- 1Department of Optical Science and Engineering, Fudan University, Shanghai, China
- 2Jiangsu Key Laboratory of Advanced Laser Materials and Devices, School of Physics and Electronic Engineering, Jiangsu Normal University, Xuzhou, China
- 3Jiangsu Collaborative Innovation Center of Advanced Laser Technology and Emerging Industry, Xuzhou, China
We report on high power and efficient operation of a compact Ho: YAP laser in-band pumped at 1931 and 1943 nm with a high-power and narrow-linewidth Tm-doped fiber laser. Using a 0.5at. % Ho3+ doped Ho: YAP cut along the b-axis and a simple two-mirror resonator, up to 202 W of output power has been generated at ∼2118 nm for 336 W of absorbed pump power at 1931 nm, corresponding to a slope efficiency of 61.2% with respect to the absorbed pump power. The laser output is linearly polarized along the c-axis with a polarization extinction ratio (PER) of ∼20.0 dB. Results presented in this work verify the superior power scaling capability of Ho: YAP at ∼2.1 μm with high lasing efficiency.
1 Introduction
High-power solid-state lasers operating in the 2.1 μm spectral region have numerous applications in medical treatment, Lidar, material processing, and pumping the mid-infrared optical parametric oscillators [1–5]. Conventionally, laser radiation at this wavelength regime could be obtained via non-linear frequency conversion or Tm, Ho co-doped solid-state lasers pumped with commercially available high power laser diodes (LD) at ∼800 nm. Power and brightness scaling of the Tm, Ho co-doped approaches, however, would be limited by the severe thermal problems induced by the relatively low quantum efficiency, high energy transfer up-conversion (ETU) rate and the energy migrations between the Tm3+ and Ho3+ ions [6–8]. In-band pumping of Ho3+ doped solid-state lasers directly to the upper laser level (5I7 manifold) with 1.9 μm laser diodes [9, 10], Tm-doped bulk [11, 12] and fiber laser sources (TDFLs) [13, 14] has proven to be an attractive approach for power and brightness scaling of ∼2.1 μm lasers due to the inherent advantages of the low quantum defect heating (∼10%), reduced up-conversion rate and high lasing efficiencies. Moreover, the good beam quality of Tm fiber and bulk pump sources makes it possible that relatively low Ho3+ concentrations can be used with reduced ETU rates and hence improved energy storage capability in pulsed mode of operation. So far, high power and efficient laser operation of Ho3+ doped diverse gain media, such as YAG, Y2O3, YLF, LuAG and LuLiF4 etc., have been demonstrated [12–19]. Up to 142 W and 450 W of continuous-wave (CW) output power at ∼2.1 μm have been generated from Ho: YAG oscillator and amplifier with slope efficiencies of 56.7% and 62.5% [12, 13]. In-band pumping of high optical quality Ho: Y2O3 ceramic has yielded 210.5 W of output power at 2117 nm with a slope efficiency 60.0% [14]. With cryogenic cooling, pulses of 310 mJ energy and ∼80 ns duration has been generated from Q-switched Ho: YAG laser [15], and up to 550 mJ and 260 mJ of pulse energy with 14 ns and 16 ps of duration have been demonstrated from Ho: YLF oscillator and amplifier [16, 17].
Yttrium aluminum oxide (YAP), a biaxial crystal with orthorhombic symmetry and a perovskite structure, has similar thermal and mechanical properties as YAG but with lower phonon energy (∼580 cm−1) and natural birefringence favoring linearly polarized laser operation without the need of extra intracavity polarizer, and hence avoiding the unfavorable depolarization losses [20–22]. To date, laser oscillation of YAP doped with Tm, Ho, Er and Pr have been demonstrated at different wavelength region [23–25]. Up to 254 W of 1.99 μm and 26.7 W of 2.7 μm output has been generated with Tm3+ and Er3+ doping [23, 24]. Ho3+ doped YAP has broad 5I8 →5I7 absorption spectrum with peak absorptions centered at 1.917, 1.930 and 1.940–1.947 μm, allowing for more flexibility in pump source selection [26]. Efficient laser operation of Ho: YAP at ∼2.1 μm have been demonstrated with either Tm fiber or bulk (YLF, YAP) laser pumping at 1.91–1.94 μm [27–31]. In the CW mode of operation, up to 107 W of 2117 nm linearly polarized output power have been generated with a slope efficiency of 50.6% [30]. Narrow linewidth pulses of 8 mJ energy and ∼150 ns width are reported from a Q-switched and injection-seeded Ho: YAP laser in-band pumped with a Tm: YLF laser [31].
