- 1Aerospace Information Research Institute, Chinese Academy of Sciences, Beijing, China
- 2College of Optoelectronics, University of Chinese Academy of Sciences, Beijing, China
- 3Shanghai Astronomical Observatory, Chinese Academy of Sciences, Shanghai, China
Picosecond pulse laser is the main light source for satellite laser ranging. In this paper, a 10 kHz repetition rate picosecond green laser with an average output power of 5.3 W is demonstrated. The laser generates a pulse width of 18.6 ps at a center wavelength of 532.20 nm with a spectral width of .066 nm. The beam quality is well preserved with M2 of 1.1 with the beam divergence measured to be .62 mrad and pointing stability of 7 μrad over 30 min of operation. The laser system was then applied to measure the BeiDou satellite (Compass-I3) and generated a single range accuracy of 3.2 mm, which is the highest reported range accuracy for synchronous orbit satellite laser ranging.
Introduction
Satellite laser ranging (SLR) which uses a laser to detect the variation of a satellite from its predicted orbit is the most accurate satellite ranging technique [1–3]. With laser as the core light source, SLR uses the time-of-flight (TOF) measurement method for ranging [4, 5]. Generally, a laser with narrower pulse width contributes to higher ranging accuracy; meanwhile, a higher repetition rate leads to a higher probability of range echoes. At present, along with the technological breakthrough of kilohertz picosecond lasers, lasers with high repetition rate are rapidly becoming the landmark light source for fourth-generation satellite laser ranging [6–11]. Mode-locking technology is the main approach to achieve picosecond pulses, but the energy of the ultrashort pulses generated by mode-locked is low (∼nanojoule) [12, 13]. In order to increase the pulse energy while maintaining the narrow pulse width, laser amplifiers (viz. regenerative amplifier and traveling wave amplifiers) are usually used to amplify the pulses output from picosecond oscillators [14–17]. Regenerative amplifiers have been widely applied to amplify the picosecond pulses from the nanojoule level to the millijoule level with a peak power enhancement of 106 times [18, 19].
In this letter, a picosecond green laser with a high repetition rate, high beam quality, high pointing stability and high-power stability is presented. In this system, a regenerative amplifier is used to increase the single pulse energy while keeping the pulse width constant. Then, the energy is further amplified using a traveling wave amplifier. Finally, the green laser output is achieved by second harmonic generation (SHG) with a conversion efficiency of up to 81.5%. The laser generates 10 kHz repetition rate picosecond pulses with an average output power of 5.3 W and pulse width of 18.6 ps. The center wavelength of the laser is 532.20 nm with a spectral width of .066 nm. It has a beam divergence angle of .62 mrad and a beam quality factor (M2) of 1.1. The peak-to-peak energy fluctuations of the laser are 1.32% at .5 h.
Experiment and results
The experimental setup of the green picosecond laser is shown in Figure 1, which mainly consists of a picosecond seed source module, a regenerative amplifier module, a power amplifier module, and an electric control module. The seed source outputs 1,064 nm ps pulses with a pulse energy of 2 nJ and a pulse width of 20 ps at a repetition rate of 84 MHz. The seed passes through the optical isolator and then enters the regenerative amplifier. The optical isolator which is to prevent the amplified laser from returning and causing damage to the picosecond seed source consists of a polarizer, a half-wave plate, and a Faraday rotator. The regenerative amplification module consists of a polarizer, a quarter-wave plate, a gain medium, an electro-optical switch—Pockels cells (PC), a pump source, and a highly reflective mirror. The seed light pulse energy is amplified to .32 mJ in the regenerative amplification module with a repetition rate of 10 kHz. In the regenerative amplification module, the gain medium uses .3 at % doped Nd: YVO4 crystal, which has a large excited emission cross-section and a wide pumping bandwidth. And the laser output is linearly polarized due to its natural birefringence property, which can mitigate thermogenic birefringence [20]. By using a semiconductor laser to end-pump the gain medium, a better mode matching of the pump light to the signal light is achieved and a better beam quality is obtained. The in-band pumping method is used to excite the particles directly to the upper energy level of the laser (4I9/2→4F3/2), which can avoid the non-radiative leap process (4F5/2→4F3/2), reduce the quantum loss, reduce the thermal effect, and improve the beam quality and stability [21, 22]. The size of Nd:YVO4 is 3 mm × 3 mm × 20 mm. The pump light with a power of 25 W is injected into the Nd: YVO4 crystal after being collimated and focused by a coupling lens set.
