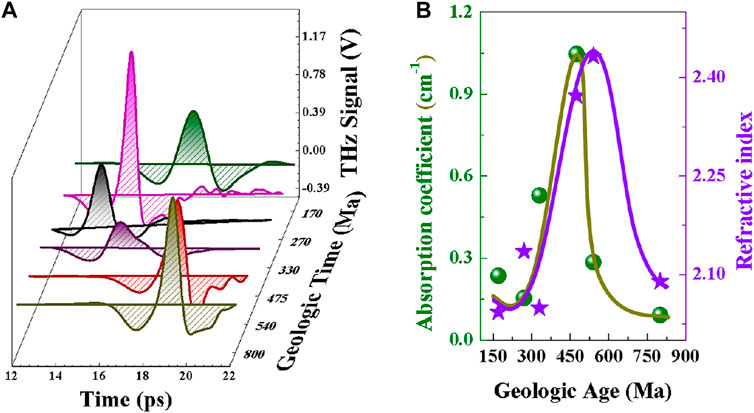
FIGURE 4. (A) THz-TDS results for the six sedimentary rocks with different geological ages; and (B) Absorption coefficients and refractive indexes of the six sedimentary rocks with different geological ages.
The Ordovician rocks had the lowest peak intensities, followed by the Carboniferous, Jurassic, Permian, Cambrian, and Neoproterozoic rocks. In addition, there were also significant differences in the time delays of the six sedimentary rocks. Therefore, fast Fourier transform (FFT) was used to derive the THz frequency domain spectra, and the THz absorption coefficient spectra and refractive index spectra were calculated.
The refractive indexes and absorption coefficients of the sedimentary rock samples with six different geological ages are shown in Figure 4B. As the geological age in-creases, the absorption coefficient and refractive index initially increase and then decrease. The peaks ap-pear in the Ordovician and Cambrian. The THz tests were carried out on a large number of sedimentary rock samples to ensure the accuracy of the absorption coefficient and refractive index variations.
The THz tests were performed on the sedimentary rock samples from the Liujiang Basin (rocks of Permian, Carboniferous, Ordovician, Cambrian and Neoproterozoic), and the absorption coefficients obtained are shown in Figure 5A. The absorption coefficients of these samples were analyzed, and the collective tendency of the absorption coefficient with geological age was plotted. The absorption coefficients of the sedimentary rock samples of each age were averaged, and the mean values were used to plot a curve. As the geological age increased, overall the absorption coefficient initially increased and then decreased. By plotting the samples from the Sichuan Basin (sedimentary rocks of the Jurassic Period) and the sedimentary rocks from the Liujiang Basin on the same graph, it was found that the samples from Sichuan Basin conform to the evolution law of the sedimentary rocks from the Liujiang Basin. This demonstrates that the geological evolutions of the two basins were similar in the Jurassic and Cambrian. It is of great interest that 475 Ma was the turning point, to the left of which the absorption coefficient increased gradually and to the right of which the absorption coefficient de-creased. Similar to the absorption coefficient values at any one frequency, the Ordovician samples had higher absorption coefficients than the other five geologic ages. The Neoproterozoic samples had the lowest values. Moreover, the differences in the absorption coefficients of the other four geologic ages were small, which made qualitative identification and determination difficult. Thus, the present spectra are not sufficient to directly reveal the relationship between the components and the THz spectra.
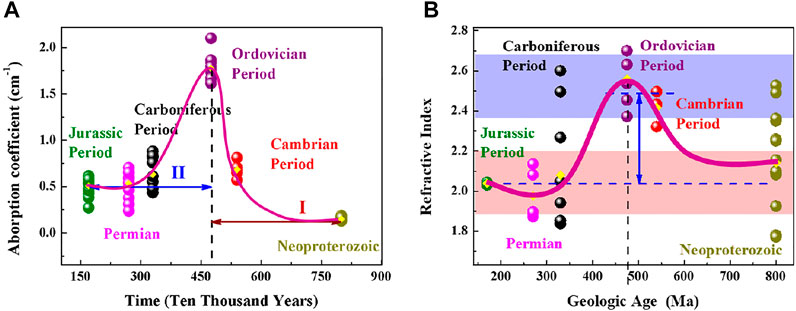
FIGURE 5. (A) Variations in the absorption coefficient with geologic age; (B) Variations in the THz refractive index with geo-logic age.