In this paper, we report on power scaling performances of Ho: YAP laser in-band pumped by a high-power and narrow-linewidth Tm fiber laser. Lasing characteristics with 1931 and 1943 nm pumping were investigated and compared, showing comparable lasing thresholds and efficiencies with respect to absorbed pump power. Using a simple two-mirror cavity, up to 202 W of linearly polarized output at 2118 nm has been generated for 336 W of absorbed pump power at 1931 nm, corresponding to a slope efficiency of 61.2%. The PER of the laser output was estimated to be ∼20 dB. This is, to the best of our knowledge, the highest laser power so far extracted from a Ho: YAP laser.
2 Experimental setup
The .5 at % Ho3+ doped YAP crystal was b-axis cut to have dimensions of 3 mm in diameter and 50 mm long with end surfaces optically polished without any coating. For efficient heat removal, the Ho: YAP crystal was directly water cooled at a temperature of 15°C. Absorption characteristics of the Ho: YAP in wavelength region of 1922 nm–1954 nm were measured using a home-made tunable and unpolarized Tm fiber laser, as shown in Figure 1. The absorbed pump power was derived by measuring the input pump power before the laser crystal and the unabsorbed pump power. To avoid ground-state bleaching and ensure the absorption measurement small signal, incident pump power was kept <100 mW (∼750 μm beam diameter). It can be seen that the crystal has a broad absorption band with absorption maxima centered at 1.93 μm and 1.94 μm. Output spectra of the Tm fiber pump laser centered at 1931 nm and 1943 nm are also shown in Figure 1 with a linewidth of <.3 nm. The corresponding absorption efficiency for the two pump wavelengths are ∼95.3% and ∼85.7%, respectively.
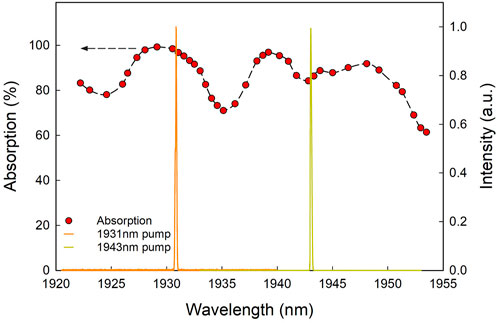
FIGURE 1. Single-pass absorption of the 0.5at. % doped Ho: YAP crystal and the pump laser spectra at 1931 and 1943 nm.