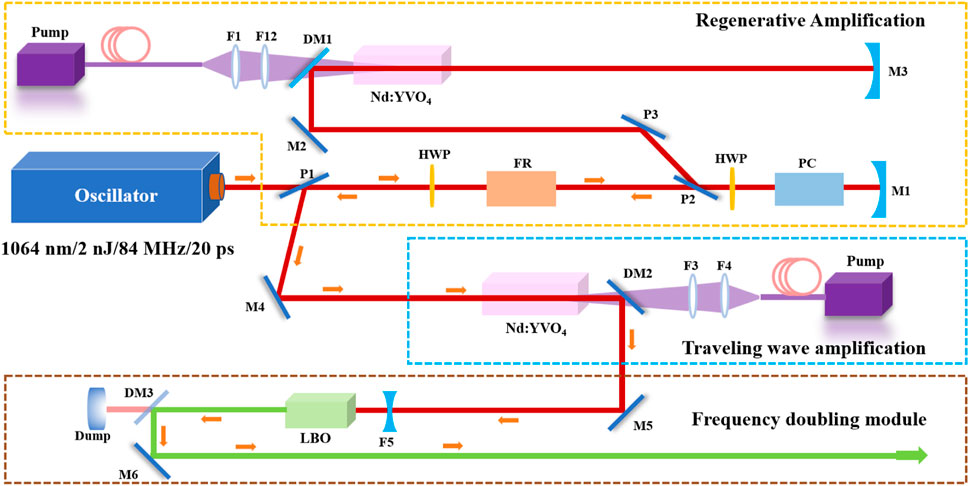
FIGURE 1. Schematic showing the layout of the laser system. PC, BBO crystal Pockels cell; HWP, half-wave plate; FR, Faraday rotator; M, mirrors; F, focus lens; P, Brewster angle polarizer.
The seed light is output from the regenerative amplifier module and then enters the traveling wave amplifier module, which consists of a pump source, a gain medium, and a highly reflective mirror. Similar to the regenerative amplification module, the gain medium of the traveling wave amplification module is selected as .5 at % doped Nd: YVO4 crystal with a size of 4 mm × 4 mm × 32 mm, using the same pumping geometry. The pump power is 90 W. After the signal light is amplified by the traveling wave module, the pulse energy is amplified to .65 mJ and the repetition rate is 10 kHz. The signal light amplified by the traveling wave amplifier module enters the frequency doubling module. It consists of a lens, a frequency doubling crystal, a dichroic mirror, and a highly reflective mirror. The frequency doubling crystal is selected as LBO crystal, which has a wide transmission range, good optical uniformity, high damage threshold, good mechanical properties, and high frequency doubling conversion efficiency [23, 24]. LBO adopts a combination of angular phase matching and temperature phase matching. The LBO is cut at θ = 90° and φ = 10.6° with dimensions of 4 mm × 4 mm × 12 mm. In the experiment, the LBO achieves the best matching at around 50°C. This matching method can avoid the system disturbance caused by the high local temperature of the system, and at the same time suppress the micro-dampness of the crystal to improve the service life.
The amplified pulse is focused by the lens and then enters the LBO crystal for frequency doubling. The frequency doubled light is separated from the fundamental frequency light at the dichroic mirror, and the fundamental frequency light is output at the dichroic mirror and collected by the collector, while the frequency doubled light is reflected by the dichroic mirror and then reflected and output by the high reflector. After the frequency doubling crystal, the pulse energy was converted from .65 to .53 mJ, and the frequency doubling efficiency was 81.5%. The central wavelength of green light is 532.20 nm, with a single pulse energy of .53 mJ, a repetition rate of 10 kHz, and a pulse width of 18.6 ps.
The encapsulated laser is shown in Figure 2A. The output laser parameters are measured. The spectrum of the output laser is measured by using a spectrometer (AQ6374 OPTICAL SPECTRUM ANALYZER) as shown in Figure 2B. The central wavelength of the output laser is 532.20 nm and the linewidth is .066 nm. The pulse width of the output laser is measured by using an autocorrelator as shown in Figure 2C. The pulse width of the output laser is about 18.6 ps. The repetition rate of the output laser is measured by using an oscilloscope, as shown in Figure 2D. The beam quality of the output laser is measured by using a beam quality analyzer, as shown in Figure 2E. The beam quality factor of the output laser is Mx2 = 1.09, My2 = 1.12, and the beam divergence angle is about .62 mrad. The laser has good power stability (.11% at 2 h) and energy stability (Pulse Energy Peak—Peak Fluctuation 1.32% at .5 h). Output laser pointing stability is 7 μrad at .5 h.