The change in the refractive indexes of all of the samples with geologic time is shown in Figure 5B. Overall, the differences in the refractive indexes of the sedimentary rock samples with different geological ages are nuancepatent differences. However, by using the average value of each age group, it was found that as the geological age increased, the refractive index initially in-creased and then decreased. The sedimentary rock samples can be divided into two stages based on their refractive indexes: 1) the Ordovician and Cambrian sedimentary rock samples, and 2) the Neoproterozoic, Carboniferous, Permian, and Jurassic sedimentary rock samples. Because the mineral composition of rock samples is basically the same, the age of rock samples can be divided and the sedimentary environment can be predicted by the different content of organic matter. Based on the sensitivity of THz wave to organic matter, it can be inferred that organic matter content and different composition types lead to significant differences in the conversion rate of samples in the Terahertz band.
In the Neoproterozoic, there was basically no life on the Earth. The Liujiang Basin was all land, and the sedimentation mode was continental deposition. Due to the absence of higher plants, there was almost no organic matter deposition in the Neoproterozoic sedimentary rocks. With the advance of time, life gradually began to appear on Earth. Therefore, organic matter deposited in the same environment will gradually increase with the enrichment of biological species. Therefore, it can be inferred that the variation of absorption coefficient of sedimentary rock samples with age is due to the influence of ocean cover.
Marine deposition mainly involves the deposition of aquatic organisms. The organic matter deposited is usually rich in lipids, proteins, and others, and the oxygen and nitrogen con-tents are relatively high. During continental sedimentation, the organic matter deposited is mainly higher plants, which are usually rich in oxygen and low in hydrogen and nitrogen. The higher the C/H ratio of the organic matter is, the higher the absorption coefficient and refractive index will be [31]. Therefore, the higher the ab-sorption coefficients and refractive indexes of the Ordovician and Cambrian sedimentary rocks will be. Therefore, it can be inferred that the Liujiang Basin was a Marine deposit in the Ordovician period. After the Ordovician period, due to further movement of the Earth’s crust, the ocean gradually withdrew from the Liujiang Basin.
During its geological evolution, the Liujiang Basin was repeatedly covered by the ocean (Figure 6). In the Neoproterozoic, there was basically no life on the Earth. The Liujiang Basin was all land, and the sedimentation mode was continental deposition. Due to the movement of the Earth’s crust, in the Cambrian the basin was covered by a shallow sea, and the deposition pattern changed from continental to neritic. The abundance of life in the oceans caused the sedimentary rocks of this period to begin to include the deposition of organic matter. Until the Ordovician, the ocean covered the Liujiang Basin, and the number of living organisms also reached an unprecedented degree. In this period, the sedimentation mode in the Liujiang Basin was deep-water marine deposition, and the organic matter was richer than that in the Cambrian. Due to further movement of the Earth’s crust, the ocean gradually withdrew from the Liujiang Basin. The sedimentation modes in the Carboniferous and Permian were marine and continental inshore ocean facies, respectively. During this period, the deposition of organic matter in the Liujiang Basin mainly involved the deposition of higher plants.
The sedimentary rocks formed in the Ordovician contain more organic matter than in other periods, while the Neoproterozoic sediments contain almost no organic matter due to the absence of living organisms at this time. This is consistent with the absorption coefficients obtained using the THz method. In the first stage, the ab-sorption increased due to the amount of organic matter. In contrast, the decrease in absorption increased in the second stage, reflecting the retreat of the sea from the Liujiang Basin. This is illustrated by the schematic diagrams of the geological evolution of the Liujiang Basin shown in Figure 5B. The absorption coefficient related to the critical turning point is in good agreement with the geo-logical evolution of the Liujiang Basin. Thus, Figure 5 indicates that the THz method can be used to investigate the geological evolution of sedimentary rocks. The sedimentary rocks formed in the Ordovician contain more organic matter than in other periods, while the Neoproterozoic sediments contain almost no organic matter due to the absence of living organisms at this time.