The experimental setup of the Ho: YAP laser is depicted in Figure 2. A simple two-mirror cavity was used, consisting of a plan input coupler with high reflectivity (>99.8%) at 2050–2160 nm and high transmission (>98%) at 1880–1980 nm, and a plan output coupler (OC) with transmissivity of TOC = 50% at 2050–2160 nm and high transmission (>95%) at the pump wavelength. The pump source is an in-house constructed beam-combined high power Tm fiber master oscillator power amplifier (MOPA). A single-mode narrow linewidth (∼.25 nm) Tm fiber oscillator at 1931 or 1943 nm was separated by a 1 × 3 coupler for seeding three two-stage amplifiers. Outputs form the fiber amplifiers were then power combined using a 3 × 1 signal combiner of 100 μm diameter core (NA = .22), producing an overall pump power of ∼800 W with a beam quality factor M2 of ∼6.2. The combined beam was collimated and focused into the Ho: YAP crystal by a plano-convex lens assembly of 20 mm and 150 mm focal length, giving a pump beam diameter of ∼750 μm. The focus of the pump light was located roughly at the center of crystal and the corresponding confocal parameter was estimated to be ∼140 mm. Both cavity mirrors were positioned as close as possible to the laser crystal, forming a relatively compact resonator of ∼56 mm cavity length. A pair of 45° dichroic mirrors (R > 99.8% at 2050–2160 nm, R < 5% at 1880–1950 nm) were utilized to separate the laser and the residual pump light. The resonator optical axis was positioned approximately ∼4° away to the pump incident direction to prevent any feedback into the TDFL pump source. Laser output power was recorded using a thermal-sensor power meter (Ophir, FL250A-BB50-PPS) and laser spectrum was monitored by an optical spectrum analyzer (AQ6375B, Yokogawa) with a resolution of .05 nm. The beam quality factor, M2, was analyzed via a beam profiler (NanoScan, Photon Inc.)
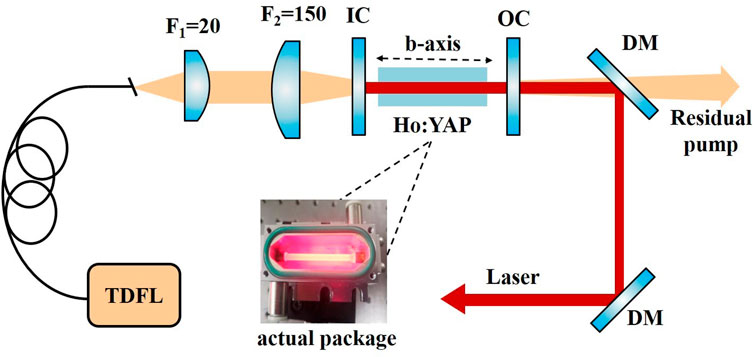
FIGURE 2. Experimental setup of the Ho, YAP laser pumped with a Tm fiber laser. IC, input coupler; OC, output coupler; DM, dichroic mirror. Inset, actual package image of cooling system.
3 Experimental results and discussion
Figure 3 shows output power as a function of absorbed pump power at 1931 and 1943 nm. The absorbed pump power under lasing condition was estimated according to the incident pump power and unabsorbed pump power measured behind a pair of 45° dichroic mirrors. The absorption efficiency at 1931 nm and 1943 nm was thus estimated to be 92.0% and 80.0%, respectively. The laser reached threshold at a pump power of 7.13 W and 7.75 W, and increased linearly to 84.8 W and 85.2 W for an absorbed pump power of 148.3 W at 1931 and 145.1 W at 1943 nm, corresponding to a slope efficiency of 61.5% and 63.0%, respectively. It can be seen that comparable lasing thresholds and slope efficiencies are exhibited for either 1931 nm or 1943 nm pumping. And not surprisingly, pump absorption under lasing condition shows negligible ground-state bleaching up to even the highest pump power level due to the fact that population inversion nearly clamped once the laser get lasing.
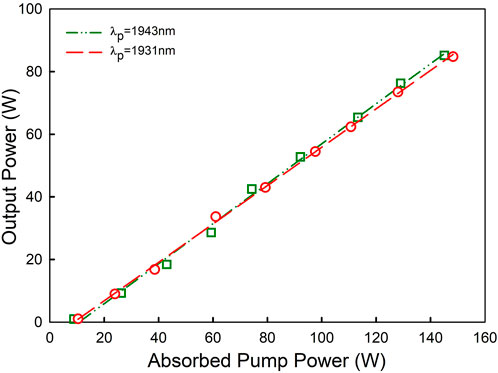
FIGURE 3. Output power of the Ho, YAP laser versus the absorbed pump power pumped at 1931 and 1943 nm.