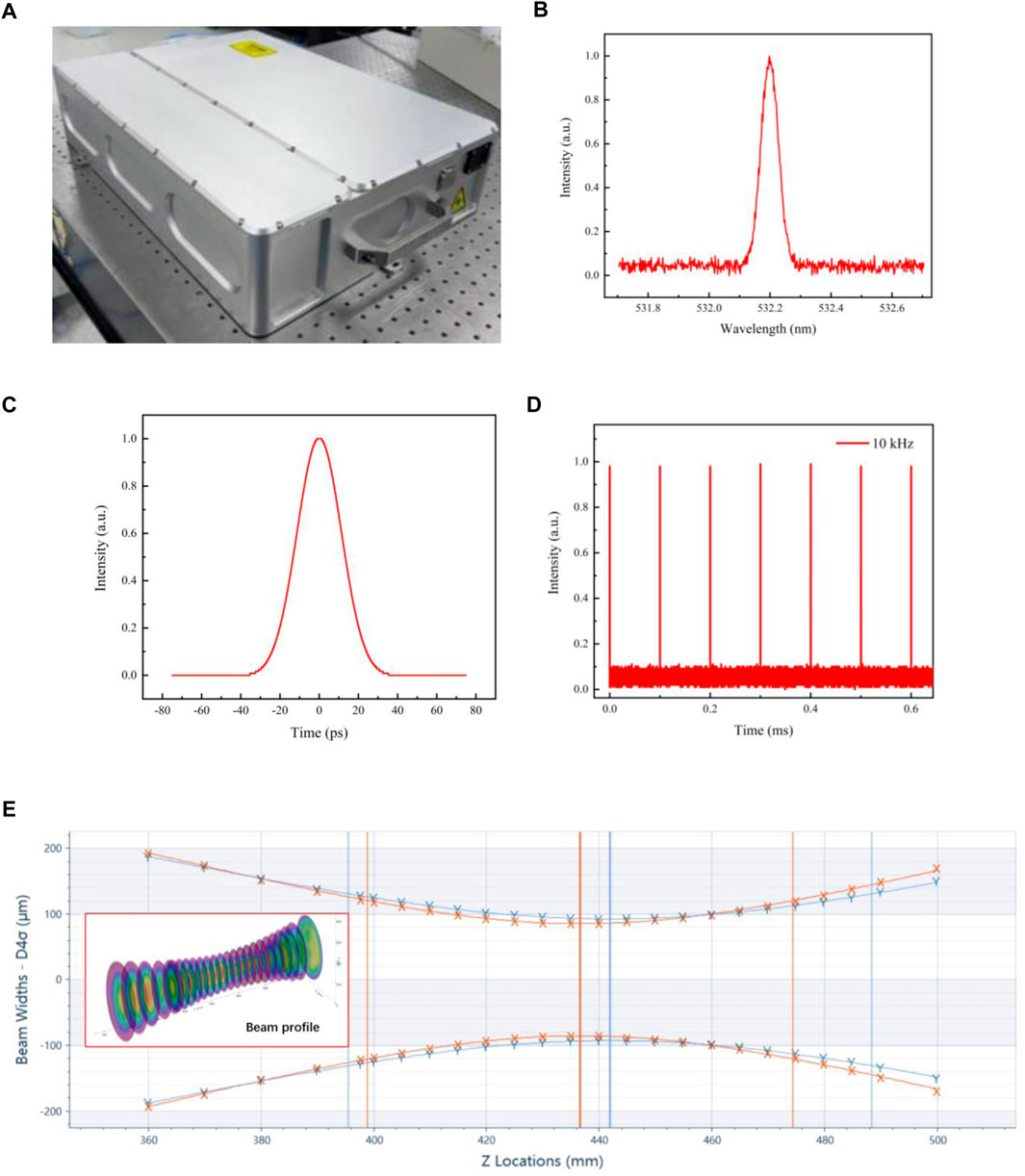
FIGURE 2. Compilation of output characteristics of the green laser. (A) Encapsulated lasers; (B) Normalized wavelength spectrum of the amplified laser output; (C) Characteristic of a single output pulse with an autocorrelation trace; (D) Normalized pulse characteristics of the output (E) plot of the focusing characteristics of the output beam (inset: beam profile).
According to the above experimental results, the green picosecond laser output laser has a narrow pulse width, which can improve the measurement accuracy of a single pulse; high energy stability, which can reduce the main wave timing jitter and further improve the measurement accuracy; narrower spectral width can better match the narrow band filter and improve the reception efficiency; high repetition rate of 10 kHz can obtain more effective data and reduce the fitting error. In summary, the laser can effectively improve the satellite laser ranging accuracy.