Marine deposition and continental deposition are two different deposition modes, and the organic matter types deposited in these modes are also different. By analyzing the absorption coefficients and refractive indexes of the samples with different ages, the evolution of the marine and continental sediments can be roughly determined. However, it is difficult to distinguish between similar continental sediments using the absorption coefficient and refractive index. To construct a more precise model of the relationship between the rocks’ parameters and THz spectra, PCA was used with Vp/d as the input. PC1, the variance of which was maximized, contains the most information according to the largest contribution rate, so it can be used to describe the importance of the PCs to the samples. PC2 contains the second most abundant information. By plotting the scores of the early PCs against each other, a two or three-dimensional score space can be obtained, in which samples that are closely related cluster together and unrelated samples plot as outliers. As is shown in Figure 7, the two-dimensional space displays the two principal components (PC1 and PC2) and their contribution rates. Specifically, the first two points in the data set were found to describe 94 and 5% of the variance within the data, with a total contribution rate of 99% to all of the deviations. As PC2 increases, the Jurassic samples are significantly different from those of the other eras. Due to its large contribution, PC1 is of great importance to analyzing the components and absorption effect. All of the samples can be arranged in order of ascending PC1 score as follows: Neoproterozoic, Permian, Cambrian, Carboniferous, and Ordovician. The Permian, Cambrian, and Carboniferous samples plot close to each other. Secondary principal component analysis of the rock samples of these three geological ages can be used for the sample division (Figure 7B). The first two points in the data set were found to describe 91.37 and 7.09% of the variance within the data, with a total contribution rate of 98.46% to all of the deviations. Consequently, the PC1 score is an effective parameter that reflects the geologic age, and the THz method is a promising supplementary tool for determining the geological ages of sedimentary rocks. In terms of the geologic ages of the sedimentary rocks, the PC1 scores based on the Vp/d can directly identify coals. The absorption coefficient can be used as an important reference for determining the geologic ages of sedimentary rocks in future research. In order to further confirm that THz technology combined with PCA method can realize the recognition of rock geological age, we carried out THz test on another batch of samples and got the results as shown in Figure 8.
As shown in Figure 8A, Mapping the change of THz response relative time delay (the time difference between sample peak time and reference peak time) of each test sample can realize geological age division of some rocks. The Permian and Jurassic samples are close to each other, secondary principal component analysis of rock samples of these three geo-logical ages can realize sample division (as shown in Figure 8B). The first two of the data set are found to describe 92.93 and 5.6% of the variance within the data, with the total contribution rate equaling 98.53% in all deviations. Consequently, the testing of samples with other geological ages Figure 8 further demonstrates that the THz method can be used to determine the geological ages of sedimentary rocks.
The more sedimentary rocks, especially the common types, are analyzed using this method, the more models can be constructed and the higher the accuracy of the models developed, which will improve the prediction of unknown strata. Based on the results in this research, more and more sedimentary rocks can be measured using the THz method, and then, the results can be combined with PCA to obtain a large database, which can be used as an important reference standard to judge sedimentary environment of sedimentary rocks.
Conclusion
In this study, sedimentary rocks with different geologic ages from the same area were investigated using THz time domain spectroscopy. The research focused on identifying that the relative geological ages of the sedimentary rocks and revealing the geological evolution of the area can be characterized using a combination of THz-TDS and PCA. Moreover, the THz absorption coefficients and refractive indexes of the rocks initially in-creased and then decreased with increasing geological age. Moreover, the Ordovician is the turning point in the absorption coefficient values. The investigation revealed that this is related to the deposition modes of the rocks. Compared with the organic matter in continental sediments, that in marine sediments has higher C/H values and higher absorption coefficients and refractive index-es. Therefore, the absorption coefficient and refractive index can reflect the type of organic matter deposited. The sedimentary modes of rocks can be inferred from the change in the absorption coefficient and the refractive index. The results of this study demonstrate that the THz dating method is a significant supplementary method for geological surveys.
Data Availability Statement
The original contributions presented in the study are included in the article/Supplementary Material, and further inquiries can be directed to the corresponding author.
Author Contributions
ZK: Conceptualization, Superviser. MX: Software. MZ: Data curation, Writing-Original draft preparation. CR: Visualization, Investigation. ZH: Writing-Reviewing and Editing.
Funding
The authors acknowledge the National Nature Science Foundation of China (No. 11804392) and the Science Foundation of China University of Petroleum, Beijing (Nos. ZX20190163, 2462020YXZZ019, and 2462020YXZZ017) for financial support.
Conflict of Interest
The authors declare that the research was conducted in the absence of any commercial or financial relationships that could be construed as a potential conflict of interest.
Publisher’s Note
All claims expressed in this article are solely those of the authors and do not necessarily represent those of their affiliated organizations, or those of the publisher, the editors, and the reviewers. Any product that may be evaluated in this article, or claim that may be made by its manufacturer, is not guaranteed or endorsed by the publisher.
Acknowledgments
We thank LetPub (www.letpub.com) for its linguistic assistance during the preparation of this article.
References
1. Shapley H. The Age of the Earth. Sci Am (1919) 87(2246):34–43. doi:10.1038/scientificamerican01181919-34supp
2. Shanan EP, Jon MH, Julia W. The Rise and Fall of Stromatolites in Shallow marine Environments. Geology (2017) 45(6):487–90.