Power scaling characteristics of the Ho: YAP laser was further investigated with 1931 nm pumping, which allows for more efficient pump absorption. Output power as a function of absorbed pump power is plotted in Figure 4. The laser output power increased linearly to 202 W for an absorbed pump power of 336 W, corresponding to a slope efficiency of 61.2%. This should represent, to the best of our knowledge, the highest power so far reported from a Ho: YAP laser at ∼2.1 µm. It is noteworthy that the output power obtained in this experiment is limited only by the available incident pump power, further attempt in power scaling is hindered by the thermal induced fracture of the focusing lens. A typical output spectrum of the Ho: YAP laser is shown in the inset of Figure 4, characterized with multi-peak structure and centered at ∼2118.1 nm with a wavelength span of ∼2.4 nm.
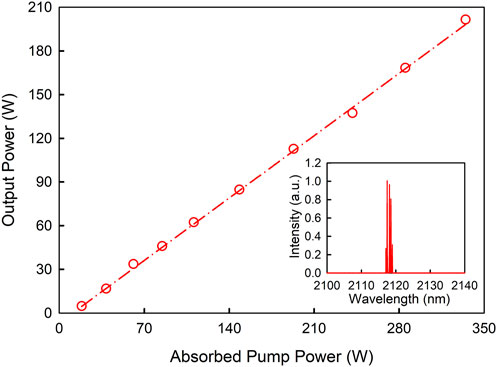
FIGURE 4. Output power as a function of the absorbed pump power at 1931 nm. Inset, typical output spectrum of the Ho, YAP laser.
Figure 5 shows the absorption efficiency of Ho: YAP at 1931 nm under both lasing and non-lasing conditions. One can see that the measured absorption curves exhibit typical absorption characteristics of in-band pumped quasi-four-level laser system. Single-pass absorption under non-lasing condition is ∼96.8% for .1 W of incident pump power, and dropped to ∼54.3% for 365 W of incident pump due to severe ground state bleaching. Absorption efficiency under lasing condition, however, exhibits only slight reduction from 92.3% at just above threshold to 86.3% for 388 W of incident pump. This is because the fact that the population inversion tends to clamped at the threshold of the laser.
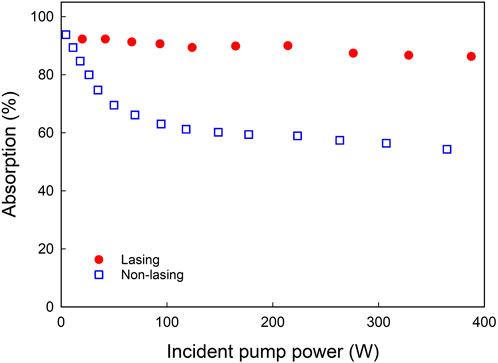
FIGURE 5. Sing pass absorption of the Ho: YAP under both lasing and non-lasing conditions versus incident pump power at 1931 nm.
Polarization state of the laser output was analyzed using a Glan Prism Polarizer. Benefitting from the inherent birefringence of YAP, linearly polarized operation along c-axis of the crystal was confirmed with a PER of ∼20.6 dB at 5 W and ∼19.8 dB at 100 W of output power, as depicted in Figure 6A. This suggests that Ho: YAP should be especially attractive for construction of high power and linearly polarized laser sources at 2.1 μm without the need of extra intracavity polarizers, which eliminates the detrimental depolarization losses and ensure high lasing efficiencies.
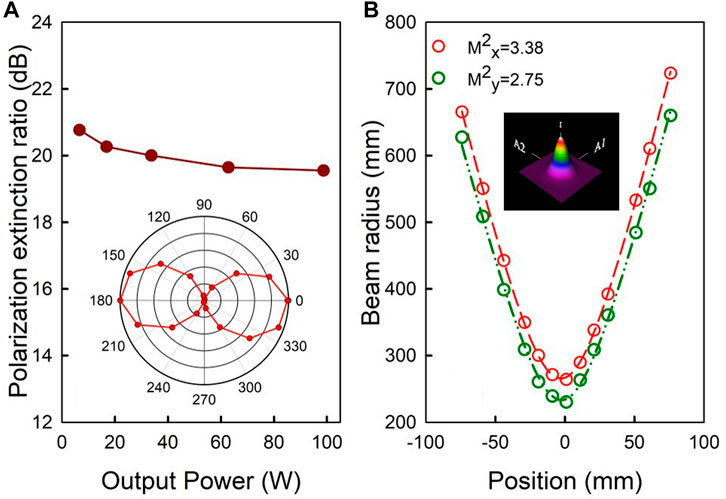
FIGURE 6. (A) The polarization extinction ratio of the Ho: YAP laser as a function of output power. Inset is the polarization measurement of laser beam (B) The beam quality factor of the Ho: YAP laser at ∼100 W of output power. Inset shows the 3-D transverse beam profile.