Applied to the satellite laser ranging system of Shanghai Astronomical Observatory, the Compass-I3 satellite in geosynchronous orbit (36,000 km) was measured, as shown in Figure 3, and a single ranging accuracy of 3.2 mm was obtained. To the best of our knowledge, this is the highest SLR accuracy obtained in synchronous orbit.
Conclusion
In this paper, a green picosecond laser with high beam quality, high pointing stability and high power stability is demonstrated. The final output laser has a power of 5.3 W, a central wavelength of 532.20 nm, a spectral width of .066 nm, a pulse width of 18.6 ps, and a repetition rate of 10 kHz. The laser has high beam quality and repetition rate, as well as very high power, energy, and pointing stability, and was applied to a satellite laser ranging system to measure the Compass-I3 satellite in geosynchronous orbit (36,000 km). The final single range accuracy of 3.2 mm was obtained, which is the highest range accuracy we know of for laser ranging of satellites in synchronous orbit. This research provides a high-quality laser source for high-precision space detection.
Data availability statement
The original contributions presented in the study are included in the article/supplementary material, further inquiries can be directed to the corresponding authors.
Author contributions
ZF, conceptualization, writing-original draft preparation, supervision, and funding acquisition. XL, investigation, formal analysis, writing-review, and editing. ZZ, formal analysis, investigation, writing—review, and editing. WM, visualization and writing-original draft. ML, formal analysis, investigation, and validation. ZB, conceptualization, formal analysis, writing-review and editing, and funding acquisition. All authors have read and agreed to the published version of the manuscript.
Funding
This work was supported by the Research Group of Short Pulse Laser Technology (GJJSTD20200009), and Guangdong Key Research and Development Program (2018B090904003).
Conflict of interest
The authors declare that the research was conducted in the absence of any commercial or financial relationships that could be construed as a potential conflict of interest.
Publisher’s note
All claims expressed in this article are solely those of the authors and do not necessarily represent those of their affiliated organizations, or those of the publisher, the editors and the reviewers. Any product that may be evaluated in this article, or claim that may be made by its manufacturer, is not guaranteed or endorsed by the publisher.
References
1. Lucchesi DM, Anselmo L, Bassan M, Pardini C, Peron R, Pucacco G, et al. Testing the gravitational interaction in the field of the Earth via satellite laser ranging and the Laser Ranged Satellites Experiment (LARASE). Classical Quan Gravity (2015) 32(15):155012. doi:10.1088/0264-9381/32/15/155012
2. Wilkinson M, Schreiber U, Procházka I, Moore C, Degnan J, Kirchner G, et al. The next generation of satellite laser ranging systems. J Geodesy (2019) 93(11):2227–47. doi:10.1007/s00190-018-1196-1
3. Long M, Zhang H, Yu RZ, Wu Z, Qin S, Zhang Z. Satellite laser ranging at ultra-high PRF of hundreds of kilohertz all day. Front Phys (2022) 10:1036346. doi:10.3389/fphy.2022.1036346
4. Degnan J, Jan M. SLR2000: Eyesafe and autonomous single photoelectron satellite laser ranging at kilohertz rates. Proc SPIE (1997) 3218:63–77.
5. Lucchesi D, Anselmo L, Bassan M, Magnafico C, Pardini C, Peron R, et al. General relativity measurements in the field of earth with laser-ranged satellites: State of the art and perspectives. Universe (2019) 5(6):141. doi:10.3390/universe5060141
6. Deng H, Zhang H, Long M, Wu Z, Tang K, Zhang Z. 4 kHz repetition rate satellite laser ranging system and its application. Acta Optica Sinica (2019) 39(03):0314002. (in Chinese). doi:10.3788/aos201939.0314002
7. Sung K-P, Choi E-J, Lim H-C, Jung CG, Kim IY. Development of operation software for high repetition rate satellite laser ranging. J Korean Soc Aeronaut Space Sci (2016) 44(12):1103–11. doi:10.5139/jksas.2016.44.12.1103
8. Wu Z, Zhang Z, Zhang H, Li P, Meng W, Chen J. The preliminary results of SLR with 10 kHz laser system at Shanghai station[C]//International workshop on laser ranging. Japan: Fujiyoshida (2013).
9. Gen R, Wu Z, Tang K, Li Y, Meng W, Zhang Z Precise control of laser emission epoch in laser time transfer with high repetition rate. Infrared Laser Eng (2021) 50(10):135–43.
10. Long M, Zhang H, Men L, Wu Z, Deng H, Qin S, et al. Satellite laser ranging at high-repetition 10 kHz in all day. Infrared Millim Waves (2020) 39(6):778–85.