3. Fang ZY, Qin LP, Liu W, Yao T, Chen XY, Wei SQ. Absence of Hexavalent Chromium in marine Carbonates: Implications for Chromium Isotopes as Paleoenvironment Proxy. Natl Sci Rev (2020) 0:1–8. doi:10.1093/nsr/nwaa090
4. Pang X-Q, Jia C-Z, Wang W-Y. Petroleum Geology Features and Research Developments of Hydrocarbon Accumulation in Deep Petroliferous Basins. Pet Sci (2015) 12:1–53. doi:10.1007/s12182-015-0014-0
5. Li Y, Wang C, Li Y, Ma C, Wang L, Peng S. The Cretaceous Tectonic Event in the Qiangtang Basin and its Implications for Hydrocarbon Accumulation. Pet Sci (2010) 7:466–71. doi:10.1007/s12182-010-0096-7
6. Deng Y. River-gulf System-The Major Location of marine Source Rock Formation. Pet Sci (2012) 9:281–9. doi:10.1007/s12182-012-0210-0
7. Hu D, Rao S, Wang Z-T, Hu S-B. Thermal and Maturation History for Carboniferous Source Rocks in the Junggar Basin, Northwest China: Implications for Hydrocarbon Exploration. Pet Sci (2020) 17:36–50. doi:10.1007/s12182-019-00392-2
8. Zhang F, Chen Y, Li C, Zhang J, Ma J, Li X. Geological and Geochemical Character and Genesis of the Jinlongshan-Qiuling Gold Deposits in Qinling Orogen: Metallogenic Mechanism of the Qinling-Pattern Carlin-type Gold Deposits. Sci China Ser D-earth Sci (2000) 43:95–107. doi:10.1007/bf02911936
9. Pye K, Stokes S, Neal A. Optical Dating of Aeolian Sediments from the Sefton Coast, Northwest England. Proc Geologists' Assoc (1995) 106(4):281–92. doi:10.1016/s0016-7878(08)80239-8
10. Martin D, Axel KS, Daniel FS, Oscar ML, Istvan D, Noreen JE. Application of Combined U-Th-disequilibrium/U-Pb and (U-Th)/He Zircon Dating to Tephrochronology. Quat Geochronol (2017) 40:23–32. doi:10.1016/j.quageo.2016.07.005
11. Michelle G, Spencer S, Barnes JD, Lackey JS, Stockli DF. Unraveling Histories of Hydrothermal Systems via U-Pb Laser Ablation Dating of Skarn Garnet. Earth Planet Sc Lett (2018) 498:237–46. doi:10.1016/j.epsl.2018.06.036
12. Mushkin A, Sagy A, Trabelci E, Amit R, Porat N. Measuring the Time and Scale-Dependency of Subaerial Rock Weathering Rates over Geologic Time Scales with Ground-Based Lidar. Geology (2014) 42(12):1063–6. doi:10.1130/g35866.1
13. Singhvi AK, Sharma YP, Arawa DP. Thermoluminescence Dating of Sand Dunes in Rajasthan, India. Nature (1982) 295(28):313–5. doi:10.1038/295313a0
14. Huntley DJ, Godfrey-Smith DI, Thewalt MLW. Optical Dating of Sediments. Nature (1985) 313(10):105–7. doi:10.1038/313105a0
15. Guo Y, Sun C, Luo L, Yang L, Han F, Tu H, et al. 26Al/10Be Burial Dating of the Middle Pleistocene Yiyuan Hominin Fossil Site, Shandong Province, Northern China. Sci Rep (2019) 9:6961. doi:10.1038/s41598-019-43401-5
16. Wang Y, Bai Q, Tian Z, Du H. Detrital Zircon U-Pb Dating in the Southern Hefei Basin: Evidence for Exhumation of HP-UHP Rocks of the Dabie Orogen. Sci China Earth Sci (2020) 63:954–68. doi:10.1007/s11430-019-9565-3
17. Liu Q, Wu Y-B, Wang H, Gao S, Qin Z-W, Liu X-C, et al. Zircon U-Pb Ages and Hf Isotope Compositions of Migmatites from the North Qinling Terrane and Their Geological Implications. J Meta Geol (2014) 32(2):177–93. doi:10.1111/jmg.12065
18. Bröcker M, Baldwin S, Arkudas R. The Geological Significance of 40 Ar/39 Ar and Rb-Sr white Mica Ages from Syros and Sifnos, Greece: a Record of Continuous (Re)crystallization during Exhumation? J Meta Geol (2013) 31(6):629–46. doi:10.1111/jmg.12037