With a plano-convex lens of 100 mm focal length placed behind the output coupler to focus the output beam onto a beam profiler, the beam quality factor, M2, of the Ho: YAP laser at ∼100 W of output power was estimated to be 3.38 and 2.75 in the horizontal and vertical direction, as shown in Figure 6B. The beam quality difference in the orthogonal directions could be attributed to the slight difference of cooling efficiency at high power levels in the two directions. The inset of Figure 6B shows the near-field 3-D profile of the laser beam (∼100 W) with a near-Gaussian intensity distribution.
4 Conclusion
In summary, we report on a high power and efficient Ho: YAP laser in-band pumped by a high-power and narrow-linewidth Tm-doped fiber laser at 1931 and 1943 nm. Using a simple two-mirror resonator, the laser generated a maximum output power of 202 W for 336 W of absorbed pump power pumped at 1931 nm, corresponding to a slope efficiency of 61.2%. This, to the best of our knowledge, should represent the highest power so far obtained from a Ho: YAP laser at ∼2.1 µm. Owing to the inherent birefringence of YAP, the laser output is linearly polarized with a polarization extinction ratio of ∼20 dB. The results presented in this work confirms the great potential of Ho: YAP in generation of high power and high energy 2.1 µm lasers.
Data availability statement
The raw data supporting the conclusions of this article will be made available by the authors, without undue reservation.
Author contributions
All authors listed have made a substantial, direct, and intellectual contribution to the work and approved it for publication.
Funding
This work was supported by the National Natural Science Foundation of China (62035007).
Conflict of interest
The authors declare that the research was conducted in the absence of any commercial or financial relationships that could be construed as a potential conflict of interest.
Publisher’s note
All claims expressed in this article are solely those of the authors and do not necessarily represent those of their affiliated organizations, or those of the publisher, the editors and the reviewers. Any product that may be evaluated in this article, or claim that may be made by its manufacturer, is not guaranteed or endorsed by the publisher.
References
1. Rieken M, Bachmann A. Laser treatment of benign prostate enlargement-which laser for which prostate? Nat Rev Urol (2014) 11(3):142–52. doi:10.1038/nrurol.2014.23
2. Kohei M, Shoken I, Makoto A, Hironori I, Ryohei O, Hirotake F, et al. 2 μm Doppler wind lidar with a Tm:fiber-laser pumped Ho:YLF laser. Opt Lett (2018) 43(2):202–5. doi:10.1364/ol.43.000202