11. Bai Z, Chen H, Gao X, Li S, Qi Y, Bai Z. Highly compact nanosecond laser for space debris tracking. Opt Mater (2019) 98:109470. doi:10.1016/j.optmat.2019.109470
12. Keller U, Weingarten KJ, Kartner FX, Kopf D, Braun B, Jung I, et al. Semiconductor saturable absorber mirrors (SESAM's) for femtosecond to nanosecond pulse generation in solid-state lasers. IEEE J Selected Top Quan Electron (1996) 2(3):435–53. doi:10.1109/2944.571743
13. Gong M, Yu H, Wushouer X, Yan P. Passively mode-locked Nd: YVO4 picosecond laser with oblique incidence on SESAM. Laser Phys Lett (2008) 5(7):514–7. doi:10.1002/lapl.200810025
14. Kim GH, Yang JH, Lee DS, Kulik AV, Sall EG, Chizhov SA, et al. Femtosecond laser based on Yb:KYW crystals with suppression of spectral narrowing in a regenerative amplifier by spectral profiling of the pulse. J Opt Technol (2013) 80(3):142–7. doi:10.1364/jot.80.000142
15. Kleinbauer J, Knappe R, Wallenstein R. 13-W picosecond Nd:GdVO4 regenerative amplifier with 200-kHz repetition rate. Appl Phys B (2005) 81(2):163–6. doi:10.1007/s00340-005-1903-5
16. Hong KH, Gopinath JT, Rand D, Siddiqui AM, Huang SW, Li E, et al. High-energy, kHz-repetition-rate, ps cryogenic Yb:YAG chirped-pulse amplifier. Opt Lett (2010) 35(11):1752–4. doi:10.1364/ol.35.001752
17. Li K, Wang Y, Yu Y, Yue J, Song C, Cao C, et al. Amplification of high repetition-rate, picosecond laser pulses using a zig-zag slab configuration. Opt Laser Techn (2023) 157:108717. doi:10.1016/j.optlastec.2022.108717
18. Bai Z, Bai Z, Kang Z, Lian F, Lin W, Fan Z. Non-pulse-leakage 100-kHz level, high beam quality industrial grade Nd: YVO4 picosecond amplifier. Appl Sci (2017) 7(6):615. doi:10.3390/app7060615
19. Bai Z, Bai Z, Yang C, Chen L, Chen M, Li G. High pulse energy, high repetition picosecond chirped-multi-pulse regenerative amplifier laser. Opt Laser Techn (2013) 46:25–8. doi:10.1016/j.optlastec.2012.04.019
21. McDonagh L, Wallenstein R, Nebel A. 111 W, 110 MHz repetition-rate, passively mode-locked TEM00Nd:YVO4 master oscillator power amplifier pumped at 888 nm. Opt Lett (2007) 32:1259–61. doi:10.1364/ol.32.001259
22. Bai ZA, Fan ZW, Bai ZX, Lian FQ, Kang ZJ, Lin WR. Optical fiber pumped high repetition rate and high power Nd: YVO4 picosecond regenerative amplifier. Appl Sci (2015) 5:359–66. doi:10.3390/app5030359
23. Chen CT, Wu YC, Jing A, Wu B, You G, Li R, et al. New nonlinear-optical crystal: LiB3O5. J Opt Soc Am B (1989) 6:616–21. doi:10.1364/josab.6.000616
Keywords: picosecond, regenerative amplifier, second harmonic generation, green laser, satellite laser ranging
Citation: Fan Z, Liu X, Zhang Z, Meng W, Long M and Bai Z (2023) 10 kHz repetition rate picosecond green laser for high-accuracy satellite ranging. Front. Phys. 10:1115330. doi: 10.3389/fphy.2022.1115330
Received: 03 December 2022; Accepted: 20 December 2022;
Published: 06 January 2023.
Edited by:
Bofeng Zhu, Nanyang Technological University, SingaporeReviewed by:
Wenlong Tian, Xidian University, ChinaDai Tongyu, Harbin Institute of Technology, China
Copyright © 2023 Fan, Liu, Zhang, Meng, Long and Bai. This is an open-access article distributed under the terms of the Creative Commons Attribution License (CC BY). The use, distribution or reproduction in other forums is permitted, provided the original author(s) and the copyright owner(s) are credited and that the original publication in this journal is cited, in accordance with accepted academic practice. No use, distribution or reproduction is permitted which does not comply with these terms.
*Correspondence: Zhongwei Fan, ZmFuemhvbmd3ZWlAYW9lLmFjLmNu; Zhenao Bai, YmFpemhlbmFvQGhvdG1haWwuY29t