19. Xu X-T, Shao L-Y, Lan B, Wang S, Hilton J, Qin J-Y, et al. Continental Chemical Weathering during the Early Cretaceous Oceanic Anoxic Event (OAE1b): a Case Study from the Fuxin Fluvio-Lacustrine Basin, Liaoning Province, NE China. J Palaeogeogr (2020) 9:12. doi:10.1186/s42501-020-00056-y
20. Yang Y, Yang M, Wang H, Yang J, Wu F. Methodology for In Situ Wolframite U-Pb Dating and its Application. Sci China Earth Sci (2021) 64:187–90. doi:10.1007/s11430-020-9676-3
21. Brian RJ, Brad SS, Li YJ. Intercalibration of 40Ar/39Ar Laborato-Ries in China, the USA and Russia for Emeishan Volcanism and the Guadalupian–Lopingian Boundary. Natl Sci Rev (2019) 6(4):614–6. doi:10.1093/nsr/nwz044
22. Ao S, Xiao W, Khalatbari Jafari M, Talebian M, Chen L, Wan B, et al. U-pb Zircon Ages, Field Geology and Geochemistry of the Kermanshah Ophiolite (Iran): From continental Rifting at 79Ma to Oceanic Core Complex at Ca. 36Ma in the Southern Neo-Tethys. Gondwana Res (2016) 31:305–18. doi:10.1016/j.gr.2015.01.014
23. Olierook HKH, Kirkland CL, Barham M, Daggitt ML, Hollis J, Hartnady M. Extracting Meaningful U-Pb Ages from Core-Rim Mixtures. Gondwana Res (2021) 92:102–12. doi:10.1016/j.gr.2020.12.021
24. Leng WX, Li QY, Bao RM, Zhao K, Miao XY, Li YZ. Characterizing the Rock Geological Time by Terahertz Spectrum. Sci China Phys Mech (2019) 62:014221. doi:10.1007/s11433-018-9229-4
25. Bao R, Qin F, Chen R, Chen S, Zhan H, Zhao K, et al. Optical Detection of Oil Bearing in Reservoir Rock: Terahertz Spectroscopy Investigation. IEEE Access (2019) 7:121755–9. doi:10.1109/access.2019.2938027
26. Zhan H, Chen M, Zhao K, Li Y, Miao X, Ye H, et al. The Mechanism of the Terahertz Spectroscopy for Oil Shale Detection. Energy (2018) 161:46–51. doi:10.1016/j.energy.2018.07.112
27. Li YZ, Wu SX, Yu XL, Bao RM, Wu ZK, Wang W, et al. Optimization of Pyrolysis Efficiency Based on Optical Property of Semicoke in Terahertz Region. Energy (2017) 126:202–7. doi:10.1016/j.energy.2017.03.020
28. Jiusheng Li J. Support Vector Regression for the Determination of the Nutritional Components of Edible Oil by Terahertz Spectroscopy. IEEE Trans Instrum Meas (2010) 59:2094–8. doi:10.1109/tim.2009.2030927
29. Baxter HW, Worrall AA, Pang J, Chen R, Yang B. Volatile Liquid Detection by Terahertz Technologies. Front Phys (2021) 9:639151. doi:10.3389/fphy.2021.639151
30. Li B, Wang R, Ma J, Xu W. Research on Crop Water Status Monitoring and Diagnosis by Terahertz Imaging. Front Phys (2020) 8:571628. doi:10.3389/fphy.2020.571628
Keywords: terahertz dating, sedimentary rocks, geologic age, absorption coefficient, refractive index, PCA
Citation: Meng Z, Zhan H, Chen R, Miao X and Zhao K (2022) Terahertz Dating of Sedimentary Rocks. Front. Phys. 10:838275. doi: 10.3389/fphy.2022.838275
Received: 17 December 2021; Accepted: 26 January 2022;
Published: 22 February 2022.
Edited by:
Yuping Yang, Minzu University of China, ChinaReviewed by:
Zhaohui Zhang, University of Science and Technology Beijing, ChinaHao Liu, China University of Geosciences, China
Copyright © 2022 Meng, Zhan, Chen, Miao and Zhao. This is an open-access article distributed under the terms of the Creative Commons Attribution License (CC BY). The use, distribution or reproduction in other forums is permitted, provided the original author(s) and the copyright owner(s) are credited and that the original publication in this journal is cited, in accordance with accepted academic practice. No use, distribution or reproduction is permitted which does not comply with these terms.
*Correspondence: Kun Zhao, zhk@cup.edu.cn