3. Mingareev I, Weirauch F, Olowinsky A, Shah L, Kadwani P, Richardson M. Welding of polymers using a 2μm thulium fiber laserm thulium fiber laser. Opt Laser Technol (2012) 44(7):2095–9. doi:10.1016/j.optlastec.2012.03.020μ
4. Liu G, Mi SY, Yang K, Wei DS, Yao BQ, Yang C, et al. 161 W middle infrared ZnGeP2 MOPA system pumped by 300 W-class Ho:YAG MOPA system. Opt Lett (2020) 46(1):82–5. doi:10.1364/ol.413755
5. Sanchez D, Hemmer M, Baudisch M, Cousin SL, Zawilski K, Schunemann P, et al. 7 μm, ultrafast, sub-millijoule-level mid-infrared optical parametric chirped pulse amplifier pumped at 2 μm. Optica (2016) 3(2):147–50. doi:10.1364/optica.3.000147
6. Rustad G, Stenersen K. Modeling of laser-pumped Tm and Ho lasers accounting for upconversion and ground-state depletion. IEEE J Quan Electron (1996) 32(9):1645–56. doi:10.1109/3.535370
7. French VA, Petrin RR, Powell RC, Kokta M. Energy-transfer processes in Y3Al5O12:Tm, Ho. Phys Rev B (1992) 46:8018–26. doi:10.1103/physrevb.46.8018
8. Loiko P, Kifle E, Brasse G, Thouroude R, Starecki F, Benayad A, et al. In-band pumped Tm, Ho:LiYF4 waveguide laser. Opt Express (2022) 30(7):11840–7. doi:10.1364/oe.449126
9. Lamrini S, Koopmann P, Schäfer M, Scholle K, Fuhrberg P. Efficient high-power Ho:YAG laser directly in-band pumped by a GaSb-based laser diode stack at 1.9 µm. Appl Phys B (2012) 106(2):315–9. doi:10.1007/s00340-011-4670-5
10. Ji E, Liu Q, Nie MM, Cao XZ, Fu X, Gong ML. High-slope-efficiency 2.06 μm Ho: YLF laser in-band pumped by a fiber-coupled broadband diode. Opt Lett (2016) 41(6):1237–40. doi:10.1364/ol.41.001237
11. Wang QC, Long QL, Gao Y, Xue JY, Xu ZP, Mao YF, et al. High-efficiency Ho:YLF slab laser with 125 W continuous-wave output power. Appl Opt (2021) 60(26):8046–9. doi:10.1364/ao.434708
12. Mi SY, Tang JW, Wei DS, Yao BQ, Li JH, Yang K, et al. Thermal-birefringence-induced depolarization in a 450 W Ho:YAG MOPA system. Opt Express (2022) 30(12):21501–11. doi:10.1364/oe.462617
13. Yao WC, Li EH, Shen YJ, Ren CY, Zhao YG, Tang DY, et al. A 142 W Ho:YAG laser single-end-pumped by a Tm-doped fiber laser at 1931 nm. Laser Phys Lett (2019) 16(11):115001. doi:10.1088/1612-202x/ab459d
14. Ren CY, Huang WW, Xie HB, Wang F, Shen DY, Wang J, et al. High power and efficient operation of a Ho:Y2O3 ceramic laser with over 210 W of output power at 2.1 µm. Opt Express (2022) 30(17):31407–14. doi:10.1364/oe.468211
15. Ganija M, Boyd K, Hemming A, Carmody N, Simakov N, Veitch P, Munch J. High energy cryogenically cooled Ho:YAG oscillator. In: Conference on Lasers and Electro-Optics Europe and European Quantum Electronics Conference; 21-25 June 2021 (2021). 1.
16. Fonnum H, Lippert E, Haakestad MW. 550 mJ Q-switched cryogenic Ho:YLF oscillator pumped with a 100 W Tm:fiber laser. Opt Lett (2013) 38(11):1884–6. doi:10.1364/ol.38.001884
17. Elu U, Steinle T, Sánchez D, Maidment L, Zawilski K, Schunemann P, et al. Table-top high-energy 7 μm OPCPA and 260 mJ Ho:YLF pump laser. Opt Lett (2019) 44(13):3194–7. doi:10.1364/ol.44.003194
18. Liu J, Song Q, Wang Z, Wang Y, Dong J, Xu J, et al. Ho:LuAG single crystal fiber: Growth, spectroscopy and laser characteristics. Opt Express (2022) 30(4):5826–34. doi:10.1364/oe.449790
19. Kim JW, Mackenzie JI, Parisi D, Veronesi S, Tonelli M, Clarkson WA. Efficient in-band pumped Ho:LuLiF4 2µm laser. Opt Lett (2010) 35(3):420–2. doi:10.1364/ol.35.000420
20. Dong Q, Zhao G, Chen J, Ding Y, Zhao C. Growth and anisotropic thermal properties of biaxial Ho:YAlO3 crystal. J Appl Phys (2010) 108(2):023108. doi:10.1063/1.3460645
21. Lauer HV, Fong FK. Coupling strength in the theory of radiationless transitions: f → f and d → f relaxation of rare-earth ions in YAlO3 and Y3Al5O12. J Chem Phys (1974) 60(1):274–80. doi:10.1063/1.1680780
22. Weber MJ, Bass M, Andringa K, Monchamp RR, Comperchio E. Czochralski growth and properties of YAlO3 laser crystal. Appl Phys Lett (1969) 15(10):342–5. doi:10.1063/1.1652851
23. Mao YF, Gao Y, Wang L. 254 W laser-diode dual-end-pumped Tm:YAP InnoSlab laser. Appl Opt (2020) 59(27):8224–7. doi:10.1364/ao.399341
24. Quan C, Sun DL, Zhang HL, Luo JQ, Hu LZ, Han ZY, et al. 13-W and 1000-Hz of a 2.7-µm laser on the 968 nm LD side-pumped Er:YAP crystal with concave end-faces. Opt Express (2021) 29(14):21655–63. doi:10.1364/oe.428874
25. Fibrich M, Šulc J, JelínkováPr H. YAlO3 laser performance enhancement by operating the laser close to liquid helium temperatures. Opt Laser Technol (2022) 148:107653. doi:10.1016/j.optlastec.2021.107653
26. Payne SA, Chase LL, Smith LK, Kway WL, Krupke W. Infrared cross-section measurements for crystals doped with Er3+, Tm3+, and Ho3+. IEEE J Quan Electron (1992) 28(11):2619–30. doi:10.1109/3.161321
27. Yao BQ, Duan XM, Zheng LL, Jun YL, Wang YZ, Zhao GJ, et al. Continuous-wave and Q-switched operation of a resonantly pumped Ho:YAlO3 laser. Opt Express (2008) 16(19):14668–74. doi:10.1364/oe.16.014668
28. Šulca J, Němeca M, Jelíneka M, Jelínkováa H, Kubečeka V, Nejezchlebb K, et al. Anisotropy of spectroscopic and laser properties of Ho:YAP crystal. Proc SPIE (2022) 119800:119800D.
29. Yu T, Ye XS, Yang ZG, Chen XL, Xia WB, Zhang JY, et al. All fiber thulium-doped fiber laser pumped Q-switched Ho: YAP laser. Proc SPIE (2017) 10173:10173M.
30. Tang JW, Li EH, Wang F, Yao WC, Shen CF, Shen DY. High power Ho:YAP laser with 107 W of output power at 2117 nm. IEEE Photon J (2020) 12(2):1–7. doi:10.1109/jphot.2020.2968745
Keywords: high power, solid-state laser, 2.1 μm spectral region, Ho: YAP laser, in-band pumping
Citation: Ren C, Ma K, Wang F, Shen D and Zhu H (2023) Highly efficient Ho: YAP laser with 202 W of linearly polarized output power at 2118 nm. Front. Phys. 10:1117958. doi: 10.3389/fphy.2022.1117958
Received: 07 December 2022; Accepted: 28 December 2022;
Published: 10 January 2023.
Edited by:
Houkun Liang, Sichuan University, ChinaReviewed by:
Haiyong Zhu, Wenzhou University, ChinaZhenxu Bai, Hebei University of Technology, China
Copyright © 2023 Ren, Ma, Wang, Shen and Zhu. This is an open-access article distributed under the terms of the Creative Commons Attribution License (CC BY). The use, distribution or reproduction in other forums is permitted, provided the original author(s) and the copyright owner(s) are credited and that the original publication in this journal is cited, in accordance with accepted academic practice. No use, distribution or reproduction is permitted which does not comply with these terms.
*Correspondence: Deyuan Shen, mrde@jsnu.edu.cn